INTRODUCTION
Exploring the relationship between environmental change and human adaptation during the Holocene is crucial to a better understanding of ancient human-environment interactions (Butzer, Reference Butzer2012; Hsiang et al., Reference Hsiang, Burke and Miguel2013). The rapid climatic deterioration ca. 4200 cal yr BP has been termed the “Holocene Event 3” (Perry and Hsu, Reference Perry and Hsu2000), and its significant effect on ancient civilizations has gained worldwide attention (Weiss et al., Reference Weiss, Courty, Wetterstrom, Guichard, Senior, Meadow and Curnow1993; Cullen et al., Reference Cullen, Demenocal, Hemming, Hemming, Brown, Guilderson and Sirocko2000; deMenocal, Reference deMenocal2001; Staubwasser et al., Reference Staubwasser, Sirocko, Grootes and Segl2003; Wu and Liu, Reference Wu and Liu2004; Lawler, Reference Lawler2007; Sun et al., Reference Sun, Liu, Wünnemann, Peng, Jiang, Deng, Chen, Li and Chen2019). Almost all prehistoric archaeological cultures in border regions in China declined during this climate event (Wang, Reference Wang2004; Wu and Liu, Reference Wu and Liu2004; An et al., Reference An, Tang, Barton and Chen2005; Chen et al., Reference Chen, Wang, Schneiderman, Tao and Cai2005; Wu et al., Reference Wu, Zhu, Zheng, Li, Wang, Li and Sun2014; Jia et al., Reference Jia, Yi, Sun, Wu, Lee, Wang and Lu2017; Xu et al., Reference Xu, Liu, Sun, Chen, Cheng and Chen2017; Guo et al., Reference Guo, Xiong., Ding, Jin, Wu and Ye2018a; Sun et al., Reference Sun, Liu, Wünnemann, Peng, Jiang, Deng, Chen, Li and Chen2019). However, three dominant civilization centers emerged after the “Holocene Event 3” in northern China (Zhang, Reference Zhang1997), including the Erlitou Culture in the Central Plain (3800–3500 cal yr BP) (Zhang et al., Reference Zhang, Pollard, Rawson, Huan, Liu and Tang2019), the Yueshi Culture in the Haidai region (3800–3450 cal yr BP) (Fang, Reference Fang1998), and the Lower Xiajiadian Culture in the West Liao River Basin (4000–3500 cal yr BP) (Shelach, Reference Shelach1994; Shelach et al., Reference Shelach, Raphael and Jaffe2011).
The West Liao River Basin was one of the centers of the origin of Chinese civilization. The site of Xinglongwa is considered to be one of the earliest broomcorn millet cultivation sites (7800 cal yr BP) (Jones and Liu, Reference Jones and Liu2009; Zhao Reference Zhao2014). The finely carved jades that were excavated from the Hongshan Culture Niuheliang site first appeared ca. 5500 yr BP (Lawler, Reference Lawler2009). Ultimately, an advanced society was founded during the Lower Xiajiadian period, defined by the construction of stone cities such as the Sanzuodian site (Shelach, Reference Shelach1994; Shelach et al., Reference Shelach, Raphael and Jaffe2011). Previous research has shown that there was a 500 year cycle of human activity intensity, which was driven by climate changes in northeastern China during the Neolithic and Bronze ages (Xu et al., Reference Xu, Lu, Chu, Liu, Shen, Li, Wang and Wu2019). The warm and wet climate facilitated cultural prosperity and population growth, including the Xinglongwa, Hongshan, and Lower Xiajiadian cultures (Xia et al., Reference Xia, Deng and Wu2000; Jin and Liu, Reference Jin and Liu2001; Q. Xu et al., Reference Xu, Yang, Cui, Yang and Liang2002; Li et al., Reference Li, Wilis, Zhou and Cui2006; Jia et al., Reference Jia, Yi, Sun, Wu, Lee, Wang and Lu2017; D. Xu et al., Reference Xu, Lu, Chu, Liu, Shen, Li, Wang and Wu2019). The prosperity of the Lower Xiajiadian Culture has been attributed to the development of millet agriculture in the warm and wet climate (Jia et al., Reference Jia, Sun, Wang, Sun, Zhao, Lee, Huang, Wu and Lu2016a, Reference Jia, Yi, Sun, Wu, Lee, Wang and Lu2017). Nevertheless, in the West Liao River Basin, only scattered qualitative paleoclimate records exist that cover the Lower Xiajiadian period (Xu et al., Reference Xu, Yang, Cui, Yang and Liang2002; Li et al., Reference Li, Wilis, Zhou and Cui2006; Mu et al., Reference Mu, Qin, Zhang and Xu2016; Guo et al., Reference Guo, Xiong, Yang, Ye, Jin, Wu and Zhao2018b; Zhao et al., Reference Zhao, Xia, Jin, Jia and Liu2018), and these are inadequate for us to explore the ancient human-environment relationships in the region.
