Introduction
The broad fish tapeworm Dibothriocephalus latus (Cestoda; Diphyllobothriidea) is the most frequent causative agent of diphyllobothriosis, a fish-borne zoonosis, in Europe. Copepods and freshwater fish are the first and second intermediate hosts, respectively. The European perch Perca fluviatilis (Percidae), Northern pike Esox lucius (Esocidae), burbot Lota lota (Lotidae) and ruffe Gymnocephalus cernua (Percidae) are the most suitable and frequent fish hosts of D. latus (for a review, see Králová-Hromadová et al., Reference Králová-Hromadová, Radačovská, Čisovská Bazsalovicsová and Kuchta2021). Fish-eating mammals (mainly Canidae and Felidae) may serve as definitive hosts of D. latus (Bouvier et al., Reference Bouvier, Hörning and Matthey1963; Zottler et al., Reference Zottler, Bieri, Basso and Schnyder2019), but humans play the most important role in the circulation of D. latus in the environment (von Bonsdorff, Reference von Bonsdorff1977). The life cycle of D. latus is maintained when humans consume raw or insufficiently cooked fish products harbouring larval stages, plerocercoids.
The tapeworm is distributed in Eurasia and North and South America (for a review, see Králová-Hromadová et al., Reference Králová-Hromadová, Radačovská, Čisovská Bazsalovicsová and Kuchta2021). Some studies support the theory of the introduction of D. latus to North America by immigrants from endemic European regions (von Bonsdorff, Reference von Bonsdorff1977), but others have suggested that D. latus was already present in North America prior to European immigration (Jenkins et al., Reference Jenkins, Castrodale, de Rosemond, Dixon, Elmore, Gesy, Hoberg, Polley, Schurer, Simard and Thompson2013). The tapeworm was also introduced to some Patagonian lakes in South America (for a review, see Torres and Yera, Reference Torres, Yera, Rose and Jiménez-Cisneros2018; Kuchta et al., Reference Kuchta, Radačovská, Bazsalovicsová, Viozzi, Semenas, Arbetman and Scholz2019).
Several foci of diphyllobothriosis with different dynamics of occurrence have developed in Europe. The level of infection decreased substantially or was eliminated after massive health campaigns and effective treatment in Fennoscandia, the Baltic region, and the Danube Delta in the last decades of the 20th century. In contrast, the ongoing circulation of D. latus in the natural environment and in humans has been documented in the Alpine lakes region (ALR) (for a review, see Králová-Hromadová et al., Reference Králová-Hromadová, Radačovská, Čisovská Bazsalovicsová and Kuchta2021) and throughout Russia (von Bonsdorff, Reference von Bonsdorff1977; Dugarov and Pronin, Reference Dugarov and Pronin2017; Chugunova et al., Reference Chugunova, Ronzhina and Syromyatnikov2020).
Diphyllobothriosis has persisted in ALR despite great efforts to eliminate the further spread of the disease, mainly due to eating habits and the proliferation of restaurants serving raw fish, insufficient disinfection of wastewater and the many yachters and fishermen using the lakes (Dupouy-Camet and Peduzzi, Reference Dupouy-Camet and Peduzzi2004; Jackson et al., Reference Jackson, Pastore, Sudre, Loutan and Chappuis2007). Since 2000, diphyllobothriosis has been diagnosed in human patients from Switzerland, Italy and France and D. latus has also been frequently detected in fish from various subalpine lakes (Wicht et al., Reference Wicht, Ruggeri-Bernardi, Yanagida, Nakao, Peduzzi and Ito2010a, Reference Wicht, Yanagida, Scholz, Ito, Jiménez and Brabec2010b; Dupouy-Camet et al., Reference Dupouy-Camet, Haidar, Dei-Cas, Yera, Espinat, Benmostefa, Guillard and Aliouat-Denis2015; Menconi et al. Reference Menconi, Pastorino, Momo, Mugetti, Bona, Levetti, Tomasoni, Pizzul, Ru, Dondo and Prearo2020, Reference Menconi, Zoppi, Pastorino, Di Blasio, Tedeschi, Pizzul, Mugetti, Tomasoni, Dondo and Prearo2021).
The ongoing circulation of D. latus in the natural environment of subalpine lakes has offered biological material for molecular and genetic studies of the parasite (Nicoulaud et al., Reference Nicoulaud, Yéra and Dupouy-Camet2005; Yera et al., Reference Yera, Estran, Delaunay, Gari-Toussaint, Dupouy-Camet and Marty2006, Reference Yera, Nicoulaud and Dupouy-Camet2008; Wicht et al., Reference Wicht, de Marval and Peduzzi2007, Reference Wicht, Ruggeri-Bernardi, Yanagida, Nakao, Peduzzi and Ito2010a, Reference Wicht, Yanagida, Scholz, Ito, Jiménez and Brabec2010b; Bazsalovicsová et al., Reference Bazsalovicsová, Koleničová, Králová-Hromadová, Minárik, Šoltys, Kuchta and Štefka2018). A recent in-depth karyological analysis of 2 D. latus specimens from European perch from Iseo lake (northern Italy) found a very atypical chromosomal structure (Orosová et al., Reference Orosová, Marková, Provazníková, Oros, Radačovská, Čadková and Marec2021). Both tapeworms contained majority of the cells with the triploid number of chromosomes (3n = 27, 46.7%), but 5.9% of cells displayed diploid (2n = 18) chromosomal set. A high level of aneuploidy, represented by 20, 22, 24 and 26 chromosomes, was also detected in 3.7, 7.0, 12.9 and 23.9% of the cells, respectively. Fluorescent in situ hybridization using a probe for the small ribosomal subunit demonstrated the presence of 3 clusters of hybridization signals on chromosome triple no. 7, thus confirming the triploidy of the specimens. The course of meiosis was aberrant and indicated an absence of fully developed spermatic cells and mature functional spermatozoa. Parthenogenetic reproduction was concluded to be the most probable method of reproduction of triploid D. latus from Iseo lake (Orosová et al., Reference Orosová, Marková, Provazníková, Oros, Radačovská, Čadková and Marec2021).
The unique chromosomal structure of D. latus raised the question of how intraindividual chromosomal polymorphism is displayed at the DNA level and how is parthenogenesis linked to the genetic structure of the species. To answer these questions, 3 mitochondrial genes (mtDNA) were first used to analyse the structure and overall level of intraspecific polymorphism of D. latus populations from the ALR and a selected locality in Russia. Microsatellites (short tandem repeats, STRs) have been used for obtaining a more detailed assessment of the genetic structure of populations relative to mtDNA. Six microsatellite loci, previously designed for D. latus by microsatellite library screening (Bazsalovicsová et al., Reference Bazsalovicsová, Koleničová, Králová-Hromadová, Minárik, Šoltys, Kuchta and Štefka2018), were analysed to determine the number of allelic variants at each locus and their possible association with the variable number of chromosomes and method of reproduction.
Materials and methods
Study areas
Plerocercoids of D. latus were isolated from fish intermediate hosts from 6 lakes in ALR: (1) Iseo lake, Italy (IT-IS), (2) Como lake, Italy (IT-CO), (3) Maggiore lake, Italy (IT-MA), (4) Lake Geneva, Switzerland (CH-GE), (5) Neuchâtel lake, Switzerland (CH-NE) and (6) Biel lake, Switzerland (CH-BI) (Fig. 1; Table 1). Maggiore lake is on the border between Italy and Switzerland; our samples originated from the Italian side of the lake. Similarly, Lake Geneva lies between Switzerland and France; plerocercoids were obtained from the Swiss part of the lake.
