Introduction
Modern-day Species distributions in many regions are a consequence of complex dispersal events shaped by the last glaciation (Pedreschi et al., Reference Pedreschi, Garcia-Rodriguez, Yannic, Cantarello, Diaz, Golicher, Korstjens, Heckel, Searle, Gillingham, Hardouin and Stewart2019). Complex colonization histories include the water vole Arvicola amphibius in the British Isles, where there are two genetically distinct populations today, both of which were previously assumed to have arrived during the Holocene. However, ancient DNA (aDNA) analysis of fossil water voles from Britain has suggested that one of the populations of the species had arrived earlier during the last glaciation (Brace et al., Reference Brace, Ruddy, Miller, Schreve, Stewart and Barnes2016). Similar patterns have been made apparent from aDNA analyses of brown bear Ursus arctos that is now believed to have persisted in NW Europe during the Last Glacial Maximum (LGM- c.20.000-c.16.000 BC), possibly including in Britain (Ersmark et al., Reference Ersmark, Baryshnikov, Higham, Argant, Castanos, Doppes, Gasparik, Germonpre, Liden, Lipecki, Marciszak, Miller, Moreno-Garcia, Pacher, Robu, Rodriguez-Varela, Guerra, Sabol, Spassov, Stora, Valdiosera, Villaluenga, Stewart and Dalen2019). This further raises the possibility of other species being present in northern regions during the last glaciation that were previously believed to have colonized in the Holocene. It also raises important questions about how co-evolutionary relationships can be maintained through such climate changes (Graham and Lundelius, Reference Graham, Lundelius, Martin and Klein1984), including those between free-living host species and their parasites. It also prompts questions regarding how vicariance in such organisms takes place (Schlutter, Reference Schlutter2000).
The issues of understanding dispersal and colonization events for parasites with complex lifecycles is important given their frequent use in studies on their ecology and co-evolutionary relationships with free-living hosts (e.g. Lefevre et al., Reference Lefevre, Lebarbenchon, Gauthier-Clerc, Misse, Poulin and Thomas2009). For example, Pomphorhynchus laevis (Zoega in Müller, 1776) is a generalist parasite with a complex lifecycle involving a wide range of definitive hosts and has a broad geographical distribution in the Palaearctic (Kennedy, Reference Kennedy2006; Spakulova et al., Reference Spakulova, Perrot-Minnot and Neuhaus2011). It has been frequently used as a model for testing hypotheses on parasite manipulation, where its modification of the behaviour of its intermediate Gammaridae hosts has been studied extensively (e.g. Fayard et al., Reference Fayard, Francois-Xavier, Wattier and Perrot-Minnot2020). However, the phylogenetic and phylogeographic affinities of P. laevis in relation to that of its various hosts have not been considered. Moreover, high morphological and physiological variability has been reported in P. laevis in both continental Europe (Perrot-Minnot, Reference Perrot-Minnot2004) and the British Isles (Kennedy et al., Reference Kennedy, Bates and Brown1989; O'Mahony et al., Reference O'mahony, Bradley, Kennedy and Holland2004). In combination, both of these aspects are important given that genetic and morphological studies have recently indicated the existence of a cryptic species within the P. laevis species complex (Kral'ova-Hromadova et al., Reference Kral'ova-Hromadova, Tietz, Shinn and Spakulova2003; Perrot-Minnot, Reference Perrot-Minnot2004; Spakulova et al., Reference Spakulova, Perrot-Minnot and Neuhaus2011; Perrot-Minnot et al., Reference Perrot-Minnot, Spakulova, Wattier, Kotlik, Dusen, Aydogdu and Tougard2018; Reier et al., Reference Reier, Sattmann, Schwaha, Harl, Konecny and Haring2019). The presence of two Pomphorhynchus species has also been supported through karyotype analysis (Bombarova et al., Reference Bombarova, Marec, Nguyen and Spakulova2007) with Pomphorhynchus tereticollis (Rudolphi, 1809) recently re-described as a distinct species following genetic work that indicated its divergence from P. laevis (Spakulova et al., Reference Spakulova, Perrot-Minnot and Neuhaus2011; Perrot-Minnot et al., Reference Perrot-Minnot, Spakulova, Wattier, Kotlik, Dusen, Aydogdu and Tougard2018).