As the key component of biodiversity, plant diversity plays a vital role in the conservation and sustainable growth of habitats around the world (Wilson and Ehrlich, Reference Wilson and Ehrlich1991; Pimm et al., Reference Pimm, Russell, Gittleman and Brooks1995; Thomas et al., Reference Thomas, Cameron, Green, Bakkenes, Beaumont, Collingham and Erasmus2004). Based on plant microfossils (pollen, phytoliths, starch granules), plant macrofossils (seeds, charcoal, wood), and biomarkers (aromatic hydrocarbons), it is possible to reconstruct the past vegetation composition, ancient agricultural evolution, and climate change (Piperno, Reference Piperno1988; Birks and Line, Reference Birks and Line1992; Shackleton and Prins, Reference Shackleton and Prins1992, Wang and Lu, Reference Wang and Lu1993; Piperno and Pearsall, Reference Piperno and Pearsall1998; Odgaard, Reference Odgaard1999; Jérémy et al., Reference Jérémy, Disnar, Arnaud, Chapron, Debret, Lallier, Desmet and Revel2008; Zhao, Reference Zhao2014; Matthias et al., Reference Matthias, Semmler and Giesecke2015; Chen et al., Reference Chen, Xu, Chen, Birks, Liu, Zhang and Jin2015a, Reference Chen, Dong, Zhang, Liu, Jia, An and Mab; Li et al., Reference Li, Gaillard, Cao, Herzschuh, Sugita, Tarasov and Wagner2020; Zhou et al., Reference Zhou, Yu, Spengler, Shen, Zhao, Ge and Bao2020). The investigation of ancient human-environment relationships requires a precise chronology of paleoclimate changes and human activities. However, there are often inconsistencies between the paleoclimate records obtained from sediments and the human activity records obtained from archaeological sites. For example, the lake and loess sediment records provide a large number of quantitative paleoclimate reconstructions (Chen et al., Reference Chen, Xu, Chen, Birks, Liu, Zhang and Jin2015a; Cao et al., Reference Cao, Tian, Telford, Ni, Xu, Chen, Liu, Stebich, Zhao and Herzschuh2017; Herzschuh et al., Reference Herzschuh, Cao, Laepple, Dallmeyer, Telford, Ni, Chen, Kong, Liu and Liu2019; Zhang et al., Reference Zhang, Li, Li, Xu, Zhang, Ge, Li, Deng, Li and Zhang2020), but due to the reservoir effect, it is difficult to obtain accurate chronological frameworks (Geyh et al., Reference Geyh, Schotterer and Grosjean1997; Christine et al., Reference Christine, Pessenda, Lang and Paterne2001; Stein et al., Reference Stein, Lazar and Goldstein2013, Long et al., Reference Long, Shen, Wang, Gao and Frechen2015).
First-hand paleoclimate records reconstructed from materials in archaeological sites are likely to help solve the age non-correspondence problems. Fortunately, since humans have been able to manipulate fire, large amounts of fossil charcoals remain in archaeological sites. Owing to the incomplete combustion of wood (McGinnes et al., Reference McGinnes, Szopa and Phelps1974), plenty of fossil charcoals that have retained their anatomical characteristics can be retrieved from the archaeological sites. In addition, reconstructions based on fossil charcoals are more responsive to local environmental conditions, owing to the immobility of large pieces of charcoal (Blackford, Reference Blackford2000). Furthermore, in prehistoric periods, human activities were likely confined to 5 km or 1 hour walking distance from the settlement (Shackleton and Prins, Reference Shackleton and Prins1992; Rodríguez, Reference Rodríguez2004; Fontenari et al., Reference Fontenari, Franceschetti, Sorrentino, Mussi, Pasolli, Napolitano and Flor2005; Li et al., Reference Li, Sun, Dodson, Zhou and Zhao2013). This also ensures that fossil charcoal, the by-product of human activities, reflects local scales of human-environment interactions (Vita-Finzi, Reference Vita-Finzi, Cooke and Johnson1969). Therefore, the fossil charcoals from archaeological sites were ideal materials for local vegetation reconstruction. They provide ideal materials for revealing paleo-vegetation, paleoclimate, and past human activities (Figueiral and Mosbrugger, Reference Figueiral and Mosbrugger2000; Cui et al., Reference Cui, Li, Hu, Yao and Li2002; Asouti and Austin, Reference Asouti and Austin2005; Asouti and Fuller, Reference Asouti and Fuller2008; Sun and Li, Reference Sun and Li2012; X. Li et al., Reference Li, Sun, Dodson, Zhou and Zhao2013; An et al., Reference An, Li, Dong, Chen, Zhao and Shi2014; Wang et al., Reference Wang, Wang, Wang, Liang, Qi and Ren2016; H. Li et al., Reference Li, An, Dong, Hu, Wang, Zhao, Yang and Yang2017; Liu et al., Reference Liu, Li, Cui, Yang, Lee, Ding, Hou and Dong2019a, Reference Liu, Yang, Shi, Storozum and Dongb). However, there have been only four cases of quantitative reconstructions of paleoclimate from fossil charcoals in western China (Sun and Li, Reference Sun and Li2012; Li et al., Reference Li, Sun, Dodson, Zhou and Zhao2013; N. Sun et al., Reference Sun, Li, Shang, Zhou and Dodson2014, Reference Sun N., Dodson J, Zhou and Yang2016; Wang et al., Reference Wang, Wang, Wang, Liang, Qi and Ren2016), and none located in eastern China, particularly in places where Chinese civilization originated, such as the Central Plain, the Haidai Region, and the West Liao River Basin.
