INTRODUCTION
The southern Levant (comprising modern-day southern Syria, Lebanon, Jordan, Israel, and the Sinai Peninsula of Egypt), is a narrow strip of land, constrained by the Mediterranean Sea to the west, and dry steppe or deserts (the Negev, Sinai, and Arabia) to the south and in the hinterland east of the Jordan Valley. This region forms a natural land passage between Africa and Eurasia and has played a major role in early hominin dispersal, probably functioning as a conduit into Asia and Europe, as attested by some of the earliest evidence of hominin occupations outside of Africa (Aguirre and Carbonell, Reference Aguirre and Carbonell2001; Bar-Yosef and Belfer-Cohen, Reference Bar-Yosef and Belfer-Cohen2001; Saragusti and Goren-Inbar, Reference Saragusti and Goren-Inbar2001; Templeton, Reference Templeton2002; Goren-Inbar and Speth, Reference Goren-Inbar and Speth2004; Gunz et al., Reference Gunz, Bookstein, Mitteroecker, Stadlmayr, Seidler and Webber2009; Sharon, Reference Sharon2014).
Lower Paleolithic (LP) sites in the southern Levant are located in a variety of geographic settings, but share common characteristics: most are located in open landscapes and are consistently situated near freshwater sources (Stekelis and Gilead, Reference Stekelis and Gilead1966; Gilead and Israel, Reference Gilead and Israel1975; Gilead and Ronen, Reference Gilead and Ronen1977; Bar-Yosef and Goren-Inbar, Reference Bar-Yosef and Goren-Inbar1993; Guérin et al., Reference Guérin, Eisenmann and Faure1993; Ronen, Reference Ronen1993; Ronen and Winter, Reference Ronen and Winter1997; Rollefson et al., Reference Rollefson, Schnurrenberger, Quintero, Watson and Low1997; Shea, Reference Shea1999; Goren-Inbar et al., Reference Goren-Inbar, Feibel, Verosub, Melamed, Kislev, Tchernov and Saragusti2000; Barzilai et al., Reference Barzilai, Malinsky-Buller and Ackermann2006; Rollefson et al., Reference Rollefson, Quintero and Wilke2006; Horwitz and Chazan, Reference Horwitz and Chazan2007; Rech et al., Reference Rech, Quintero, Wilke and Winer2007; Malinsky-Buller et al., Reference Malinsky-Buller, Hovers and Marder2011; Marder et al., Reference Marder, Malinsky-Buller, Shahack-Gross, Ackermann, Ayalon, Bar-Matthews, Goldsmith, Inbar, Rabinovich and Hovers2011; Lister et al., Reference Lister, Dirks, Assaf, Chazan, Goldberg, Applbaum, Greenbaum and Horwitz2013). An additional characteristic common to these sites is the composition of the faunal assemblages, demonstrating the preferential exploitation of medium to large-sized mammals and, in some cases, extremely large-sized mammals such as elephant and hippopotamus (Yisraeli, Reference Yisraeli1967; Tchernov, Reference Tchernov1987; Horwitz and Tchernov, Reference Horwitz and Tchernov1989; Tchernov, Reference Tchernov1992, Tchernov et al., Reference Tchernov, Horwitz, Ronen and Lister1994; Goren-Inbar et al., Reference Goren-Inbar, Lister, Werker and Chech1994; Horwitz and Monchot, Reference Horwitz and Monchot2007; Martínez-Navarro et al., Reference Martínez-Navarro, Belmaker and Bar-Yosef2009; Rabinovich et al., Reference Rabinovich, Gaudzinski-Windheuser, Kindler and Goren-Inbar2011; Yeshurun et al., Reference Yeshurun, Zaidner, Eisenmann, Martínez-Navarro and Bar-Oz2011; Rabinovich et al., Reference Rabinovich, Ackermann, Aladjem, Barkai, Biton, Milevski, Solodenko and Marder2012; Lister et al., Reference Lister, Dirks, Assaf, Chazan, Goldberg, Applbaum, Greenbaum and Horwitz2013). The observed settlement patterns demonstrate repeated visits to favored localities (Gilead and Ronen, Reference Gilead and Ronen1977; Goren-Inbar and Speth, Reference Goren-Inbar and Speth2004; Barzilai et al., Reference Barzilai, Malinsky-Buller and Ackermann2006; Horwitz and Chazan, Reference Horwitz and Chazan2007; Goring-Morris et al., Reference Goring-Morris, Hovers and Belfer-Cohen2009; Marder et al., Reference Marder, Malinsky-Buller, Shahack-Gross, Ackermann, Ayalon, Bar-Matthews, Goldsmith, Inbar, Rabinovich and Hovers2011; Bar-Yosef and Belfer-Cohen, Reference Bar-Yosef and Belfer-Cohen2013). There is also evidence for cave exploitation, mostly characterizing the late LP (e.g., Neuville, Reference Neuville1951; Garrod, Reference Garrod1956, Reference Garrod1970; Jelinek, Reference Jelinek1990; Weinstein-Evron et al., Reference Weinstein-Evron, Tsatskin, Porat and Kronfeld1999, Reference Weinstein-Evron, Bar-Oz, Zaidner, Tsatskin, Druck, Porat and Hershkovitz2003; Gopher et al., Reference Gopher, Barkai, Shimelmitz, Khalaily, Lemorini, Hershkovitz and Stiner2005, Reference Gopher, Ayalon, Bar-Matthews, Barkai, Frumkin, Karkanas and Shahack-Gross2010; Valladas et al., Reference Valladas, Mercier, Hershkovitz, Zaidner, Tsatskin, Yeshurun, Vialettes, Joron, Reyss and Weinstein-Evron2013).
Culturally, most of the LP open-air occupations in the southern Levant are ascribed to the Acheulian techno-complex. This lithic tradition is typically associated with the production and use of bifacial tools, of which hand axes and cleavers are the most well-known types (e.g., Gilead, Reference Gilead1970; Debénath and Dibble, Reference Debénath and Dibble1994; Sharon, Reference Sharon2007; Herzlinger et al., Reference Herzlinger, Wynn and Goren-Inbar2017). Today, a division of the Levantine Acheulian techno-complex into three or four phases (where the Acheulo-Yabrudian marks the final phase) is generally accepted, corresponding to environmental changes as well as technological and quantitative differences observed within and between assemblages (Bar-Yosef, Reference Bar-Yosef1994; Sharon, Reference Sharon2014).
The absolute dating of Acheulian occupations and their attribution to a specific cultural phase is a challenging task, mainly due to the fact that few absolute dating methods can reach back further than 0.5 Ma. Worldwide, the most reliable ages for LP sites are bounded by K-Ar/Ar-Ar dating of overlaying and/or underlying volcanic strata (e.g., Goren-Inbar et al., Reference Goren-Inbar, Belitzky, Verosub, Werker, Kislev, Heimann, Carmi and Rosenfield1992; Mishra et al., Reference Mishra, Venkatesan, Rajaguru and Somayajulu1995; Porat et al., Reference Porat, Zhou, Chazan, Noy and Horwitz1999; Barkai et al., Reference Barkai, Gopher, Lauritzen and Frumkin2003; Coltorti et al., Reference Coltorti, Feraud, Marzoli, Peretto, Ton-That, Voinchet, Bahain, Minelli and Hohenstein2005; Norton et al., Reference Norton, Bae, Harris and Lee2006). These strata are rare in the southern Levant, however, and are absent in most Acheulian contexts in this region, with the exception of the site of Gesher Benot Ya'akov (Goren-Inbar et al., Reference Goren-Inbar, Belitzky, Verosub, Werker, Kislev, Heimann, Carmi and Rosenfield1992). In many of the early excavations, site chronology relied upon biostratigraphy and lithic technological and typological analyses (e.g., Bar-Yosef, Reference Bar-Yosef1994; Tchernov et al., Reference Tchernov, Horwitz, Ronen and Lister1994 and references therein). Nowadays, a suite of advanced dating techniques can provide ages for older time spans (e.g., TT-OSL, pIR-IR290, cosmogenic isotope burial age, and paleomagnetic stratigraphy), thus allowing the reconstruction of a more reliable chronometric framework for the LP in the southern Levant (e.g., Porat et al., Reference Porat, Chazan, Schwarcz and Horwitz2002; Porat and Ronen, Reference Porat and Ronen2002; Malinsky-Buller et al., Reference Malinsky-Buller, Barzilai, Ayalon, Bar-Matthews, Birkenfeld, Porat, Ron and Ackermann2016; Zaidner et al., Reference Zaidner, Porat, Zilberman, Herzlinger, Almogi-Labin and Roskin2018).
At the center of this paper is a complex of Acheulian sites located near Kibbutz ‘Evron (western Galilee, Israel; Fig. 1). Seven distinct localities, representing various phases of the Acheulian techno-complex, have been discovered to date at ‘Evron, implying that this environment was a favorable setting for hominin activity during the LP (Stekelis, Reference Stekelis1950; Prausnitz, Reference Prausnitz1969; Gilead and Ronen, Reference Gilead and Ronen1977; Ronen, Reference Ronen1991, Reference Ronen1993). The sites are situated ~400 m north of the Beit Ha'emeq stream, which drains the western Galilee Mountains into the Mediterranean Sea. More specifically, this area is situated in an alluvial plain (~30 m above sea level [asl]), accreted between a prominent eolianite (kurkar) ridge, ~40 m asl, running parallel and 1.5–2 km east of the current shoreline and the Galilee Mountains (Fig. 1). The exact location of some of the LP localities in this complex is unclear, as many were found by amateur archaeologists who did not systematically document their position. Figure 1 presents the estimated location of some of the ‘Evron sites, based on relevant published data (Stekelis, Reference Stekelis1950; Gilead and Ronen, Reference Gilead and Ronen1977; Ronen, Reference Ronen1991, Reference Ronen1993).