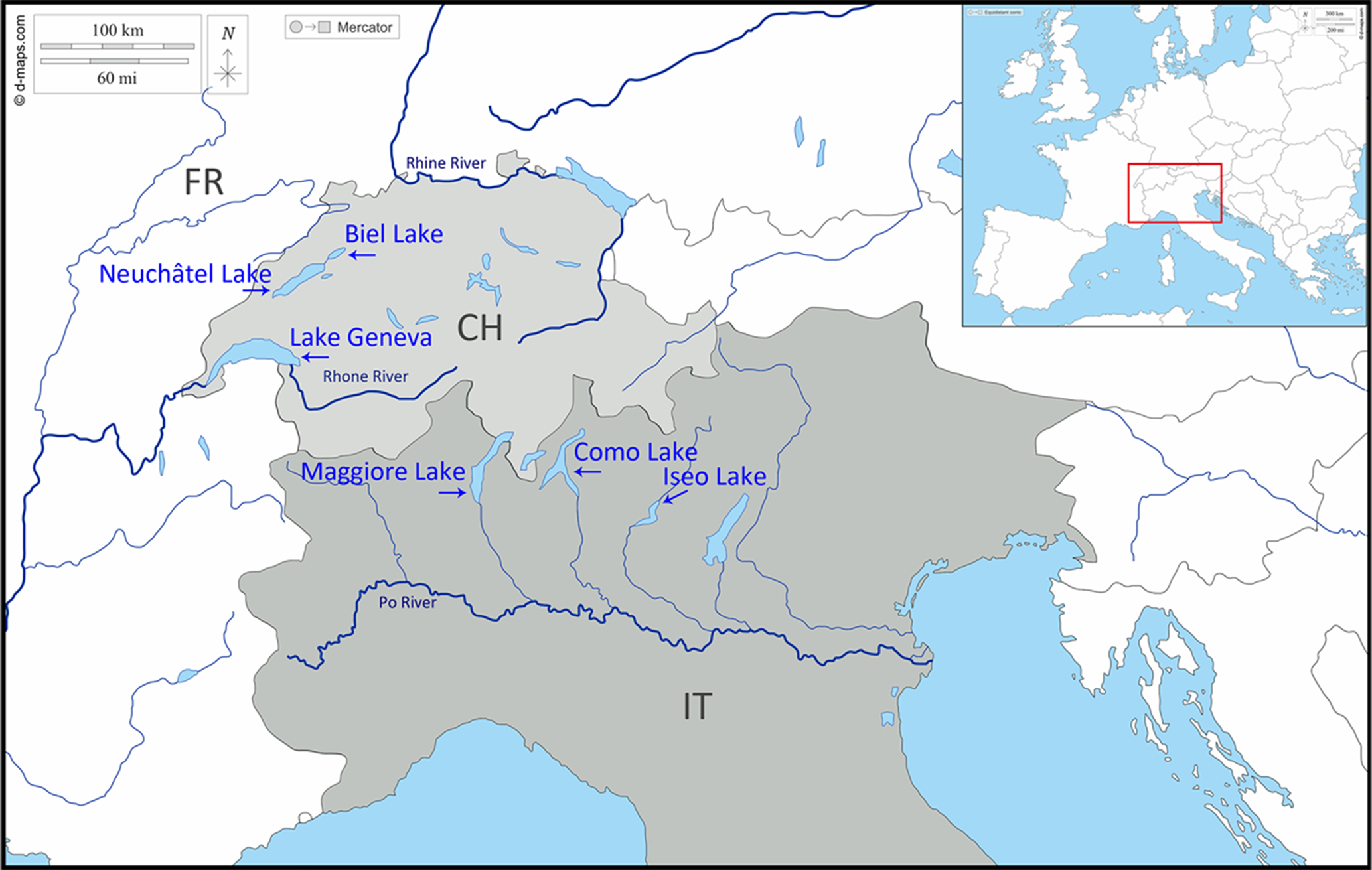
Fig. 1. Hydrological map of the study area of Dibothriocephalus latus from the ALR. CH, Switzerland; FR, France; IT, Italy.
Table 1. Details of the Dibothriocephalus latus populations from ALR and RU-KR

Iseo lake was our first choice for collecting samples because intraindividual chromosomal polymorphism has recently been detected in D. latus from this lake (Orosová et al., Reference Orosová, Marková, Provazníková, Oros, Radačovská, Čadková and Marec2021). The other 5 subalpine lakes were chosen as study areas due to their similar ecological conditions. The Italian lakes Iseo, Como and Maggiore were originally oligotrophic but underwent severe cultural eutrophication caused by urban, industrial and/or agricultural pollution (Manca et al., Reference Manca, Torretta, Comoli, Amsinck and Jeppesen2007; Menconi et al., Reference Menconi, Zoppi, Pastorino, Di Blasio, Tedeschi, Pizzul, Mugetti, Tomasoni, Dondo and Prearo2021). Lakes Geneva, Biel and Neuchâtel in Switzerland suffered from eutrophication but later became mainly mesotrophic (Lang, Reference Lang1989; Anneville et al., Reference Anneville, Molinero, Souissi, Balvay and Gerdeaux2007). Lakes Iseo, Como, Maggiore and Geneva represent isolated aquatic biotopes with no connection via common tributaries or outlets; Neuchâtel and Biel lakes are connected by the Thielle channel (Fig. 1). The high prevalence of D. latus has been confirmed in European perch, Northern pike and burbot in all 6 subalpine lakes (for a review, see Králová-Hromadová et al., Reference Králová-Hromadová, Radačovská, Čisovská Bazsalovicsová and Kuchta2021).
Dibothriocephalus latus specimens from fish from a distant locality, the Krasnoyarsk Reservoir in Russia (RU-KR) (southern Siberia, Asian part of Russia), were analysed as a comparative population (Fig. 2A; Table 1). This reservoir is one of the largest artificial reservoirs in the world. The construction of its dam began in 1956, and subsequent filling with water from Yenisey River ended in 1970. The reservoir is >388 km long and 15 km wide with an average depth of 36.7 m (Vishegorodtsev et al., Reference Vishegorodtsev, Kosmakov, Anufrieva and Kuznetsova2005). Several major rivers flow into the reservoir and form bays. The section of the Yenisey River downstream from the city of Krasnoyarsk never freezes in winter as the dam is located at the Krasnoyarsk hydroelectric power station, even though the river is in Siberia. Fish, mainly European perch and Northern pike, have an important role in human nutrition in this region. Diphyllobothriosis remains an important problem due to the frequent consumption of fish and ranks third in the statistics of helminthiases (11%) in the Krasnoyarsk territory (Chugunova et al., Reference Chugunova, Ronzhina and Syromyatnikov2020).