The use of paleoenvironmental reconstructions based on fossil charcoal obtained from archaeological sites in the pivotal areas and periods of civilization origin is of great significance for exploring the environmental background and its influence on civilization. In this study, we reconstructed the vegetation and paleoclimate at the Erdaojingzi site using fossil charcoals. Combined with other archaeological materials unearthed from this site, we explored the ancient human-environment interactions in the West Liao River Basin (i.e., the eastern part of the agricultural-pastoral zone in northern China) during the Lower Xiajiadian period.
STUDY AREA
The Erdaojingzi site (119.08°N, 42.17°E, 617 m above sea level [a.s.l.]) is located in the Hongshan District to the southeast of Chifeng City, Inner Mongolia (Figure 1). Five thousand two hundred square meters were uncovered, and 305 archaeological features were unearthed, including city walls, surrounding ditches (moats), courtyards, house foundations, paths, cellars, ash pits and burials. In addition, the site contained 1000 excavated items filled with pottery wares, stone and bone implements, jades, and other artifacts (Cao et al., Reference Cao, Sun. and Dang2010). The excavation at the Erdaojingzi site provided sufficient materials to examine the human-environment relationships during a crucial stage of the origin of civilization.

Figure 1. (color online) Location of the Erdaojingzi site in Chifeng, Inner Mongolia, northeast China. (a) Map of China; (b) location of the Erdaojingzi site; (c) landscape of the Erdaojingzi site.
Chifeng is located in the southeast portion of Inner Mongolia, the eastern part of the farming-pastoral zone, in northeast China. This area also contains extensive loess accumulations, with loess tableland, hills, valleys, and other landform types. The West Liao River joins the upper branches of the Xar Moron River and the Laoha River (Figure 1), and feeds into the Liao River, ultimately flowing into the Bohai Sea. Uplift of the Great Khingan Mountains resulted in higher topography west of the West Liao River Basin. The average elevation ranges from 2833 m a.s.l. in the west to 280 m a.s.l. in the east, with mountains gradually giving way to hills and eventually to plains. The precipitation diminishes from the southeast (500 mm) to northwest (200 mm) owing to the characteristics of the East Asian monsoon, which likewise lessens from southeast to northwest (An et al., Reference An, Porter, Kutzbach, Wu, Wang, Liu, Li and Zhou2000). According to the meteorological data provided by the Chifeng Weather Station (http://data.cma.cn/), the average annual temperature is 7.8°C (with an annual extreme maximum and minimum temperature of 40.4 and −27.8°C, respectively), the average annual precipitation is 370 mm, and the average annual relative humidity is 50%. The temperate semi-humid and semi-arid continental monsoon climate promotes the vegetation of the temperate grassland in this area.
Today, shrub meadow dominates the vegetation around the Erdaojingzi site, which is situated in the Loess hills and lower hilly areas of southeastern Chifeng. The main shrubs include Lespedeza bicolor Turcz., Vitex negundo Linn., Ziziphus jujube var. spinose (Bunge) Hu, Hoppophae Linn., Armeniaca sibirica (Linn.) Lam., among others. Some sporadic trees are distributed on the shrub grassland, including Pinus tabularformis Carr., Ulmus pumila Linn., Sophora japonica Linn., and Populus Linn. (Local History Compilation Committee of Chifeng, 1996).
METHODS
Radiocarbon dating
Two carbonized seed samples collected from the archaeological sites were dated with accelerator mass spectrometry (AMS) by Beta Analytic in Miami, Florida, USA. The IntCal20 curve (Reimer et al., Reference Reimer, Austin, Bard, Bayliss, Blackwell, Bronk Ramsey and Butzin2020) and the Libby half-life of 5568 years were used in calculating all ages, with the calibration performed using the OxCal 4.4 program (https://c14.arch.ox.ac.uk/oxcal/OxCal.html). All ages reported are relative to AD 1950 (referred to as “cal yr BP”).
