Introduction
Drought is a common environmental stress experienced by plants negatively affecting plant development, growth and productivity (Slama et al., Reference Slama, Messedi, Ghnaya, Savouré and Abdelly2006; Tuberosa and Salvi, Reference Tuberosa and Salvi2006; Verslues et al., Reference Verslues, Agarwal, Katiyar-Agarwal, Zhu and Zhu2006). Improving drought tolerance is probably one of the most difficult tasks for plant breeders. The difficulty comes from the diversity and unpredictability of drought conditions in the field, and from the diversity of drought tolerance strategies developed by the plants that are targeted and subjected to selection criteria (Teulat et al., Reference Teulat, Merah, Souyris and This2001).
Legumes are the main source of vegetable protein in human diets and livestock feed, having major impacts on agriculture, environment and health (Graham and Vance, Reference Graham and Vance2003; Dita et al., Reference Dita, Rispail, Prats, Rubiales and Singh2006). In the world, the limiting factors for high yield of major crop legumes are their low adaptation to the diverse local eco-geographical parameters and the slowness of genetic improvements due to their complex ploidy and large genomes (Cook, Reference Cook1999). Medicago truncatula, commonly known as barrel medic, is a forage legume plant of Mediterranean origin, well adapted to semi-arid conditions, namely to alkaline clay soils (Lesins and Lesins, Reference Lesins, Lesins and Junk1979). This species was identified as a suitable model legume because of its small genome size (500–550 Mbp), simple diploid genetics (2n= 16), self-fertility, short life cycle, rapid germination, excellent mutant populations and large collections of diverse ecotypes (Young and Udvardi, Reference Young and Udvardi2009).
Although responses and physiological mechanisms to drought stress have been extensively studied in different plant species (Yamaguchi-Shinozaki et al., Reference Yamaguchi-Shinozaki, Kasuga, Liu, Nakashima, Sakuma, Abe, Shinwari, Seki and Shinozaki2002; Leung, Reference Leung2008; Badri et al., Reference Badri, Arraouadi, Huguet and Aouani2010, Reference Badri, Chardon, Huguet and Aouani2011), research exploring natural diversity in drought tolerance is still limited in many plant species. Conventional linkage mapping can be an effective tool for identifying genes underlying natural variation. However, the genes identified by this method are restricted to the ones segregating in the cross under consideration. Genome-wide association studies (GWAS) overcome this limitation and have recently been shown to successfully reveal common variants responsible for the variation in phenotypes of Arabidopsis thaliana natural accessions (Atwell et al., Reference Atwell, Huang, Vilhjalmsson, Willems, Horton, Li, Meng, Platt, Tarone, Hu, Jiang, Muliyati, Zhang, Amer, Baxter, Brachi, Chory, Dean, Debieu, de Meaux, Ecker, Faure, Kniskern, Jones, Michael, Nemri, Roux, Salt, Tang, Todesco, Traw, Weigel, Marjoram, Borevitz, Bergelson and Nordborg2010). The GWAS are often complementary to QTL mapping and, when conducted together, they mitigate each other's limitations (Korte and Farlow, Reference Korte and Farlow2013).
Natural plant populations are often collected from a wide range of geographical locations that have a relatively large genetic diversity. Maintaining genetic diversity within natural populations can maximize their potential to withstand and adapt to biotic and abiotic environmental changes (Jump et al., Reference Jump, Marchant and Peñuelas2009). To date, little is known about natural variation in the drought responses of M. truncatula collections. Considering the heterogeneous distribution of ground water and precipitations across Tunisian areas, different natural populations are potentially subjected to different selective pressures. In these conditions, we expect variation in the response to water deficit of different genotypes. This research aims to evaluate the phenotypic diversity of drought tolerance in 11 natural Tunisian populations of M. truncatula. The results can be used for further investigating the molecular and genetic basis of the drought tolerance of M. truncatula, which may contribute to the discovery of drought tolerance mechanisms of other legumes.