Fig. 2 (A) Map of the ALR and the RU-KR, with detected concatenated mitochondrial haplotypes (con-Ha). The map was obtained from d-maps.com. (B) Haplotype network of D. latus from the ALR and RU-KR, based on con-Ha of the mitochondrial cox1, cob and nad3 genes. The sizes of the haplotypes are proportional to the number of samples. Each hatch mark represents a mutation, and black dots represent intermediate missing or unsampled haplotypes.
Parasitic material
A total of 146 samples of D. latus (113 plerocercoids and 15 adult tapeworms from ALR and 18 plerocercoids from RU-KR) was analysed (Table 1). Details for each specimen are provided in Supplementary material Table 1. Plerocercoids (n = 131) were obtained mainly from the musculature and/or abdominal cavity and internal organs of P. fluviatilis, E. lucius and L. lota caught by professional fishermen in the 6 subalpine lakes (Fig. 1) and RU-KR (Fig. 2A) in 2017 and 2018. Fish were kept on ice and dissected immediately without previous freezing. Complete helminthological dissection of each fish included examination of the abdominal cavity and internal organs and detailed inspection of thin slices (approximately 5 mm) of musculature. Plerocercoids were rinsed in a saline solution and preserved in 96% ethanol for analysis.
Pieces of strobilae of adult tapeworms (n = 15) were collected by Dr Barbara Wicht from Switzerland, deposited in the helminthological collection of the Institute of Parasitology, Biology Centre, Czech Academy of Sciences, České Budějovice, Czech Republic, and kindly provided by Professor Tomáš Scholz and Dr Roman Kuchta. Samples from human patients (n = 14) originated from different localities in the Swiss cantons of Geneva, Vaud and Valais (shore of lake Geneva) and from the canton of St. Gallen (the northeastern part of Switzerland) near lake Constance. A single adult tapeworm isolated from a red fox (Vulpes vulpes) from the canton of the Grisons was also included in the analysis (Table 1; Supplementary material Table 1).
Isolation of DNA
Genomic DNA was isolated either from entire plerocercoids or from 20 mg of tissue from adult tapeworms using the QIAamp® DNA mini Kit (Qiagen, Hilden, Germany) following the manufacturer's recommendations. The DNA was diluted in deionized water and stored at −20°C for molecular analysis.
Molecular identification by multiplex polymerase chain reaction
A differential multiplex polymerase chain reaction (PCR) test based on the mitochondrial cytochrome c oxidase subunit I gene (cox1 mtDNA) was designed by Wicht et al. (Reference Wicht, Yanagida, Scholz, Ito, Jiménez and Brabec2010b) for discriminating between the most common diphyllobothriid species infecting humans: D. latus, Dibothriocephalus dendriticus, Dibothriocephalus nihonkaiensis and Adenocephalus pacificus. This test was applied for the species-specific identification of all specimens. Five primers were used in each multiplex PCR. One reverse primer (MulRevCom) was common for all diphyllobothriid species, and 4 forward primers were species-specific: MulLat3 for D. latus, MulDen4 for D. dendriticus, MulPac2 for A. pacificus and MulNih5 for D. nihonkaiensis. Details of the primers are provided in Table 2 (see section I). The PCR amplification was performed in a total volume of 20 μL containing 10–20 ng of genomic DNA, 10 pmol of each of the 5 primers and 1× Master Mix (Thermo Fisher Scientific Inc., Waltham, USA). The PCR amplification conditions were 5 min at 95°C as an initial denaturation step, then 40 cycles of 30 s at 95°C, 1 min at 60°C, 1 min at 72°C and a final polymerization step for 10 min at 72°C. The PCR products were visualized on a 1.5% agarose gel.
Table 2. Summary and details of the primers

Ref., reference; 1, Wicht et al. (Reference Wicht, Yanagida, Scholz, Ito, Jiménez and Brabec2010b); 2, Wicht et al. (Reference Wicht, Ruggeri-Bernardi, Yanagida, Nakao, Peduzzi and Ito2010a); 3, Yera et al. (Reference Yera, Estran, Delaunay, Gari-Toussaint, Dupouy-Camet and Marty2006); 4, Bazsalovicsová et al. (Reference Bazsalovicsová, Koleničová, Králová-Hromadová, Minárik, Šoltys, Kuchta and Štefka2018).
Selection and PCR amplification of mitochondrial genes
Three mitochondrial genes were analysed: (1) partial cox1 (426 bp; primers MulLat3 and MulRevCom) obtained during the identification of species by multiplex PCR, (2) complete cytochrome b (cob, 1107 bp; primers F2Dnihcob and R2Dnihcob) and (3) complete nicotinamide dehydrogenase subunit 3 (nad3, 357 bp; primers DnND3Fo and DnND3Re). The sequences of the primers used for the PCR amplification and sequencing of the mitochondrial genes are presented in Table 2 (see section II); the scheme of the amplified regions and locations of the primers is provided in Supplementary material Fig. 1. The PCR amplification of partial cox1 was as described above. The amplification of the cob and nad3 genes was carried out using the following conditions: 5 min at 95°C as an initial denaturation step, then 35 cycles of 1 min at 94°C, 30 sec at 56°C (cob) or 1 min at 50°C (nad3), 1 min at 72°C and a final polymerization step for 4 min (cob) or 10 min (nad3) at 72°C.
Purification and sequencing of the mitochondrial genes
The PCR products were visualized on a 1.5% agarose gel and purified using ExoProStar™ 1-Step (Illustra™, GE Healthcare, Little Chalfont, UK). The products were sequenced using a 3500 Genetic Analyser (Thermo Fisher Scientific) and the BigDye Terminator v3.1 Cycle Sequencing kit (Thermo Fisher Scientific). Contiguous sequences were assembled and inspected for errors using Geneious software (version 10.0.5, Biomatters, Auckland, New Zealand). The cox1, cob and nad3 datasets were concatenated using SeaView 4.2 (Gouy et al., Reference Gouy, Guindon and Gascuel2010).
Statistical analysis of the sequences of the mitochondrial genes
Genealogical information was visualized by a haplotype network using concatenated mitochondrial data in PopArt (Leigh and Bryant, Reference Leigh and Bryant2015) with the TCS 1.21 algorithm (Clement et al., Reference Clement, Posada and Crandall2000). Haplotype diversity (Hd), nucleotide diversity (Pi) and neutrality test statistics (Tajima's D, Fu and Li's F* and Ramos-Onsins and Rozas’ R 2) were calculated for the concatenated data using DNASP 6 (Rozas et al., Reference Rozas, Ferrer-Mata, Sánchez-DelBarrio, Guirao-Rico, Librado, Ramos-Onsins and Sánchez-Gracia2017). The statistical parameters were calculated independently for (1) the entire dataset, (2) the ALR population and (3) the RU-KR population. The significance of all tests was obtained using 10 000 coalescent simulations.
PCR amplification and fragment analysis of microsatellite loci
Six microsatellite loci, DL_6/(agg)n, DL_8/(tcc)n, DL_10/(cta)n, DL_36/(ctt)n, DL_104/(tag)n and DL_112/(ttc)n, were previously identified for D. latus by screening a microsatellite library (Bazsalovicsová et al., Reference Bazsalovicsová, Koleničová, Králová-Hromadová, Minárik, Šoltys, Kuchta and Štefka2018). Primers for their PCR amplification are summarized in Table 2 (see section III). The PCR amplification for the microsatellite assays was performed in a total volume of 20 μL containing 10–20 ng of genomic DNA, 5 pmol of each primer (1 was fluorescently labelled), and 1× HOT FIREPol® Blend Master Mix with 7.5 mm MgCl2 (Solis Biodyne, Tartu, Estonia). The conditions of the PCRs were: 15 min at 95°C, followed by 26 cycles of 15 s at 95°C, 90 s at 60°C, 90 s at 72°C and a final step for 10 min at 72°C.