Plant flotation and fossil charcoal identification
The application of flotation and the identification of plant remains have greatly promoted the study of the subsistence strategies of prehistoric societies in recent years (Zhao, Reference Zhao2010). Eighty-four soil samples with a total volume of 405 liters were collected for flotation. Carbonized plant remains were obtained using a sieve with #80 mesh (aperture size of 0.2 mm) and then dried in the shade and sorted. Five thousand eight hundred eleven fossil charcoals were collected with a sieve with an aperture size of 2 mm. A metallographic microscope (Nikon LV150) was employed to identify the microstructure of the fossil charcoals using the cross, radial, and tangential sections, and the plant taxa were identified based on wood anatomy atlases (Cheng et al., Reference Cheng, Yang and Liu1992; Yao, Reference Yao1988). The fossil charcoals with obvious morphological characteristics were adhered to an aluminum sample stage and were overgilded on the sample surface by an ion-sputtering instrument. Then, they were scanned by a Quanta 650 electron microscope. Based on the results of the identification of the fossil charcoals, the percent abundance ratio and frequency of each taxon were calculated. All samples were identified in the Archaeobotany Laboratory, Institute of Archaeology, Chinese Academy of Social Sciences.
Quantitative reconstruction of the paleoclimate
The coexistence approach (CA) is based on the assumption that fossil plants have similar climatic requirements as their nearest living relative species. The CA provides a quantitative estimate of past climate by setting out the climatic tolerances of individual species, and then identifying the range of climatic conditions under which all species in a fossil assemblage could co-exist today (Mosbrugger and Utescher, Reference Mosbrugger and Utescher1997). The coexistence approach (CA) was employed to quantitatively reconstruct the paleoclimate in the Erdaojingzi site associated with the Lower Xiajiadian Culture. Based on the similar living environment of modern plants, the climatic tolerance ranges of every taxon of the fossil charcoals were derived from Fang et al. (Reference Fang, Wang and Tang2009), including the maximum and minimum of the mean annual temperature (MAT), mean annual precipitation (MAP), and index of moisture content in the soil (IM) (Fang and Yoda, Reference Fang and Yoda1990), respectively.
RESULTS
Radiocarbon dating
Systematic archaeological excavation confirmed that the Erdaojingzi site is an archaeological site that belongs in the Lower Xiajiadian period, and no remains indicate that it fits other periods (Cao et al., Reference Cao, Sun. and Dang2010). The age range of Lower Xiajiadian Culture is 3900–2800 cal yr BP, based on 32 radiocarbon dates in the West Liao River Basin (Jia et al., Reference Jia, Yi, Sun, Wu, Lee, Wang and Lu2017). The two calibrated 14C ages of the Erdaojingzi site are within the range of 3600–3500 cal yr BP. The calibrated 14C ages from charcoals collected in the Erdaojingzi site are shown in Table 1.
Table 1. 14C dates from the Erdaojingzi site.

Fossil charcoals
Five thousand eight hundred eleven charcoal fragments were identified, and 21 different taxa were identified in 84 samples from the Erdaojingzi site (Fig. 2, Table 2). The three most abundant types of fossil charcoals made up 75.03% of the total, including Quercus (2031, 34.95%), Pinus (1477, 25.42%), and Ulmus (852, 14.66%). There were also seven types of fossil charcoals with more than 100 pieces, including Tilia (271, 4.66%), Populus (270, 4.65%), Betula (191, 3.29%), Amygdalus/Armeniaca (190, 3.27%), Phellodendron (159, 2.74%), Cotoneaster (128, 2.20%), and Salix (102, 1.76%). There were also seven types that appeared sporadically, including Acer (23, 0.40%), Ziziphus (19, 0.33%), Alnus (9, 0.15%), Koelreuteria (3, 0.05%), Rhamnus (3, 0.05%), Fraxinus (2, 0.03%), and Ostrya (1, 0.02%). Four different types of fossil charcoals could not be identified.