Within the British Isles, P. laevis was originally recorded from three river systems, the Thames, the Severn and the Hampshire Avon, with these populations comprising the ‘British strain’. A ‘marine strain’ was also believed to exist in the Baltic and North Sea (Kennedy et al., Reference Kennedy, Bates and Brown1989), which was genetically similar to that found in Ireland and Scotland (O'Mahony et al., Reference O'mahony, Bradley, Kennedy and Holland2004). This discontinuous distribution was explained by natural post-glacial events and subsequent dispersal by anthropogenic activities, such as the translocation of infected fish, especially European barbel Barbus barbus. In the introduced range, the parasite utilized locally available species of Gammaridae as intermediate hosts and cyprinid fishes as final hosts (Kennedy and Rumpus, Reference Kennedy and Rumpus1977). Whilst the only genetic investigation into P. laevis in the British Isles gave support for two major British strains that were associated with different host typologies, this was based on a short fragment of subunit I of cytochrome c oxidase and used only a limited number of samples from specific hosts (O'Mahony et al., Reference O'mahony, Bradley, Kennedy and Holland2004), thus limiting the inferences that can be drawn from these data.
More recent work on Pomphorhynchus spp. across a wide European range used a longer fragment of subunit I of cytochrome c oxidace and included 13 samples from the UK and Ireland, with all samples having been identified as P. tereticollis (Perrot-Minnot et al., Reference Perrot-Minnot, Spakulova, Wattier, Kotlik, Dusen, Aydogdu and Tougard2018). The samples from Great Britain had sequences that belonged to the two main clades, PtL1 and PtL2, which have different phylogeographic histories (Perrot-Minnot et al., Reference Perrot-Minnot, Spakulova, Wattier, Kotlik, Dusen, Aydogdu and Tougard2018). Perrot-Minnot et al. (Reference Perrot-Minnot, Spakulova, Wattier, Kotlik, Dusen, Aydogdu and Tougard2018) demonstrated that P. tereticollis had differentiated in Western and Central Europe and then expanded to form two main lineages; PtL1 (Central and European lineage) which subsequently underwent dispersal, vicariance and admixture between the Baltic Sea and the British Isles, and PtL2 (Western and Ponto-Caspian lineage) whose dispersal, vicariance and admixture occurred within the Rhone and Rhine rivers, the Carpathians and the British Isles. In light of these recent results, it could be hypothesized that the ‘marine strain’ of the British Isles (Kennedy et al., Reference Kennedy, Bates and Brown1989) belongs to the PtL1 lineage whereas the ‘British strain’ (Kennedy et al., Reference Kennedy, Bates and Brown1989) would be of the PtL2 lineage.
Consequently, following this new information on the phylogeography of P. tereticollis (Perrot-Minnot et al., Reference Perrot-Minnot, Spakulova, Wattier, Kotlik, Dusen, Aydogdu and Tougard2018) and the non-species specificity of the genetic region amplified by O'Mahony et al. (Reference O'mahony, Bradley, Kennedy and Holland2004), the aims here were to (a) investigate the genetic and morphological identity of British Pomphorhynchus parasites by increasing the number of Pomphorhynchus parasites tested; and (b) extending the work of O'Mahony et al. (Reference O'mahony, Bradley, Kennedy and Holland2004) and Perrot-Minnot et al. (Reference Perrot-Minnot, Spakulova, Wattier, Kotlik, Dusen, Aydogdu and Tougard2018) by further testing the role of host and vicariance in driving strain formation in Pomphorhynchus spp. in Britain through using newly generated genetic sequences combined with publicly available sequences.
Materials and methods
Sample Collection
Adult Pomphorhynchus spp. samples were obtained from their fish hosts by electric fishing six rivers in England (Table 1). The sampled fish species, comprising of both definitive and paratenic hosts, were Cottus gobio, Squalius cephalus, Barbatula barbatula, Phoxinus phoxinus, Anguila anguila, Salmo trutta, Gobio gobio, Rutilus rutilus and Cyprinus carpio. The fish were identified to species, euthanized (anaesthetic overdose; Tricaine methanesulfonate) and dissected in the laboratory, with any Pomphorhynchus spp. present in the gastrointestinal tract removed. These adult Pomphorhynchus samples were supplemented by the collection of larval samples from five rivers, four for which adult samples were already available (Table 1). The larval samples were collected from their amphipod intermediate hosts. This required the hosts to be collected by kick-sampling in the rivers, sorting for infected amphipods (identified by an orange spot on their back; Sheath et al., Reference Sheath, Dick, Dickey, Guo, Andreou and Britton2018), followed by dissection of the larval parasites from the amphipod host in the laboratory. All Pomphorhynchus spp. samples were then preserved in 98% ethanol.