Figure 1. (color online) Main Lower Paleolithic sites in Israel over topographic map. Enlarged: Estimated location of selected sites in the ‘Evron complex, including the newly found locality of ‘Evron Landfill.
The most well-known site in the ‘Evron complex, and the one most extensively excavated and studied to date, is Evron Quarry, considered to represent an early Acheulian occurrence based on lithic analyses (Ronen and Prausnitz, Reference Ronen and Prausnitz1979; Gilead and Ronen, Reference Gilead and Ronen1977; Ronen, Reference Ronen and Prausnitz1979; Ronen, Reference Ronen1991; Chazan, Reference Chazan2013). Discovered in a once-active eolianite quarry, this site was interpreted as a series of repeated occupations, as was demonstrated by three distinct archaeological layers (Ronen and Prausnitz, 1969; Ronen, Reference Ronen1991). The lithic industry from Evron Quarry includes coarse hand axes and chopping tools (Gilead and Ronen, Reference Gilead and Ronen1977) as well as a small-flake industry (Chazan, Reference Chazan2013). The faunal assemblage yielded remains of two Proboscideans - Elephas sp. and Stegodon sp., as well as an endemic suid Kolpochoerus evronensis, Hippopotamus amphibus, Cervus elaphus, Bos cf. primigenius, Gazella cf. gazella, Capreolus sp., a hyaenid, Gerbillus, and Trionyx (Haas, Reference Haas1970; Ronen and Prausnitz, Reference Ronen and Prausnitz1979; Tchernov et al., Reference Tchernov, Horwitz, Ronen and Lister1994).
Based on comparative analyses of lithic and faunal assemblages, the age of Evron Quarry was initially estimated to range between 1.0–0.8 Ma (Gilead and Ronen, Reference Gilead and Ronen1977; Ronen, Reference Ronen1991; Tchernov et al., Reference Tchernov, Horwitz, Ronen and Lister1994). In the late 1990s, site chronology was established using optically stimulated luminescence (OSL), electron spin resonance (ESR), and paleomagnetic stratigraphy of sediments from trench-sections dug in an adjacent field and from an exposed 5-m-deep section, located some 500 m northeast of the original site (Porat and Ronen, Reference Porat and Ronen2002; Ron et al., Reference Ron, Porat, Ronen, Tchernov and Horwitz2003). OSL and ESR results yielded a minimum age of 0.50–0.65 Ma for the geological unit that is assumed to correspond to the archaeological layer (Porat and Ronen, Reference Porat and Ronen2002), while the paleomagnetic stratigraphy constrained its age between the Jaramillo subchron and the Brunhes/Matuyama transitions. This points to a time of deposition sometime during the interval of 1.0–0.8 Ma, similar to the initial chronology that was suggested based on regional comparative analyses of the lithic and faunal assemblages (Ron et al., Reference Ron, Porat, Ronen, Tchernov and Horwitz2003). Since absolute ages were retrieved from off-site sections, however, these ages relied on a correlation between geological units in the trenches, the exposed section and in the archaeological site. Therefore, their association to the excavated archaeological layers is considered by many as insecure.
During October 2014, a massive construction operation was initiated ca. 300 m northwest of the Evron Quarry site. Large-scale earthworks exposed a deep (~14 m) sedimentary sequence, revealing a complex stratigraphy of the ancient Mediterranean coastal plain (Shemer and Barzilai, Reference Shemer and Barzilai2017). Animal bones and flint artifacts were identified at depths of 9–11 m, originating from a red clayey sediment, indicating the possible presence of a new Acheulian occurrence within the Evron site complex (henceforth ‘Evron Landfill; Fig. 1).
Based on a multidisciplinary study conducted on-site at the ‘Evron Landfill locality that incorporated archaeological, geological, and paleomagnetic stratigraphy analyses, as well as dating of the archaeological layer based on cosmogenic isotope burial age, we introduce new data regarding the chronological framework and depositional environments of the Acheulian complexes near ‘Evron.
METHODS
Site settings and the archaeological excavation
During October 2014, massive earthworks aiming to expand the landfill operating in the quarry grounds since the 1990s were initiated in the fields to its north. These included a large-scale digging operation using heavy machinery, covering an area of ca. 4 ha and designated to reach a maximum depth of 19 m below the surface. Due to safety considerations and in order to prevent collapses, the walls of this pit were artificially stepped, with each step reaching a maximum depth of 2–3 m (Supplementary Figures 1–6).
Upon reaching a depth of 9–11 m, animal bones and flint artifacts were discovered embedded in red clayey sediments. Following this discovery, a series of test trenches were dug using a JCB backhoe, indicating the possible existence of concentrations of archaeological finds in the western part of the landfill. Archaeological excavations were then conducted, focused on four of the trenches (T1–T4; Fig. 2). The trenches were subjected to manual section cleaning in search of an archaeological layer. In situ finds were discovered only in T2, leading to the opening of 1×1-m excavation square at its southern edge (Shemer and Barzilai, Reference Shemer and Barzilai2017). All sediments were dry sieved using a 10-mm mesh.

Figure 2. (color online) ‘Evron Landfill sampling locations. Numeric values indicate elevation (m) above sea level.
Soil stratigraphy and pedo-sedimentry units
The soil stratigraphy of the ‘Evron Landfill was determined based on detailed description and sampling of three pedo-sedimentary sequences, following standard procedures (Soil Survey Staff, 1999). Two of the documented sequences are located on the eastern and western edges of the landfill, and are termed Eastern and Western sequences, respectively (Fig. 2 and 3). Due to the artificial, stepped morphology of the landfill walls, the units were described and sampled along a ~30 m horizontal strip. The third sequence (termed the Central-Southern sequence) is composed of two sections: the base of the sequence (termed the Central sequence) was sampled in Trench T2 (the main archaeological excavation area; Fig. 2) and in an exposed section located ~30 m west of the trench. The top of the sequence was sampled in the southern wall of the landfill (termed the Southern sequence). The correlation between the Central and the Southern sequences was carefully inspected and validated in the field, and thus they are reported here as a single continuous sequence (Central-Southern sequence).