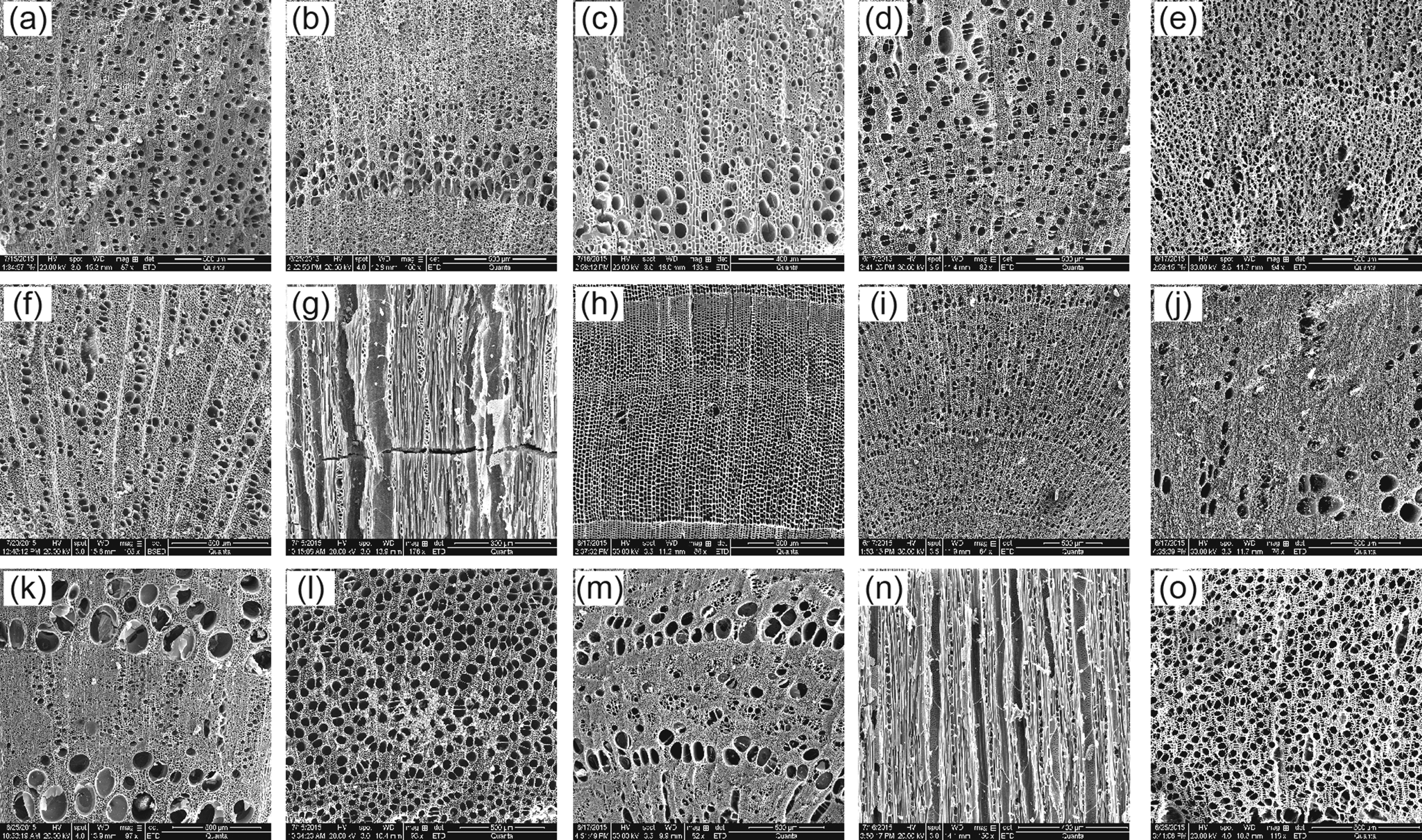
Figure 2. Microstructures of charcoal from the Erdaojingzi site; (g) and (n) are tangential sections, others are cross-sections. (a) Acer sp.; (b, c) Amygdalus sp./Armeniaca sp.; (d) Betula sp.; (e) Cotoneaster sp.; (f) Ostrya sp.; (g) Phellodendron sp.; (h) Pinus sp.; (i) Populus sp.; (j, k) Quercus sp.; (l) Salix sp.; (m) Ulmus sp.; (n) Ziziphus sp.; (o) unknown 4.
Table 2. The fossil charcoal assemblages from the Erdaojingzi site.
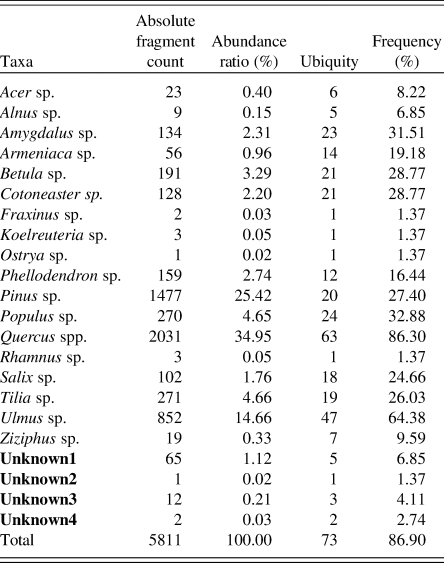
With the exception of Pinus, a temperate evergreen conifer, most of the woody plants in China are broad-leaved deciduous taxa, including Quercus, Ulmus, Tilia, Populus, Betula, Amygdalus, Armeniaca, Phellodendron, Cotoneaster, Salix, Acer, Ziziphus, Alnus, Koelreuteria, Rhamnus, Fraxinus, and Ostrya (Wu, Reference Wu1980). Thus, the fossil charcoal assemblage at Erdaojingzi indicates the presence of temperate deciduous and mixed conifer-broadleaved forests ca. 3500 cal yr BP, distinct from the shrub meadow vegetation found there today.
