INTRODUCTION
Several studies have summarized the sparse stratigraphic and paleoenvironmental records from eastern Beringia (the unglaciated regions of Alaska, USA, and Yukon, Canada) that date beyond the Marine Oxygen Isotope Stage (MIS) 2 (29,000–14,000 cal yr BP; Lisiecki and Raymo, Reference Lisiecki and Raymo2005), or the last glacial maximum (LGM), noting that past climate and environmental records dating beyond 20,000 cal yr BP remain an enigmatic sketch (Anderson and Lozhkin, Reference Anderson and Lozhkin2001; Elias, Reference Elias2001; Bigelow, Reference Bigelow and Elias2007; Kurek et al., Reference Kurek, Cwynar, Ager, Abbott and Edwards2009; Bigelow et al., Reference Bigelow, Zazula, Atkinson and Elias2013). Few continuous sedimentary and soil stratigraphic records in eastern Beringia extend back beyond 30,000 cal yr BP and into the MIS 3 interstade (57,000–29,000 cal yr BP; Lisiecki and Raymo, Reference Lisiecki and Raymo2005), a period of reduced ice sheets occurring between major Wisconsinan glaciations. The MIS 3 interstade is a particularly poorly understood climatic interval in Beringia (Anderson and Lozhkin, Reference Anderson and Lozhkin2001; Lozhkin and Anderson, Reference Lozhkin and Anderson2011).
The regions of eastern Beringia that were outside the extent of the MIS 2 glaciation have the potential to contain deposits that date to the MIS 3 interstade and earlier stages, as these were areas least affected by the erosional nature of glacial processes. In addition, many regions of eastern Beringia lack records of full glacial MIS 2 sediments (Muhs et al., Reference Muhs, Ager, Been, Bradbury and Dean2003; Zazula et al., Reference Zazula, Froese, Elias, Kuzmina and Mathewes2007). Within the region of western Alaska described in this report, isolated, rugged mountain ranges such as the Horn, Russian, Kiokluk, and Chuilnik mountains experienced multiple periods of Pleistocene glaciation, but ice accumulations were generally restricted to the cores of those respective upland areas (Kline and Bundtzen, Reference Kline, Bundtzen, Hamilton, Reed and Thorson1986; Bundtzen et al., Reference Bundtzen, Harris, Miller, Layer and Laird1999). Hence, western Alaska is a region where much of the land was far beyond the limits of late Quaternary glaciations (Briner et al., Reference Briner, Tulkeno, Kaufman, Young, Baichtal and Lesnek2017), and the varied topography holds areas where deposits of MIS 2 age and older are likely to be preserved. This region was once part of the highlands of eastern Beringia, at its interface with central Beringia and the Bering Land Bridge, where glacial extent was restricted during MIS 2–4 (71 ka to 14,000 cal yr BP), the Wisconsinan glaciations, and the region was repeatedly linked to northeastern Asia via the Bering Land Bridge during low stands of sea level resulting from continental ice caps and extensive alpine glaciation (Hopkins, Reference Hopkins1973; Ager, Reference Ager, Hopkins, Matthews, Schweger and Young1982). These areas served as corridors both for colonization of Asiatic biota from the Bering Land Bridge to other parts of interior and southern Alaska, as well as movement of North American taxa into Asia (Hopkins et al., Reference Hopkins, Matthews, Schweger and Young1982; Elias et al., Reference Elias, Short, Nelson and Birks1996; Cook et al., Reference Cook, Hoberg, Koehler, Henttonen, Wickström, Haukisalmi and Galbreath2005). However, this region has comparatively little research published on late Quaternary sedimentary and soil sequences and paleoenvironmental records compared to other regions of eastern Beringia (Reuther et al., Reference Reuther, Rogers, Roussaeu and Druckenmiller2015). This paper presents radiocarbon and tephrochronological data from three stratigraphic sequences from the central Kuskokwim River region that transgress the MIS 3 interstade, and potentially older, to MIS 1 (14,000 cal yr BP to present) and the Holocene.
BACKGROUND
Our study area is situated along the central portion of the Kuskokwim River in western Alaska, between two of its tributaries, the George and Kolmakof rivers (Fig. 1). This stretch of the river transects the southwestern extent of the Kuskokwim Mountains, with the lower river valley basin between 40 and 75 m above sea level (m asl) in elevation, rounded ridges averaging about 500 m asl, and the rugged Horn and Russian mountains reaching maximums of 938 and 1072 m asl, respectively. The adjacent Holitna and Upper Kuskokwim lowlands, to the east of the study area, are broader sediment-filled basins between 70 and 110 m asl.
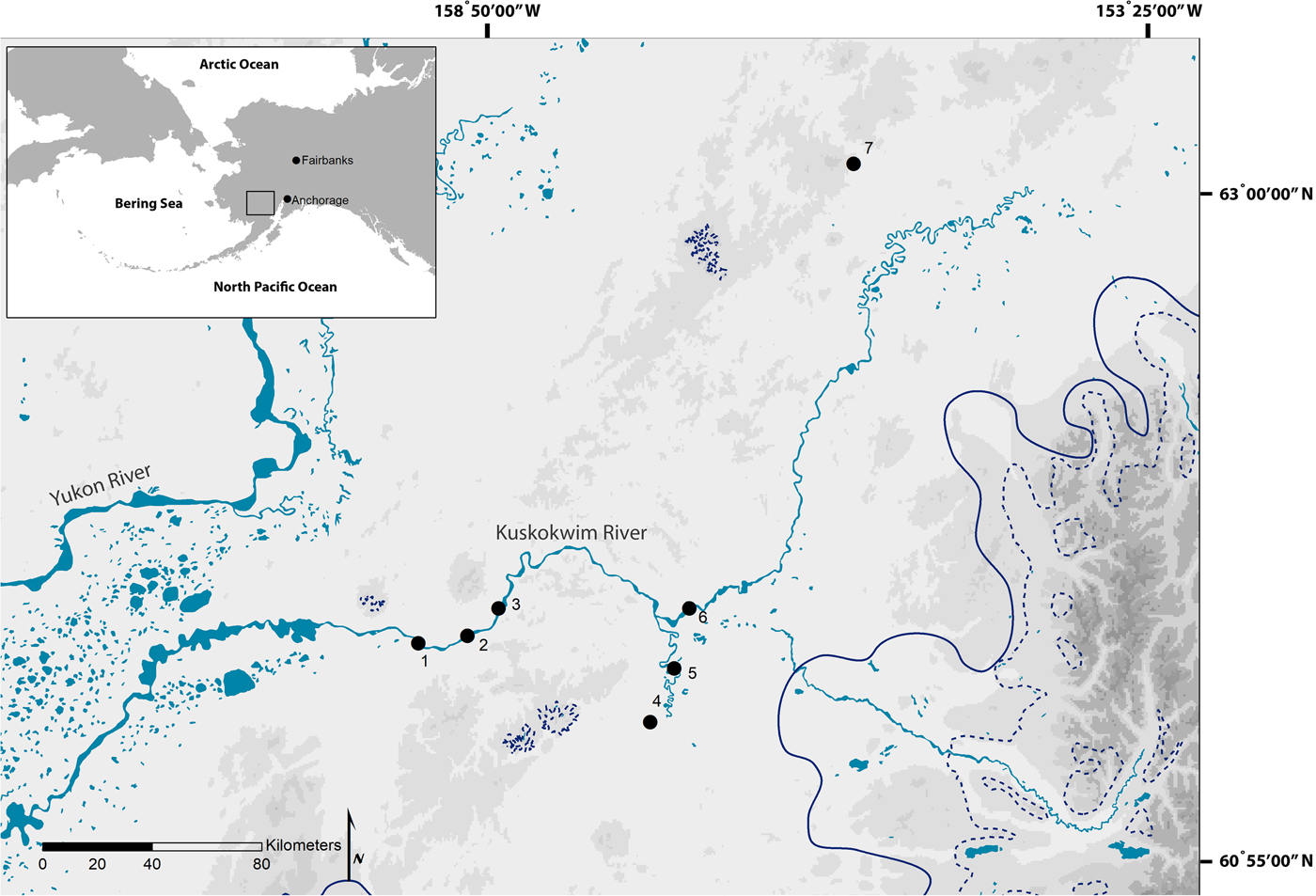
Figure 1. (color online) Locations of the study area, extent of late Quaternary glaciations, and geological sections mentioned in the text. Study areas: (1) Kolmakof section, (2) VABM Kuskokwim section, (3) Sue Creek section, (4) Kulukbuk Bluffs, (5) Beaverhouse Hill, (6) Inowak Bluff site, and (7) the Colorado Creek site. Pleistocene glacial maximum extent (solid line); late Wisconsin glacial extent (dashed line; Kaufman et al., Reference Kaufman, Young, Briner, Manley, Ehlers, Gibbard and Hughes2011).