Figure 3. (color online) Schematic cross sections (a) WWN-EES and (b) SSW-NNE, with the location of paleomagnetic results.
The coordinates and elevation of the unit boundaries for each sequence were determined using a RTK-GPS device. Correlations between the sequences and lateral changes within the units are based on field observations along the exposed landfill walls. Based on the above, two schematic cross sections were constructed for the entire landfill (Fig. 2 and 3); one from WNW to ESE (A–A′) and the second from SSW to NNE (B–B′).
The sediment samples were oven dried, divided and sieved through 2-mm mesh. All samples were analyzed for bulk particle-size distribution (PSD) using Malvern MS-2000 laser diffraction device, following the procedure of Crouvi et al. (Reference Crouvi, Amit, Enzel, Porat and Sandler2008). Samples from three units were examined for micromorphology using thin sections.
Paleomagnetic stratigraphy
Within the framework of the paleomagnetic study, 101 oriented samples were collected from 26 different sedimentary horizons in the Southern, Central, and Northern sequences (Fig. 2 and 3 and Supplementary Material) in a twofold effort: (a) to construct a magnetostratigraphic age model of the site, and (b) to cross-correlate the sedimentary units in the three sequences. From each horizon, three oriented samples were collected for alternating field (AF) demagnetization experiment using non-magnetic plastic boxes (1.5 × 1.5 × 1 cm). In this method, a cube-shaped sample with three vertical faces and two horizontal faces is carved from the section. After the sediment sample is placed in a plastic box, its orientation is precisely measured. It is then carefully separated from the section and glued within the plastic box using non-magnetic glue. In addition, from all horizons except the three sampled in Trench T2 (Fig. 2), a fourth sample was collected using a quartz cylindrical crucible, measuring 2.54 cm (1 inch) in diameter and height, for thermal demagnetization experiments.
Paleomagnetic experiments were carried out at the paleomagnetic laboratory in the Institute of Earth Sciences, the Hebrew University of Jerusalem, using a 2G-750 superconducting rock magnetometer equipped with in-line 2-axis AF coils, and ASC-TD48 thermal demagnetizer. AF demagnetizations were done at progressively elevated peak magnetic field strength in 5 mT or 10 mT steps up to peak field strength ranging between 50 mT to 120 mT, until the entire magnetization vector was removed. Thermal demagnetizations were done in 50°C steps from 50°C up to 300°C, and in 40°C steps up to 680°C, or until the sample was completely demagnetized. The direction of the characteristic remanence magnetization was determined based on end-point Zijderveld (Reference Zijderveld1967) diagrams using the Demag-GUI program, a part of the PmagPy software package (Tauxe et al., Reference Tauxe, Shaar, Jonestrask, Swanson-Hysell, Minnett, Koppers, Constable, Jarboe, Gaastra and Fairchild2016). The polarity sequence was compared with the Quaternary Geomagnetic Instability Time Scale of Singer (Reference Singer2014).
Cosmogenic isotope burial dating
Cosmogenic isotope burial dating enables the dating of continental sediments over a time range of 0.5–5 Ma and in settings where other dating methods do not apply (Granger and Muzikar, Reference Granger and Muzikar2001; Granger, Reference Granger2006; Hidy et al., Reference Hidy, Gosse, Blum and Gibling2014; Matmon et al., Reference Matmon, Fink, Davis, Niedermann, Rood and Frumkin2014). 10Be–26Al burial dating of sediment is based on a process in which these two nuclides are produced in a material at, or near, the surface at a known ratio and then buried and shielded from further cosmic-ray bombardment such that production effectively ceases. Once production is shut off, nuclide concentrations are governed by radioactive decay. This ratio decreases with time since 26Al decays about twice as fast as 10Be: the half-life of 10Be is 1.387 Ma (Chmeleff et al., Reference Chmeleff, von Blanckenburg, Kossert and Jakob2010; Korschinek et al., Reference Korschinek, Bergmaier, Faestermann, Gerstmann, Knie, Rugel, Wallner, Dillmann, Dollinger and Von Gostomski2010) and the half-life of 26Al is 0.705 Ma (Nishiizumi, Reference Nishiizumi2004).
The accepted surface production ratio of 26Al and 10Be in quartz in most natural settings is ~6.8 ( Nishiizumi et al., Reference Nishiizumi, Imamura, Caffee, Southon, Finkel and McAninch2007; Balco et al., Reference Balco, Stone, Lifton and Dunai2008), although recent studies suggest this ratio may vary slightly depending on altitude (Argento et al., Reference Argento, Reedy and Stone2013; Lifton et al., Reference Lifton, Sato and Dunai2014). Using Equation 1, a simple burial age may be easily calculated (Granger et al., Reference Granger, Kirchner and Finkel1997):

Where
$\left( {{{N_{{26}} } \over {N_{{10}} }}} \right)_{{\left( t \right)}} $
is the ratio of the measured 26Al and 10Be concentrations,
$\left( {{{N_{{26}} } \over {N_{{10}} }}} \right)_{{\left( 0 \right)}} $
is the initial ratio of 26Al and 10Be concentrations (at the time of burial), t burial is the time since burial, and τ10 and τ26 are the mean lives of 10Be and 26Al (Granger and Muzikar, Reference Granger and Muzikar2001), respectively.
Complications to this simple approach arise if the pre-burial ratio is below that of the production ratio (for example, if there was a previous burial period or if bedrock was exhumed very slowly), or if burial was shallow such that post-burial nuclide production by deeply penetrating muons still occurred. In these cases, either a maximum or minimum age for the case of a significant inherited depositional ratio or post-burial production, respectively, is given, or additional independent constraints are required.
10Be–26Al burial dating methods can generally be applied to sediments that are buried longer than 0.3 Ma, at which time the measured 26Al/10Be ratio can be reliably distinguished from the surface production ratio. The maximum limit of the method is ~5 Ma, at which time ~7 half-lives of 26Al reduce its concentration either to secular equilibrium, which is controlled by the very low production rate of nuclides by deeply penetrating muons, or to a level beneath current accelerator mass spectrometry (AMS) measurement capabilities (Matmon et al., Reference Matmon, Fink, Davis, Niedermann, Rood and Frumkin2014).
Three samples were collected to constrain the burial age of the lower part of the ‘Evron Landfill sequence (Fig. 2, Supplementary Figure 1). Samples EQ-1 and EQ-2 were collected from a pit excavated in the middle of the site (Unit 8), where the deepest possible part of the section was exposed. Carbonate cemented eolianite was exposed at the base of the pit (Supplementary Figure 1a); its lower part is massive while the upper part is finely bedded. Sample EQ-1 was collected from the lower massive unit, 12.9 m below the pre-excavation surface, while sample EQ-2 was collected from the layered unit, 12.5 m below the pre-excavation surface. Sample EQ-3 was collected from the main archeological area (Trench 2), closer to the western side of the site (Fig. 2, Supplementary Figure 1b). This sample was collected from a massive red clay unit rich in quartz grains, which correlates with the artifact-bearing Unit 5 in the Central sequence of the landfill. This sample is positioned 10.0 m below the pre-landfill surface.
Measurements of 10Be and 26Al were made on the quartz 250–850 µm grain-size fraction. Quartz cleaning procedure and the extraction of Be and Al cations (converted to oxides) followed standard procedures (Kohl and Nishiizumi, Reference Kohl and Nishiizumi1992; Bierman and Caffee, Reference Bierman and Caffee2001). Samples were analyzed at ASTER, CEREGE, Aix en Provence, France. Isotopic ratios of 10Be/Be and 26Al/Al were corrected with chemistry process blanks and normalized to accepted in-house AMS standard reference materials with agreed nominal isotopic ratios.
RESULTS
Soil stratigraphy and pedo-sedimentry units
The ‘Evron Landfill presents a ~14 m complex pedo-sedimentary sequence of coastal and on-land, near-coastal sediments, including beach rock, eolianite, sands, and wetland deposits (Fig. 3; Tables 1–3). The entire sequence unconformably overlies yellow marl (not sampled, base not exposed, Unit 9 in Fig. 3). The lowest sampled Unit 8 is a 3-m-thick carbonate-rich beach rock with prominent horizontal bedding and signs of pedogenesis (roots casts and burrowing). A ~30-cm-thick calcrete caps the beach rock. The top of Unit 8 dips to the west and south.
Table 1. The Eastern pedo-sedimentary (PS) sequence.