Quantitative reconstruction of paleoclimate
The climatic factors were obtained by applying CA based on 21 fossil charcoal taxa from the Erdaojingzi site (Table 3). The results indicated that MAT was 2.0–13.8°C, MAP was 471–1073 mm, and IM was -28.7–74.1 (Fig. 3).

Figure 3. Coexistence intervals for the Erdaojingzi site (shadow represents the overlapping climate range, dotted line represents the modern climate in the areas).
Table 3. The climate ranges (from Fang et al., Reference Fang, Wang and Tang2009) of individual plant taxa in the Erdaojingzi site.

DISCUSSION
Paleovegetation and paleoclimate
Because plant species have particular climate tolerances (Thornthwaite, Reference Thornthwaite1948; Fang and Yoda, Reference Fang and Yoda1990; Wang, Reference Wang1992), plant composition from sedimentary records or archaeobotanical assemblages can indicate climate history (Piperno, Reference Piperno1988; Birks and Line, Reference Birks and Line1992; Shackleton and Prins, Reference Shackleton and Prins1992; Wang and Lu, Reference Wang and Lu1993; Odgaard, Reference Odgaard1999; Matthias et al., Reference Matthias, Semmler and Giesecke2015; Chen et al., Reference Chen, Xu, Chen, Birks, Liu, Zhang and Jin2015a; Cao et al., Reference Cao, Tian, Telford, Ni, Xu, Chen, Liu, Stebich, Zhao and Herzschuh2017; Xu et al., Reference Xu, Liu, Sun, Chen, Cheng and Chen2017; Li et al., Reference Li, Gaillard, Cao, Herzschuh, Sugita, Tarasov and Wagner2020). Pollen-based vegetation and climate reconstructions typically reflect the paleoenvironmental conditions in a wide area due to the long distance of pollen dispersion (Xu et al., Reference Xu, Li, Zhang, Zhang, Zhang and Lu2015). The pollen of herbaceous plants dominated the pollen assemblage (60–80%) from the Wangxianggou profile in this area during the Lower Xiajiadian period, including Artemisia, Chenopodiaceae, and Fagopyrum (Li et al., Reference Li, Wilis, Zhou and Cui2006). Furthermore, 30% of the area was covered with woody plants (Li et al., Reference Li, Gaillard, Cao, Herzschuh, Sugita, Tarasov and Wagner2020), including temperate broadleaved trees such as Quercus, Ulmus, Betula, Juglans, Alnus, Castanea, Celtis, Anacardiaceae, Pterocarya, Tilia, and Salix, and temperate coniferous trees such as Pinus (Li et al., Reference Li, Wilis, Zhou and Cui2006). However, there are some differences in pollen assemblage between the archaeological sites and the natural profiles. A large number of pollen specimens in the Dadianzi and Chengzishan sites in the Lower Xiajiadian period were identified as Pinus (Kong et al., Reference Kong, Du, Liu, Yang and Zhou1991; Zhao et al., Reference Zhao, Li, Shang, Zhou and Sun2009). Our pollen records, which were obtained from the natural sand-dune profiles in the West Liao River Basin, indicated that temperate grassland was the dominant vegetation in this area during the Lower Xiajiadian period (Jia et al., Reference Jia, Yi, Sun, Wu, Lee, Wang and Lu2017). Such a difference may be attributable to secondary accumulation in the archaeological strata.
Fortunately, the woody plants that were completely recovered by the fossil charcoals obtained from the Erdaojingzi site can make up for the deficiency of pollen in grassland vegetation. There were 21 different woody plants growing near the Erdaojingzi site ca. 3500 cal yr BP, including Quercus, Pinus, Ulmus, Tilia, Populus, Betula, Phellodendron, Amygdalus/Armeniaca, Cotoneaster, Salix, Acer, Ziziphus, Alnus, Koelreuteria, Rhamnus, Fraxinus, Ostrya, and four unknown plants (Table 2). However, six species of woody plants that were found in this area 3500 cal yr BP are not distributed here today, including Phellodendron, Cotoneaster, Alnus, Koelreuteria, Fraxinus, and Ostrya. Three types of woody plants probably were the dominant species, including Quercus (34.95%), Pinus (25.42%), and Ulmus (14.66%). Seven other woody plants probably appeared around the site because their percent abundance ratio all exceed 1%, including Populus (4.65%), Amygdalus/Armeniaca (3.27%), Cotoneaster (2.20%), Betula (3.29%), Salix (1.76%), Tilia (4.66%), and Phellodendron (2.74%). Also, some woody plants appeared sporadically, as indicated by their small amount of charcoal remains, such as Acer (0.40%), Ziziphus (0.33%), Alnus (0.15%), Koelreuteria (0.05%), Rhamnus (0.05%), Fraxinus (0.03%), and Ostrya (0.02%). The unearthed percent ubiquity refers to the percentage of a specific taxon of charcoal in all samples. It was employed to reflect the combination of woody vegetation around the site in the corresponding period (Salisbury and Jane, Reference Salisbury and Jane1940). As reflected by both the percentage of absolute number and unearthed percent ubiquity, Quercus (34.95%, 86.3%) and Ulmus (14.66%, 64.38%) were likely to be the dominant woody plants distributed around the Erdaojingzi site ca. 3500 cal yr BP. Most woody plants are temperate broad-leaved deciduous taxa, except the evergreen coniferous plants of Pinus.