For the fragment analysis, 1 μL of the amplified PCR product was mixed with 8.5 μL of HiDi formamide and 0.5 μL of GeneScan-LIZ 500 Size Standard (Thermo Fisher Scientific). The mixture was then denatured for 5 min at 95°C and capillary electrophoresis on 3500 Genetic Analyser (Thermo Fisher Scientific) was performed. GeneMapper v.3.7 (Applied Biosystems) was used for genotyping the samples.
Statistical analysis of the microsatellite allelic variants
Genodive 3.0 (Meirmans, Reference Meirmans2020) was used for the calculation of basic statistical parameters of genetic diversity (number of alleles, effective number of alleles, observed heterozygosity and heterozygosity within a population), Nei's D genetic distance (Nei, Reference Nei1972) and genotype assignment within populations. A principal coordinates analysis (PCoA) was used for visualizing the pattern of genetic relationships of D. latus individuals and populations using GenAlex 6.6 (Peakall and Smouse, Reference Peakall and Smouse2012). Data for PCoA were exported from Genodive 3.0 and analysed independently in 2 dataset formats. The first format was based on an analysis that included all alleles detected in each individual, including 3 alleles of the triploid individuals, and the analysis of the second format was based on 2 alleles, which were randomly downsampled in the triploid individuals. They were assigned to unique multilocus genotypes (genets) per population using the Meirmans and Van Tienderen's (Reference Meirmans and Van Tienderen2004) algorithm, which tested the probability of finding the observed genotype assignment under random mating. A threshold distance of 3 was chosen based on the ‘valley’ between the first and second peaks in a histogram of pairwise distances. Samples from CH-BI, CH-NE and CH-F were not included in the analyses of Nei's D genetic distance and genotype diversity due to the small number of samples. Statistical support for the pairwise Nei's D values was obtained by 10 000 permutations of the data.
Results
Structure and number of mitochondrial haplotypes
Partial cox1 (426 bp), complete cob (1107 bp) and complete nad3 (357 bp) were sequenced for 146 D. latus specimens from all localities; their accession numbers are provided in Supplementary material Table 1. We detected totals of 3 cox1 (CO1-Ha), 11 cob (COB-Ha) and 3 nad3 (ND3-Ha) haplotypes. Of the 3 polymorphic sites (all transitions) detected in cox1, two were responsible for a change in amino acid sequence. Twenty-two substitutions (transitions/transversions ratio of 21/1) were identified for the cob gene, 9 of which changed the amino acid sequence. The analysis of the nad3 sequences identified 2 transitions, one of which caused a change in amino acid. Concatenation of the data resulted in 12 haplotypes (con-Ha) (Supplementary material Table 2). The concatenated dataset contained 25 variable characters, 13 of which were parsimoniously informative.
All plerocercoids from fish from lakes Iseo, Como, Maggiore, Geneva, Biel and Neuchâtel, 1 plerocercoid from fish from RU-KR (RU-KR/6) and adult tapeworms from all humans except for 1 and from the fox from Switzerland possessed identical sequence structures of the cox1, cob and nad3 genes, resulting in a common concatenated con-Ha1 haplotype (total of 128 individuals) (Supplementary material Table 1; Fig. 2A). One adult tapeworm isolated from a human patient from Switzerland (CH-H/12) possessed a specific COB-Ha2, which resulted in a unique con-Ha2.
Intrapopulation polymorphism was substantially higher in the population of D. latus from RU-KR. Three cox1 haplotypes (CO1-Ha1–Ha3), 10 cob haplotypes (COB-Ha1, COB-Ha3–Ha11) and 3 nad3 haplotypes (ND3-Ha1–Ha3) were detected in 18 D. latus individuals. They resulted in 11 concatenated haplotypes, particularly con-Ha1 (detected only in RU-KR/6) specific also for tapeworms from ALR, and con-Ha3–Ha12 (Supplementary material Tables 1 and 2) unique to the population from RU-KR (Fig. 2A).
Haplotype network
The haplotype network based on the concatenated dataset of the cox1, cob and nad3 sequences showed differences between the ALR and RU-KR populations (Fig. 2B). The analysis found a star-like pattern, with 1 dominant central haplotype (con-Ha1) shared by the majority of the D. latus samples (n = 128; for details see above). In contrast to ALR, notable genetic diversity in the D. latus population from RU-KR was demonstrated by a higher number of concatenated haplotypes. Of 11 haplotypes, 6 unique haplotypes from RU-KR were separated from the central haplotype by 1 or 2 mutations (con-Ha3, 4, 6, 8, 9 and 11). Haplotype con-Ha10 from RU-KR was placed on the mutation pathway with a specific haplotype detected in 1 adult tapeworm from a human infection (con-Ha2; CH-H/12) from Switzerland. Three additional concatenated haplotypes (con-Ha5, 7 and 12) from RU-KR differed from the central haplotype by 3–7 mutations.
Characterization of the populations of D. latus from ALR and RU-KR performed using DNASP produced similar results. The ALR population had fewer haplotypes and lower haplotype (Hd) and nucleotide (Pi) diversities compared to the RU-KR population (Table 3).
Table 3. Molecular variability and tests of neutrality for the 3 mtDNA genes of D. latus

ALR, Alpine lakes region; RU-KR, Krasnoyarsk reservoir in Russia; N, number of samples; Hn, number of haplotypes; Hd, haplotype diversity; Pi, nucleotide diversity.
*P < 0.05; **P < 0.02; ***P < 0.001.
The neutrality tests, Tajima's D and Fu and Li's F*, which were applied to indicate past demographic events or other processes, found significant negative values in the ALR population and the entire dataset. Ramos-Onsins and Rozas’ R 2 test value was significantly low for the entire dataset. Negative values of the neutrality tests, indicating an excess of rare nucleotide-site variants under the neutral model of evolution, are usually interpreted as a sign of population expansion after a bottleneck or a selective sweep. The lower degree of mitochondrial polymorphism, however, may be a result of either purifying or adaptive natural selection. Mitochondrial DNA acts as a single locus due to the lack of recombination, so selection anywhere in mtDNA would exactly mimic a demographic bottleneck/expansion (Bazin et al., Reference Bazin, Glémin and Galtier2006). However, the results of statistical tests of neutrality can provide only hypothetical explanations of the past events responsible for the genetic structure of the population.
Tajima's D and Fu and Li's F* tests identified negative but non-significant values in the RU-KR population, whereas the value of Ramos-Onsins and Rozas’ R 2 test was significantly low (Table 3). These results suggested that the RU-KR population may also have experienced a sudden expansion in its recent evolutionary history.
