Introduction
An attenuated blood-oxygen-level dependent (BOLD) response in the ventral striatum (VS) during reward anticipation has been a consistent finding in functional magnetic resonance imaging (fMRI) studies of ultra-high risk (UHR) (Juckel et al., Reference Juckel, Friedel, Koslowski, Witthaus, Ozgürdal, Gudlowski, Knutson, Wrase, Brüne, Heinz and Schlagenhauf2012; Roiser et al., Reference Roiser, Howes, Chaddock, Joyce and McGuire2013), antipsychotic-naïve (Esslinger et al., Reference Esslinger, Englisch, Inta, Rausch, Schirmbeck, Mier, Kirsch, Meyer-Lindenberg and Zink2012; Nielsen et al., Reference Nielsen, Rostrup, Wulff, Bak, Broberg, Lublin, Kapur and Glenthoj2012a, Reference Nielsen, Rostrup, Wulff, Bak, Lublin, Kapur and Glenthøj2012b) and unmedicated schizophrenia patients (Juckel et al., Reference Juckel, Schlagenhauf, Koslowski, Filonov, Wüstenberg, Villringer, Knutson, Kienast, Gallinat, Wrase and Heinz2006; Schlagenhauf et al., Reference Schlagenhauf, Sterzer, Schmack, Ballmaier, Rapp, Wrase, Juckel, Gallinat and Heinz2009, Reference Schlagenhauf, Huys, Deserno, Rapp, Beck, Heinze, Dolan and Heinz2014), as well as medicated patients, as reviewed by Radua et al. (Reference Radua, Schmidt, Borgwardt, Heinz, Schlagenhauf, McGuire and Fusar-Poli2015). Preclinical data indicate that subcortical dopamine systems play a distinct role in incentive salience and reward prediction (Berridge and Robinson, Reference Berridge and Robinson1998; Schultz et al., Reference Schultz, Dayan and Montague1997). Studies in healthy humans where fMRI has been combined with measuring or manipulating the subcortical dopamine indicate that dopamine plays a role in the reward-related BOLD response (Knutson et al., Reference Knutson, Bjork, Fong, Hommer, Mattay and Weinberger2004; Pessiglione et al., Reference Pessiglione, Seymour, Flandin, Dolan and Frith2006; Schott et al., Reference Schott, Minuzzi, Krebs, Elmenhorst, Lang, Winz, Seidenbecher, Coenen, Heinze, Zilles, Duzel and Bauer2008; Urban et al., Reference Urban, Slifstein, Meda, Xu, Ayoub, Medina, Pearlson, Krystal and Abi-Dargham2012). Increased subcortical dopaminergic activity is a consistent finding in schizophrenia, and the salience hypothesis suggests that the altered activity of the reward system leads to aberrant assignment of salience, causing delusions in schizophrenia (Miller, Reference Miller1984; Heinz, Reference Heinz2002; Kapur, Reference Kapur2003). In line with this, an association between the attenuated BOLD response in VS and positive symptoms in antipsychotic-naïve first-episode patients with schizophrenia has been reported (Esslinger et al., Reference Esslinger, Englisch, Inta, Rausch, Schirmbeck, Mier, Kirsch, Meyer-Lindenberg and Zink2012; Nielsen et al., Reference Nielsen, Rostrup, Wulff, Bak, Lublin, Kapur and Glenthøj2012b).
The attenuated striatal BOLD response observed in schizophrenia patients during reward anticipation has been explained by an increased dopaminergic tone leading to a decreased signal-to-noise ratio and an attenuated event-related BOLD response (Heinz and Schlagenhauf, Reference Heinz and Schlagenhauf2010). Treatment with a dopamine antagonist will reduce the aberrant assignment of salience and dampen the psychotic symptoms by decreasing dopaminergic activity. Theoretically, this will increase the signal-to-noise ratio and normalise the BOLD response. Previous fMRI findings support this (Nielsen et al., Reference Nielsen, Rostrup, Wulff, Bak, Broberg, Lublin, Kapur and Glenthoj2012a), but the direct link between blocking dopaminergic transmission and normalising the BOLD response in patients is still missing and can only be found by combining different imaging methods.
There are, however, patients with schizophrenia who do not benefit from blockade of dopamine D2 receptors and it has been hypothesised that these patients belong to a subgroup characterised by a normal or less altered subcortical dopaminergic activity (Howes and Kapur, Reference Howes and Kapur2014; Howes et al., Reference Howes, Mccutcheon and Stone2016; McCutcheon et al., Reference McCutcheon, Beck, Jauhar and Howes2018). In line with this, we have previously demonstrated that antipsychotic-naïve patients responding to dopamine D2 blockade were characterised by an initial, lower binding potential (BPp) in the caudate nucleus compared to non-responding patients (Wulff et al., Reference Wulff, Pinborg, Svarer, Jensen, Nielsen, Allerup, Bak, Rasmussen, Frandsen, Rostrup and Glenthøj2015). Additionally, a recent meta-analysis found that dopaminergic alterations in schizophrenia are most pronounced in the caudate nucleus compared to limbic subdivisions of the striatum (McCutcheon et al., Reference McCutcheon, Beck, Jauhar and Howes2018), and several studies have found alterations in the reward response in caudate regions in schizophrenia patients (Morris et al., Reference Morris, Quail, Griffiths, Green and Balleine2015; Mucci et al., Reference Mucci, Dima, Soricelli, Volpe, Bucci, Frangou, Prinster, Salvatore, Galderisi and Maj2015; Dowd et al., Reference Dowd, Frank, Collins, Gold and Barch2016; Nielsen et al., Reference Nielsen, Rostrup, Broberg, Wulff and Glenthøj2018).
In the current study we combined fMRI and single-photon emission computed tomography (SPECT) data to investigate how blockade of D2 receptors in the caudate nucleus affects salience abnormalities and improves positive symptoms. We hypothesised that:
(1) Blockade of caudate dopamine D2 receptors will normalise the BOLD response during anticipation of salient events and thereby improve positive symptoms.
(2) Accordingly, there will be a positive correlation between caudate D2 receptor occupancy and the change in caudate BOLD response, and between the change in caudate BOLD response and the improvement in positive symptoms.
(3) The relationship between caudate D2 receptor occupancy and the change in caudate BOLD response hypothesised above is expected to be most pronounced in patients responding clinically to dopamine D2 blockade by improvement in positive symptoms.