Although most of the central Kuskokwim drainage has remained unglaciated, four localized Pleistocene glaciations and associated outwash deposits are known and form a radial pattern around the Horn and Russian Mountains uplands. Intensive MIS 6 (Illinoian, 191 to 131 ka) and MIS 2–4 (Wisconsinan) glaciations occurred in the Alaska Range to the east (Fig. 1). As a consequence of Alaska Range glaciation, and localized alpine glaciations in the Horn and Russian Mountains, vast amounts of unconsolidated aeolian sand and silt from outwash rivers were deposited in and blanketed periglacial zones during the late Pleistocene (Cady et al., Reference Cady, Wallace, Hoare and Webber1955; Waythomas, Reference Waythomas1990; Bundtzen et al., Reference Bundtzen, Harris, Miller, Layer and Laird1999).
Waythomas (Reference Waythomas1990), Hoffecker and Waythomas (Reference Hoffecker and Waythomas1991), and Thorson and Guthrie (Reference Thorson and Guthrie1992) described and provided chronologies for three sedimentary and soil sequences from the Upper Kuskokwim and Holitna lowlands that date at least back to the MIS 2 stade and MIS 3 interstade: the Beaverhouse Hill, Inowak Bluff, Kulukbuk, and Colorado Creek sections (Fig. 1 and 2). The Kulukbuk section has deposits that date back to the last interglacial MIS 5 (130 to 71 ka) or late stages of the MIS 6 glacial period based on the presence of the 124 ka Old Crow tephra (Waythomas, Reference Waythomas1990; Preece et al., Reference Preece, Pearce, Westgate, Froese, Jensen and Perkins2011).
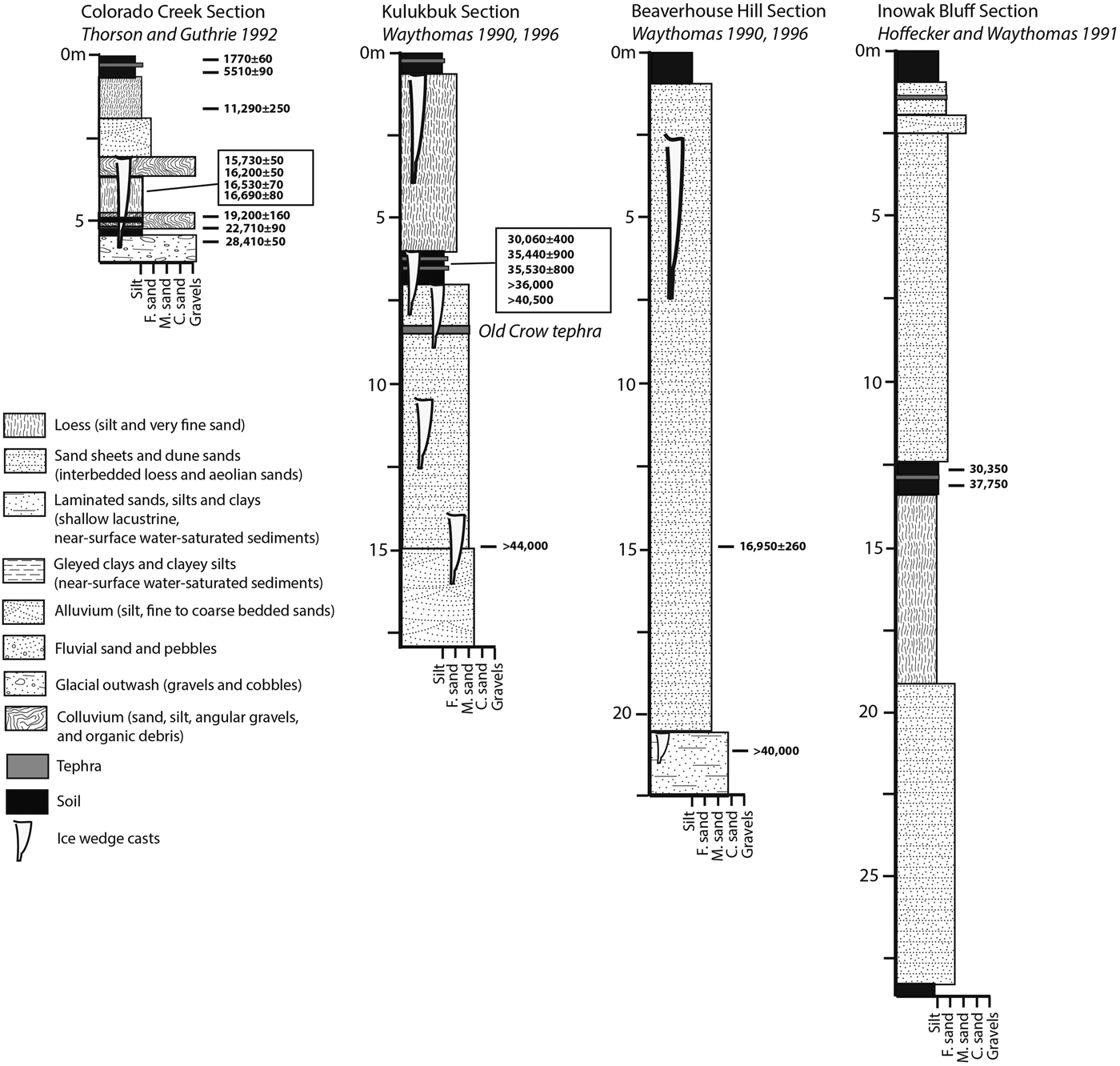
Figure 2. Stratigraphic profiles of the Beaverhouse Hill, Kulukbuk, Inowak Bluff, and Colorado Creek sections. Sections adapted from Waythomas (Reference Waythomas1990, Reference Waythomas and West1996), Hoffecker and Waythomas (Reference Hoffecker and Waythomas1991), and Thorson and Guthrie (Reference Thorson and Guthrie1992). Radiocarbon ages (14C yr BP) are quoted in bold; tephra samples labeled in italics.
In the higher elevations of the Kuskokwim Mountains north of the Kuskokwim River, Kline and Bundtzen (Reference Kaltenrieder, Tinner, Lee and Hu1986) and Bundtzen et al. (Reference Bundtzen, Harris, Miller, Layer and Laird1999) provide limited radiocarbon age determinations of two glacial stades: the Late Wisconsinan Tolstoi Lake glaciation (~16,300 cal yr BP, or 13,550 14C yr BP) and Early Holocene Crater Mountain glaciation (~8400 cal yr BP, or 7,660 14C yr BP). Older glaciogenic deposits have not been dated. Bundtzen et al. (Reference Bundtzen, Harris, Miller, Layer and Laird1999) also provided brief descriptions and the initial radiocarbon chronology of three stratigraphic sequences—the Kolmakof, VABM Kuskokwim, and Sue Creek sections—along the central portion of the Kuskokwim River (Fig. 1 and 3). This initial study established the presence of MIS 2 and MIS 3, and potentially older, deposits.

Figure 3. (color online) Photographs of the (a) Kolmakof, (b) VABM Kuskokwim, and (c) Sue Creek sections. Photos courtesy of Northern Land Use Research Alaska.