Variations in allelic numbers of microsatellite loci
A standard number of 1 (homozygous) or 2 (heterozygous) alleles per microsatellite locus was detected in all plerocercoids from fish from RU-KR and in 2 D. latus adults (CH-H/1, CH-H/12) obtained from the human patients from Switzerland (Supplementary material Table 1). This type of microsatellite pattern was assigned to allelic type A1-2 and is typical for a diploid organism. Higher percentages (65–90%) of heterozygous forms were observed in all loci except for one (DL_6) (Table 4).
Table 4. Numbers of alleles (A) present in sampled individuals for each microsatellite locus with the percentages (%) and number of individuals (no. of ind.) with 1 allele (1A), 2 alleles (2A) and 3 alleles (3A)

Fragment analysis of D. latus from fish from ALR, from the fox, and from the majority of the humans from Switzerland identified 1, 2 or 3 peaks corresponding to 1, 2 or 3 alleles, respectively. This allelic type of microsatellite pattern was assigned to A1-3 and corresponded to a triploid organism. The majority of loci (DL_10, DL_36, DL_104 and DL_112) had 1 to 3 alleles, with a notably lower percentage (0.79–8.73%) of variants of 1 allele (homozygous) (Table 4). Locus DL_6 completely lacked the 1-allele variant, whereas heterozygous variants of 3 alleles were present in most of the specimens (96.04%). Three alleles were not observed only at the locus DL_8, which had 2 alleles in 99.21% (125 of 126 tapeworms).
Basic statistics of allelic variants of microsatellite loci and genetic diversity
The level of polymorphism was evaluated across the 6 microsatellite loci in 141 individuals from ALR (IT-IS, IT-CO, IT-MA, CH-GE and CH-H) and RU-KR (Table 5). Populations that contained fewer than 4 samples (CH-BI, CH-NE and CH-F) were excluded from the descriptive statistics, although a correction for the bias from sampling a limited number of individuals per population was included in some tests.
Table 5. Descriptive statistics for the 6 microsatellite loci of D. latus

Num, number of alleles observed; Eff_num, effective number of alleles; Ho, observed heterozygosity; Hs, heterozygosity within populations.
See Table 1 for the population codes.
The total number of observed (Num) and the effective number of alleles (Eff_num) differed between the populations from ALR and RU-KR (Table 5). The number of alleles per population was highest in D. latus from RU-KR (8.333), and fewer alleles were generally detected in ALR, with the fewest in larvae from lake Geneva (CH-GE, 2.667). Similarly, Eff_num was highest in D. latus from RU-KR (4.814) and lower in the ALR populations (2.581–2.867).
Observed heterozygosity (0.729–0.869) was similar in the entire dataset, indicating more heterozygotes in both populations. The heterozygosity within populations (Hs, gene diversity), however, was highest in RU-KR (0.783) (Table 5). The results indicated similar Hs values and close genetic relationships amongst D. latus from ALR, but the population from RU-KR was genetically diverse.
Genetic relatedness/dissimilarity and genotype diversity amongst populations
Genetic distance amongst the populations was determined using Nei's D matrix of genetic distances, which included a bias correction for small sample sizes. The populations from ALR were genetically very similar. The RU-KR population was genetically the most distant based on the means of Nei's D (mean distance = 0.17, Table 6), consistent with the geographic distance between the RU-KR and ALR populations.
Table 6. Pairwise population matrix of Nei's D genetic distance for the populations

a Mean distance of a population was determined by dividing the sum of all pairwise distances of a particular population by 6, which is the number of populations paired with the concerned population.
All pairwise values were statistically significant at P < 0.001.
See Table 1 for the population codes.
Genotype assignment analysis identified 51 genets in the entire dataset. In the ALR population, 123 individuals comprised of only 36 genets, substantially less than expected under random mating. In contrast, the RU-KR population had equal numbers of observed and expected genets (Table 7).
Table 7. Number of observed (Num_obs) and expected (Num_exp) genotypes assigned to specific populations

P, low value rejects the null hypothesis that expected and observed values are equal.
See Table 1 for the population codes.
PCoA of microsatellites
The results of the PCoA were in agreement with the descriptive statistics but identified a more detailed pattern of relationships amongst the populations. Slightly different results were obtained with the 2 formats of input data applied for the PCoA.
The results of the first dataset, which accepted all alleles detected in each individual, including the 3 alleles present in triploids in the input data, identified 2 clusters of D. latus individuals collected from all populations. The first cluster was formed by tapeworms from RU-KR and 2 specimens from the human infections from Switzerland (CH-H/1 and CH-H/12) (Fig. 3A). These data imply that the 2 patients from Switzerland probably acquired diphyllobothriosis abroad, although D. latus was circulating in the aquatic environment of the Swiss lakes. The second cluster was formed by D. latus from the Swiss and Italian lakes (IT-IS, IT-MA, IT-CO, CH-GE, CH-BI and CH-NE) and by the majority of adult tapeworms obtained from the humans (CH-H) and fox from Switzerland (CH-F). The tapeworms from ALR did not form separate clusters; in fact, several individuals from Italy overlapped with plerocercoids and the adults from Switzerland. Note that many individuals from ALR are not visible in Fig. 3A as separate points due to the presence of identical multilocus genotypes across all loci.

Fig. 3. Population genetic structure of D. latus individuals (A, C) and populations (B, D) from the ALR and the RU-KR, derived from the PCoA based on the triploid dataset (A, B) and a randomly downsampled dataset of diploids (C, D). See Table 1 for the population codes.
The PCoA based on the populations (Fig. 3B) supported the distinct position of the genetically diverse population from RU-KR. In contrast, the populations from ALR clustered differently than the individuals (Fig. 3A). Populations of D. latus from 3 lakes in Switzerland (CH-NE, CH-GE and CH-BI) formed a separate cluster, and adult tapeworms isolated from the humans and fox from Switzerland (CH-H and CH-F) were genetically related to the Italian samples.
The second dataset, based on 2 alleles randomly downsampled from the 3 alleles in the triploid specimens, provided slightly different results. At the larger scale, the PCoA pattern for individuals supported the distant geographic origin of D. latus from RU-KR and indicated a genetic structure similar to the samples from ALR (Fig. 3C and D). Again, 2 samples from the human infections from Switzerland had affinity to the individuals from Russia (Fig. 3C). The downsampled data at a more detailed scale, however, provided less-informative results on the clustering of individuals and populations (Fig. 3C and D). Dibothriocephalus latus from different subalpine lakes and hosts overlapped and clustered in adjoining assemblages.
Discussion
A polyploid (triploid/aneuploid) population of D. latus from ALR was analysed and its population genetics was compared with a geographically distant population from Siberia in Russia using mitochondrial and microsatellite DNA. Both mtDNA and microsatellite data differed greatly between the allopatric populations and were associated with the differences in ploidy, mode of reproduction, the historical colonization of the Alpine region and the geographic distance. The data and factors contributing to the reconstructed population genetic patterns are discussed below.