Methods
Conducted in accordance with the Declaration of Helsinki II, this study was approved by the Danish National Committee on Biomedical Research Ethics (H-D-2008-088). Written informed consent was obtained from all participants.
Participants
Patients were recruited from in- and out-patient mental health centres in the Capital Region of Denmark as part of the Pan European Collaboration on Antipsychotic-Naïve Schizophrenia (PECANS) project; see online Supplementary material.
Thirty-two antipsychotic-naïve patients, age 18–45 years who met the International Classification of Diseases, 10th revision (ICD-10) criteria for schizophrenia, were included for the SPECT part of the project (Fig. 1). A structured diagnostic interview [Schedule of Clinical Assessment in Neuropsychiatry (SCAN), version 2.1] was performed to verify the diagnosis. None of the patients had ever been treated with antipsychotic medications or methylphenidate at inclusion. Patients receiving antidepressants during the preceding month were excluded. Other exclusion criteria were: severe head traumas, neurological diseases, developmental disorders, pregnancy and current drug dependency (according to ICD-10), although occasional use of alcohol and drugs was allowed.
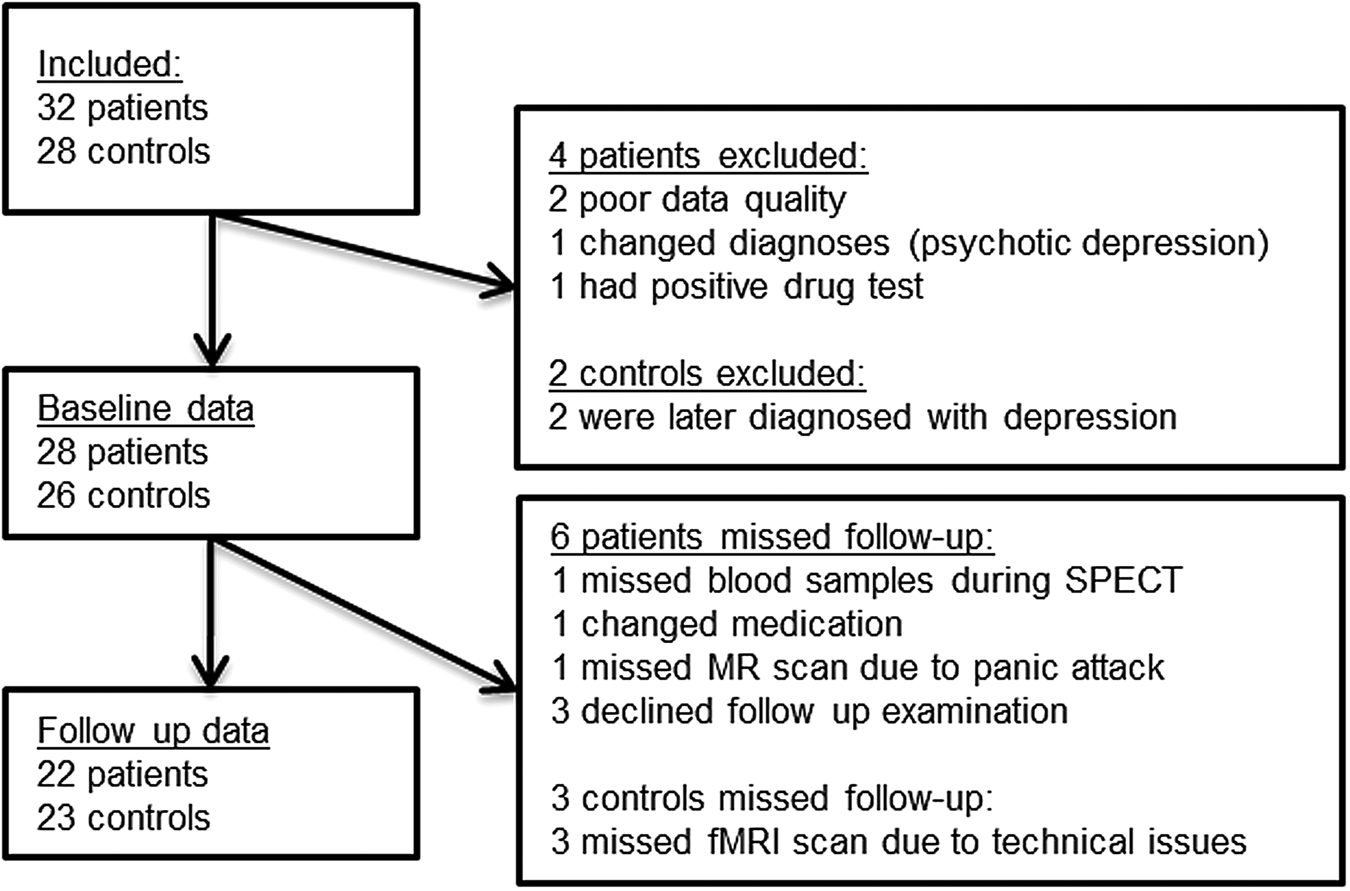
Fig. 1. Flowchart of the study. Number of participants included and reasons for not completing SPECT and fMRI for the present analyses.
Twenty-eight healthy controls (HCs) matched by sex, age and parental socioeconomic status were recruited through advertisement. Exclusion criteria were the same as for patients but also included former or current psychiatric illnesses, drug abuse and psychiatric diagnosis among first-degree relatives (Fig. 1).
All participants were asked about present substance use, and urine samples were obtained for drug screening (Rapid Response; Jepsen HealthCare).
There is a partial overlap between the participants in the current study and participants in previous publications from the PECANS study on reward processing in the VS (eight patients and four HCs) (Nielsen et al., Reference Nielsen, Rostrup, Wulff, Bak, Broberg, Lublin, Kapur and Glenthoj2012a, Reference Nielsen, Rostrup, Wulff, Bak, Lublin, Kapur and Glenthøj2012b). The participants in the current study were also included in two recent publications on reward activity and weight changes, as well as negative symptoms (Nielsen et al., Reference Nielsen, Rostrup, Wulff, Glenthøj and Ebdrup2016, Reference Nielsen, Rostrup, Broberg, Wulff and Glenthøj2018), and a publication on baseline dopamine D2 receptor BPp in the caudate nucleus and treatment outcome (Wulff et al., Reference Wulff, Pinborg, Svarer, Jensen, Nielsen, Allerup, Bak, Rasmussen, Frandsen, Rostrup and Glenthøj2015). None of the previous papers combined fMRI and SPECT data.