Materials and Methods
Plant material and growing conditions
108 lines of M. truncatula, from 11 Tunisian populations, collected in different eco-geographical sites (Enfidha (1), Jelma (2), Amra (3), Deguache (4), Thala (6), El Kef (7), Soliman (TN8), Bulla Regia (TN9), Gabès (TN11), Djerba (TN12), and Tataouine (TN14)) and five reference lines were used (Fig. 1) (Arraouadi et al., Reference Arraouadi, Badri, Jaleel, Djébali, Ilahi, Huguet and Aouani2009; Lazrek et al., Reference Lazrek, Roussel, Ronfort, Cardinet, Chardon, Aouani and Huguet2009). The eco-geographical variables of the collection sites of these populations are summarized in Table S1 (available online).

Fig. 1 Map showing the 11 Tunisian populations from which 108 lines of M. truncatula were obtained.
Seeds were surface-sterilized and scarified by immersion in concentrated H2SO4 for 7 min and rinsed ten times with sterile distilled water. The soaked seeds were sown in Petri dishes on 0.9% agar medium before being vernalized at 4°C for 96 h in darkness. Once the emerging root attained a length of 4 mm, seedlings were transferred to 33 cl pots (8 cm diameter and 10.5 cm deep) filled with sterilized sand that was previously washed using HCl 0.05%. Each plant was grown in a separate pot under greenhouse conditions at the Centre of Biotechnology of Borj Cedria, Tunisia. Treatments and lines were arranged in a randomized complete block design with five blocks and five replicates per experimental unit. Plants were grown in one of the two water treatments, as follows: well-irrigated treatment or mild water-deficit treatment (50% of field capacity (FC)). In order to simulate natural water-deficit conditions similar to field, water stress was applied after a growth period of 10 d. Water levels were maintained for each treatment by weighing pots every 2 d and the water lost was replaced carefully to maintain soil water content near the 100 and 50% of FC in each pot. Plants were irrigated using a nutritive solution as described by Vadez et al. (Reference Vadez, Rodier, Payre and Drevon1996), with the source of iron modified to be Fe-EDTA. For each treatment, each genotype was replicated five times, resulting in a total of 1130 planted seeds. After 2 months, for each individual plant we measured 15 quantitative traits of shoot and root growth (Table 1). For dry weight biomass, plant organs were dried at 70°C for 48 h. The aerial water content (AWC) and root water content (RWC) were estimated as follows:


where AFW and RFW are the aerial and root fresh weights, respectively, while ADW and RDW are the aerial and root dry weights, respectively.
Table 1 List of measured quantitative traits and their abbreviations
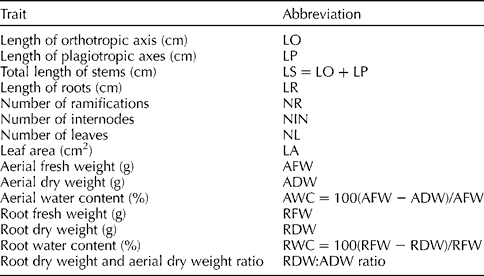
Statistical analyses
Genetic variation of drought response in Medicago truncatula
From each population, a single seed per pod was used to initiate lines of progeny from self-pollination. Although the Tunisian populations of M. truncatula we used are highly selfing in nature (Arraouadi et al., Reference Arraouadi, Badri, Jaleel, Djébali, Ilahi, Huguet and Aouani2009; Lazrek et al., Reference Lazrek, Roussel, Ronfort, Cardinet, Chardon, Aouani and Huguet2009), each line was selfed twice or three times in the greenhouse to lower residual heterozygosity. The offspring in each presumed line was considered genetically identical. Consequently, the within-line variance can be assumed as the effect of environment while the variation among the lines is assumed to be solely genetic. Drought response index (DRI) was calculated per line and for each trait as the mean of the ratios between the observed mean value under water-deficit condition and the mean values under well-watered condition. Analysis of the effects of population, treatment, line within population, and population × treatment and treatment × line within population interactions on measured traits was performed using Proc GLM in SPSS version 16 (2007 Rel 1600 SPSS Inc., Chicago, IL, USA). Phenotypic mean values of each population were compared for all quantitative traits using the Duncan multiple range test at 5%.