METHODS
Field sampling
In 2013, we surveyed between the George and Kolmakof rivers for deposits with an antiquity beyond 30,000 cal yr BP (Fig. 1; Reuther et al., Reference Reuther, Druckenmiller, Rogers, Bundtzen, May and Bowman2014). The majority of the exposures we examined are at the edges of fluvial and alluvial terraces and glacial outwash fans that were dissected by the Kuskokwim River and its tributaries. Exposure heights range between 3 and 20 m and lengths can extend laterally for distances over 1.6 km.
We employed a trenching strategy across each exposure's face that aimed to uncover and document the intact stratigraphy of the deposits, capture the variability of the sediment and soil stratigraphy, episodes, and origins of deposition, and identify any unconformities indicating periods of erosion. Trenches were excavated with shovels, with trench widths ranging between 1 and 2.5 m. The number of trenches placed at any given section depended on the height, length, and variability of the deposits. Several of the trenches vertically overlapped, creating a continuous stratigraphic record from the vegetative surface to the river.
At several sections, substantial amounts of upper sediments were slumped over and buried underlying sediments across vast portions of the exposures. In some cases, trenching had to be offset both horizontally and vertically due to blocks of slumped sediment that were overly thick and cumbersome to dig through to intact underlying deposits (Fig. 3). At many of the exposures, slumped sediments did not hamper digging through to unmodified primary stratigraphy; however, in the case of Sue Creek (described below), slumped sediments did present a problem in our attempts to develop complete stratigraphic sequence at this section.
Bulk sediment samples were taken from stratigraphic layers and horizons to sieve for organic materials for radiocarbon dating, and conduct geochemical analyses on tephras (volcanic ash). In some cases, single wood, charcoal, and mollusk shells were sampled directly in the field from in situ deposits. A sample for luminescence dating was taken from a tephra unit at the VABM Kuskokwim section (see Supplementary Materials). Large mammal remains were also collected when encountered during trenching.
Laboratory methods
Bulk sediment samples were sieved through a series of nested sieve sizes (250 to 63-μm mesh). Filtrated and deionized water was used to separate lighter plant remains from heavier sediments; mollusk shells were separated by hand from sediment. We focused on submitting materials from soil matrices that represent more stable surfaces that likely have minimal reworking of materials and deposits, rather than isolated plant bits and charcoal in alluvial and aeolian deposits where older organics may be reworked into younger sediments.
Sample pretreatments and accelerator mass spectrometry (AMS) radiocarbon measurements were performed at the Center for Applied Isotope Studies at the University of Georgia. Charcoal, wood and plant samples were treated with 5% HCl at a temperature of 80°C for 1 hour, then washed with deionized water on a fiberglass filter and rinsed with diluted NaOH to remove any potential contamination by humic acids. The samples were subsequently treated with diluted HCl, washed with deionized water, and dried at 60°C. The cleaned samples were combusted at 900°C in evacuated and sealed ampoules in the presence of CuO.
Mammoth ivory samples were treated with 1N HCl to dissolve the mineral component, rinsed with Milli-Q water, and subsequently treated with a 0.1M NaOH solution to remove potential humic acid contaminants. The samples were rinsed with Milli-Q water, and then treated with 1N HCl. The residues were filtered and rinsed with deionized water, and heated at 80°C for 6 hours under slightly acid conditions (pH = 3) to dissolve the collagen into a solution. This collagenous solution was filtered to isolate pure collagen and then freeze-dried. The collagen was combusted in sealed and evacuated ampoules in the presence of CuO at a temperature of 575°C.
The shell sample was treated with diluted HCl to remove any surface contaminants, then washed with deionized water and dried. The cleaned sample was then treated in the vacuum with concentrated H3PO4 to recover carbon dioxide.
For all samples, the carbon dioxide was cryogenically purified from other reaction products and catalytically converted to graphite using the method of Vogel et al. (Reference Vogel, Southon, Nelson and Brown1984). Graphite 14C/13C ratios were measured using a CAIS 0.5 MeV accelerator mass spectrometer. The sample ratios were compared to the ratio measured from the Oxalic Acid I (NBS SRM 4990). All radiocarbon ages are quoted as uncalibrated dates with an error of one standard deviation (1σ), and expressed as radiocarbon years before 1950 (BP) using the 14C half-life of 5568 yr. The sample 13C/12C ratios were measured (with an error of less than 0.1‰) separately using a stable isotope ratio mass spectrometer and expressed as δ13C with respect to the PDB, and all ages have been corrected for isotope fractionation. Ages that are near the detection limits of radiocarbon were corrected for background variance (Cherkinsky et al., Reference Cherkinsky, Culp, Dvoracek and Noakes2010).
Radiocarbon ages are reported as finite ages by CAIS when they are statistically indistinguishable from background values at a 95.4% confidence interval (following Stuiver and Polach [Reference Stuiver and Polach1977]). Ages that are statistically similar to background values are considered infinite ages. In the Supplementary Materials, we provide statistical comparisons between radiocarbon ages and background values from CAIS to show which ages we consider finite and which we consider infinite because several of the ages from this study are near the upper limits for finite ages (60,000 to 40,000 14C yr BP; Taylor and Bar-Yosef, Reference Taylor and Bar-Yosef2014).
The Beta Analytic ages from Bundtzen et al. (Reference Bundtzen, Harris, Miller, Layer and Laird1999) are radiometric ages that have larger standard deviations, and are very close to the limits of radiocarbon detection (35,000 to 40,000 BP) for most labs during the 1990s. Many of these samples were composed of bulk organics and sediment, materials that have been continually shown to be fraught with problems in removing younger contaminants (Holliday, Reference Holliday2004). We do not have background values for the Beta Analytic ages from the Bundtzen et al. (Reference Bundtzen, Harris, Miller, Layer and Laird1999) study and cannot assess whether they are finite or infinite ages, but we assume that Beta followed the same standard procedures for defining finite and infinite ages. We are unable to reject these ages as unreliable given the information that we have, and include them in our discussion.
Tephra samples were processed for geochemical analysis in the United States Geological Survey (USGS) Alaska Tephra Laboratory and Data Center in Anchorage, Alaska and elemental concentrations were measured on the electron probe microanalysis (EPMA) at the USGS in Menlo Park, California. A tephra sample containing feldspar grains from the VABM Kuskokwim section was luminescence dated at the University of Washington Luminescence Lab. The laboratory procedures for the tephra geochemical analyses and the infrared-stimulated luminescence (IRSL) dating are described in the Supplementary Materials.
RESULTS
While numerous geologic sections along the central Kuskokwim River were investigated, many were Holocene in age (Reuther et al., Reference Reuther, Druckenmiller, Rogers, Bundtzen, May and Bowman2014). Here, we describe three sections with stratigraphic sequences that are greater than 24,000 cal yr BP (20,000 14C yr BP) in age: the VABM Kuskokwim, Kolmakof, and Sue Creek sections. Bundtzen et al. (Reference Bundtzen, Harris, Miller, Layer and Laird1999) reported brief field stratigraphic descriptions and chronologies for these sections. We provide more refined descriptions and chronologies with updated information from our 2013 survey. Sediment and soil descriptions for each section are detailed in the Supplementary Materials. Hopkins (Reference Hopkins, Hopkins, Matthews, Schweger and Young1982) provided a standard nomenclature of Wisconsin stadial and interstadial periods for Beringia: the Happy (MIS 4 stade), Boutellier (MIS 3 interstade), Duvanny Yar (MIS 2 stade), and Birch (the early part of MIS 1) intervals. We restrict our use of terminology to the MIS. Radiocarbon data are summarized in Table 1.
Table 1. Radiocarbon dates from the Kolmakof, VABM Kuskokwim, and Sue Creek sections.

a Calibrations were conducted using Calib v7.1 software (Stuiver et al., Reference Stuiver, Reimer and Reimer2013) and the IntCal13 terrestrial calibration model (Reimer et al., Reference Reimer, Bard, Bayliss, Beck, Blackwell, Bronk Ramsey and Buck2013).
b These ages are considered infinite ages within our study (see the Radiocarbon section in the Supplemental Materials for details): 52250 ± 520 14C yr BP (UGAMS#15795), 53110 ± 630 14C yr BP (UGAMS#15794), 52120 ± 920 14C yr BP (UGAMS#16737), and 50680 ± 830 14C yr BP (UGAMS#16488).
c These ages from Bundtzen et al. (Reference Bundtzen, Harris, Miller, Layer and Laird1999) were not used in our study because of a lack of correlation to the 2013 stratigraphy and are not shown in Figure 4.