Study design and psychopathology
At baseline all participants underwent MRI, fMRI and SPECT scans. In the patient group, psychopathology was assessed with the Positive and Negative Syndrome Scale (PANSS) (Kay et al., Reference Kay, Fiszbein and Opler1987) within the same week as the fMRI and SPECT scans. Follow-up examinations were performed after 6 weeks of treatment. The HC did not receive medication and only one SPECT scan was performed to minimise the radiation dose. With regard to treatment response, we wanted to focus on the short term effect of dopamine blockade on positive symptoms, and in accordance with previous studies with this specific focus, we defined responders as having a reduction in positive symptoms above 30% (Meisenzahl et al., Reference Meisenzahl, Schmitt, Gründer, Dresel, Frodl, la Fougère, Scheuerecker, Schwarz, Boerner, Stauss, Hahn and Möller2008; Wulff et al., Reference Wulff, Pinborg, Svarer, Jensen, Nielsen, Allerup, Bak, Rasmussen, Frandsen, Rostrup and Glenthøj2015). Thus, the change in PANSS scores was calculated as a percentage change between scores at follow-up and baseline.
Medication
Amisulpride was chosen due to its relatively selective binding to and high affinity for dopamine D2 and D3 receptors (Schoemaker et al., Reference Schoemaker, Claustre, Fage, Rouquier, Chergui, Curet, Oblin, Gonon, Carter, Benavides and Scatton1997). Treatment was initiated after the baseline examinations and the dosage was slowly increased and individually adjusted. Medical treatment against side effects was not permitted. To ensure amisulpride steady-state conditions in the brain and blood at follow-up examinations, the dose of amisulpride was not allowed to be adjusted in the last week prior to the examinations.
SPECT acquisition
The ligand (S)-N-[(1-ethyl-2-pyrrolidinyl)]-methyl-2-hydroxy-3-iodo-6-methoxybenzamide ([123I]-IBZM) was chosen due to its selectivity for striatal D2 receptors (Kung et al., Reference Kung, Alavi, Chang, Kung, Keyes, Velchik, Billings, Pan and Noto1990; Seibyl et al., Reference Seibyl, Woods, Zoghbi, Baldwin, Dey, Goddard, Zea-ponce, Zubal, Germine, Smith, Heninger, Charney, Kung, Alavi, Hoffer and Innis1992). The participants received 185 MBq of [123I]-IBZM (GE Healthcare), with half of the dose given as a bolus injection and the other half given as a constant infusion during the entire 240-min session (Seibyl et al., Reference Seibyl, Zea-ponce, Brenner, Baldwin, Krystal, Offord, Mochoviak, Charney, Hoffer and Innis1996). In addition, all patients received their individual dose of amisulpride along with the [123I]-IBZM bolus injection three hours prior to the scan to reduce the effect of individual differences in timing of amisulpride administration on the day of the SPECT scans. Serum amisulpride (s-amisulpride) was measured prior to the dose of amisulpride and at 60, 120, 150, 180, 210, 240 min after the administered dose. The mean value of s-amisulpride during the 1-h scanning period (2 × 30 min) was used in the analyses. Further details concerning the SPECT procedure can be found in the online Supplementary material.
Image analyses
SPECT images were reconstructed with scatter correction and attenuation correction using Flash 3D iterative reconstruction (four subsets, eight iterations, Gaussian filter 9 mm) on a Siemens Syngo workstation (software version VA 60 B).
The two obtained IBZM tomographies (2 × 30 min) were summed and activity measurements were decay-corrected to the time of the radioligand injection. The computed tomography (CT) image from the SPECT scan and the MRI image were co-registered using Statistical Parametric Mapping (SPM) software (SPM v. 8, https://www.fil.ion.ucl.ac.uk/spm). The result of the SPM co-registration was then inspected in all three planes and, if needed, adjusted manually using a local implementation of an image overlay method.
The information from the co-registration between CT and MRI images was used for co-registration between SPECT and MRI. Inspection and manual adjustments were repeated, if needed (Willendrup et al., Reference Willendrup, Pinborg, Hasselbalch, Adams, Stahr, Knudsen and Svarer2004).
The BPp was defined as the steady-state ratio of specifically bound radioligand to that of total parent radioligand in plasma and used as a measure of the regional dopamine D2 receptor density available for [123I]-IBZM binding (Innis et al., Reference Innis, Cunningham, Delforge, Fujita, Gjedde, Gunn, Holden, Houle, Huang, Ichise, Iida, Ito, Kimura, Koeppe, Knudsen, Knuuti, Lammertsma, Laruelle, Logan, Maguire, Mintun, Morris, Parsey, Price, Slifstein, Sossi, Suhara, Votaw, Wong and Carson2007). The cerebellum was chosen as the reference region in the SPECT data analysis (Farde et al., Reference Farde, Wiesel, Stone-Elander, Halldin, Nordstrom, Hall and Sedvall1990). The occupancy was calculated as:

MRI and fMRI acquisition
All participants had a structural MRI scan performed using a Philips Achieva 3.0T whole-body MRI scanner (Philips Healthcare) with an eight-channel SENSE Head Coil. Whole-brain three-dimensional (3D) high-resolution T1-weighted structural images were acquired for anatomical reference (repetition time = 10 ms, echo time = 4.6 ms, flip angle = 8°, and voxel size = 0.79 × 0.79 × 0.80 mm). For the fMRI, 1080 (540/run) whole-brain functional echo-planar images were acquired interleaved (repetition time = 2 s, echo time = 25 ms, flip angle = 75°, 38 slices and voxel size = 2.9 × 2.9 × 2.4 mm, gap 1 mm). Smoking was restricted 1 h before scanning was performed.