The variance components were estimated using the procedure VARCOMP in the SPSS software. The level of population differentiation (Q
ST) for quantitative traits was computed as described by Neji et al. (Reference Neji, Geuna, Taamalli, Ibrahim, Smida, Badri, Abdelly and Gandour2014) for a predominantly selfing species as
$$Q _{ST} = \sigma _{p}^{2}/( \sigma _{p}^{2} + \sigma _{w}^{2}), $$
where
$$\sigma _{p}^{2} $$
is the variance among populations and
$$\sigma _{w}^{2} $$
the variance within populations. Broad-sense heritability (H
2) was calculated for each trait as
$$H ^{2} = \sigma _{g}^{2}/( \sigma _{g}^{2} + \sigma _{e}^{2}), $$
where
$$\sigma _{g}^{2} $$
is the variance between lines and
$$\sigma _{e}^{2} $$
the environmental effect. The coefficient of genetic variation (CV) was estimated as
$$CV = 100( \sigma _{ g }^{2})^{1/2}/m $$
, where m is the population phenotypic mean.
Phenotypic correlations between all trait combinations for each treatment were estimated by computing the Pearson correlation coefficient (r) using the SPSS Correlate procedure.
Clustering analysis
A standardized principal component analysis (PCA) (Data reduction-Factor procedure – SPSS) was performed on the correlation matrix of the synthetic variable based on the mean line values. To represent the relationships between studied populations and lines within populations in water-deficit conditions, cluster analysis was performed to generate phenograms based on the Euclidean distance matrix of dissimilarity, calculated using all PCA scores (Badri et al., Reference Badri, Ilahi, Huguet and Aouani2007), by the Ward method using the XLSTAT software v 7.5 (Addinsoft, USA). A Discriminant Analysis (DA) was performed on DRI values for the groups of M. truncatula lines. Finally, the relationship between the Euclidean distance between populations based on quantitative traits and the geographical distance matrix was analysed using a Mantel test (XLSTAT software v 7.5, Addinsoft, USA). The matrix of geographical distance between populations was calculated by measuring the shortest distance between two points in the map, using geographical coordinates for each site.
Results
Genetic variation of drought response in Medicago truncatula
ANOVA showed that the variation of drought response was explained by the effects of population, line, treatment and interactions between population and treatment, and line and treatment. The maximum effect was observed for treatment factor (Table 2). Thus, M. truncatula lines differ in their response to drought treatment.
Table 2 Contribution of population, line within population, treatment, and population×treatment (P×T) and line×treatment (L×T) interactions to the total variance of measured traits for studied populations of Medicago truncatula

F, coefficient of Snedecor–Fisher.
Significant (P≤ 0.05), non significant (P>0.05).
a Abbreviations of the traits are defined in Table 1.
Most of the measured traits showed a significant difference between studied populations of M. truncatula under control treatment and drought stress (Tables S2 and S3, available online). In the control treatment, the Bulla Regia population exhibited the largest ADW, and the highest RDW was observed for the El Kef population. The Enfidha population had the highest length of stems (LS), while the maximum number of ramifications (NR) was recorded for the Soliman population. Furthermore, the Amra and Deguache populations showed the highest RDW:ADW ratio.