Table 1. Sampling location and haplotype distribution by species for all samples collected in the present study
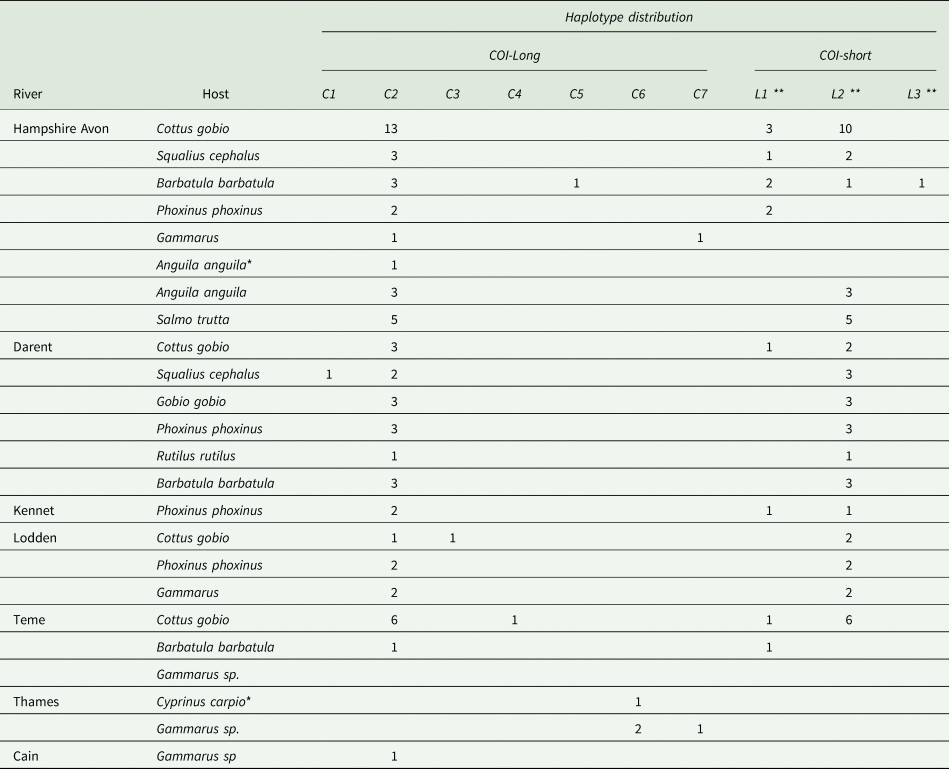
*Old samples; **L1 = AY390511; L2 = AY390509; L3 = AY390512;Haplotypes C2 and C6 correspond to haplotypes Pt22 and Pt23 in Perrot-Minnot et al. (Reference Perrot-Minnot, Spakulova, Wattier, Kotlik, Dusen, Aydogdu and Tougard2018) respectively.
Genetic analyses
Two genetic regions were amplified for each Pomphorhynchus spp. sample (adult and juvenile): (1) subunit I of cytochrome c oxidase (a mitochondrial gene) (Kral'ova-Hromadova et al., Reference Kral'ova-Hromadova, Tietz, Shinn and Spakulova2003; Spakulova et al., Reference Spakulova, Perrot-Minnot and Neuhaus2011); and, (2) the small fragment of subunit I of cytochrome oxidase I used by O'Mahony et al. (Reference O'mahony, Bradley, Kennedy and Holland2004) (with the latter analysed to provide consistency across all the studies, as it represents the only genetic region from historical British Pomphorhynchus samples).