Table 2. Central and southern pedo-sedimentary (PS) sequences.

Table 3. The Western pedo-sedimentary (PS) sequence.

Unit 7 uncomfortably overlies the beach rock with an abrupt and wavy boundary. It is composed of brown sticky clay to silty clay, rich in black iron-manganese nodules. In the eastern wall, a well-developed calcic horizon was observed at the upper part of this unit. Farther west, clear erosional features were identified cutting the top of this unit (see Unit 5).
Unit 6 is a 1.3-m-thick, dull orange eolianite that abruptly overlies the clay sediments of Unit 7. This unit appears only in the eastern part of the landfill. It dips to the west and can be traced towards the central part of the landfill to a point where it is cut by Unit 5.
Unit 5 is composed mostly of brown clay sediments similar to Unit 7, with alternating fluvial layers/lenses rich with sand grains. This unit shows clear cut-and-fill morphologies and cut into Units 7 and 6. The fluvial layers within Unit 5 vary in thickness from a few centimeters to ~0.5 m and are composed of many angular quartz sand grains, with a few carbonate rock fragments; in a few of these fragments Cretaceous foraminifera were identified, meaning these fragments originated east of the site in bedrock exposures. The cement is composed of calcite and iron oxides, and fills small pores between grains, indicating a vadose environment (i.e., water leaching above water level). The erosional contact between Unit 5 and the eolianite of Unit 6 resembles sea-cliff morphology. Eolianite clasts were identified within Unit 5 near the contact between these units. Unit 5 was identified only in the Western, Central and Northern sequences. It thickens to the west.
Unit 4 uncomfortably overlies the eolianite of Unit 6 in the eastern part of the landfill, the fluvial sediments of Unit 5 in the Central and Western parts, and the clay sediments of Unit 7 in the southern part. The characteristics of this unit vary laterally and appear differently in each of the studied sequences. Thus, we cannot assume that this unit is a simple chronostratigraphic unit. In the Eastern sequence, Unit 4 is 1.1-m-thick reddish-brown clay, with a well-developed calcic horizon. In the Central sequence, Unit 4 is thicker (3.3 m) dark-grayish yellow clay, with few iron-manganese nodules and with a well-developed calcic horizon at the top. In the Southern sequence, this unit is bright reddish-brown sandy loam to sandy clay loam, with calcic horizon at the top. The lateral boundaries between these facies comprising Unit 4 were not clearly identified in the field.
Unit 3 abruptly overlies Unit 4. It is composed of 1–2 m-thick, gray silty clay to clay with well-developed calcic horizon at the upper part of the unit.
Unit 2 appears only in the eastern part of the landfill. It is a 2-m-thick bright yellowish-brown loam, with very well-developed calcic nodules. The unit gradually disappears to the west and does not appear in the Central sequence.
Unit 1 abruptly overlies Unit 2 in the eastern part of the landfill and Unit 3 in its western part. It is 1–2.5 m-thick, brownish-black silty clay that caps the entire sequence of the ‘Evron Landfill.
Flint artifacts and faunal remains found during the excavation originated from the contact between stratigraphic Units 7 and 5. Most of the finds were collected from the northwestern part of the landfill. During the excavation some sorting of the finds was observed: all animal bones and large artifacts (>5 cm) were embedded in the brown clayey sediment of Unit 7, whereas smaller flint artifacts tended to be more common in the fluvial sediments associated with Unit 5.
Paleomagnetic stratigraphy
Our criterion for a reliable magnetic polarity interpretation of a horizon (where N stands for normal, and R stands for reverse) is that at least two samples, one demagnetized thermally and another with AF, show similar and identical unambiguous results. Horizons that do not meet the above criterion but have at least one sample with clear polarity interpretation are marked with a question mark (i.e., N? or R+?). Figure 4 shows demagnetization results from two horizons meeting the above reliability criterion. The upper panel shows a univectorial normal polarity magnetization with north-northeast declinations and downward inclinations. The lower panel shows a primary reversed polarity vector overprinted by secondary normal polarity viscous magnetization. Out of 26 horizons, 11 had reliable polarity interpretations, 14 had questionable interpretations, and one did not provide any successful interpretation (Fig. 3 and 4; Supplementary Figures 2–6; Supplementary Tables 1–5). The horizon that failed is a fluvial horizon in Unit 5 that contained a major component of small flint items (Fig. 5b).

Figure 4. (a and c) Representative results of alternating field and (b and d) thermal demagnetizations shown as Zijderveld plots (Zijderveld, Reference Zijderveld1967) and equal area projection. (a) and (b) display two samples from the same horizon showing univectorial normal polarity magnetization. (c) and (d) from the same horizon show primary reverse magnetization, where (d) is overprinted by a secondary normal magnetization. Blue (red) squares (circles) are projections of the paleomagnetic direction on the horizontal (vertical) planes. (For interpretation of the references to color in this figure legend, the reader is referred to the web version of this article.)

Figure 5. Paleomagnetic stratigraphy. White dashed lines show main stratigraphic boundaries; black dashed line shows erosional disconformity in Unit 5; numbers correspond to units within the pedo-sedimentary sequence (see text). Paleomagnetic interpretations are marked with N (normal) and R (reverse). A question mark is added when interpretation is not supported by both AF and thermal demagentizations.
Southern sequence (Fig. 5a)
The southern landfill wall provided the most complete sedimentological sequence to be sampled for paleomagnetic stratigraphy. Three reversals were identified in this sequence—one in Unit 4b and two in Unit 7. Assuming that the age of Unit 3 is a few thousand years at the most, based on previous works (Porat and Ronen, Reference Porat and Ronen2002; Ron et al., Reference Ron, Porat, Ronen, Tchernov and Horwitz2003), the Brunhes–Matuyama (BM) boundary (0.77 Ma) is located in Unit 4b. The normal horizon bracketed by reversed horizons in Unit 7 is most likely the Jaramillo normal subchron (1.076–1.008 Ma). Two additional possible interpretations for the normal horizon are the Cobb Mtn (1.221–1.189 Ma) and Olduvai (1.934–1.787 Ma). The Cobb Mtn is too short to enable magnetic recording in these types of sediments, and therefore this interpretation is unlikely. The Olduvai interpretation demands that either the Jaramillo subchron is not represented in the sequence, or that the sampling resolution is insufficient for recovering the Jaramillo. Taken altogether, we conclude the base of the southern section within Unit 7 must be older than the Jaramillo, i.e., older than 1.076 Ma.
Central sequence (Fig. 5b)
The Central sequence comprises a segment of Units 5 with a fluvial wedge, rich with small flint items, that thickens northward. The polarity of this fluvial unit could not be determined because one sample (EQP-29, Supplementary Material) showed a primary reverse magnetization overprinted by a secondary normal magnetization, but a neighboring sample (EQP-30, Supplementary Material) showed a contradicting result of a primary normal magnetization. This unit separates an overlying normal polarity horizon from an underlying reversed horizon. Thus, even if the polarity of the unit could not be determined, its age is likely to be near the BM boundary.
Trench T2 (Fig. 5d)
The polarity of the three horizons in trench T2 is normal. The stratigraphic correlation between the upper fine-grained alluvial unit in the trench (normal polarity) and the lowermost horizon in the Central section (Fig 5b., reverse polarity) is not clear, owing to the cut-and-fill morphology. Thus, a clear magnetostratigraphic affiliation of the trench cannot be firmly determined.
Northern sequence (Fig. 5c)
The stratigraphy of the Northern sequence comprises Units 3–7. Here, no clear reversals where found in Units 4–5, but a questionable reversed horizon overlying a normal horizon was found in the transition between Unit 5 and Unit 7. We suggest that this reverse horizon is in the same stratigraphic position as the reverse horizon in the Central sequence (Fig. 5b).
Dating by cosmogenic isotope burial ages
10Be concentrations range between 20.97±0.60×104 atoms/g quartz and 26.42±0.76×104 atoms/g quartz. 26Al concentrations follow a similar pattern to that of 10Be and they range between 58.76±3.5×104 atoms/g quartz and 87.17±4.5×104 atoms/g quartz (Table 4). 26Al/10Be ratios range between 2.80±0.18 and 3.30±0.20. Concentrations and 26Al/10Be in the two lower samples (EQ-1 and EQ-2) are similar. Concentrations and 26Al/10Be in the upper sample (EQ-3) are higher.
Table 4. Field and cosmogenic data for ‘Evron Landfill samples. Be spike concentration = −1414 ppm. Uncertainties in concentrations include spike uncertainty added in quadrature. 10Be/9Be are blank corrected and normalized to NIST SRM-4325=2.790×10−11. Presented values are corrected for procedural blank. 26Al/27Al ratios are blank corrected and normalized to Al2O3 SD=7.401×10−12. Presented values are corrected for procedural blank.