Microsatellite allelic patterns and chromosomal ploidy
Microsatellites in tapeworms have been predominantly applied in studies of diploids, such as the caryophyllidean Caryophyllaeus laticeps (2n = 20; Bombarová and Špakulová, Reference Bombarová and Špakulová2015; Králová-Hromadová et al., Reference Králová-Hromadová, Minárik, Bazsalovicsová, Mikulíček, Oravcová, Pálková and Hanzelová2015; Bazsalovicsová et al., Reference Bazsalovicsová, Minárik, Šoltys, Radačovská, Kuhn, Karlsbakk, Skírnisson and Králová-Hromadová2020) and the diphyllobothriideans Ligula intestinalis (2n = 18; Petkevičiūtė and Kuperman, Reference Petkevičiūtė and Kuperman1992; Štefka et al., Reference Štefka, Gilleard, Grillo and Hypša2007, Reference Štefka, Hypša and Scholz2009) and D. dendriticus (2n = 18; Wikgren and Gustafsson, Reference Wikgren and Gustafsson1965; Bazsalovicsová et al., Reference Bazsalovicsová, Minárik, Šoltys, Radačovská, Kuhn, Karlsbakk, Skírnisson and Králová-Hromadová2020). Microsatellites have rarely been used in genetic studies of polyploid organisms. In platyhelminths, STR markers were applied to study the triploid liver flukes of the genus Fasciola (Trematoda; Fasciolidae) from Japan, in which 3 alleles were present in 1 of 12 STR loci in the majority of the flukes (Ohari et al., Reference Ohari, Hayashi, Takashima and Itagaki2021a). Identical genetic types of all STR loci determined in different generations of the species suggested that aspermic triploid Fasciola reproduce clonally by parthenogenesis, and possibly gynogenesis (Ohari et al., Reference Ohari, Matsuo, Yoshida, Nonaka, Sato and Itagaki2021b).
Our study is the first to use STR markers in triploid tapeworms. The results of the microsatellite analysis of the ALR population were in accordance with the previously detected triploidy of D. latus from Iseo lake (Orosová et al., Reference Orosová, Marková, Provazníková, Oros, Radačovská, Čadková and Marec2021); 1 to 2 alleles were observed in 5 of 6 loci analysed in individuals from ALR. Three alleles detected at a particular locus represented a fully heterozygous form and were recognized as 3 distinct peaks in a fragment analysis. The third allele missing in the variant of 2 alleles (2A), or 2 alleles missing in the variant of 1 allele (A1), can be explained by the presence of homozygous forms of allele/alleles within the particular locus, which could not be detected as an individual peak during the fragment analysis.
In contrast to ALR, the standard pattern of 1 and 2 alleles (allelic type A1-2) was detected in all 6 loci in D. latus from fish from RU-KR. The tapeworms from RU-KR have not yet been studied cytogenetically, but a diploid set of chromosomes (2n = 18) would be anticipated. Studies of the basic karyological characteristics and chromosomal architecture of D. latus from RU-KR are needed to verify this assumption.
The allelic type A1-2 was also found in 2 adult D. latus specimens obtained from humans from Switzerland. Detailed medical and travel history and knowledge of the eating habits of patients are necessary for identifying the origin and exact source of infections. Such information was not available to us, but the genetic structure of the tapeworms from the 2 patients from Switzerland implies an allochthonous (imported) infection. One tapeworm with allelic type A1-2 was characterized by the most frequent con-Ha1, typical for ALR, and 1 sample from RU-KR. The second tapeworm with A1-2 possessed a unique con-Ha2, which was placed on the same mutation pathway with the haplotypes specific for RU-KR. These 2 patients were probably not infected by the consumption of raw fish from the local aquatic resources of subalpine lakes but acquired diphyllobothriosis abroad.
All other patients from Switzerland were infected with tapeworms whose genetic structure (allelic type A1-3 and haplotype con-Ha1) was identical to the plerocercoids isolated from fish from the subalpine lakes. This type of infection was evidently local (autochthonous).
Triploidy and parthenogenesis
Chromosome numbers and levels of ploidy in platyhelminths can vary considerably within species and even within individuals (Grey and Mackiewicz, Reference Grey and Mackiewicz1980; Petkevičiūtė, Reference Petkevičiūtė1996; Pongratz et al., Reference Pongratz, Storhas, Carranza and Michiels2003). Hermaphroditic platyhelminths have versatile mechanisms of reproduction; populations with high chromosome numbers tend to reproduce asexually, whereas those possessing low and even ploidy levels (di-, tetra- or hexaploid) reproduce sexually (Lorch et al., Reference Lorch, Zeuss, Brandl and Brändle2016).
Polyploidy is frequently associated with parthenogenesis (Otto and Whitton, Reference Otto and Whitton2000), which can be apomictic (ameiotic), automictic (meiotic) and generative (haploid) (Whitfield and Evans, Reference Whitfield and Evans1983). Polyploidy combined with parthenogenesis offers advantages for plasticity and the evolution of broad ecological tolerance in species (Lorch et al., Reference Lorch, Zeuss, Brandl and Brändle2016).
Until recently, the cytogenetic studies on D. latus were rather scarce. The first data on unusual features of spermatogenesis were documented in the adult tapeworms from Russia and Finland (von Bonsdorff and Telkkä, Reference von Bonsdorff and Telkkä1965; Levron et al., Reference Levron, Brunaňská and Poddubnaya2006). The latest study on chromosomes and meiotic division of D. latus from the ALR was carried out by Orosová et al. (Reference Orosová, Marková, Provazníková, Oros, Radačovská, Čadková and Marec2021) who found some stages of spermatocyte division in the middle part of the strobila. The early stages of developing spermatozoa were also found but fully developed spermatozoa were missing. The nuclear divisions during oogenesis were exclusively mitotic and meiotic figures were observed only very sporadically. Spermatogenesis was abnormal and meiotic figures occurred only very sporadically in the part of strobila which often contained proglottids full of eggs. No mature functional spermatozoa were detected in D. latus from Iseo lake, so parthenogenesis was likely the only possible method of reproduction (Orosová et al., Reference Orosová, Marková, Provazníková, Oros, Radačovská, Čadková and Marec2021).
The currently identified allelic structure of the STR loci corresponded to the specific chromosomal structure, abnormal meiosis and asexual reproduction of D. latus from Iseo lake. First, a low number of observed and effective alleles was detected in tapeworms from all subalpine lakes. Next, the variant of 3 alleles of a particular locus was constant in the vast majority of individuals. In particular, locus DL_6 was displayed by multilocus genetic type 106/109/112 in 96.04% (121 samples) of the individuals from ALR, and no other combination of 3 alleles was observed. Identical genetic types of the STR loci were detected in all loci and the majority of individuals, indicating that the aspermic triploid D. latus from ALR reproduce asexually.
Triploid parthenogens (ALR) vs diploid sexuals (RU-KR)
Parthenogens have better colonizing capacities and wider geographic distributions because they can colonize areas where sexuals have difficulties establishing a population (Lorch et al., Reference Lorch, Zeuss, Brandl and Brändle2016). Ancestral diploid/sexual populations dominate in stable habitats and are in the centre of the distribution of the species, while parthenogens are at the margins of the distributions (Pongratz et al., Reference Pongratz, Storhas, Carranza and Michiels2003).
The latest results on the chromosomal architecture of D. latus from ALR (Orosová et al., Reference Orosová, Marková, Provazníková, Oros, Radačovská, Čadková and Marec2021) and the allelic structure of the STR loci (current results) provided the first and preliminary indication that the diploid/sexually reproducing population from RU-KR was ancestral, located within the centre of the distribution of the species, but the triploid/parthenogenetically reproducing subalpine population seems to be at the margin of the distribution. However, we have to be careful when interpreting the results, since the number of plerocercoids from RU-KR was rather low (n = 18) in comparison with number of plerocercoids from ALR (n = 113). Nevertheless, the outputs based on the microsatellite data were also well supported by the results on the mtDNA of D. latus from RU-KR (11 haplotypes/18 plerocercoids), in strong contrast to the mitochondrial structure of the tapeworms from ALR (1 haplotype/113 plerocercoids).