VABM Kuskokwim section
The VABM Kuskokwim section is a 15-m-high exposure along the right side of the Kuskokwim River, approximately 8.8 km downriver from the mouth of the Oskawalik River (Fig. 1). The exposure stretches for 1.8 km along the riverbank, and displays an impressive sequence of aeolian deposits that cover near-surface, water-saturated (shallow lacustrine [lake and pond deposits] or slow-moving alluvial) deposits, and glaciofluvial sand and gravel from glacial outwash. This section is the location of finds of mammoth and bison remains dating back to and beyond 40,600 cal yr BP (or 36,000 14C yr BP; Reuther et al., Reference Reuther, Rogers, Roussaeu and Druckenmiller2015). Four trenches were placed across a 25 m segment of the exposure with nearly 12 m of vertical stratigraphy exposed (Fig. 3).
Very poorly sorted angular gravel and coarse-to-medium sand were the deepest deposits described at this section, and represent outwash fan deposits from a glacial event in the Horn Mountains (Fig. 4). Bundtzen et al. (Reference Bundtzen, Harris, Miller, Layer and Laird1999) previously reported infinite radiocarbon ages of ≥43,500–42,980 cal yr BP (≥39,600 14C yr BP; Beta-47367) and ≥42,820–42,380 cal yr BP (≥38,600 14C yr BP; Beta-47638) for these deposits. Bundtzen et al. (Reference Bundtzen, Harris, Miller, Layer and Laird1999, p. 19) noted a “bluish organic clay” and “peat layer” interspersed within the lower gravel where these ages came from.

Figure 4. Stratigraphic profiles of the of the Kolmakof, VABM Kuskokwim, and Sue Creek sections. Radiocarbon ages (14C yr BP) are quoted in bold; tephra samples labeled in italics. ** These ages are considered infinite ages within our study (see the Radiocarbon section in the Supplementary Materials for details).
AMS dates on collagen from mammoth tusks embedded in the gravel date to ≥53,110 14C yr BP (UGAMS#15795) and ≥52,250 14C yr BP (UGAMS#15794). Bundtzen et al. (Reference Bundtzen, Harris, Miller, Layer and Laird1999) observed a tephra (sample 91BT6a) within the gravel and believed it related to the Old Crow tephra that dates to 124 ± 10 ka, based on glass-fission track ages, in the last stages of MIS 6 glaciation (Preece et al., Reference Preece, Pearce, Westgate, Froese, Jensen and Perkins2011). However, the geochemistry of the 91BT6a tephra sample was not compared to known Old Crow samples. This tephra was not recognized in the 2013 trenches and could not be resampled for geochemical comparisons. The ages of ≥53,110 and ≥52,250 14C yr BP and their infinite values are consistent with the potential presence of an Old Crow tephra layer dating to 124 ± 10 ka in this deposit.
Alternating deposits of clayey silt and sand overlie the lower glacial outwash gravel. These deposits grade from a poorly to moderately sorted mixture of coarse sand and gravel to finer deposits of clayey silt and finer sand with lesser amounts of gravel. They show minimal input by glacial outwash, most likely developing after glacial recession was initiated, and display a possible mixture of aeolian and alluvial deposits indicative of lower-energy depositional environments. A moderately to well-sorted, fine sand bed represents aeolian deposited sediments overlying these deposits. A buried soil (organic-rich, dark reddish-brown silt loam) developed at the top of this fine sand bed, indicates a period of landform stability and very low sediment accumulation and/or erosion.
A series of clayey silts with interbedded fine sands overlie the aeolian fine sand bed and buried soil. These clayey silts show gleying, oxidization, and iron accumulation, and may have be the product of aeolian silt accumulating in a near-surface, water-saturated environment such as a shallow pond or wetland (Fig. 4). Fine sand beds are relatively thin (0.5 to 5 cm thick) and may mark periods of increased wind energy that mobilized fine sand particles. Sediments are turbated within the middle of the deposit, possibly relating to minor ice-wedge development.
A prominent erosional contact is present between the gleyed and highly oxidized clayey silt and overlying loess and aeolian fine sand. A possible whitish tephra bed (not collected) is present in the clayey silt immediately below the erosional contact. The timing of this erosional period is unclear but occurred prior to 41,850–36,450 cal yr BP (34,650 ± 1,240 14C yr BP; Beta-47636), based on radiocarbon dates from organics in the overlying massive loess deposit (Bundtzen et al., Reference Bundtzen, Harris, Miller, Layer and Laird1999).
The interbedded aeolian silt and fine-to-medium sand beds (loess and sand sheet accumulations) comprise over 5 m of deposits that overlie the erosional contact. Weakly developed (<0.5 to 4 cm thick) soils formed during periods of relatively lower sediment accumulation and landform stability. However, during this period, aeolian sediment accumulation appears to overwhelm the development of more substantial soils.
A 5 to 6 m thick massive loess deposit caps the lower interbedded aeolian silts and sand sheets. A series of 6 to 7 thicker buried soils are present in the middle and upper portions of the massive loess deposits and developed between 48,660 ± 420 14C yr BP (UGAMS#32334) and 13,960–13,850 cal yr BP (11,930 ± 40 14C yr BP; UGAMS#16740).
Ice-wedge casts are present below a soil dated to 28,900–26,980 cal yr BP (23,660 ± 510 14C yr BP; Beta-47634), as well as the 13,960–13,850 cal yr BP (11,930 14C yr BP) soil, and seem to disappear after 13,960–13,850 cal yr BP at this section.
A boreal forest soil (O-A-B horizons; inceptisol) has developed at the surface of these massive loess deposits. A volcanic ash deposit (sample NLUR-TEPHRA-13-41) is present below the surficial soil and in the upper 20–30 cm of the loess. This tephra bed was geochemically correlated to proximal source samples related to the Aniakchak eruption that occurred on the Alaska Peninsula at ~3950 cal yr BP (or ~3645 14C yr BP; Begét et al., Reference Begét, Mason and Anderson1992; Pearce et al., Reference Pearce, Westgate, Preece, Eastwood and Perkins2004). Loess deposition diminished before ~3950 cal yr BP, allowing for the surficial surface soils to develop.
Kolmakof section
The Kolmakof section is an approximately 25-m-high and 1.6-km-long exposure on the left bank of the Kuskokwim River, approximately 1 km downstream from the village of Napaimute (Fig. 1). Four trenches were excavated across a 40 m span of this section, and exposed 21 vertical meters of stratigraphy of the 25-m-high exposure.
The deepest deposits identified at this location consist of subrounded to rounded gravel and sand, interpreted as fluvial deposits related to a bed of the ancestral Kuskokwim River (Bundtzen et al., Reference Bundtzen, Harris, Miller, Layer and Laird1999). Over 6 m of gleyed clay and clayey silt overlies these fluvial gravel and sand deposits (Fig. 4). Buried soils (possibly peats) that are highly organic are present in the gleyed clay and clayey silt, with a few having moderately decomposed plant materials still present. A highly compacted, organic and iron-rich buried soil near the base of the clay and clayey silt deposits was dated to ≥52,120 14C yr BP (UGAMS#16737).
A second prominent compacted, organic, and iron-rich, buried soil, or set of soils, lies in the middle of the deposits and dates between 49,780–47,240 cal yr BP (45,130 ± 510 14C yr BP; UGAMS#17195) and 49,320–47,160 cal yr BP (44,980 ± 380 14C yr BP; UGAMS#17194). The part of the Kolmakof section where this soil is most expressed shows either one single soil that is bifurcated by a 25-cm-thick tephra deposit, or a set of buried soils that are 42 and 23 cm thick that are separated by this tephra. This tephra (sample NLUR-TEPHRA-13-31) was not documented in other trenches along the section and may have been removed through localized ancient erosional events, or turbated within other sedimentary matrices obscuring its presence as a primary deposit. Feldspar from a sample of this tephra bed was dated by infrared-stimulated luminescence (IRSL) to estimate the timing of the volcanic ash's exposure and burial. The IRSL age estimate is 26.7 ± 1.7 ka, a substantially younger estimate, by nearly 20,000 yr, than the bracketing radiocarbon ages indicate.