When assuming an initial 26Al/10Be ratio of 6.8 (the production rate ratio) these ratios imply ages of 1.69±0.21 Ma (EQ-1), 1.60±0.20 Ma (EQ-2), and 1.36±0.18 Ma (EQ-3 and Fig. 6). However, Davis et al. (Reference Davis, Matmon, Rood and Avnaim-Katav2012) showed that Nilotic sands along the eastern coast of the Mediterranean are typically deposited with 26Al/10Be ratios that range between 4.46±0.28 and 4.92±0.35 (n=4), corresponding to an average apparent burial age 0.69±0.085 ka. This depressed ratio, relative to the production rate ratio, results from a complex exposure-burial history of the sands while being transported along the Nile, its delta, and then along the eastern Mediterranean shore by long-shore currents. Thus, corrected ages can be obtained by subtracting the apparent initial age from the simple burial ages. This subtraction yields corrected ages of 0.99±0.1 Ma (EQ-1), 0.90±0.13 Ma (EQ-2), and 0.66±0.11 Ma for the upper sample (EQ-3; Table 5). It is important to clarify here, however, that, although the corrected cosmogenic burial ages are the most probable ones, within analytical error, the age of EQ3 may be as old as 0.77 Ma (nearly at the BM transition). Furthermore, it is theoretically possible that for a specific sample, the initial ratio at the time of burial may be over- or underestimated, invalidating the corrected age.

Figure 6. 10Be concentrations and 26Al/10Be ratios of samples from ‘Evron Landfill (blue markers). Modern sand samples (Davis et al., Reference Davis, Matmon, Rood and Avnaim-Katav2012) are indicated with red markers. Initial exposure periods are indicated with plain numbers. Burial isochrones are indicated by bold numbers. (For interpretation of the references to color in this figure legend, the reader is referred to the web version of this article.)
Table 5. Burial ages for ‘Evron Landfill samples. Scaling factors for spallation were calculated using Lal-Stone (Reference Stone2000). Sea-level high-latitude production rates due to spallation for 10Be and 26Al in quartz are 4.5±0.52 and 30.0±3.5 atoms/g/yr, respectively (Balco et al., Reference Balco, Stone, Lifton and Dunai2008). Muon production rates and altitude scaling are based on Granger and Muzikar (Reference Granger and Muzikar2001). Half-lives for 10Be and 26Al are 1.39±0.01 and 0.705±0.02 Ma, respectively (Chmeleff et al., Reference Chmeleff, von Blanckenburg, Kossert and Jakob2010; Korschinek et al., Reference Korschinek, Bergmaier, Faestermann, Gerstmann, Knie, Rugel, Wallner, Dillmann, Dollinger and Von Gostomski2010; Nishiizumi, Reference Nishiizumi2004). Elevation=30 m asl; latitude=33°N. Corrected age is calculated by subtracting the average apparent burial age of modern coastal-plain sands from the simple burial age of each sample. The apparent average burial age of modern sands is calculated from the 26Al/10Be ratio measured in four modern sand samples (Davis et al., Reference Davis, Matmon, Rood and Avnaim-Katav2012).

The archaeological finds
The lithic assemblage
The lithic assemblage from ‘Evron Landfill is small, comprising a total of 236 items (Table 6). Only 31 artifacts were retrieved from clear excavation contexts, while the rest were collected from the surrounding area, enabling their ascription to a sedimentological unit, but with no clear stratigraphic correlation to the finds in trench T2. Most prominent are several large core tools: two hand axes, three spheroids, and three choppers (Fig. 7), comprising a third of all tools in the assemblage (Table 7).
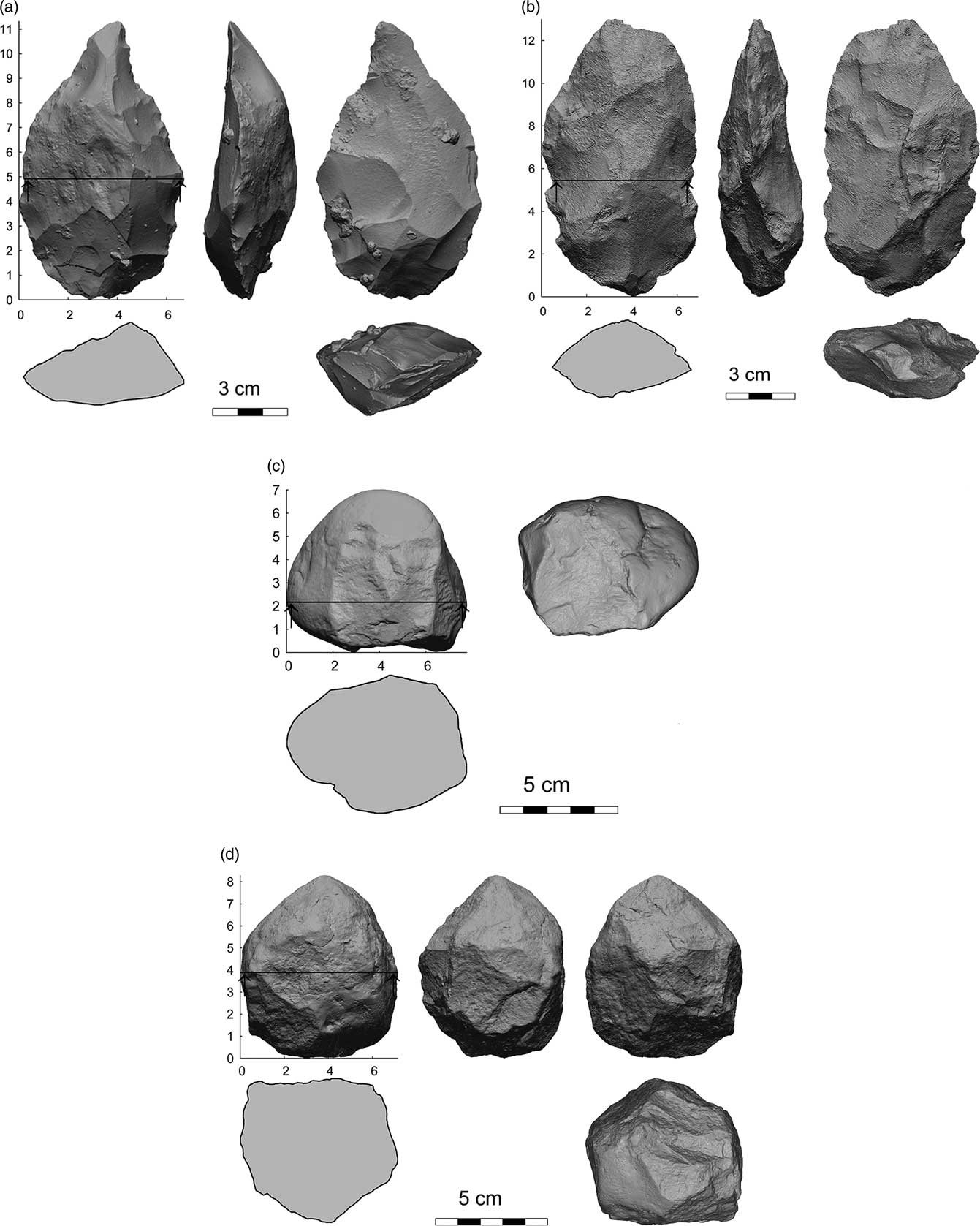
Figure 7. Large tools from the ‘Evron Landfill assemblage: (a and b) handaxes, (c) a chopper, and (d) a spheroid (3-d scans by Argita Levanon, courtesy of the Israel Antiquities Authority).
Table 6. General breakdown of the lithic assemblage from ‘Evron Landfill.