The mitochondrial diversity displayed by the 11 haplotypes in 18 larvae from RU-KR implied an original and relatively stable population, with some previous changes in the past in population size. In contrast, the identical structure of the mitochondrial haplotypes of all D. latus specimens from ALR indicated relatively ‘recent’ population expansion, supported by the tests of neutrality. Such high homogeneity is a consequence of a bottleneck effect typical for the recent (possibly post-glacial) introduction of the tapeworm to the area, followed by an expansion in range with limited gene flow. The high homogeneity may also be due to a reduction of mitochondrial polymorphism by natural selection and an increased effect of drift in the population of parthenogens.
The ‘recent’ introduction of the Alpine population of D. latus has to be perceived in a broader historical context. The long co-existence of broad fish tapeworm with humans in western Europe was evidenced by palaeo-parasitological analyses of coprolites, cesspit material and soil sediments, which showed its presence in Europe at least since the Bronze age (Gonçalves et al., Reference Gonçalves, Araújo and Ferreira2003; Flammer et al., Reference Flammer, Dellicour, Preston, Rieger, Warren, Tan, Nicholson, Přichystalová, Bleicher, Wahl, Faria, Pybus, Pollard and Smith2018; Ledger et al., Reference Ledger, Grimshaw, Fairey, Whelton, Bull, Ballantyne, Knight and Mitchell2019). In Switzerland, the palaeo-parasitological study documented the presence of diphyllobothriosis caused by D. latus in the lakeside of Lake Chalain since about 3000 BC (Dommelier et al., Reference Dommelier, Bentrad, Paicheler, Pétrequin and Bouchet1998). The first recognizable description of D. latus in the ALR was reported more than 430 years ago by the 2 Swiss physicians (Thaddeus Dunus in Lucarno and Gaspard Wolphius in Zürich) who published data on human diphyllobothriosis at least since 1592 (Grove, Reference Grove1990).
In Eurasia, the last glacial period resulted in the modification of river systems and creation of millions of lakes as the glacier retreated (Pongratz et al., Reference Pongratz, Storhas, Carranza and Michiels2003). Fish parasites moved along with their fish hosts from Siberia to Europe during interglacial period (11 700 years ago until now) (Rumyantsev, Reference Rumyantsev1984). Introduction of D. latus from Siberia to Europe could be linked with the postglacial expansion of European perch, in which specific colonization routes depended on the retreated glacier (Nesbø et al., Reference Nesbø, Fossheim, Vøllestad and Jakobsen1999). As the Alps presented solid ice fields and montane glaciers (Spötl et al., Reference Spötl, Koltai, Jarosch and Cheng2021), present-day populations of D. latus in this region must be descendants which persisted in the refugia. The dispersal history and introductions of D. latus to the distant regions was sustained by large-scale migrations of humans throughout history (Katkar, Reference Katkar2011; Haak et al., Reference Haak, Lazaridis, Patterson, Rohland, Mallick, Llamas, Brandt, Nordenfelt, Harney, Stewardson, Fu, Mittnik, Bánffy, Economou, Francken, Friederich, Pena, Hallgren, Khartanovich, Khokhlov, Kunst, Kuznetsov, Meller, Mochalov, Moiseyev, Nicklisch, Pichler, Risch, Rojo Guerra, Roth, Szécsényi-Nagy, Wahl, Meyer, Krause, Brown, Anthony, Cooper, Ait and Reich2015).
The detection of identical haplotypes in all plerocercoids from ALR suggests the existence of only 1 population, even though the Italian lakes and lake Geneva (Neuchâtel and Biel lakes are connected by the Thielle channel) represent isolated biotopes with no aquatic connections. Humans, however, represent the most important hosts of D. latus and have contributed substantially to the spread and maintenance of diphyllobothriosis in the subalpine environment, providing an efficient means of gene flow between the populations.
Factors maintaining the unique genetic structure of the ALR population
The specific molecular and genetic structure of D. latus from ALR has likely been maintained by several biological and environmental factors. In particular, the number of adult tapeworms in the intestine of a definitive host is the crucial biological factor influencing the reproduction of the tapeworm. Dibothriocephalus latus in ALR has usually been found as a single plerocercoid in the musculature of the most common second intermediate fish host, the European perch (Gustinelli et al., Reference Gustinelli, Menconi, Prearo, Caffara, Righetti, Scanzio, Raglio and Fioravanti2016; Radačovská et al., Reference Radačovská, Bazsalovicsová, Blasco Costa, Orosová, Gustinelli and Králová-Hromadová2019a). Humans acquire diphyllobothriosis by consuming raw or insufficiently cooked fish products, including marinated or smoked fish-harbouring plerocercoids. Infection is thus attributable to particular geographic regions with local dietary habits and traditions of consuming raw fish.
ALR is specific in this aspect, because the consumption of raw fish is more occasional than regular in this part of Europe. The increased risk of diphyllobothriosis for consumers in the subalpine territory is mainly due to the popularity of local food specialities, such as perch carpaccio, and extreme food choices (e.g. raw-food diet) (Wicht, Reference Wicht2008). Humans consequently usually acquire diphyllobothriosis only sporadically by the consumption of infected perch fillets, which mainly harbour only 1 plerocercoid in the musculature, developing into a single adult in the definitive host.
A different situation has been documented in Russia and Estonia, historically amongst the oldest foci of diphyllobothriosis in Europe. Patients harbouring up to 11 tapeworms (Kondrateva, Reference Kondrateva1961; Rossolovskaya, Reference Rossolovskaya, Prokopenko, Semenova, Pov, Leikina, Khodakova and VI1968), or even as many as 106 D. latus specimens (Grant, Reference Grant1930), were diagnosed in these countries. A high intensity of infection can be explained by the historic eating habits of consuming raw fillets of fish and/or pike caviar on a regular basis. Pike is a paratenic host of D. latus that may harbour numerous plerocercoids in the musculature, but also on internal organs, including ovaries (for a review, see Králová-Hromadová et al., Reference Králová-Hromadová, Radačovská, Čisovská Bazsalovicsová and Kuchta2021). Consumption of infected pike caviar may thus lead to multiple D. latus infections, which is a good prerequisite of sexual reproduction of tapeworms. Indeed, the statistical analysis of STR loci with 1 or 2 alleles implied a sexually reproducing panmictic population in RU-KR. Sexual reproduction was also supported by the analysis of genotype diversity with a random segregation of alleles.
Ecological stress may be another factor contributing to the specific genetic composition of the ALR population. The probability of errors during meiosis and mitosis can increase under severe environmental conditions (Lorch et al., Reference Lorch, Zeuss, Brandl and Brändle2016). The subalpine lakes belong to anthropized water systems affected by intensive industrial, agricultural and urban activities (Lang, Reference Lang1989; Anneville et al., Reference Anneville, Molinero, Souissi, Balvay and Gerdeaux2007; Manca et al., Reference Manca, Torretta, Comoli, Amsinck and Jeppesen2007; Menconi et al., Reference Menconi, Zoppi, Pastorino, Di Blasio, Tedeschi, Pizzul, Mugetti, Tomasoni, Dondo and Prearo2021). It could contribute to spontaneous polyploidization and aneuploidy and to changes in reproductive strategy, which are common in harsh environments and bottlenecked populations (Lorch et al., Reference Lorch, Zeuss, Brandl and Brändle2016).