All measured traits, except the AFW and AWC, showed significant reductions, caused by water-deficit treatment, between studied populations of M. truncatula (Table S4, available online). In the drought stress, the lowest decrease in LS was recorded for the reference lines, but these lines experienced the strongest reductions in the number of leaves (NL), AFW, ADW and RDW. The highest reduction in LS was recorded for Deguache (DRI = 43.65%), Djerba (DRI = 43.36%), Amra (DRI = 46.85%) and Tataouine (DRI = 47.21%), while El Kef (DRI = 121.92%), Soliman (DRI = 114.27%) and Gabès (DRI = 108.94%) were most susceptible according to the RDW:ADW ratio. The lowest reductions were found for NR in Deguache (DRI = 79.45%) and Enfidha (DRI = 33.02%), for length of roots (LR) for Deguache (DRI = 112.38%), for NL for Thala (DRI = 71.58%), for AFW for Soliman (DRI = 53.95%), for RDW for Jelma (DRI = 87.03%) and for RDW:ADW ratio for Deguache (DRI = 167.11%). Overall, the population of Deguache allocated highest proportion of its biomass to roots in both control and water-deficit treatments. Table S4 (available online) gives the CV of each trait for studied populations in M. truncatula. We will arbitrarily consider as large those CV above 40%. The largest variations within populations (CV>40%) were noticed for the length of orthotropic axis (LO), LS, RFW, RDW and RDW:ADW ratio.
H 2 of measured traits ranged from 0.00 to 0.74 and from 0.02 to 0.65 under control and drought stress treatments, respectively (Table 3). In the well-irrigated treatment, high heritabilities (H 2>0.4) were recorded for LO, length of plagiotropic axes (LP) and NL, moderate values (0.2 < H 2≤ 0.4) were for LS, NR, leaf area (LA), ADW, RDW and RDW:ADW ratio, and lower levels (H 2≤ 0.2) were registered for LR, number of internodes (NIN), AWC, RFW and RWC. Furthermore, in the drought stress treatment, high heritabilities (H 2) were observed for LO and NL, low levels were for LR, NR, AFW, ADW, AWC, RFW, RDW and RWC, and moderate values were recorded for the remaining traits. Overall, most of the measured traits showed highest heritabilities (H 2) in well-irrigated treatment (Table 3).
Table 3 Variance among populations (σ2 p), genetic variance (σ2 g), environmental variance (σ2 e), heritabilities (H 2) and population differentiation for quantitative traits (Q ST) in measured traits for Medicago truncatula lines under well-watered (control) and water-stressed (drought) conditions

a Abbreviations of the traits are defined in Table 1.
Q ST ranged from 0 to 1 and from 0 to 0.73 under control and water-deficit treatments, respectively (Table 3). In the well-watered condition, the highest Q ST values were recorded for LO, NR, LA, AFW, ADW, AWC and RFW, moderate Q ST were for LS, NIN, NL, RDW and RDW:ADW ratio, and lower Q ST were registered for the remaining traits. In the water-deficit conditions, the highest Q ST were found for LO, LR, NR, NIN, NL, LA, AFW, ADW, AWC, RFW, RWC and RDW:ADW ratio, moderate Q ST values were recorded for LP and LS, and lower Q ST was for RDW. Overall, the highest Q ST values for most traits were recorded in drought stress. Moreover, Q ST results indicated a lack of population structuring, in both treatments, such that most of the overall variation for the majority of investigated traits was held within populations.
Among the 105 possible correlations between measured traits in control treatment, 81 were significant and 66 of them were positive (Table S5, available online). Most traits of aerial and root growths were positive. The RDW:ADW ratio was negatively correlated with the characters of aerial growth and positively with AWC and RDW. In the water-deficit condition, among the 105 correlations between measured characters, 73 were significant and 59 of them were positive. The RDW:ADW ratio was negatively correlated with the characters of aerial growth and positively with AWC, RFW and RDW.
Comparison between two matrices of intertrait correlations in inbred lines of M. truncatula in control and drought stress treatments (Table S5, available online) shows that (1) several correlations are specific in well-irrigated treatment such as positive correlation between LO and RWC, and (2) specific correlations in drought stress were noticed such as a positive correlation between LS and RDW.