Total genomic DNA was extracted from adult and larval parasites using the Qiagen DNeasy extraction kit (Qiagen) and genetic analyses were performed on the cytochrome oxidase subunit I (COI) gene using primers LCOI1490 and HCO2198 and yielding 583 bp DNA fragments which from here on are referred to as COI-long (primer details in Perrot-Minnot et al., Reference Perrot-Minnot, Spakulova, Wattier, Kotlik, Dusen, Aydogdu and Tougard2018). Strain formation in the British Isles was further investigated by amplifying a separate region of the COI gene using the primers p4 and p3, as per O'Mahony et al. (Reference O'mahony, Bradley, Kennedy and Holland2004), yielding a DNA fragment of 295 bp, hereafter referred to as ‘COI-short’. Polymerase chain reactions (PCRs) were performed using the standard protocol of the Multiplex PCR kit (Qiagen) in 10 μL reaction volume with 10 ng of template DNA and annealing temperature of 50°C for all primer pairs.
Phylogenetic analyses
All sequences were manually aligned using BioEdit ver. 5.0.9 (Hall, Reference Hall1999), with haplotype number determined using DnaSP v.5 (Librado and Rozas, Reference Librado and Rozas2009). The haplotype sequences were deposited in the GenBank database (accession numbers KY075791-KY075800). For phylogenetic analyses, sequences available from GenBank (which included information on the host from which the parasite was extracted from) were added to the dataset (Supplementary material Table 1). Phylogenetic relationships for the COI-long region were reconstructed using MrBayes (Ronquist et al., Reference Ronquist, Teslenko, Van Der Mark, Ayres, Darling, Hohna, Larget, Liu, Suchard and Huelsenbeck2012) using sequences from the present study, plus all the sequences of P. tereticollis available from GenBank, with P. laevis used as an outgroup (LN994840, LN994890, LN994940, LN994943, LN994930 and LN994924). The substitution model, GTR + I, was chosen using jmodeltest (Darriba et al., Reference Darriba, Taboada, Doallo and Posada2012). Robustness of the nodes was estimated using posterior probability with MrBayes (Ronquist et al., Reference Ronquist, Teslenko, Van Der Mark, Ayres, Darling, Hohna, Larget, Liu, Suchard and Huelsenbeck2012). Two independent runs of four heated Markov chains Monte Carlo (MCMC) were used. The generation number was set to 1 000 000 MCMC replications with one tree sampled every 500 generations. The first 250 000 trees were discarded from the analysis (25% burn-in). The tree was visualized using figtree (http://tree.bio.ed.ac.uk/software/figtree/). The median-joining haplotype network was calculated using PopART (Leigh and Bryant, Reference Leigh and Bryant2015).
Testing the influence of host salinity tolerance, country and biogeographical region on strain formation
The influence of host, host salinity tolerance and biogeographical region in explaining the genetic variation with the Pomphorhynchus spp. samples was investigated using all of the COI-long sequences generated in this study, plus the available P. tereticollis sequences available in Genbank (see Supplementary material Table 1); this resulted in 87 sequences for analysis in AMOVA (Arlequin ver 3.5.2.2; Excoffier and Lischer, Reference Excoffier and Lischer2010). Using the COI-short region, the data generated in this study were combined with those of O'Mahony et al. (Reference O'mahony, Bradley, Kennedy and Holland2004) and used in AMOVA to test which variable [country (England, Scotland and Ireland) and host salinity tolerance] explained the most variation within Britain and Ireland. This provided a more balanced design (sequences from 75 stenohaline vs 48 euryhaline hosts) when compared to O'Mahony et al. (Reference O'mahony, Bradley, Kennedy and Holland2004) (20 stenohaline vs 41 euryhaline sequences).
The biogeographical region used followed the work of Perrot-Minnot et al. (Reference Perrot-Minnot, Spakulova, Wattier, Kotlik, Dusen, Aydogdu and Tougard2018). As Perrot-Minnot et al. (Reference Perrot-Minnot, Spakulova, Wattier, Kotlik, Dusen, Aydogdu and Tougard2018) did not assign a biogeographical region to samples from Scotland and Ireland and then in the AMOVA analysis performed here, two possible biogeographical groupings for Scottish and Irish samples were tested for: (a) grouped within the Western Europe–English Channel and (b) in a proposed Northern Europe group (encompassing samples from Central Europe-Baltic Sea, Ireland and Scotland).