Therefore, the assemblages of fossil charcoal at the Erdaojingzi sites reflected that temperate deciduous and mixed conifer-broadleaved forests were found near the site ca. 3500 cal yr BP. Similar vegetation types occur in the middle and lower mountain areas in the Songnen Plain and the Liaohe Plain, as well as the low-elevation area of the Changbai Mountains in the east nowadays (Wu, Reference Wu1980).
Almost all climate records from soil/sand-dune profiles (Ren, Reference Ren1998; Xia et al., Reference Xia, Deng and Wu2000; Jin and Liu, Reference Jin and Liu2001; Xu et al., Reference Xu, Yang, Cui, Yang and Liang2002; Yang et al., Reference Yang, Wang, Zhou, Lai and Long2012; Zhao et al., Reference Zhao, Xia, Jin, Jia and Liu2018; Xue et al., Reference Xue, Jin, Liu, Sun and Liu2020), lakes (Li et al., Reference Li, Wilis, Zhou and Cui2006), and archaeological samples (Kong et al., Reference Kong, Du, Liu, Yang and Zhou1991) indicated a wet climate in the West Liao River Basin ca. 3500 BP. Because previous studies only qualitatively describe the climate in our study area, we made an attempt to use fossil charcoals obtained from the Erdaojingzi site to reconstruct the past climate. Based on CA, the MAT ca. 3500 cal yr BP was 7.9 ± 5.9°C, which is very similar to the present one (7.8°C), while MAP was almost halved, from 772 ± 301 mm at ca. 3500 cal yr BP to 370 mm currently (Fig. 3). Also, the moisture index reduced sharply from 22.7 ± 51.4 at ca. 3500 cal yr BP (Fig. 3) to −30 ± 10 nowadays (Fang et al., Reference Fang, Wang and Tang2009). The charcoal assemblage at the Erdaojingzi site indicated a relatively wetter climate for the temperate zone in the West Liao River Basin. This echoes previous results about the past climate in our study area (Ren, Reference Ren1998; Xia et al., Reference Xia, Deng and Wu2000; Jin and Liu, Reference Jin and Liu2001; Xu et al., Reference Xu, Yang, Cui, Yang and Liang2002; Li et al., Reference Li, Wilis, Zhou and Cui2006; Yang et al., Reference Yang, Wang, Zhou, Lai and Long2012; Zhao et al., Reference Zhao, Xia, Jin, Jia and Liu2018; Xue et al., Reference Xue, Jin, Liu, Sun and Liu2020). The past forest was dominated by temperate deciduous and mixed conifer-broadleaved species, such as Quercus, Pinus, and Ulmus.
Human utilization of wood resources
Timber is one of the main resources for humans and is closely related to human activities identified by archaeological remains (Shackleton and Prins, Reference Shackleton and Prins1992). There were four main purposes for human utilization of woody plant resources: building materials, making tools, fuelwood, and food. In addition to being used as firewood, almost all woody plants in the Erdaojingzi site could be utilized as construction and tool-making materials.