Clustering analysis based on the DRI values
Six principal components, whose eigenvalues were higher than 0.80, were found to account for 81.93% of the total phenotypic variation. Using these six components, lines of M. truncatula were clustered based on Euclidean distances of dissimilarity. Four groups of lines differing in their sensitivities to drought stress were generally identified, based on the DRI values (Fig. S1, available online). A first group contained 31 lines with one line per population from Amra and Deguache, one reference line, two lines from Gabès, three lines per population from Enfidha, El Kef, Djerba and Tataouine, four lines from Soliman and five lines per population from Thala and Bulla Regia. A second group included 45 lines with one line of Thala, three reference lines, three lines per population from Jelma, Deguache, Soliman and Bulla Regia, four lines per population from El Kef, Djerba and Tataouine, five lines per population from Amra and Gabès, and seven lines from Enfidha. A third group constituted by 23 lines with one reference line, one line per population from Amra, Bulla Regia, Djerba and Tataouine, two lines from El Kef, three lines per population from Deguache, Thala, Soliman and Gabès, and four lines from Jelma. A fourth group contained 14 lines with one line per population from Thala, El Kef and Bulla Regia, two lines per population from Amra, Deguache, Djerba and Tataouine, and three lines from Jelma.
Five principal components, whose eigenvalues were higher than 0.80, were found to account for 91.92% of the total variation. Using these five components, populations of M. truncatula were clustered based on Euclidean distances of dissimilarity into three groups differing in their water-deficit response (Fig. 2). A first group contained reference lines, Enfidha (TN1), Gabès (TN11) and Tataouine (TN14) populations. A second group comprised Deguache (TN4), Amra (TN3) and Djerba (TN12). A third group included Jelma (TN2), Bulla Regia (TN9), Soliman (TN8), Thala (TN6) and El Kef (TN7).

Fig. 2 Clustering of populations of M. truncatula based on Euclidean distances of dissimilarity into three groups with different water-deficit responses.
Based on the DA, LP, LS, NIN, NL, RFW, RWC and RDW:ADW ratio are the main determinant traits for the classification of studied lines of M. truncatula into the four clusters (Table 4). Groups 1 and 3 were less affected by drought for LP, LS, NIN and NL, group 3 was least affected for RFW and RWC and group 4 had lowest effect for RDW:ADW ratio. Group 2 was, on average, the most affected group by drought.
Table 4 Means of drought response index (DRI) for classes of Medicago truncatula lines

λ, Lambda of Wilks; F, coefficient of Snedecor–Fisher.
Means of each trait followed by different letters are significantly different between the four lines’ groups.
a Abbreviations of the traits are defined in Table 1.
On the other hand, DA showed that the LO (λ = 0.52, F= 4.17, P= 0.05), NIN (λ = 0.4, F= 6.88, P= 0.015) and RWC (λ = 0.25, F= 13.26, P= 0.002) are the main determinant characters for the classification of the studied populations. The first class contained the populations and the reference lines, which are most affected for RWC, the second group included the populations less affected for NIN and the third group had the populations with highest reductions in LO.
Discussion
The significant genotype × treatment interaction observed for most of the traits suggests that lines of M. truncatula may have developed an adaptive response to drought that can be exploited to further determine the genetic basis of this physiological adaptation.
The LP was the most seriously affected trait (DRI = 28.59%) for populations while the RDW:ADW ratio had lowest reductions (DRI = 135.36%). The importance of root systems in acquiring water has long been recognized (Jaleel et al., Reference Jaleel, Manivannan, Wahid, Farooq, Al-Juburi, Somasundaram and Panneerselvam2009). A prolific root system can confer the advantage to support accelerated plant growth during the early crop growth stage and extract water from shallow soil layers that is otherwise easily lost by evaporation in legumes (Johansen et al., Reference Johansen, Baldev, Brouwer, Erskine, Jermyn, Li-Juan, Malik, Ahad Miah, Silim, Muehlbauer and Kaiser1992). An increased root growth due to water stress was reported in sunflower (Tahir et al., Reference Tahir, Imran and Hussain2002), Catharanthus roseus (Jaleel et al., Reference Jaleel, Gopi, Sankar, Gomathinayagam and Panneerselvam2008), and M. truncatula and M. laciniata (Badri et al., Reference Badri, Chardon, Huguet and Aouani2011). The root dry weight was decreased under mild and severe water stress in Populus species (Wullschleger et al., Reference Wullschleger, Yin, DiFazio, Tschaplinski, Gunter, Davis and Tuskan2005), and in maize and wheat (Sacks et al., Reference Sacks, Silk and Burman1997).