The UGAMS#17194 and UGAMS#17195 dates are statistically distinguishable from background values and we considered them to be finite ages (see Supplementary Materials). We consider the radiocarbon ages to accurately reflect the age of the tephra deposition as being deposited around 48,000 cal yr BP. The geochemistry of this tephra bed could not be correlated with other known tephra deposits, both distal and proximal, including the Old Crow tephra (see Supplementary Materials).
A third, but less prominent, soil lies at the top of the clay and clayey silt deposits, just below an erosional unconformity, and was dated to 40,530–39,570 cal yr BP (35,460 ± 170 14C yr BP; UGAMS#16736). These clay and clayey silt deposits and soils indicate organic development within a slow accumulation of aeolian silt in a near-surface, water-saturated environment, such as a shallow pond or wetland, during the MIS 3 interstade.
A 5-m-thick deposit of interbedded aeolian silt and fine sand beds (loess and sand sheets) covers this prominent soil, marking a shift to a more arid climate and a geological environment dominated by aeolian deposition. A 2-m-thick bed of laminated sand and clayey silt that are lightly gleyed and containing thin layers of oxidized fibrous organic materials covers these interbedded aeolian silt and fine sand beds. A fingernail clam shell (Sphaeriidae) recovered from these laminated sand and clayey silt deposits was radiocarbon dated to 24,240–23,810 cal yr BP (19,960 ± 60 14C yr BP; UGAMS#16735). The presence of these clams indicates a localized, near-surface, water-saturated environment at the Kolmakof section during the MIS 2 stade.
A nearly 5-m-thick unit of massive loess covers the lower interbedded aeolian silt and fine sand beds. An upper unit of interbedded aeolian silt and sand overlies the massive loess unit. Buried soils are present throughout the loess and sand sheet deposits and are very weakly expressed (<3 cm thick).
Soil development was likely hampered by the high rates of aeolian sediments accumulating on the landscape that covered and outcompeted substantial vegetative growth. Aeolian sand and silt accumulation appeared to continue throughout this period but likely at lower rates than in prior and later periods. Bundtzen et al. (Reference Bundtzen, Harris, Miller, Layer and Laird1999) reported a radiocarbon date of 20,960–19,180 cal yr BP (16,640 ± 360 14C yr BP; Beta-47643) from a soil within the loess and directly below the upper unit of interbedded aeolian silt and sand. The loess unit accumulated during the later stages of the MIS 2 stade, while the upper interbedded aeolian silt and sand was deposited in later stages of the MIS 2 stade and into the MIS 1 warming period. A boreal forest soil (O-A-B horizons; inceptisol) developed at the surface of these the upper interbedded aeolian silt and sand deposits.
Sue Creek section
The Sue Creek section is a roughly 18-m-high exposure along the right bank of the Kuskokwim River, approximately 2 km downriver from the mouth of the Sue Creek (Fig. 1). This exposure extends approximately 1.8 km along the river's edge. Three trenches were excavated over a 12 m segment of the Sue Creek exposure, allowing us to describe nearly 8 m of this section's lower stratigraphy (Fig. 3). Our 2013 trenches appear to be in the lower 5–10 m of this section's stratigraphic column characterized by Bundtzen et al. (Reference Bundtzen, Harris, Miller, Layer and Laird1999; Fig. 4). Therefore, we did not expose the upper 7 m of vertical stratigraphy that represents the last 40,000 to 50,000 cal yr BP of deposition and soil development at this locale. Here, we combine the characterization of the Sue Creek section's upper stratigraphy by Bundtzen et al. (Reference Bundtzen, Harris, Miller, Layer and Laird1999) and the 2013 descriptions of its lower stratigraphy in our stratigraphic interpretations.
The Sue Creek section, like the VABM Kuskokwim section, has a thick cap of aeolian deposits that cover shallow lacustrine, alluvium, and glaciofluvial sand and gravel from glacial outwash. Very poorly sorted, subrounded to subangular gravel and cobbles are the deepest deposits recognized at the Sue Creek section (Bundtzen et al., Reference Bundtzen, Harris, Miller, Layer and Laird1999). These gravels represent outwash from a glacial event in the Horn Mountains.
The Sue Creek section provides minimum ages of ≥50,680 14C yr BP (UGAMS#16488) to 44,980–43,590 cal yr BP (40,780 ± 330 14C yr BP; UGAMS#16491) for the formation of these deposits. Bundtzen et al. (Reference Bundtzen, Harris, Miller, Layer and Laird1999) originally recorded a minimum age of ≥40,920 14C yr BP (Beta-47641) from 30 cm above the gravels, and recognized a tephra deposit (sample 91BT32c) at the top of this gravel that they tentatively correlated, based on major oxide composition, to the Old Crow tephra. If this tephra is a primary deposit of Old Crow tephra, then the glacial outwash deposition occurred ca. 124 ± 10 ka, based on glass-fission track ages, in the last stages of MIS 6 glaciation (Preece et al., Reference Preece, Pearce, Westgate, Froese, Jensen and Perkins2011). If the tephra is reworked into glacial deposits, however, this would represent a much later depositional event, which is discussed below.
Bundtzen et al. (Reference Bundtzen, Harris, Miller, Layer and Laird1999) described fluvial sand and pebbles, similar to the lowest fluvial deposits at the Kolmakof section, as overlying the lowest tephra at Sue Creek. Unfortunately, we could not obtain the original tephra sample (91BT32c), or a sample from field deposits, to confirm this tephra's correlation to the Old Crow tephra.
Interbedded fine sand, silt, and clayey silt overlie the glacial outwash, and likely represent shallow lacustrine deposits or near-surface, water-saturated environments with minor contributions of aeolian silt and sand. Thin (1–3 cm thick), dark reddish-brown, organic-rich silt represents buried soils that developed at the top of sand beds. Charcoal fragments were recovered from two of these buried soils and radiocarbon dated to 44,980–43,590 cal yr BP (40,780 ± 330 14C yr BP; UGAMS#16491), 46,290–44,930 cal yr BP (42,320 ± 360 14C yr BP; UGAMS#16490) and 47,770–46,200 cal yr BP (43,770 ± 250 14C yr BP; UGAMS#17410). These radiocarbon dates appear as an age reversal in the stratigraphy, which we discuss further below.
Similar to the Kuskokwim section, loess and sand sheets appear to overlie shallow lacustrine deposits or near-surface, water-saturated deposits. Nearly 7 m of aeolian silt and sand deposits cover the lower lacustrine and gravel deposits. Several buried soils were identified in the loess and sand deposits. These soils range from very weakly formed (<1 cm thick) organic stringers to thicker (3–5 cm thick), more developed, and platy silt loams that present plant and wood macrofossils. Wood fragments from the more developed buried soils within the loess and sand sheet deposits were radiocarbon dated to 46,190 ± 550 14C yr BP (UGAMS#16492), 49,490 ± 850 14C yr BP (UGAMS#16489), and ≥50,680 14C yr BP (UGAMS#16488) within the early stages of the MIS 3 interstade. Age reversals occur again within this unit and between the assumed underlying interbedded fine sand, silt, and clayey silt deposits. We discuss these age reversals more in depth in our Discussion section below.
A thin, pale brown tephra (sample NLUR-TEPHRA-13-36) is present in loess around 80 cm below the ~50,000 14C yr BP soils, and above the ~46,190 14C yr BP soil and the anomalously young 44,980–43,590 and 46,290–44,930 cal yr BP (40,780 and 42,320 14C yr BP) dates from soils in the lower near-surface water deposits. This tephra was most likely deposited during the MIS 3 interstade. A correlation of this tephra to known proximal and distal tephras remains unclear. The closest geochemical similarities occurred with a discontinuous tephra deposit, the SD tephra (UT743), at the Halfway House section near Fairbanks, northeast of the Kuskokwim River, that likely dates older than 50,000 BP but younger than 124 ka Old Crow tephra (Preece et al., Reference Preece, Westgate, Stemper and Péwé1999; Jensen et al., Reference Jensen, Evans, Froese and Kravchinsky2016). The geochemical similarities between the two tephras, however, are not statistically significant.