The reward paradigm
A variant of Knutson's monetary incentive delay task (Knutson et al., Reference Knutson, Westdorp, Kaiser and Hommer2000, Reference Knutson, Adams, Fong and Hommer2001a, Reference Knutson, Fong, Adams, Varner and Hommer2001b; Cooper and Knutson, Reference Cooper and Knutson2008) was used to elicit caudate activation in response to cues indicating trial condition. There were certain and uncertain monetary gain and loss, as well as neutral cues presented in a pseudorandomised order. Shortly after the cue, a target appears briefly, adjusted by an algorithm to reach a hit rate of 66%. After another short delay, the result for the trial was presented together with the total amount in the bank. A detailed description of the paradigm can be found elsewhere (Nielsen et al., Reference Nielsen, Rostrup, Wulff, Bak, Lublin, Kapur and Glenthøj2012b) and in the online Supplementary material.
fMRI analyses
The fMRI analyses were conducted using tools from the FMRIB Software Library (John Radcliffe Hospital, Oxford, England; http://fsl.fmrib.ox.ac.uk/fsl/fslwiki/). Images were corrected for 3D motion and for slice-time effect (38 sections within each repetition time of 2 s). Spatial smoothing was performed with a five mm full-width at half-maximum Gaussian kernel. Low-frequency noise was reduced using a high-pass filter with a cut-off at 200 s. The images were spatially aligned in Talairach space and normalised to a Montreal Neurological Institute (MNI) standard template using non-linear warping.
A general linear model consisting of 15 predictors was constructed for statistical analysis. Each of the six different cues during the anticipation phase was modelled as separate predictors. Target onset was modelled with two predictors, one for uncertain events, and one for all other events. The outcome phase was modelled with seven predictors, one for each of the possible outcomes. Additionally, six motion parameters were included, and relative and absolute motion were extracted and compared across groups. All explanatory variables were convolved with the hemodynamic response function.
Results from previous analyses of the reward paradigm (Nielsen et al., Reference Nielsen, Rostrup, Wulff, Bak, Lublin, Kapur and Glenthøj2012b) showed that the contrast with the most pronounced alterations in patients was the overall effect of salience during anticipation. This BOLD contrast was modelled by uncertain (salient) gain and loss cues v. neutral cues and was therefore chosen for the analyses.
For each individual, the average contrast signal of the salience contrast was extracted from the region of interest (ROI) for further analyses.
Additional second-level whole-brain analyses of group differences at baseline, follow-up and interaction was performed, please see the online Supplemental Material.
Regions of interest
Recently, we found that the initial treatment response to dopamine D2 receptor blockade was linked to the baseline D2 receptor BPp in the caudate nucleus (Wulff et al., Reference Wulff, Pinborg, Svarer, Jensen, Nielsen, Allerup, Bak, Rasmussen, Frandsen, Rostrup and Glenthøj2015). In the current study the aim was to explore the direct relationship between dopamine D2 receptor blockade and the BOLD response during salience anticipation in the same region. Literature points to the caudate nucleus, and not VS, as the site of altered dopaminergic transmission in schizophrenia and UHR patients (Howes et al., Reference Howes, Montgomery, Asselin, Murray, Grasby and McGuire2007, Reference Howes, Montgomery, Asselin, Murray, Valli, Tabraham, Bramon-Bosch, Valmaggia, Johns, Broome, McGuire and Grasby2009; Kegeles et al., Reference Kegeles, Abi-Dargham, Gordon Frankle, Gil, Cooper, Slifstein, Hwang, Huang, Haber and Laruelle2010; McCutcheon et al., Reference McCutcheon, Beck, Jauhar and Howes2018), hereby stressing the need for linking reward processing to dopamine activity in this subdivision of the striatum.
ROI was defined anatomically from a volume-of-interest brain template (Svarer et al., Reference Svarer, Madsen, Hasselbalch, Pinborg, Haugbøl, Frøkjær, Holm, Paulson and Knudsen2005). Only one ROI was chosen to focus the study and limit the multiple testing. For the SPECT images, we used an automatic application of regions dividing the striatum into its anatomic subdivisions and used the caudate as our ROI. The same ROI was defined in MNI space, and the mean salience contrast signal in percent signal change was extracted from this region using FSL featquery (Fig. 2).
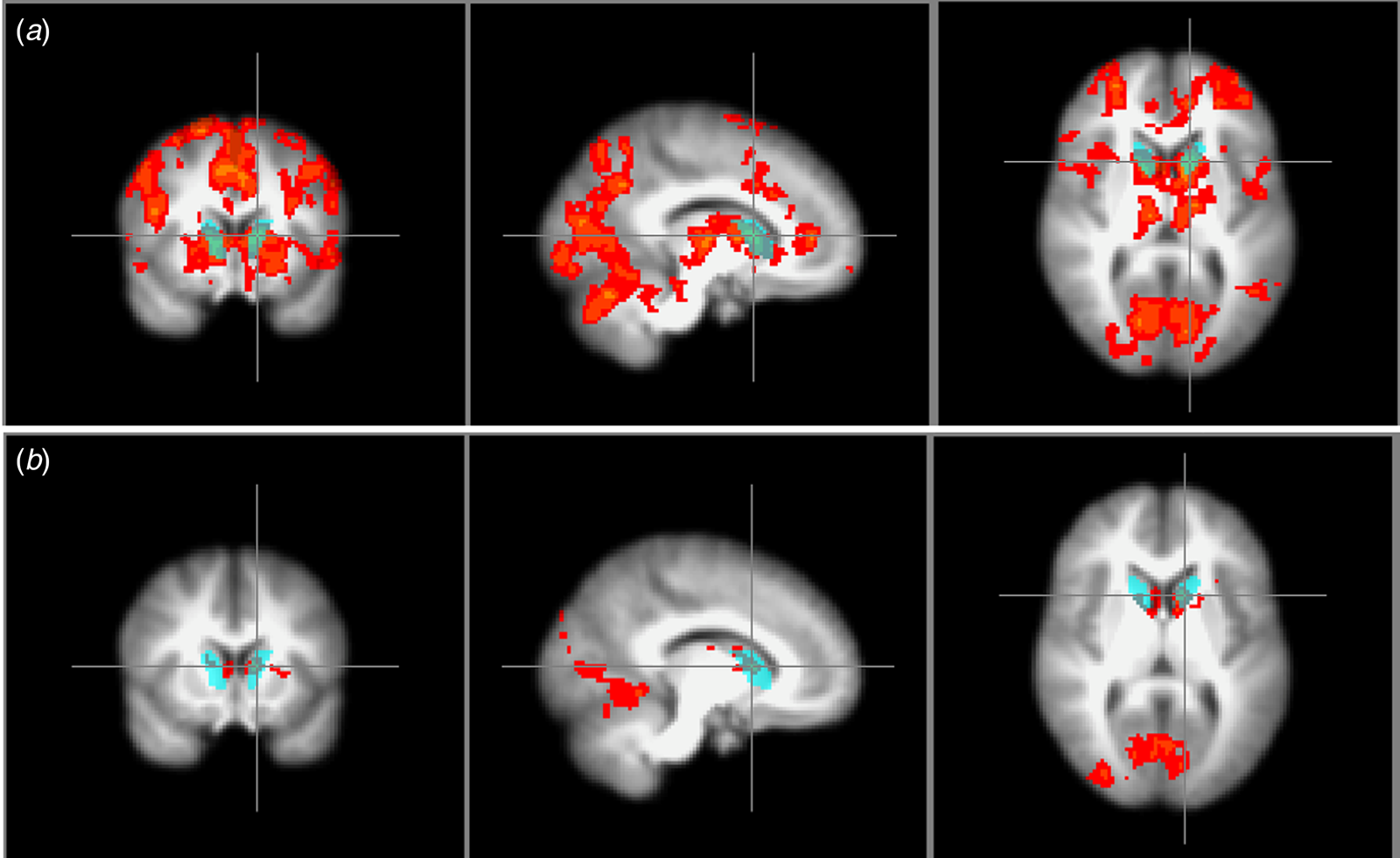
Fig. 2. Region of interest. The blue region marks the anatomically defined ROI from which the SPECT binding potential BPp and occupancy and the mean attenuated BOLD salience contrast signal were extracted. (a) also shows the whole-brain salience contrast signal of the healthy controls, whereas (b) shows the group difference of the whole-brain salience contrast signal.