Table 7. Tools and blank types frequencies.

Flint is the dominant raw material used;, however, all three spheroids and the hammerstone found in the assemblage are made of hard limestone. Two additional limestone pebbles that display several removal scars, as well as several limestone flakes, might represent broken or discarded hammerstones.
Cores comprise ca. 23% of the assemblage (Table 6). Polyhedral cores are the most common (26 of 54 cores), demonstrating the utilization of multiple faces for flake production (Fig. 8.1 and 8.3). Prismatic cores are relatively few (n=7), displaying a volumetric concept for the production of elongated flakes (Fig. 8.2). Tested nodules (n=21) comprise the rest of this category. Many of the cores (n=34; ca. 64%) are small, with metric values measuring less than 3 cm. While many are very exhausted, some of these cores still retain cortex and demonstrate that the initial size of the nodules was small. Accordingly, these nodules were designated for the intentional production of small flakes (Fig. 8). These somewhat resemble the industry described by Chazan (Reference Chazan2013) in the Evron Quarry assemblage. A similar industry was also described at the site of Bizat Ruhama (Zaidner et al., Reference Zaidner, Ronen and Burdukiewicz2003; Zaidner, Reference Zaidner2014).

Figure 8. The small flakes industry component in the ‘Evron Landfill assemblage: (a–c) cores, (d and e) “Levallois-like” flakes, and (f) CTE (3-d scans by Argita Levanon, courtesy of the Israel Antiquities Authority).
Tools comprise ca. 10% of the assemblage, including both retouched and core tools. Flakes and primary flakes are the most common blank types, representing 25% and ca. 17% of this category, respectively (Table 7). Most frequent tool types are side scrapers (n=5) and perforators (n=5), each comprise ca. 21% of this category. There is one multiple tool, displaying a combination of truncation with limited scraper retouch. Other retouched tools include end scrapers, denticulates, and retouched flakes (Table 7).
Choppers vary in size, presenting a range of 4–7.8 cm in length, 4.3–6.9 cm in width, and 5.1–7.6 cm in thickness. Hand axes are relatively coarse, with an average length of 12.4 cm and an average width of 6.7 cm (Fig. 7). Both present deep scars, indicating the use of hard-hammer percussion for their production. These were made of two distinct raw material types: the first (Fig. 7:1) was made of a whitish-gray coarse-grained flint; the second, oval in shape (Fig. 7:2), was made of a translucent quarzitic raw material.
The Faunal assemblage
Seven faunal skeletal elements were recovered from ‘Evron Landfill, representing three different taxa:
(1) A medium-sized artiodactyl cf. Dama sp., represented by a humerus shaft (Fig. 9a), with minimal shaft width above the distal end of 26.18 mm. Remains of Cervus elaphus and of a smaller cervid cf. Capreolus, were identified from the earlier Evron Quarry excavations (Tchernov et al., Reference Tchernov, Horwitz, Ronen and Lister1994), but no remains of fallow deer. At the LP site of Gesher Benot Ya’akov, remains of Dama sp. comprise the majority of identified fauna and fall within the size range documented for modern female Dama mesopotamica (Rabinovich et al., Reference Rabinovich, Gaudzinski-Windheuser, Kindler and Goren-Inbar2011).
(2) An extinct equid, Equus. cf. tabeti, represented by an upper right first (M1) or second molar (M2; Fig. 9b) using dental criteria developed by Eisenmann et al. (Reference Eisenmann, Alberdi, De Giuli and Staesche1988) and papers on the dental biometry and morphology of Levantine Paleolithic equids (e.g. Davis Reference Davis1980; Eisenmann Reference Eisenmann1986, Reference Eisenmann1992, Reference Eisenmann2012a, Reference Eisenmann2012b). The ‘Evron Landfill molar has a small protocone with a small caballine fold present, while the enamel of the fossettes is moderately plicated (Fig. 9b). These morphological features are also visible in upper molars from ‘Ubeidiya and Gesher Benot Ya’akov that have also been assigned to E. cf. tabeti (Eisenmann, Reference Eisenmann1986, Reference Eisenmann2012a). Dimensions (in mm) of the ‘Evron Landfill tooth are: occlusal length=25.28; occlusal breadth=26.14; basal length=21.33; basal breadth=24.36; and protocone length=11.44. Based on occlusal size and protocone length, as illustrated in Figure 10, the ‘Evron Landfill specimen falls within the upper size range E. tabeti M1/M2’s from Aїn Hanech (Algeria) and close to a specimen from Gesher Benot Ya’akov (and also Bizat Ruhama; fig. 9 in Yeshurun et al., Reference Yeshurun, Zaidner, Eisenmann, Martínez-Navarro and Bar-Oz2011), while it differs slightly from a specimen from ‘Ubeidiya, which has a shorter protocone but larger occlusal diameter. Though no equid remains were recovered during the initial investigations at Evron Quarry (Tchernov et al., Reference Tchernov, Horwitz, Ronen and Lister1994), three of the four equid cheek teeth from surface collections at the LP site of Oumm Zinat, located some 500 m east of Kibbutz Evron (Horwitz and Tchernov, Reference Horwitz and Tchernov1989), resemble those of E. cf. tabeti (Eisenmann, Reference Eisenmann2012b), indicating continuity of this taxon during the LP in the Evron find locality.
(3) A large bovid identified as Bos cf. primigenius is represented by a lower left second molar (M2, still erupting; Fig. 9c and d) as well as two unconjoined lobes (anterior and middle) of a lower left third molar (M3) from an older individual. Following criteria given in Slott-Muller (Reference Slott-Moller1990), both molars from the new excavations at ‘Evron Landfill conform to morphological features considered typical of Bos rather than Bison. The central buccal column of the M2 is straight and begins at the cemento-enamel junction, with no apparent thickening at the cervix; the crown base, though damaged, appears to be straight and not “swollen” as in Bison. The tooth is quite large (Supplementary Table 6) and moderately hyposodont; the enamel is thick and the roots are patent. A lower right M1 from the excavations at Evron Quarry was also attributed to Bos cf. primigenius (Tchernov et al., Reference Tchernov, Horwitz, Ronen and Lister1994) and closely resembles the finds from the Landfill site. Bos cf. primigenius has been identified in the middle Pleistocene quarry of Qadib el-Ban located within the Latamne Formation in Lebanon, where it occurs together with Bison priscus (Guérin et al., Reference Guérin, Eisenmann and Faure1993), while at the roughly contemporaneous site of Gesher Benot Ya’akov, an unspecified form of Bos was recognized (Hooijer, Reference Hooijer1959; Geraads and Tchernov, Reference Geraads and Tchernov1983; Martínez-Navarro and Rabinovich, Reference Martínez-Navarro and Rabinovich2011; Rabinovich et al., Reference Rabinovich, Gaudzinski-Windheuser, Kindler and Goren-Inbar2011). Bos primigenius is absent in the earlier site of ‘Ubeidiya (Bar-Yosef and Belmaker, Reference Bar-Yosef and Belmaker2011; Martínez-Navarro et al., Reference Martínez-Navarro, Belmaker and Bar-Yosef2012) such that finds from the ‘Evron site complex, Latamne and possibly also Gesher Benot Ya’akov may represent the earliest remains of this species in the Levant. From ca. 0.5 Ma onwards, Bos primigenius is well-established in the Levant and occurs in numerous sites (e.g. Horwitz and Monchot, Reference Horwitz and Monchot2007; Stiner et al., Reference Stiner, Barkai and Gopher2009; Reynaud Savioz, Reference Reynaud-Savioz2011; Yeshurun et al., Reference Yeshurun, Zaidner, Eisenmann, Martínez-Navarro and Bar-Oz2011; Rabinovich et al., Reference Rabinovich, Ackermann, Aladjem, Barkai, Biton, Milevski, Solodenko and Marder2012).
(4) Additional faunal remains from ‘Evron Landfill that could not be identified to taxon are: a fragment of mammalian tooth enamel; six long-bone shaft fragments from a large-sized mammal (not Proboscidean); proximal metapodial fragment of a large-sized mammal.