Morphological analysis
The genetic analyses were then complemented by morphological characterization (as per Spakulová et al., Reference Spakulova, Perrot-Minnot and Neuhaus2011) of 10 individual Pomphorhynchus parasites collected from 10 fish (Anguila anguila and Oncorhynchus mykiss) from the rivers Thames and Hampshire Avon (Supplementary material Table S1). Archived specimens from the rivers Kennet, Thames and Hampshire Avon were obtained from the Environment Agency (n = 6, England) and the Natural History Museum (n = 4 London, England) and were also assigned to species through morphological identification. These samples originated from English rivers between 1951 and 2015, and all had been previously recorded as P. laevis (as P. tereticollis was considered a junior synonym at that time). For the characterization of the contemporary parasites, live adults were dissected from their fish hosts, relaxed in tap water to promote eversion of the proboscis and fixed in 100% ethanol, before they were cleared using creosote and examined microscopically (x100 to x400) to obtain hook measurements using Image J (Schneider et al., Reference Schneider, Rasband and Eliceiri2012). Small regions of the body from fixed parasites were removed and stored for molecular confirmation.
Results
Phylogenetic analyses and strain formation hypothesis
The COI-long and COI-short gene fragments were amplified from 74 and 62 samples, respectively (Supplementary material Table S2). All sequences were deposited in Genbank (KY075791-KY075817).
The phylogenetic reconstruction using the COI-long used the 74 sequences from the present study and 13 sequences from Perrot-Minnot et al. (Reference Perrot-Minnot, Spakulova, Wattier, Kotlik, Dusen, Aydogdu and Tougard2018). The analysis identified that haplotypes belonging to the two established P. tereticollis lineages are present in Britain, with individuals from Scotland and Ireland grouping within lineage PtL1 and all samples from England (collected in the present study) grouping within lineage PtL2 (Fig. 1). The effect of country, biogeographical region and host salinity tolerance as explanatory variables for the genetic differentiation between lineages PtL1 and PtL2 for the COI-long gene revealed that host salinity tolerance and a potentially northern European biogeographical grouping explained the largest proportion of genetic variation (54.33% and 63.24%, respectively, COI-long; Table 2, Fig. 1). The influence of host and host-salinity tolerance was further tested using the COI-short region using the data generated in the current study (62 sequences) and the 61 sequences generated by O'Mahony et al. (Reference O'mahony, Bradley, Kennedy and Holland2004), and revealed host salinity tolerance was the most significant explanatory variable (Table 2, Figs 2 and 3). These results also demonstrated that the parasites present in western England grouped with those in the lineage present in the River Thames basin (Fig. 1).

Fig. 1. Bayesian tree reconstructed from COI-long sequences of Pomphorhynchus tereticollis. P. laevis was used as an outgroup. Branch support values are posterior probabilities. Haplotypes are indicated in Table S1. Geographical locations are denoted next to the haplotype and are ENG-Englang; FR-France; GER1- Germany(Rhine); GER2-Germany (Baltic); IRE-Ireland; ROM-Romania; SK-Slovakia.

Fig. 2. Network of Pomphorhynchus tereticollis, COI-short (295 bp) from Ireland and Britain. Samples were categorized by host species. Data from the current study (n = 62) and O'Mahony et al. (Reference O'mahony, Bradley, Kennedy and Holland2004) (n = 56) were combined (detailed description of data in Supplementary Table 2). Each line represents 1 base difference between samples.

Fig. 3. Network of Pomphorhynchus tereticollis, COI-short (295 bp) from Ireland and Britain. Data were categorized by country of origin. Data from the current study (n = 62) and O'Mahony et al. (Reference O'mahony, Bradley, Kennedy and Holland2004) (n = 56) were combined (detailed description of data in Supplementary Table 2). Each line represents 1 base difference between samples
Table 2. Results of AMOVA analyses where populations were grouped by country, biogeographic region (as defined by Perrot-Minnot et al., Reference Perrot-Minnot, Spakulova, Wattier, Kotlik, Dusen, Aydogdu and Tougard2018), species and host salinity tolerance. Analyses were performed using sequences generated in this study plus sequences from Perrot-Minnot et al. (Reference Perrot-Minnot, Spakulova, Wattier, Kotlik, Dusen, Aydogdu and Tougard2018) for the long COI and O'Mahony et al. (Reference O'mahony, Bradley, Kennedy and Holland2004)
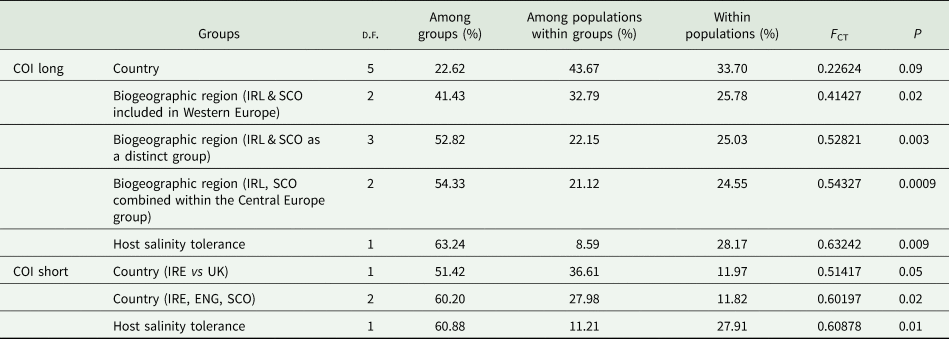
For the long COI, a number of different groupings were tested for Ireland (IRL), Scotland (SCO) and England (ENG): (a) grouped together in the Western Europe biogeographic region; (b) IRL and SCO as a distinct biogeographic region; (c) IRL, SCO grouped within central European group.