Restricted distribution in Europe vs ancestral range
ALR and RU-KR represent only a fragment of the Eurasian distribution of D. latus. The logical questions would be, what is the genetic make-up of other populations of the tapeworm and where is the boundary between ‘ancestral diploids’ and ‘recent triploids’? These questions, no matter how interesting they are, are very difficult to answer, because the broad fish tapeworm represents a very specific human parasite in its past and present occurrence.
Several foci of diphyllobothriosis have developed in Europe: (1) Fennoscandia, (2) the Baltic region, (3) ALR, (4) the Danube river region and (5) Russia (for a review, see Králová-Hromadová et al., Reference Králová-Hromadová, Radačovská, Čisovská Bazsalovicsová and Kuchta2021). Large-scale monitoring, massive health campaigns, implementation of preventive measures and effective treatment, however, led to a substantial decrease in the numbers of infections in Fennoscandia, the Baltic region and the Danube river region in the last decades of the 20th century. Consequently, collecting D. latus from its historically important endemic regions is rather difficult. Indeed, all our attempts to enlarge the D. latus dataset for the current study were unsuccessful. We have genotyped adult diphyllobothriid tapeworms from a grey wolf (Canis lupus) and a Eurasian lynx (Lynx lynx) from Latvia (Bagrade et al., Reference Bagrade, Králová-Hromadová, Bazsalovicsová, Radačovská and Kołodziej-Sobocińska2021) and from lynx from Finland (Čisovská Bazsalovicsová et al., Reference Čisovská Bazsalovicsová, Radačovská, Lavikainen, Kuchta and Králová-Hromadová2022). All tapeworms from both regions, however, were another diphyllobothriid, Spirometra erinaceieuropaei, the causative agent of sparganosis. The broad fish tapeworm was described from the further Baltic country, Poland, where eggs of D. latus were morphologically detected from European otter (Lutra lutra), wolf and lynx from Białowieża Primeval forest (Górski et al., Reference Górski, Zalewski and Łakomy2006, Reference Górski, Zalewski, Kazimierczak and Kotomski2010; Szczęsna et al., Reference Szczęsna, Popiołek, Schmidt and Kowalczyk2008) and D. latus plerocercoids were found in European perch from Pomeranian Bay in the southwestern part of the Baltic Sea (Bielat et al., Reference Bielat, Legierko and Sobecka2015). Dibothriocephalus latus, however, was not found during our ichthyoparasitological examinations of perch and pike from Pomeranian Bay and Białowieża Primeval forest (Radačovská et al., Reference Radačovská, Bazsalovicsová, Linowska, Kołodziej-Sobocińska and Králová-Hromadová2019c). The eggs detected in otter, lynx and wolf (Górski et al., Reference Górski, Zalewski and Łakomy2006, Reference Górski, Zalewski, Kazimierczak and Kotomski2010; Szczęsna et al., Reference Szczęsna, Popiołek, Schmidt and Kowalczyk2008) likely belonged to the recently intensively studied S. erinaceieuropaei in northeastern Poland (Kondzior et al., Reference Kondzior, Kowalczyk, Tokarska, Borowik, Zalewski and Kołodziej-Sobocińska2020). Finally, the examination of 700 European perch from the Slovak part of the Danube river also did not reveal any D. latus (Radačovská et al., Reference Radačovská, Bazsalovicsová and Králová-Hromadová2019b).
The only comparable molecular and genetic data on D. latus from localities other than ALR and RU-KR are from Finland. The number of chromosomes (15, 16, 17, 18, 20, 24 and 28) in somatic cells, with 18 chromosomes the most frequent, varied considerably in D. latus from Finland (Wikgren and Gustafsson, Reference Wikgren and Gustafsson1965). These authors concluded that D. latus was a diploid (2n = 18) species with a relatively large variation in the number of chromosomes. We analysed STR loci and mitochondrial haplotypes in 2 adult D. latus obtained from patients from Finland without any details on travel history and the origin of the samples (data not shown). The allelic type A1-3, specific for D. latus from ALR, was observed in both tapeworms. One specimen from Finland was in addition characterized by the mitochondrial concatenated haplotype con-Ha1, thus having the identical genetic structure with the tapeworms from ALR. The patient either acquired diphyllobothriosis in the Alpine region, or the infection was local indicating common genetic structure of populations from Finland and the Alpine lakes. The second tapeworm possessed unique con-Ha13, which was either specific for Finland, or introduced from abroad. The allelic type A1-3 of the tapeworms from Finland provided very preliminary evidence that triploidy and the specific genetic structure of D. latus may be a feature characteristic for more European populations of the parasite.
Many European populations of D. latus are either extinct or extremely difficult to sample, so the continuous geographic lineage of D. latus cannot be examined. The only promising territory for further collection and a detailed investigation of D. latus is Russia, where the tapeworms have been found in several European and Asian regions (Rumyantsev, Reference Rumyantsev2007; Dugarov and Pronin, Reference Dugarov and Pronin2017; Chugunova et al., Reference Chugunova, Ronzhina and Syromyatnikov2020).
The unique genetic structure of D. latus from the subalpine territory indicated a successful adaptation of the parasite introduced to novel and extreme habitats, which followed a bottleneck induced by colonization. More explicit assessments of genetic diversity, metapopulation dynamics, demographic changes and migratory routes of D. latus require additional genetic data based on a larger sample set. Further studies would probably identify a broader genetic diversity of D. latus and would hopefully specify the areas of distribution and boundaries between ‘ancestral diploids’ and ‘recent triploids’.
Supplementary material
The supplementary material for this article can be found at https://doi.org/10.1017/S0031182022000634
Acknowledgements
The authors thank Dr Barbara Wicht, Laboratory of Applied Microbiology, DECD, SUPSI, Bellinzona, Switzerland, and Dr Roman Kuchta and Professor Tomáš Scholz, Institute of Parasitology, Biology Centre, Czech Academy of Sciences, České Budějovice, Czech Republic for providing the material of adult tapeworms from their helminthological collections.
Author contributions
A. R., E. Č. B. and I. K.-H. conceived the study, performed molecular analyses and wrote the manuscript. A. R., E. Č. B., I. K.-H., A. G. and J. K. C. collected the parasitic material. K. Š. and G. M. performed fragment analysis and sequencing. J. Š., A. R. and E. Č. B. performed statistical analyses. All authors discussed the results, revised the manuscript and approved its final version.
Financial support
This work was financially supported by the Slovak Research and Development Agency under contract APVV-15-0004 (I. K.-H.), Slovak Grant Agency VEGA 2/0027/21 (I. K.-H.) and Bilateral Mobility Project SAS-ASCR 21-11 (E. Č. B. and J. Š.).
Conflict of interest
The authors declare there are no conflicts of interest.
Ethical standards
There is no ethical issue involved in this study.