Statistical analyses
IBM SPSS Statistics 20 was used for the statistical analyses. Group comparisons of age, handedness and baseline BPp were performed using Students t test, and χ2 was used for group comparison of gender distribution and use of substances. The effect of time on the PANSS score was analysed using paired t test. BPp and BOLD activity were normally distributed according to the Shapiro–Wilks test; thus, BOLD activity was analysed using repeated measures analysis of variance (ANOVA) with group as between-subject factor and time as within-subject factor. For comparisons between patients and HCs, analyses were carried out with and without smoking as covariates (smoking or non-smoking), and for comparisons between responders and non-responders, analyses were carried out with and without medication dose and s-amisulpride as covariates. Post-hoc analyses were carried out with two-sample t test and paired t test.
For correlations, Pearson's correlation was used for continuous and normally distributed data, and Spearman's correlation coefficient was used for ordinal data.
Results
Complete SPECT and fMRI data were available for 22 patients (10 males, mean age 23.3) and 23 HCs (12 males, mean age 23.5). Figure 1 shows the reasons for missing data.
There were no group differences in age, handedness or gender distribution (p > 0.6), nor was there any difference in baseline BPp (total caudate p = 0.63). Regarding use of substances, there was a higher rate of smokers among patients (χ2 = 10.9, p = 0.001), but no difference regarding alcohol or cannabis (Table 1).
Table 1. BPp and psychopathology in the HCs and the patients at baseline and follow-up
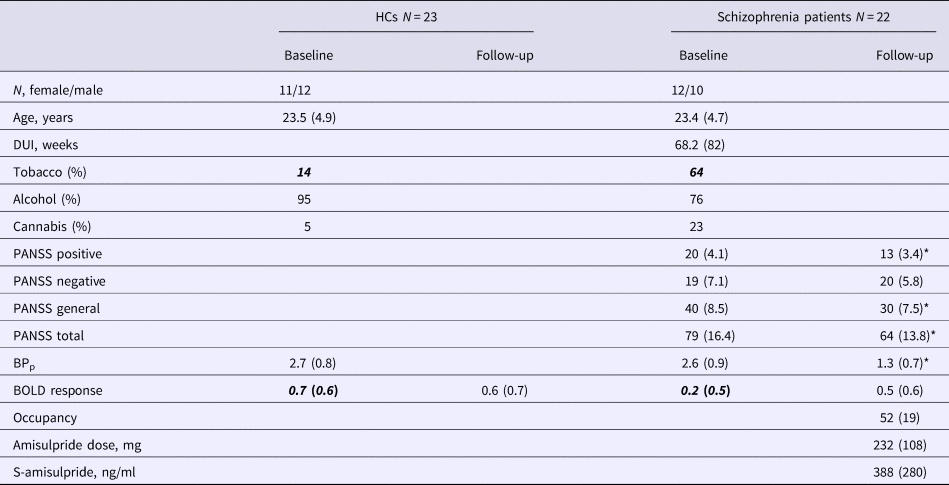
PANSS, Positive and negative syndrome scale; BPp, binding potential; BOLD, blood oxygen level dependent.
Data are specified as mean with standard deviation in parentheses. PANSS at follow-up was only available for 21 patients.
Italic-bold indicates significant difference between patients and HC, p < 0.05.
* Significantly different between baseline and follow-up, p < 0.05.
Treatment effect
After 6 weeks of treatment, patients improved on PANSS total, positive and general symptoms (all p values < 0.001), but not on negative symptoms (p = 0.186). The mean dose of amisulpride was 232 mg/day (standard deviation = 108, range 50–400) (Table 1), and this was highly correlated with s-amisulpride (r = 0.7, p < 0.001). There were no correlations between the change in any of the PANSS-scores and the medication dose, s-amisulpride or occupancy.
BOLD response
For the mean salience contrast activity extracted from the ROI, repeated measures ANOVA showed a significant effect of group (F 1,43 = 4.2, p = 0.046), but no effect of time (p = 0.34) and no group × time interaction (p = 0.16). There was no significant effect of smoking as a dichotomous covariate (p = 0.16); however, the group difference was no longer significant when smoking was included as a covariate (p = 0.23). Post-hoc analyses showed that patients had a significantly lower BOLD response at baseline (t (43) = 2.8, p = 0.008), which was not present at follow-up (p = 0.4). Paired t test showed no effect of time in HCs (p = 0.76), but a trend-level significant increase in BOLD response in patients (t (21) = 2, p = 0.06) (Table 2). For whole-brain voxel-wise group comparison, please see online Supplementary material. There was no effect of group or time and no group × time interaction for relative or absolute motion during the scanning (p > 0.1).