Moderate levels of genotypic variance and heritability estimates were found for most traits under control treatment and drought stress. It will be relatively easy to select for these traits and pass them onto offspring. H 2 of traits in this study was dependent on treatment effect with higher values generally recorded in well-irrigated treatment. Gomez-Mestre and Tejedo (Reference Gomez-Mestre and Tejedo2004) showed that the heritability of the trait in the natterjack toad (Bufo calamita) populations increased as the osmotic stress increased, as differences among full-sibs became accentuated. However, the more informative analysis of half-sibs revealed that narrow-sense heritability might be decreasing as salinity increases (Tejedo M et al., unpublished data). This apparent contradiction is most probably caused by the presence of non-additive components (dominance and epistasis) and maternal effects that may be sensitive to the level of stress.
Our results indicate that differences in water availability can result in population differentiation (Q ST) and local adaptation in natural populations of M. truncatula. Drought stress should exert strong selection on plant physiology, morphology and growth, and population differentiation in response to variation in water availability has been documented in several species and at several geographical scales (Nienhuis et al., Reference Nienhuis, Sills, Martin and King1994; Eckhart et al., Reference Eckhart, Geber and McGuire2004; Sambatti and Rice, Reference Sambatti and Rice2007; Wu et al., Reference Wu, Lowry, Nutter and Willis2010). Accordingly, Gomez-Mestre and Tejedo (Reference Gomez-Mestre and Tejedo2004) showed that most estimates of quantitative genetic distances between population pairs (Q ST) increased as salinity increased, indicating that differences in salinity tolerance among populations broadened with increased osmotic stress.
Most of the correlations between measured traits under control and water-deficit treatments were positive; they were dependent on the treatment effect. This finding is in accordance with that reported by Teulat et al. (Reference Teulat, Monneveux, Wery, Borries, Souyris, Charrier and This1997), demonstrating that genotypic correlations between growth characters were dependent on the water treatment in recombinant inbred lines of barley (Hordeum vulgare L.). In the current study, traits of M. truncatula are positively correlated, probably because they are developmentally or structurally related. Furthermore, reduced genetic correlations between investigated characters can speed adaptation to novel habitats (e.g. Badyaev et al., Reference Badyaev, Foresman and Young2005).
Using the five components of PCA that explain 91.92% of the total variation, populations of M. truncatula were clustered into three groups differing in their drought response. Some of the populations selected for this study varied with geographic information such as altitude, electro-conductivity, annual rainfall, mean temperature and mean relative humidity. However, no consistent association (r= 0.001; P= 0.99) was found between matrices of drought responses among populations and geographical distances. Similar results were recorded for natural populations of M. truncatula in salt stress (Arraouadi et al., Reference Arraouadi, Badri, Taamalli, Huguet and Aouani2011).
On the other hand, the clustering illustrated in Fig. S1 (available online) of studied lines could be a useful guide for breeding programmes.
Overall, natural populations of M. truncatula showed a large variation in drought tolerance. Four groups of lines differing in their sensitivities to drought stress were identified, with 23 tolerant, 31 moderately tolerant, 14 susceptible and 45 highly susceptible lines. The tolerant group was less affected for LP, LS, NIN and NL than the susceptible group. The results can be used for selecting materials for further investigation of the genes and alleles important for drought tolerance in M. truncatula.
Supplementary material
To view supplementary material for this article, please visit http://dx.doi.org/10.1017/S1479262115000052
Acknowledgements
The authors thank Adel Zitoun for technical assistance in the greenhouse. They thank Maren Friesen, Ken Moriuchi and two anonymous referees for their helpful comments and suggestions. Financial support from Tunisian-French collaborative project (PICS 712), the FP6 European Grain Legume Integrated Project and the Tunisian Ministry of Higher Education and Scientific Research is acknowledged.