Morphological analysis
Morphological analysis of all adult parasites had characteristics consistent with recent descriptions of P. tereticollis (Spakulova et al., Reference Spakulova, Perrot-Minnot and Neuhaus2011). These included the presence of medial hook projections, notably stouter hooks in rows 5–6, presence of hooks on the base of the proboscis bulb and hook morphometrics consistent with the ones reported by Spakulová et al., (Reference Spakulova, Perrot-Minnot and Neuhaus2011) (Supplementary Fig. 1, Supplementary Table S1). Furthermore, the re-examination of archived material also confirmed that all specimens from English rivers, previously identified as P. laevis since 1951, were morphologically consistent with recent descriptions of P. tereticollis.
Discussion
The results here indicate that P. tereticollis is present and established in British freshwaters, with no evidence that P. laevis is also present. This absence of P. laevis included rivers that have previously been reported to harbour historic P. laevis infections. The re-examination of archived material supported the misidentification of P. tereticollis as P. laevis. As such, it is proposed that P. laevis is absent from British freshwaters and that the name P. tereticollis should replace all previous literature pertaining to P. laevis in Britain (e.g Kennedy et al., Reference Kennedy, Bates and Brown1989). This includes the only genetic information purporting to be of British P. laevis, which used the short COI gene that is not species-specific (O'Mahony et al., Reference O'mahony, Bradley, Kennedy and Holland2004). It seems likely that this record also represents P. tereticollis, despite it being reported as P. laevis due to the more precise COI-long sequences reported here from four populations (O'Mahony et al., Reference O'mahony, Bradley, Kennedy and Holland2004; Perrot-Minnot et al., Reference Perrot-Minnot, Spakulova, Wattier, Kotlik, Dusen, Aydogdu and Tougard2018).
The analysis of the genetic data collected in the present study, in combination with all P. tereticollis sequences deposited in Genbank with their reported host, revealed two distinct clades within P. tereticollis, which corresponded to those reported in Perrot-Minnot et al. (Reference Perrot-Minnot, Spakulova, Wattier, Kotlik, Dusen, Aydogdu and Tougard2018). Furthermore, the analysis provides support for the hypothesis put forward by Perrot-Minnot et al. (Reference Perrot-Minnot, Spakulova, Wattier, Kotlik, Dusen, Aydogdu and Tougard2018) that parasites belonging to clade PtL1, possibly associated with cold-adapted fish, may have spread to the northern parts of Britain from the Baltic region via a northern route across the estuarine area in what is now the North Sea during the last glaciation. The second lineage, PtL2, may have arrived later via the Rhine/Thames fluvial connection during the last Glaciation or early Holocene when sea levels were low (as proposed by Perrot-Minnot et al., Reference Perrot-Minnot, Spakulova, Wattier, Kotlik, Dusen, Aydogdu and Tougard2018). The present data support this, as the English P. tereticollis populations cluster with P. tereticollis from the River Rhine which is one of the main rivers which is connected to East flowing English rivers such as the Thames at that time. Subsequently, P. tereticollis has invaded numerous western flowing rivers through the translocation of its main definitive hosts, particularly B. barbus and has been documented to expand its range soon after its introduction (Kennedy et al., Reference Kennedy, Bates and Brown1989).