Table 2. Data on responders and non-responders before and after 6 weeks of treatment with amisulpride
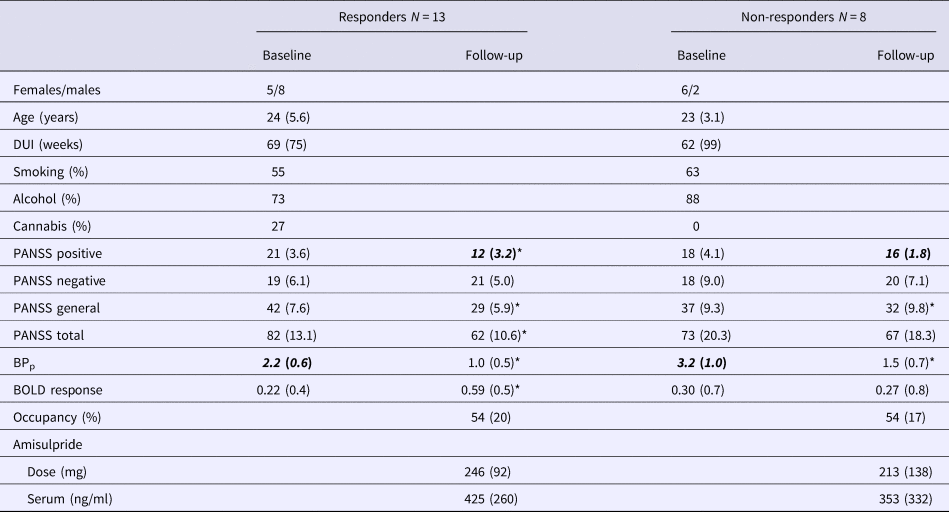
DUI, duration of untreated illness; (S)-amisulpride, serum amisulpride; PANSS, positive and negative syndrome scale; BPp, binding potential; BOLD, blood oxygen level dependent.
Smoking is the percentage reporting daily use, alcohol is the percentage reporting weekly use, cannabis is the percentage reported to have used cannabis more than a few times.
Italic-bold indicates significant difference between responders and non-responders.
* Indicates significant difference between baseline and follow-up, p < 0.05.
Correlations with BOLD response
There was a significant correlation between change in BOLD signal and improvement in PANSS positive score (Spearman's correlation; ρ = −0.435, p = 0.049) (Fig. 3b). There was no correlation between change in BOLD signal and the occupancy, the amisulpride dose or s-amisulpride, and there was no correlation between baseline BPp and baseline BOLD response or change in BOLD response.
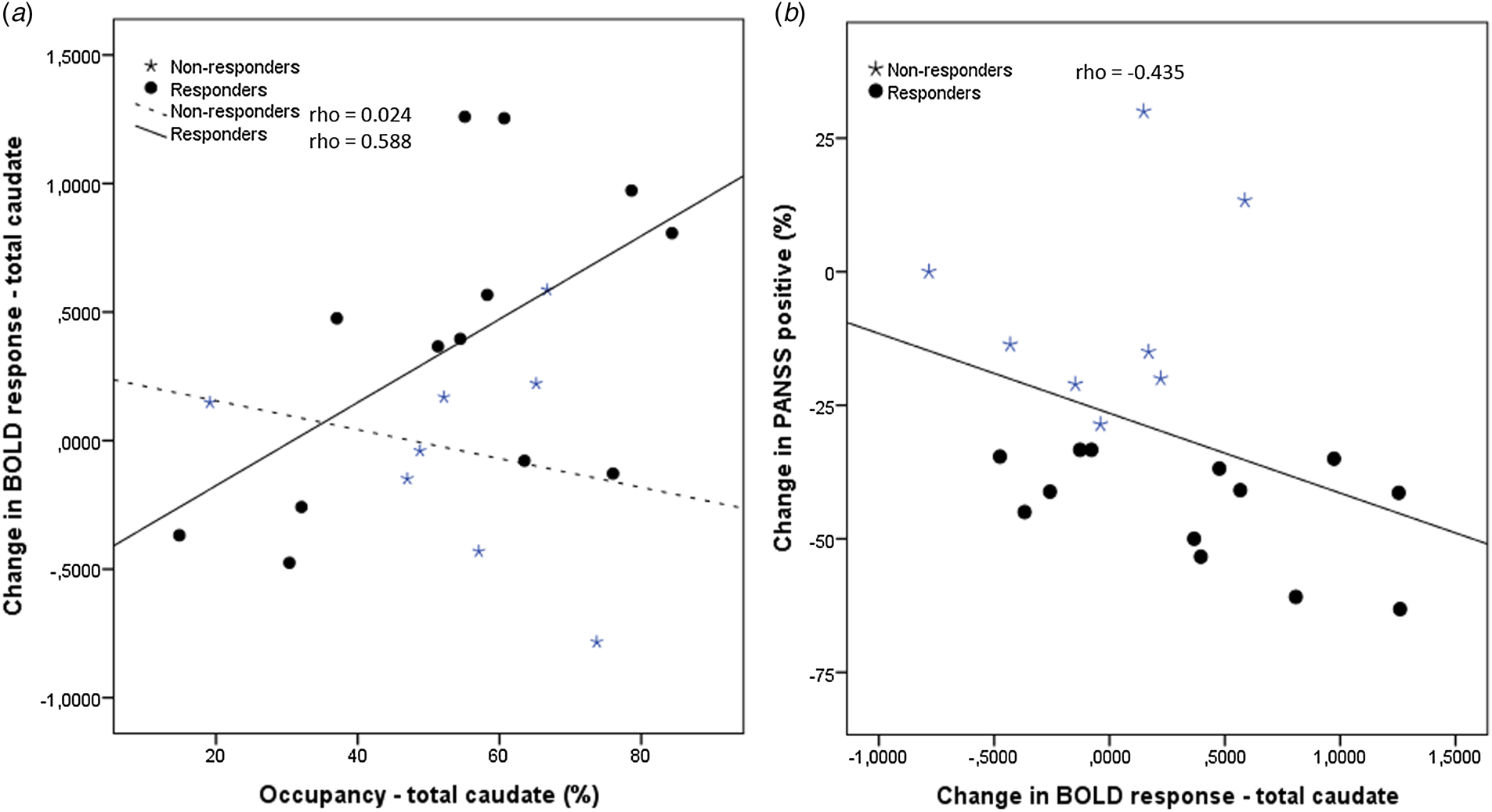
Fig. 3. Correlations. (a) The correlations between the occupancy and change in BOLD response from baseline to follow-up were significant in the group of responders (circles) and non-significant in the group of non-responders (stars). (b) The correlation between the change in BOLD response and change in the PANSS positive score was significant in the whole group of patients. For illustrative purposes the treatment respond status is shown as circles for responders and stars for non-responders.