Figure 9. (color online) Faunal remains from ‘Evron Landfill. (a) Dama sp. Right distal humerus shaft, anterior aspect. (b) Equus cf. tabeti upper first or second molar, occlusal aspect. (c) Bos sp. lower second molar, lingual aspect. (d) Bos sp. lower second molar, buccal aspect.

Figure 10. (color online) Metric values of the Equus cf. tabeti molar from ‘Evron Landfill, compared to equid molars from Gesher Benot Ya'akov (GB12) and from 'Ubeidiya (U 1944). P3P4 = third/fourth premolar; M1M2 = first/second molar.
In sum, all taxa identified in the ‘Evron Landfill assemblage occur in other Levantine early mid-Pleistocene sites such as Latamne, Bizat Ruhama, and Gesher Benot Ya'akov.
DISCUSSION
‘Evron Landfill and other LP sites in the Southern Levant
The estimated age of ‘Evron Landfill is based here on paleomagnetic stratigraphy anchored by cosmogenic isotope burial ages. The results indicate that hominin activity in this locality took place near the BM transition, ca. 0.77 Ma.
Cosmogenic isotope dating anchors the paleomagnetic stratigraphy of Unit 5. Since this unit is not represented in the Southern sequence, its correlation to Unit 4b, in which the BM transition was identified, is unknown. In addition, no clear magnetostratigraphic affiliation could be achieved from the samples of Unit 5 in the Western and Northern sequences. Still, its stratigraphic position in both described sequences indicates that the age of Unit 5 should be near the BM transition, ~0.77 Ma. The corrected cosmogenic age of the bottom part of Unit 5, just above its contact with Unit 7 in Trench 2 (Fig. 3 and 5; Table 5) is 0.66±0.11 Ma. This result supports the magnetostratigraphic observation and provides a minimum age for the accumulation of Paleolithic finds, which originated from the contact between Units 5 and 7. A maximum age is provided by paleomagnetic stratigraphy, indicating that the base of Unit 7 is older than 1.076 Ma. The stratigraphic position of the archaeological finds, however, located in the contact between sedimentological Units 5 and 7, suggests that hominin activity took place in the early depositional phases of Unit 5, when the top of Unit 7 was exposed on the surface. Following this hypothesis, the time of deposition of the archaeological finds should be closer to the age of Unit 5, i.e., ~0.77 Ma.
These ages fit well with the faunal composition, which demonstrates similarities to other, early middle-Pleistocene sites such as Latamne, with an estimated age of 0.7–0.5 Ma (Guérin et al., Reference Guérin, Eisenmann and Faure1993; Sanlaville et al., 1993) and Evron Quarry and Gesher Benot Ya’akov, dated between 1.0–0.77 Ma (e.g., Gilead and Ronen, Reference Gilead and Ronen1977; Goren-Inbar et al., Reference Goren-Inbar, Grosman and Sharon2011; Porat and Ronen, Reference Porat and Ronen2002; Ron et al., Reference Ron, Porat, Ronen, Tchernov and Horwitz2003; Rink and Schwartz, Reference Rink and Schwarcz2005; Rabinovich et al., Reference Rabinovich, Gaudzinski-Windheuser, Kindler and Goren-Inbar2011; Yeshurun et al., Reference Yeshurun, Zaidner, Eisenmann, Martínez-Navarro and Bar-Oz2011).
All the faunal taxa identified in ‘Evron Landfill were also found, either together or singly, in other roughly contemporaneous early mid-Pleistocene Levantine sites such as of Latamne, Evron Quarry, and Gesher Benot Ya’akov. In terms of biochronology the site is then constrained between the early Pleistocene site of ‘Ubeidiya which lacks three of the taxa (i.e., E. tabeti, Dama sp., Bos cf. primigenius) and numerous late LP sites in the region (Revadim, Holon, Qesem, Hummal, Nadayouieh.) which lack E. tabeti. Though chronological inferences based on isolated finds are problematic, there is a high degree of biochronological accord when all taxa are examined.
The lithic assemblage of ‘Evron Landfill presents affinities mostly with Early Acheulian industries: hand axes are relatively coarse, displaying large, deep scars indicating the use of a hard-hammer percussion through all production stages, and a distinct component of a small-flake (>3 cm) industry is present. Still, this is a small collection and by itself is insufficient for establishing a clear cultural affiliation. Considering the suggested age range obtained through paleomagnetic stratigraphy and cosmogenic isotope burial age, however, and the biochronological data retrieved from the faunal assemblage, it is suggested that the material culture and faunal assemblage of ‘Evron Landfill are sufficiently indicative to be attributed to the Early Acheulian. The geographic proximity between ‘Evron Landfill and Evron Quarry, as well as the correlation between sedimentological units and resemblance lines observed in lithic and faunal composition between both assemblages, might indicate a connection between these two localities, possibly representing a single site.
The depositional environments of ‘Evron Landfill
Most of the units at the site are part of the ‘Evron Member of the Quaternary Hefer Formation, which are comprised of alternating red sandy loam soils (Hamra) and eolianites (Gvirtzman et al., Reference Gvirtzman, Shachnai, Bakler and Ilani1984; Sivan, Reference Sivan1995; Sivan et al., Reference Sivan, Gvirtzman and Sass1999). Lithostratigraphic and pedologic studies in Evron Quarry, located only 300 m south to the study site, have defined eight pedo-sedimentary units in the 8.5-m-thick section exposed at that site, and provide us with a geological and environmental reference (Table 8; Ronen and Amiel, Reference Ronen and Amiel1974; Ronen Reference Ronen1991).
Table 8. Correlation table of the pedo-sedimentary units exposed in ‘Evron Landfill and Evron Quarry.
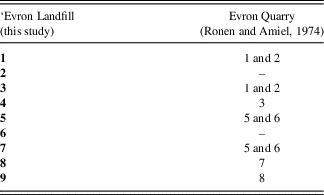
The results of the pedo-sedimentary investigation of ‘Evron Landfill reveal a ~14-m-thick complex sequence in which eight units were defined. The entire sequence can be divided into two broad depositional environments: the lower units of the sequence (Units 4–8) demonstrate dynamic coastal environments, whereas the upper units (Units 1–3) represent a more inland environment, not necessarily coastal.
The base of the landfill (Unit 9) is characterized by yellow marls, which were not sampled in this study. This unit correlates to Unit 8 in the study of Ronen and Amiel (Reference Ronen and Amiel1974), described as Miocene marl. According to Issar and Kafri (Reference Issar and Kafri1969), these marls are of early Miocene age (23–16 Ma).
Unconformably overlaying the marls is a prominent ~3-m-thick carbonate-rich beach rock (Unit 8). Its composition and inclination indicate that it formed in an ancient shore environment. This beach rock correlates to Unit 7 in Ronen and Amiel (Reference Ronen and Amiel1974), which was defined as 2–4 m thick eolianite. The facies change probably represents inland transition from beach rock to eolianite. Based on Sivan et al. (Reference Sivan, Gvirtzman and Sass1999), this unit is ascribed to the Kurdane Formation (Kafri and Ecker, Reference Kafri and Ecker1964) and is equivalent to the Pliocene Pleshet Formation (Gvirtzman, Reference Gvirtzman1970; Buchbinder, Reference Buchbinder1975). Sivan et al. (Reference Sivan, Gvirtzman and Sass1999) generated an isopach map of the Kurdani Formation in the region and concluded that the shoreline was then approximately 1 km west of our study site.
The age of the Kurdane Formation is inconclusive: it has been assigned to the Miocene (Kafri and Ecker, Reference Kafri and Ecker1964), Pliocene (Zilberman, Reference Zilberman1991), or Pliocene–Pleistocene (Gvirtzman, Reference Gvirtzman1970; Buchbinder, Reference Buchbinder1975). Corals found within this formation (Sivan, Reference Sivan1996) imply some correlation with units that are attributed to the “Calabrian” (1.8 – 0.78 Ma) or lower Pleistocene (Reiss and Issar, Reference Reiss and Issar1961). In ‘Evron Landfill, the age of the Kurdane Formation based on cosmogenic isotopes, ranges between 0.99±0.1 Ma (EQ-1) and 0.9±0.13 Ma (EQ-2). These ages correlate well with the paleomagnetic results, indicating that this unit should be older than the Jaramillo subchron, i.e., older than 1.076 Ma. Thus, we provide a more accurate age estimation for the formation of this unit.