Archaeobotanical studies of carbonized seeds have shown that ancient people were engaged in dry farming of predominantly foxtail millet at the Erdaojingzi site (Y. Sun et al., Reference Sun, Zhao, Cao, Sun and Dang2014). Apart from agriculture, fossil charcoals indicate that local people may also have engaged in some gathering activities (Y. Sun et al., Reference Sun, Zhao, Cao, Sun and Dang2014). Four woody plants have also been used as food today, including Quercus, Ulmus, Amygdalus/Armeniaca, and Ziziphus. Quercus probably was dominant in the gathering, comprising the most fossil charcoals identified from the Erdaojingzi site (Table 2). Acorns, which are the nuts of Quercus, are considered to be an important component of gathering because they were often found in the archaeological sites of China (Zhao, Reference Zhao2006; Fuller et al., Reference Fuller, Harvey and Qin2007; Sun et al., Reference Sun, Huang and Zheng2007; Yang et al., Reference Yang, Yu, Lü, Cui, Guo and Ge2009; Fuller and Qin, Reference Fuller and Qin2010; Ma et al., Reference Ma, Yang, Zhang, Sun and Jia2016). The acorns also have been processed by humans since the Neolithic Age in the West Liao River Basin (Ma et al., Reference Ma, Yang, Zhang, Sun and Jia2016), and were probably chosen as a useful supplement for food resources in the Erdaojingzi site due to their rich starch content. The second-largest number of fossil charcoals comes from Ulmus. Owing to the edibility of the tender leaves, Ulmus could provide a certain amount of food resources in the spring when food is relatively scarce. Utilization of the leaves of Ulmus was also reflected by a clay pot discovered at the Shigu site in Henan (Xu, Reference Xu1996). Apart from serving as primary food resources, some fruit-bearing woody plants were selected as supplementary food sources in ancient times, such as Amygdalus/Armeniaca and Ziziphus (42.47% and 9.59% of the unearthed percent ubiquity, respectively).
Implications for Lower Xiajiadian Culture
The relatively wet climate may represent a warm temperate humid climate in the West Liao River Basin ca. 3500 cal yr BP, which is significantly different from the middle temperate semi-humid and semi-arid climate today. Climate change has not only caused the transition of regional forest grassland vegetation ca. 3500 cal yr BP to today's retama bushland vegetation, but also facilitated the significant development of rain-fed agriculture. The main subsistence strategies transformed from a mixture of multiple survival patterns during the Neolithic Age (before 4000 cal yr BP) (Jia et al., Reference Jia, Lee, Zhang, Wang, Sun, Zhao, Yi, Huang and Lu2016b, Reference Jia, Yi, Sun, Wu, Lee, Wang and Lu2017) to millet-dominated agriculture during the Lower Xiajiadian period (Zhao et al., Reference Zhao, Li, Shang, Zhou and Sun2009; Y. Sun et al., Reference Sun, Zhao, Cao, Sun and Dang2014; Jia et al., Reference Jia, Sun, Wang, Sun, Zhao, Lee, Huang, Wu and Lu2016a, Reference Jia, Yi, Sun, Wu, Lee, Wang and Lu2017). Developed agriculture provided stable food resources for human beings, promoted the emergence of large settlements, such as Sanzuodian and Erdaojingzi (Cao et al., Reference Cao, Sun. and Dang2010; Shelach et al., Reference Shelach, Raphael and Jaffe2011), and further facilitated the birth of advanced civilization. However, good times did not last long: the 500 year cycle of climate deterioration destroyed the agricultural system in the West Liao River Basin after 3500 BP (Jia et al., Reference Jia, Sun, Wang, Sun, Zhao, Lee, Huang, Wu and Lu2016a, Reference Jia, Yi, Sun, Wu, Lee, Wang and Lu2017; Xu et al., Reference Xu, Lu, Chu, Liu, Shen, Li, Wang and Wu2019). Subject to the influence of the East Asian summer monsoon, the precipitation gradually decreased from the southeast to the northwest. The agricultural groups of the Lower Xiajiadian Culture probably followed the precipitation change and migrated southward to the North China Plain, then moved southward and integrated with the cultural system in central China.
CONCLUSION
The fossil charcoals indicate that temperate deciduous and mixed conifer-broadleaved forests were the dominant vegetation near the Erdaojingzi site at ca. 3500 cal yr BP. The three major woody plants discovered are Quercus, Pinus, and Ulmus, representative species of the warm temperate forest-steppe.
In addition to the use of woody plants for building materials, making tools, and fuel, there were four woody plants that probably supplied food for ancient humans in the Erdaojingzi site. The nuts of some woody plants such as Quercus were taken as staple foods because of their rich starch or cellulose content. The tender leaves of Ulmus might have served as supplementary food sources for humans in spring when food was relatively scarce. The other two woody plants, namely Amygdalus/Armeniaca and Ziziphus, probably provided fruits to humans.
The CA results of the quantitative reconstruction indicate that MAT was 7.9 ± 5.9°C and MAP was 772 ± 301 mm. The past environment was significantly wetter than that today. The wet climate facilitated significant development of rain-fed agriculture, the emergence of large settlements such as Sanzuodian and Erdaojingzi, and the birth of advanced civilization. Also, subject to the influence of the East Asian summer monsoon, precipitation change likely caused the agricultural groups of the Lower Xiajiadian Culture to migrate southward to the North China Plain, and then to move southward and integrate with the cultural system in central China.
ACKNOWLEDGMENTS
This research was supported by the National Social Science Foundation of China (Grant Nos. 17BKG031) and the National Natural Science Foundation of China (Grant Nos. 41771223).