The presence of a morphologically and genetically distinct P. tereticollis in Scotland and Ireland (Evans et al., Reference Evans, Matthews and McClintock2001; O'Mahony et al., Reference O'mahony, Bradley, Kennedy and Holland2004) had been initially linked to a different strain, which possibly arose due to adaptation to salinity tolerant host species. In the current study, analyses with both COI gene segments (long and short) strongly support the effect of host salinity tolerance in explaining the genetic differentiation between the P. tereticollis lineages PtL1 and PtL2, as proposed by Perrot-Minnot et al. (Reference Perrot-Minnot, Spakulova, Wattier, Kotlik, Dusen, Aydogdu and Tougard2018). The analyses of variance conducted here, where samples from Ireland and Scotland were grouped within the Central European-Baltic sea biogeographic region (as per Perrot-Minnot et al., Reference Perrot-Minnot, Spakulova, Wattier, Kotlik, Dusen, Aydogdu and Tougard2018), suggest the possibility that the PtL1 lineage spread from that region in the past. It appears to have co-evolved with cold and salinity tolerant fish hosts such as brown trout Salmo trutta, but it can still infect cyprinid species (Fig. 1). Phylogeographic studies of S. trutta have suggested they survived as a species through the Late Pleistocene in northern refugia in Europe and that British populations cannot be distinguished from the continental populations, suggesting a relatively recent dispersal or connection (Bernatchez, Reference Bernatchez2001). This may imply that a movement of S. trutta with the PtL1 lineage of the P. tereticollis parasite spread since the end of the Last Glacial Maximum (post-LGM; circa 16 kyrs ago) from the Baltic region to Britain. This, in turn, may have been enabled by the low lying northern Doggerland area, which would have been dominated by deltaic/estuarine environments that possibly provided a link between the Baltic and northern Britain, including Scotland, during low sea levels (Sturt et al., Reference Sturt, Garrow and Bradley2013). This scenario is consistent with the phylogeographic analyses of other cold-tolerant fish, such as C. gobio (Hanfling et al., Reference Hanfling, Hellemans, Volckaert and Carvalho2002), which suggest the survival of fish throughout the LGM in northern European rivers.
A second dispersal route is suggested for the PtL2 parasite lineage, with its association with warmer, freshwater adapted fish hosts. This was likely to have occurred later, during the Early Holocene, or perhaps the Late Glacial Interstadials, due to the warmer association of the specific fish hosts. However, it had to occur prior to the inundation by rising seas levels of the fluvial connection between the Rhine and the Thames (and other East flowing Southern English river catchments) by ca. 7 kyrs (Sturt et al., Reference Sturt, Garrow and Bradley2013).
The majority of the hosts sampled in this study were fish and the intermediate hosts were not as widely sampled. Therefore, the influence of the intermediate hosts and their respective vicariance in explaining the phylogeographic patterns of P. tereticollis remains largely unexplored. The parasite has a complex lifecycle and would have required the presence of both intermediate and final hosts in its colonization of the British Isles. Future work could therefore focus on the intermediate hosts, especially for the PtL1 lineage, with the exploration of the role that they have played in the colonization route of the northern British Isles. Sampling should include both fresh and estuarine waters.
In conclusion, the present study provides further support for the proposed hypotheses of Perrot-Minnot et al. (Reference Perrot-Minnot, Spakulova, Wattier, Kotlik, Dusen, Aydogdu and Tougard2018) to explain the phylogeography of P. tereticollis. It also updates the genetic work by O'Mahony et al. (Reference O'mahony, Bradley, Kennedy and Holland2004) on the Pomphorhynchus parasites from the British Isles. The results confirm that P. tereticollis is the only Pomphorhynchus parasite species present in British freshwaters, raising a series of evolutionary and ecological questions that require further investigation. Of particular interest is the potential to use P. tereticollis as a model species to address the role of host-specialization driving speciation in generalist parasites, especially as a comparator to P. laevis. In particular, P. tereticollis can be used to better understand the relationship between a generalist parasite and its variously environmentally adapted hosts, and the consequences of this to vicariance in that parasite.
Supplementary material
The supplementary material for this article can be found at https://doi.org/10.1017/S0031182020001663.
Financial support
This work received financial support from Bournemouth University – Fusion Fund
Conflict of interest
The authors declare no conflicts of interest.
Ethical standards
Not applicable.