The beach rock itself exhibits signs of pedogenesis (roots remnants), it is capped by a thick calcrete, and its upper boundary shows signs of erosion. These observations point to a long interval of subaerial exposure (and another unconformity), subsequent to the formation of the beach rock and prior the deposition of the overlying Unit 7.
Unit 7 is composed of brown sticky clay to silty clay, rich with black iron-manganese nodules. It correlates to Units 5 and 6 defined at Evron Quarry (Ronen and Amiel Reference Ronen and Amiel1974). In places, a well-developed calcic horizon appears at the upper part of the unit. The PSD of this unit is bimodal, with a dominant clay-silt mode and a second mode at the sand fraction. Most of the sediments are fine-grained and probably originated from the drainage system to the east; the minor addition of coarse grains (sand) can originate from the underlying eolianite or from the nearby coastal sediments. The appearance of a calcic horizon at the top of the unit indicates subaerial exposure for a relatively long time period. The fine-grained sediments that dominate the deposits and the widespread appearance of iron-manganese nodules indicate redux conditions, pointing to a marsh-like environment (i.e., wetland), typical adjacent to eolianite ridges found elsewhere along the coastal plain of Israel (e.g., Sivan et al., Reference Sivan, Gvirtzman and Sass1999, Reference Sivan, Wdowinski, Lambeck, Galili and Raban2001).
Unit 6 is a 1.3-m-thick eolianite, exposed only at the eastern wall of the landfill, and was not documented in previous studies. It dips to the west and is abruptly (horizontally) cut by Unit 5. Unit 6 was deposited in a coastal environment with a direct linkage to the seashore; its clear erosion towards the west resembles a sea-cliff morphology.
Unit 5, cutting both Units 6 and 7, is comprised of clay sediments similar to Unit 7 with alternating fluvial layers/lenses rich with sand grains. It correlates with Units 5 and 6 described by Ronen and Amiel (Reference Ronen and Amiel1974). The fluvial layers within this unit are composed of the nearby bedrock (mostly carbonate). Unit 5 represents varying environmental conditions, alternating both spatially and temporally between marsh and streams.
Unit 4 uncomfortably overlies Units 7, 6, and 5. It changes laterally, alternating from reddish-brown clay to dark-grayish yellow clay and to bright reddish-brown sandy loam to sandy clay loam, and is therefore probably not a chronostratigraphic unit. Due to these changes, it is hard to correlate Unit 4 to previous studies. However, it seems that at least the red clay facies should be correlated with Unit 3 described by Ronen and Amiel (Reference Ronen and Amiel1974). This unit represents spatially and temporally alternating environments, ranging from marsh to coastal environment with a direct linkage to the shore.
Units 1–3 are inland units, correlating with Ronen and Amiel’s Units 1–2 (Ronen and Amiel, Reference Ronen and Amiel1974). These units consist of silty clay to clay sediments that represent the alluvial fill of the region, originating from the mountains to the east. The well-developed calcic horizons that appear at the boundaries of these units suggests three stages of alluvial fill divided by non-depositional or erosional periods.
The stratigraphic position of the archaeological finds, alongside with the field observations mentioned above, indicate that hominin activity at ‘Evron Landfill took place on top of Unit 7, when it was subaerially exposed. This could have occurred after the coastline retreat and during the early stages of deposition of Unit 5. The presence of small flint artifacts and fragments (<3 cm) within the fluvial lenses of Unit 5 is interpreted as a secondary deposition caused by this unit cutting through the archaeological horizon. Thus, the hominin activity in ‘Evron Landfill is associated with a marsh environment.
In the scenario in which hominin activity took place in the early depositional stages of Unit 5, the suggested environment is quite a dynamic one, changing frequently between marsh and active streams. This environment presents a preferred setting for hominin activity; fresh water brought by the streams sustained a rich biotope where animals and plants could be obtained, both here and at the nearby marsh.
Together, the faunal remains from Evron Quarry (Tchernov et al., Reference Tchernov, Horwitz, Ronen and Lister1994) and the ‘Evron Landfill locality, confirm the presence at the site of an impressive biogeographical mix comprising taxa originating from at least three different biogeographic provinces; Ethiopian (e.g., E. cf. tabeti, Kolpochoerus, and Alcelaphus), Oriental (e.g., Elephas sp. and Stegodon sp.) and Palaearctic (Bos sp. and Cervidae). As noted by Tchernov et al. (Reference Tchernov, Horwitz, Ronen and Lister1994), it is unclear whether the African element in the Evron fauna represents a continuation of the early Pleistocene ‘Ubeidiya dispersal event, or is a new post-‘Ubeidiya occurrence. The presence of two grazers (an equid and a large bovid) as well as a cervid (browser) in the new finds suggests a mixed environment comprising open steppe as well as woodland. This supports the paleoecological reconstruction from the previous investigation at Evron Quarry (Tchernov et al., Reference Tchernov, Horwitz, Ronen and Lister1994) denoting a mosaic landscape of open, steppic country (Gazella, cf. Alcelaphus), woodlands (Cervidae, Stegodon sp., Bos. sp.), bare rocky slopes (cf. Gerbillus cf. dayurus), and water bodies (Hippopotamus, Trionyx) in close proximity to the site.
CONCLUSIONS
A minimum age for the hominin activity in ‘Evron Landfill was determined based on cosmogenic isotope burial age of the sediments overlaying the horizon from which archaeological finds originated, yielding an age of 0.66±0.11 Ma. This result agrees with the paleomagnetic stratigraphy of the archaeological finds, which places it near the BM transition (ca. 0.77 Ma). A pedo-sedimentary study indicated that hominin activity primarily took place within a marsh environment, which was changing both spatially and temporally to an active stream, not far from the Mediterranean shore. Comparative analyses of the flint and faunal assemblages display similarities between the Landfill locality and the nearby Evron Quarry site, suggesting that this newly identified locality should be ascribed to the same cultural phase, i.e., the Early Acheulian.
ACKNOWLEDGMENTS
The excavation at ‘Evron Landfill was directed by Maayan Shemer (Field photography) and Omry Barzilai on behalf of the Israel Antiquity Authority (IAA; Permit A7414/2015), and was financed by Kibbutz ‘Evron. Test trenches were conducted by Alla Yaroshevich. We thank Mrs. Argita Levanon and The National Laboratory for Digital Documentation of Archaeological Artifacts, for the digital documentation of selected flint artifacts found during the project; Mrs. Clara Amit for studio photographs of the finds and Mr. Mendel Kahn for map drafting and GPS documentation. The authors also would like to extend their gratitude to the IAA department of publications for permission granted to publish the assemblages collected during the excavation. In loving memory of Professor Avraham Ronen, an exceptional researcher and a dear colleague, excavator of Evron Quarry.
SUPPLEMENTARY MATERIALS
To view supplementary material for this article, please visit https://doi.org/10.1017/qua.2018.107