Responders v. non-responders
Dividing patients according to treatment response (change in positive symptoms ⩾30%) resulted in 13 patients categorised as responders and eight patients as non-responders. One patient was excluded due to missing follow-up PANSS score.
Repeated measure ANOVA showed a significant effect of group for BPp (F 1,19 = 6.1, p = 0.023) and a significant effect of time for BPp (F 1,19 = 97.0, p < 0.001), PANSS positive (F 1,19 = 82.6, p < 0.001), PANSS total (F 1,19 = 35.3, p < 0.001) and PANSS general score (F 1,19 = 49.5, p < 0.001). A significant group × time interaction was found for PANSS positive (F 1,19 = 36.0, p < 0.001), PANSS total (F 1,19 = 10.5, p = 0.004) and PANSS general score (F 1,19 = 8.5, p = 0.009). Adding medication dose and s-amisulpride did not change the significance of these results. Post-hoc analyses showed baseline group difference in BPp, which was significantly lower in the responders (t = −2.4, p = 0.037). At follow-up, only the PANSS positive score was different between the two groups (t = 3.71, p = 0.001). Both subgroups had a significant change in BPp (t 7 = 6.2, p < 0.001 and t 12 = 7.5, p < 0.001) and PANSS general score (t 7 = 2.4; p = 0.049 and t 12 = 8.6, p < 0.001) over time, whereas only responders had a significant change over time in PANSS total score (t 12 = 8.2, p < 0.001), PANSS positive score (t 12 = 13.9, p < 0.001) and BOLD response (t 12 = 2.22, p = 0.046); see Table 2 for details.
There was a positive correlation between improvement in the BOLD response and occupancy in the responders (Spearman's correlation, ρ = 0.588; p = 0.035), whereas no correlation was found in the non-responders (p = 0.955) (Fig. 3a).
Discussion
To our knowledge, this is the first study to demonstrate an association between D2 receptor blockade, normalisation of the BOLD signal, and treatment response in patients with schizophrenia. As expected, we found a decreased BOLD signal in the caudate nucleus during salience anticipation, and there was a correlation between the treatment effect on positive symptoms and the improvement in BOLD signal. In patients characterised as responders based on their improvement on positive symptoms, there was a significant improvement in the BOLD signal, which was correlated with the occupancy of the D2 receptors.
The findings regarding alterations and improvement of the BOLD response and the relationship to positive symptoms are in agreement with our previous results in the VS (Nielsen et al., Reference Nielsen, Rostrup, Wulff, Bak, Broberg, Lublin, Kapur and Glenthoj2012a). Importantly, we used another ROI in the current study, both because of the comparison with our SPECT data (Wulff et al., Reference Wulff, Pinborg, Svarer, Jensen, Nielsen, Allerup, Bak, Rasmussen, Frandsen, Rostrup and Glenthøj2015) and because we wanted to examine salience abnormalities and their association with dopamine activity in the region where increased dopamine activity most consistently has been found, i.e. in the associative striatum/the caudate nucleus (McCutcheon et al., Reference McCutcheon, Beck, Jauhar and Howes2018). Taken together, our present and previous data (Nielsen et al., Reference Nielsen, Rostrup, Wulff, Bak, Lublin, Kapur and Glenthøj2012a, Reference Nielsen, Rostrup, Wulff, Bak, Lublin, Kapur and Glenthøj2012b, Reference Nielsen, Rostrup, Wulff, Glenthøj and Ebdrup2016) support abnormalities in salience processing throughout striatum and a relationship between symptom improvement and normalisation of the BOLD response in the caudate nucleus, as well as in the VS following treatment with a dopamine D2 blocker (Nielsen et al., Reference Nielsen, Rostrup, Wulff, Bak, Broberg, Lublin, Kapur and Glenthoj2012a). The finding of a direct association between blockade of D2 receptors in the caudate nucleus, normalisation of reward processing and treatment outcome underline the importance of striatal dopamine activity for the aberrant salience in some patients with schizophrenia.
Interestingly, we only found a relationship between occupancy of dopamine D2 receptors and change in the BOLD response in the patients who improved the most in positive symptoms. A heterogeneous treatment response in schizophrenia is well known (Levine and Leucht, Reference Levine and Leucht2010), and previous findings (Howes et al., Reference Howes, Montgomery, Asselin, Murray, Valli, Tabraham, Bramon-Bosch, Valmaggia, Johns, Broome, McGuire and Grasby2009; Thomsen et al., Reference Thomsen, Knudsen, Jensen, Ziebell, Holst, Asenbaum, Booij, Darcourt, Dickson, Kapucu, Nobili, Sabri, Sera, Tatsch, Tossici-Bolt, van Laere, Borght, Varrone, Pagani and Pinborg2013; Bloomfield et al., Reference Bloomfield, Pepper, Egerton, Demjaha, Tomasi, Mouchlianitis, Maximen, Veronese, Turkheimer, Selvaraj and Howes2014b; Wulff et al., Reference Wulff, Pinborg, Svarer, Jensen, Nielsen, Allerup, Bak, Rasmussen, Frandsen, Rostrup and Glenthøj2015) support the existence of possible subgroups of patients, with more or less hyperdopaminergic activity in the caudate nucleus reflected in good and poor treatment response. The present finding of an association between occupancy and change in BOLD response only in the patients responding most to dopamine D2 blockade may very well reflect this heterogeneity. The dopamine receptors were affected similarly in both groups, as indicated by a significant decrease in BPp and a comparable level of receptor occupancy in the caudate nucleus. This resulted in a highly significant increase in BOLD contrast signal in the group of responding patients that was not observed in the non-responding patients.