Bundtzen et al. (Reference Bundtzen, Harris, Miller, Layer and Laird1999:18–19) described the sediments and soils in the upper 7–8 m of the stratigraphic column, overlying the 40,000-yr-old deposits described above. Sand sheets, dune deposits, and loess characterize these upper deposits. Three buried soils are present above sand sheets in the upper 3 m of loess deposits at the Sue Creek section. The lowest and oldest of these soils was dated to 31,300–29,390 cal yr BP (26,400 ± 530 14C yr BP; Beta-47642), within the later stages of the MIS 3 interstade near its transition into MIS 2. The upper most soils were dated to 20,150–19,400 cal yr BP (16,380 ± 150 14C yr BP; Beta-47640) and 13,730–13,440 cal yr BP (11,730 ± 70 14C yr BP; Beta-47639), and are likely similar to the relatively well-developed series of soils in the upper 6 m of the loess deposits of the Kuskokwim section that date to later stages of the MIS 2 stade and the warmer climates of the Bølling-Allerød period (15,500–12,800 cal yr BP; Mangerud et al., Reference Mangerud, Andersen, Berglund and Donner1974) into the early stages of MIS 1.
DISCUSSION
The stratigraphic transitions of the Kolmakof, Sue Creek, and VABM Kuskokwim sections comprise sediment and soil sequences that date back to at least MIS 3, and conceivably to older MIS periods, in an area at the fringe of central and eastern Beringia that has very little published paleoenvironmental records that extend beyond MIS 2.
MIS 3 (57,000–29,000 cal yr BP) and older deposits
The potential presence of the Old Crow tephra at the Sue Creek and VABM Kuskokwim sections pushes the antiquity of these deposits back to 124 ka and the early stages of MIS 6 glaciation. There may have been an erosional period during the MIS 5 interglaciation and MIS 4 glaciation, as shown in the lack of deposits dating to these stages at other sections in the region, like the Kulukbuk section in the Holitna Lowlands (Waythomas, Reference Waythomas1990, Reference Waythomas and West1996). However, deposits with infinite radiocarbon ages from the sections in this study could also fall within the MIS 5 and MIS 4 periods.
The lower gravel and fluvial sand and pebbles at the Sue Creek and Kuskokwim sections would be equivalent in age to the MIS 6 bedded fluvial sand deposition transition to sand sheet development at the Kulukbuk section. The differences between these sections may be the closer proximity of the Sue Creek and VABM Kuskokwim sections to glaciers, directly downstream and downwind of the Horn Mountains, causing higher fluvial and aeolian energy to deposit larger and heavier clastic materials, while removing the lighter, finer sized constituents.
The VABM Kuskokwim section has radiocarbon ages of ≥53,110 14C yr BP (UGAMS#15795) and ≥52,250 14C yr BP (UGAMS#15794) from mammoth tusk fragments embedded in the gravel, and with a potential deposit of Old Crow tephra. Bundtzen et al. (Reference Bundtzen, Harris, Miller, Layer and Laird1999) also report infinite ages of ≥38,600 and ≥39,600 14C yr BP (Beta-47637 and Beta-47638) from the VABM Kuskokwim section gravel, and ≥40,920 14C yr BP (Beta-47641) just above the gravel at Sue Creek. Therefore, we suggest the VABM Kuskokwim section gravel to be in comparable age to the gravel at the Sue Creek section, and likely older than MIS 3, and of the same glaciofluvial depositional event.
The ages of these gravel deposits were suggested by Bundtzen et al. (Reference Bundtzen, Harris, Miller, Layer and Laird1999) to relate to the MIS 6 Illinoian glaciation based on the presence of Old Crow tephra. The Old Crow tephra has also been identified in the nearby Holitna Lowlands by Waythomas (Reference Waythomas1990) and Waythomas and Walter (Reference Waythomas and Walter1994). If the tephras in the Sue Creek and VABM Kuskokwim gravel deposits are primary tephra deposits, then the age assignment of MIS 6 would be appropriate. We cannot currently discount reworking of Old Crow tephra deposits into younger glaciofluvial deposits, however, which would also suggest that outwash deposition may have occurred between about 50.7 ka and 124 ± 10 ka, at any time between the MIS 5 interglaciation, the MIS 4 glaciation, and early stages of MIS 3, within a highly erosive geological environment.
Based on our radiocarbon analyses, most of the deposits at the Sue Creek, Kolmakof, and VABM Kuskokwim sections appear to date to the MIS 3 interstadial and younger periods. We cannot rule out, however, that some ages near the limit of radiocarbon detection that are reported here as finite and dating to the MIS 3 period were contaminated with minute amounts of younger carbon, and the true values would potentially date to greater than MIS 3 and should be considered infinite radiocarbon ages. Our analysis of radiocarbon assays that date between 40,000 and 55,000 14C yr BP and the MIS 3 in this study (see the Supplementary Materials) showed that four ages in our study dating to 50,000 14C yr BP and older should be considered infinite ages.
Of the 16 radiocarbon ages that date to the MIS 3 period, we consider 13 of these to be finite given that they are statistically younger and do not overlap background values (Table 1; also see Supplementary Materials). We have chosen not to use the ages 43,250–34,410 cal yr BP (34,650 and 33,770 14C yr BP; Beta-47636 and Beta-47635) from Bundtzen et al. (Reference Bundtzen, Harris, Miller, Layer and Laird1999) because we cannot associate them with stratigraphic deposits observed in 2013 (Table 1). A date reversal occurs in the lower portion of the Sue Creek section during the MIS 3 period (Fig. 4). The oldest radiocarbon ages of ≥50,680 and 49,490 14C yr BP (UGAMS#16488 and UGAMS#16489) overly dates of 46,190 14C yr BP (UGAMS#16492), 47,700–46,220 cal yr BP (~43,770 14C yr BP; UGAMS#17410), 46,290–44,930 cal yr BP (~42,320 14C yr BP; UGAMS#16490), and 44,980–43,590 cal yr BP (~40,780 14C yr BP; UGAMS#16491). The radiocarbon age of ≥50,680 14C yr BP (UGAMS#16488) at the Sue Creek section (originally quoted by CAIS as the finite age of 50,680 ± 830 14C yr BP) was statistically distinguishable from its associated background measurement but not from the long-term average of the background measurements (see Supplemental Materials). It is also stratigraphically associated with UGAMS#16489, which is statistically similar to UGAMS#16488 (t = 1.003; χ20.05 = 3.84; df = 1; P = 0.317). UGAMS#16489 is statistically different from its associated background value and the long-term average measurement (Supplementary Table 1), and it does not overlap with the 68.5 and 95.4% ranges for the background measurements (Supplementary Fig. 1 and 2). We suggest that the radiocarbon ages, UGAMS#16488 and UGAMS#16489, may in fact be similar in age with both dating to the MIS 3, even though we have conservatively defined UGAMS#16488 as an infinite age because of its similarity to the long-term background measurement.
There are several possible explanations for the age reversals in the Sue Creek section lower deposits. First, some of the radiocarbon ages may not reflect the “true” ages of the deposits and contamination may be causing them to skew toward younger ages. This can be a particularly acute problem when dealing with materials whose ages are near the detection limit of radiocarbon. Given our analyses in the Supplemental Materials, however, we have no cause other than the age reversals to question the reliability of the radiocarbon age estimates. A second explanation is that large blocks of sediment from upper portions of sections slumped onto lower and older sediments, and the trenching in 2013 may not have excavated thoroughly through the slump blocks to reach more intact stratigraphy in the deeper deposits. Consequently, the younger age reversals in the deeper parts of the section are the result of dating materials from slumped blocks. A third possibility is the reworking of older organics into younger deposits, although we view reworking as less of a possibility given that we selected charcoal, wood, and plant samples from soils that represent more stable surfaces within aeolian silt and fine sand deposits. While reworking is always a possibility in areas where organics have a longer residency (Kennedy et al., Reference Kennedy, Froese, Zazula and Lauriol2010), paleosols in lower-energy aeolian environments should be less conducive to reworking than alluvial or fluvial contexts.
Despite the slumping and age reversals, if the radiocarbon measurements reflect the true ages of the material dated, and not abhorrent younger ages, the dates from the lower portion of the Sue Creek section fall within the MIS 3 interstade and are relatively congruent with most of the dates from the lower stratigraphy at the Kolmakof section and the middle portion of the VABM Kuskokwim section. Future sampling and high resolution dating efforts at these sections may clear up some of these dating discrepancies at the Sue Creek and VABM Kuskokwim sections.
The lowest buried soils at the Kolmakof, Kuskokwim and Sue Creek sections appear similar to the regional complex of soils and tephras defined as the Holitna Complex (Waythomas, Reference Waythomas1990, Reference Waythomas and West1996) dating to the MIS 3 interstade. The thick peat-like soils, gleyed clay, and clayey silt deposits at all of these sections are indicative of moisture-rich substrates with episodic landform stability and soil development, but also show a potentially slow accumulation of aeolian silts during the early part of the interstade between ~49,490 14C yr BP and ~40,050 cal yr BP (35,460 14C yr BP). Beetle remains from the Kulukbuk section dating to around ~39,500 cal yr BP (~35,000 14C yr BP) in the middle of MIS 3 indicate warmer temperatures, only 1–2°degrees cooler than modern temperatures (Elias, Reference Elias and Elias2013).
Charcoal fragments from buried soils at the VABM Kuskokwim and Sue Creek sections dating between 46,900 and 44,300 cal yr BP (43,700 to 40,780 14C yr BP) demonstrate the presence of natural fires during MIS 3 in the central Kuskokwim region. Unburned wood fragments were also found in the Kolmakof, Sue Creek and VABM Kuskokwim dating between ~49,490 14C yr BP and ~40,050 cal yr BP (35,460 14C yr BP). Plant pollen and macrofossil records and beetle remains, from areas outside of the study sections, suggest that widespread tundra, both herbaceous and shrubby tundra communities, covered eastern Beringia, while the presence of spruce appears to be limited to the valley floors (Ager, Reference Ager2003; Bigelow, Reference Bigelow and Elias2007; Bigelow et al., Reference Bigelow, Zazula, Atkinson and Elias2013; Elias, Reference Elias and Elias2013; Willerslev et al., Reference Willerslev, Davison, Moora, Zobel, Coissac, Edwards and Lorenzen2014). Charcoal and wood in the Kuskokwim River sections supports the concept of a vegetation mosaic of herbaceous and shrubby tundra in the region, and in line with an interpretation of increased landscape stability during MIS 3.
Sand sheet formation and higher rates of loess accumulation began as early as 49,490 14C yr BP at Sue Creek, but became the dominant deposits at all sections after ~40,000 cal yr BP (~35,460 14C yr BP). The increased aeolian deposition at the Sue Creek and Kolmakof sections between ~40,000 and 30,500 cal yr BP (~35,460 and 26,400 14C yr BP) is likely correlated to a change to colder and more arid climates in the later period of MIS 3 that continued into MIS 2. Zazula et al. (Reference Zazula, Froese, Elias, Kuzmina and Mathewes2007) also noted the presence of glacial conditions and xeric-adapted plants in the Yukon Territory, Canada, in the later stages of MIS 3 and the transition to MIS 2, which is a likely scenario that happened in the middle Kuskokwim River during this period. Muhs et al. (Reference Muhs, Ager, Been, Bradbury and Dean2003) also record high loess accumulations ~30,000 cal yr BP at the end of MIS 3 at St. Michael, in western Alaska, east of our study area.
In contrast to the aeolian deposition during the later MIS 3 at the Sue Creek and Kolmakof sections, if the 41,850–36,450 and 43,250–34,410 cal yr BP ages at the VABM Kuskowim section are errant, and the middle portion (5–7 m below surface) of the section dates between 48,660 to 23,660 14C yr BP, then either a period of prolonged stability and soil development with a minimal amount of loess deposition occurred. Or more likely some amount of erosion occurred between 48,000 and 23,000 14C yr BP at this section, while aeolian deposition occurred at the other sections, which would also be consistent with a transition to a more arid environment and erosional environment in the later part of MIS 3. The differences among the three sections, however, show significant variability in the depositional sequence with this region for the latter half of the MIS 3 interstade, which is to be expected as landscape become more unstable under harsh climates.
MIS 3 tephra deposits in the Kolmakof and Sue Creek sections appear to be newly identified prehistoric eruptions. Our geochemical analyses could not definitively correlate samples from these beds to other known eruptive events and tephra deposits, either distal or proximal (see Supplementary Materials). Neither of these tephra beds display geochemical similarities to the Old Crow tephra, and thus are not reworked Old Crow tephra deposits. |Waythomas (Reference Waythomas1990, Reference Waythomas and West1996) also identified tephra deposits within the Holitna complex (MIS 3); geochemical analyses were not reported, but the timing of these tephra deposits is similar to those at the Kolmakof and Sue Creek sections. Future geochemical comparisons of the Holitna Lowland, Kolmakof, and Sue Creek tephras may prove fruitful for establishing widespread MIS 3 tephra markers across the central Kuskokwim region. Future sampling of these sections may prove that finite ages in our study should be called into question and considered as infinite ages. As a result, our interpretations of the timing of geological events and paleoenvironmental changes to would need to be reconsidered, especially with dating techniques that can reliably provide ages beyond the detection limits of radiocarbon.
MIS 2 (29,000–14,000 cal yr BP) to MIS 1 (14,000 cal yr BP to present) transitions
At the Sue Creek, Kolmakof, and VABM Kuskokwim sections, massive loess deposition alternates with sand sheet formation throughout the later period of MIS 3, with highest accumulations occurring in the relatively hyper arid climates of MIS 2, and continuing through the warmer periods of the Bølling-Allerød interstadials, and into MIS 1. Thinner and less-developed soils also correlate with the MIS 2 and early MIS 1 loess deposits and sand sheets, and colder and more arid climates. All of the sections record various amounts of loess and aeolian sand sheet accumulation during the height of the LGM and MIS 2. The Kolmakof section shows the highest accumulation of aeolian sediments (~8 m) after 40,050 cal yr BP (~35,460 14C yr BP) and 20,080 cal yr BP (16,640 14C yr BP; Fig. 4), corresponding to the highest loess accumulation rates that Muhs et al. (Reference Muhs, Ager, Been, Bradbury and Dean2003) record around 27,000–24,000 and 18,000 cal yr BP at St. Michael. The Sue Creek show a slighter lower amount of loess accumulation (~2.5 m) than at Kolmakof section, and the VABM Kuskokwim section displays the least amount of aeolian accumulation (~1 m) during this period of MIS 2. Soil development occurred at these sections around 20,080 cal yr BP (16,640 14C yr BP), 19,770 cal yr BP (16,380 14C yr BP), and 16,450 cal yr BP (13,650 14C yr BP), indicating more stability on the landscape, and likely some climatic amelioration, at the end of MIS 2 was occurring in the region by ~20,000 cal yr BP. However, high rates of aeolian deposition likely hampered the development of more mature soils by stifling vegetative growth, as seen in other parts of eastern Beringia at the later stages of MIS 2 and into the early parts of MIS 1 (Muhs et al., Reference Muhs, McGeehin, Beann and Fisher2004; Reuther, Reference Reuther2013).