Although we expect dopamine to play a key role in reward anticipation in schizophrenia, the influence of other neurotransmitter systems, such as the serotonergic, GABAergic and/or glutamatergic systems, may also be involved in the observed disturbances of reward processing (Cohen et al., Reference Cohen, Haesler, Vong, Lowell and Uchida2012). It seems likely that patients responding less to D2 blockade may have a less pronounced hyperdopaminergic activity, since they either show a small worsening or no change in the BOLD response despite similar receptor occupancy. It can therefore be hypothesised that the reward disturbances found in these patients may be a result of changes in other transmitter systems. Recent findings have linked glutamate levels to reward disturbances (White et al., Reference White, Kraguljac, Reid and Lahti2015), and likewise, attenuated BOLD response during reward anticipation in alcohol dependence and major depressive disorders indirectly supports the involvement of more than one neurotransmitter system in reward processing (Hägele et al., Reference Hägele, Schlagenhauf, Rapp, Sterzer, Beck, Bermpohl, Stoy, Ströhle, Wittchen, Dolan and Heinz2015).
There may be other explanations for the lack of symptom improvement in the patients characterised as non-responders, however. Titration of the amisulpride dose was conducted by the treating physician only, who balanced between achieving an improvement in symptoms and avoiding side effects. This resulted in a wide range of occupancy between patients described as a curvilinear function in our previous study (Wulff et al., Reference Wulff, Pinborg, Svarer, Jensen, Nielsen, Allerup, Bak, Rasmussen, Frandsen, Rostrup and Glenthøj2015). It cannot be ruled out that some of the non-responding patients might have responded to a higher dose or would have improved further with additional time. Nevertheless, in line with the present data, a recent study supports different neurochemical profiles in responding and non-responding patients. They found that patients responding to first-line treatment had higher dopamine synthesis capacity compared to treatment-resistant patients who later received clozapine (Kim et al., Reference Kim, Howes, Veronese, Beck, Seo, Park, Lee, Lee and Kwon2017).
Limitations and strengths
One of the particular strengths of this study is the inclusion of initially antipsychotic-naïve first-episode schizophrenia patients, which means that the brains of the patients had not been modified by antipsychotics at baseline examinations. Additional strengths are the longitudinal study design, the monotherapy with a relatively selective D2 receptor antagonist, and the combination of several modalities. The examination programme was extensive (the patients had to stay un-medicated for 5 to 7 days), which could lead to selection bias, and exclusion of the more severely ill patients.
Patients and HCs in our study were not matched for smoking, since matching for smoking might add a bias to the HC group because the number of smokers was significantly higher in patients. We included smoking as a covariate, although it was only measured at a dichotom level (smoking or non-smoking). Smoking was not significant as a covariate; however, adding it to the analysis, unsurprisingly, eliminated the significant effect of group due to the skewed distribution across groups. Nicotine use may affect the BOLD response during reward anticipation (Peters et al., Reference Peters, Bromberg, Schneider, Brassen, Menz, Banaschewski, Conrod, Flor, Gallinat, Garavan, Heinz, Itterman, Lathrop, Martinot, Paus, Poline, Robbins, Rietschel, Smolka, Ströhle, Struve, Loth, Schumann and Büchel2011; Jasinska et al., Reference Jasinska, Zorick, Brody and Stein2014), although the effect of smoking can be debated (Bloomfield et al., Reference Bloomfield, Pepper, Egerton, Demjaha, Tomasi, Mouchlianitis, Maximen, Veronese, Turkheimer, Selvaraj and Howes2014b), and we sought to minimise any unwanted effect of smoking by restricting it before scanning. Recent data have also pointed to reduced BOLD response in alcohol dependence (Hägele et al., Reference Hägele, Schlagenhauf, Rapp, Sterzer, Beck, Bermpohl, Stoy, Ströhle, Wittchen, Dolan and Heinz2015); and drug abuse, including cannabis, is known to affect striatal dopamine transmission and reward activity (Gardner, Reference Gardner2005; Glenthoj et al., Reference Glenthoj, Mackeprang, Svarer, Rasmussen, Pinborg, Friberg, Baaré, Hemmingsen and Videbaek2006; Nestor et al., Reference Nestor, Hester and Garavan2011; Loewinger et al., Reference Loewinger, Oleson and Cheer2013; Bloomfield et al., Reference Bloomfield, Morgan, Kapur, Curran and Howes2014a). None of the participants in the current study abused or were dependent on alcohol, cannabis or other substances.
The sample size is small-moderate, the power is limited and some of the results were not highly significant. For group comparisons, there were only a few significant interactions. Therefore, the significant post-hoc results should be taken with precaution and will need replication. It is a limitation of the study that dynamic cerebral binding data following bolus plus constant infusion of radioligand was not available, however, constant levels of radioligand in the plasma strongly suggest that radioligand steady-state was attained both in blood and brain during the experiment.
Conclusion
The current study strongly supports that alterations of the reward system play a role in schizophrenia and that blockade of dopamine D2 receptors may ameliorate psychotic symptoms by normalising salience abnormalities in patients responding to first-line treatment. Other patients have similar abnormalities in the reward system but do not respond to D2 blockade. The extent to which their reward abnormalities are secondary to other disturbances is yet to be resolved.
Supplementary material
The supplementary material for this article can be found at https://doi.org/10.1017/S0033291718004099.
Author ORCIDs
Mette Ødegaard Nielsen 0000-0002-0780-7099
Acknowledgements
The authors thank participants in the study and the staff at the mental health centres that referred patients to us. We are also grateful to our research nurse, Gitte Saltoft Andersen, who helped with the practical coordination of the study and examining the patients. Additionally, we wish to acknowledge the staff at the Diagnostic Department, Functional Imaging Unit and Section of Clinical Physiology, Glostrup Hospital, University of Copenhagen for assistance in acquiring the SPECT and MRI scans and for carrying out the blood analyses. Trial registration: clinicaltrials.gov, identifier: NCT01154829.
Financial support
The study was financially supported by the Lundbeck Foundation (R13-A1349, R25-A2701), the Danish Medical Research Council, the Mental Health Service in the Capital Region of Denmark and the Faculty of Health and Medical Sciences, University of Copenhagen. The funding sources had no role in the design and conduct of the study; collection, management, analysis and interpretation of the data; preparation, review or approval of the manuscript; or the decision to submit the manuscript for publication.
Conflict of interest
None.
Ethical standards
The authors assert that all procedures contributing to this work comply with the ethical standards of the relevant national and institutional committees on human experimentation and with the Helsinki Declaration of 1975, as revised in 2008.