The nature of terrestrial environments during the MIS 2 stade has been a source of controversy (Brigham-Grette et al., Reference Brigham-Grette, Lozhkin, Anderson, Glushkova and Madson2004). Many researchers have interpreted records from locations within western Alaska as supporting a mainly dry herbaceous tundra for much of the MIS 2 stade (Ager, Reference Ager2003; Bigelow, Reference Bigelow and Elias2007; Wooller et al., Reference Wooler, Saulnier-Talbot, Potter, Belmecheri, Bigelow, Choy and Cwynar2018), although others see a mixture of mesic and xeric environments (Elias et al., Reference Elias, Short, Nelson and Birks1996). A mosaic landscape with localized microenvironments and a mixture of habitat types is proposed by Elias (Reference Elias2001) and Guthrie (Reference Guthrie2001) with the lower lying regions of the Bering Land Bridge, with herbaceous tundra prevailing in the more interior regions. It remains unclear which scenario is correct. Regardless, the expanse of herbaceous tundra in western Alaska coastal and interior regions began to decline as mesic and shrub tundra communities became more prevalent in the early years of MIS 1 around 15,000 cal yr BP (Wooller et al., Reference Wooler, Saulnier-Talbot, Potter, Belmecheri, Bigelow, Choy and Cwynar2018).
Waythomas (Reference Waythomas1990, Reference Waythomas and West1996) defined the MIS 2 to MIS 1 transition as a period of continued loess and sand sheet accumulation in the central Kuskokwim region and Holitna Lowlands as the Beaverhouse Formation. Loess and sand sheet deposition in the region appears to cease later in MIS 1 (after 5700 to 3900 cal yr BP, or 5000–3600 14C yr BP) in the region as warmer and moister climates fostered increased landscape stability, soil development, and forest and peatland expansions. Herbaceous tundra dominated by Artemisia, Poaceae, and Cyperaceae, is present in the region between at least by 16,200 to 15,800 cal yr BP. Salix shrubs began to expand into the region by 14,400–13,700 cal yr BP, changing the vegetation to an herb-shrub tundra mix (Kaltenrieder et al., Reference Kaltenrieder, Tinner, Lee and Hu2011). Regional vegetation was likely quite sparse in its coverage in the MIS 2, and into the MIS 1 period, until after 13,700 cal yr BP, which is reflected in the thin soil development in all of our study sections.
Betula shrubs began dominate to the vegetative landscape between 13,700 and 10,060 cal yr BP. By 5,000 cal yr BP, Picea (P. glauca and P. mariana) began to expand in the region, as well as moist tundra species (Kaltenrieder et al., Reference Kaltenrieder, Tinner, Lee and Hu2011), as the landscape became more stable with less aeolian accumulation and disturbance. Thicker soils and peat development became more prevalent in the study sections after ~3,950 cal yr BP which appears to correlate to an overall expansion of peats and Picea. A similar scenario occurred across much of interior eastern Beringia during the early and middle Holocene (Ager, Reference Ager2003; Bigelow, Reference Bigelow and Elias2007; Kaufman et al., Reference Kaufman, Axford, Henderson, McKay, Oswald, Sanger and Anderson2016).
Cryogenic features (involutions and ice-wedge casts) developed in fine sediment matrices (fine sand, silt, and clay) between 40,000 and 13,850 cal yr BP (35,500 and 12,000 14C yr BP) at the Kuskokwim, Kolmakof, and Sue Creek, similar to the timing of ice-wedge development described in loess and sand sheets in the Holitna and Upper Kuskokwim lowlands (Waythomas, Reference Waythomas1990; Thorson and Guthrie, Reference Thorson and Guthrie1992). Ice-wedge growth appears to have ceased by 13,850 to 13,550 cal yr BP (12,000 to 11,730 14C yr BP) in this region. The involutions likely represent the downslope reworking of silts even as primary aeolian silt deposition was occurring, similar to the situation identified at the Colorado Creek site between 27,100 and 13,120 cal yr BP (22,710 and 11,290 14C yr BP; Thorson and Guthrie, Reference Thorson and Guthrie1992). Downslope reworking of sediments due to solifluction may be associated with warmer periods, a loss of perennially frozen ground, and a deepening of the seasonally thawed zone, or related solely to slope aspect and not necessarily driven by regional climate change (Thorson and Guthrie, Reference Thorson and Guthrie1992). The timing of ice-wedge growth has been difficult to radiocarbon date due to the incorporation of old carbon-bearing particulates into the matrices of ice-wedges. However, ice-wedge growth is generally associated with colder climates during stades and the cooler periods of interstades (Thorson and Guthrie, Reference Thorson and Guthrie1992; Lachniet et al., Reference Lachniet, Lawson and Sloat2012), as our data certainly follow this trend with wedges falling mostly in MIS 2.
CONCLUSIONS
The stratigraphic transitions at the Kolmakof, Sue Creek, and VABM Kuskokwim sections provide us windows into broad environmental changes on a highland landscape in western Alaska at the fringe of the Bering Land Bridge in eastern Beringia. These stratigraphic sequences extend back to, and likely beyond, the MIS 3 interstade, and contribute to the sparse published information on environmental sequences dating back to this interstadial. The data from this region provide environmental context for a territory that served as a corridor both for colonization of Asiatic biota from the Bering Land Bridge to other parts of interior and southern Alaska, as well as movement of North American taxa into Asia (Hopkins et al., Reference Hopkins, Hopkins, Matthews, Schweger and Young1982; Elias at al., Reference Elias, Short, Nelson and Birks1996; Cook et al., Reference Cook, Hoberg, Koehler, Henttonen, Wickström, Haukisalmi and Galbreath2005; Reuther et al., Reference Reuther, Rogers, Roussaeu and Druckenmiller2015).
The MIS 3 record shows near-surface, water-saturated sediments and thick peat-like soil development with minor accumulations of aeolian silt accumulation in the early half of the interstade (prior to 40,000 cal yr BP), reflecting a milder, wetter climate and more stable landscape during the part of the interstade. After, 40,000 cal yr BP, the sequences show increasing aeolian sediment deposition, possibly erosion, in the latter half of the MIS 3, likely under cooler, more arid conditions and more unstable landscape conditions than in the earlier half of the interstade.
During the early part of the MIS 2 stade, these sequences show high rates of aeolian sediment accumulation, with the highest rates occurring between 40,050–16,450 cal yr BP; terrestrial sequences that provide unique comparable aeolian records to the loess accumulation record from Zagoskin Lake at St. Michael by Muhs et al. (Reference Muhs, Ager, Been, Bradbury and Dean2003). The climate and environment of the MIS 2 appear highly arid and colder and very unstable landscapes much like other records from eastern Beringia, with the exception of a shallow lacustrine laminated sands and clays with freshwater shells in the Kolmakof section dating to 24,000 cal yr BP that indicates pockets of water on the surface at the height of MIS 2.
In the latter half of the MIS 2, weakly developed soils formed by 20,000 cal yr BP indicating some increasing landscape stability and climatic amelioration. Loess accumulation and aeolian sand sheet development continued into the MIS 1 interglacial at lower rates with intermittent periods of slightly more mature soil development occurring between 14,000 and 13,000 cal yr BP, as climate continued to ameliorate, shrub tundra began to expand across eastern Beringia, and major changes in terrestrial mammal diversity, biogeography, and, in some cases, animal behavior and human colonization began to occur (Hoffecker and Elias, Reference Hoffecker and Elias2007; Zazula et al., Reference Zazula, Froese, Elias, Kuzmina and Mathewes2007; Mann et al., Reference Mann, Groves, Reanier, Gaglioti, Kunz and Shapiro2015; Lanoë et al., Reference Lanoë, Reuther, Holmes and Hodgins2017). MIS 1 peat development in these sections occurred in the middle half of MIS 1, just prior to 3,950 cal yr BP, corresponding with extreme landscape stability that began to occur with this region and across eastern Beringia well after the MIS 2 glaciation.
ACKNOWLEDGMENTS
First and foremost, the authors would like to thank the community of Crooked Creek for their support, participation, and mutual interest in the past. Dennis and Evelyn Thomas and their entire extended family accommodated field crews. We also acknowledge the financial support of Donlin Gold, LLC. We would also like to thank Chris B. Wooley of Chumis Cultural Resource Services, Katherine Mulliken of the Alaska Division of Geological and Geophysical Surveys, and Sam Coffman of the University of Alaska Museum of the North Archaeology Department for support in this project. We also would like to thank the anonymous reviewers that supplied constructive comments to improve this manuscript.
SUPPLEMENTARY MATERIALS
The supplementary materials for this article can be found at https://doi.org/10.1017/qua.2019.51.