Introduction
Schistosomes (Digenea: Schistosomatidae) are blood fluke parasites from birds and mammals (Khalil, Reference Khalil, Gibson, Jones and Bray2002). Several of the species in this family are well known for causing schistosomiasis, a disease that causes morbidity and mortality in humans (WHO, 2021) as well as known around the world for causing human cercarial dermatitis (HCD) or swimmer's itch (see Horák et al., Reference Horák, Mikeš, Lichtenbergová, Skála, Soldánová and Brant2015).
Gigantobilharzia Odhner, Reference Odhner1910 is one of the ten genera of avian schistosomes (Lashaki et al., Reference Lashaki, Teshnizi, Gholami, Fakhar, Brant and Dodangeh2020). It was erected to include Gigantobilharzia acotylea Odhner, Reference Odhner1910, a parasite in the intestinal veins of the gull Larus fuscus L. (Laridae) from the west coast of Sweden; the genus name refers to the long dimension of the body (Odhner, Reference Odhner1910).
Currently, the genus Gigantobilharzia includes 18 species worldwide that parasitize several orders of birds as definitive hosts, and families of gastropods as intermediate hosts from both freshwater and marine environments (see Table 1). Since the genus was established, most of the species descriptions were based on few (often single) and/or incomplete worms. Descriptions based on so few worms is a common problem, particularly in avian schistosome taxonomy, because the adult worms have long, thin and delicate bodies making them difficult to find and remove from host blood vessels. This difficulty led to several species assignments into Gigantobilharzia and over time little attention was paid to the original generic diagnosis relative to what was being described as new. Therefore, several different combinations of characters [e.g. the presence or absence of oral sucker, the presence or absence of gynaecophoric canal with different lengths and with or without transversal bands (TBs) in males, see Table 1] were amended to the generic diagnosis instead of erecting new genera, diluting the ability to diagnose and capture the diversity of the family. There is a wide variation not only in morphology/morphological combinations, but also in the diversity in hosts (avian and gastropod) and habitats (marine and freshwater) among the species. This can indicate they are not congeners, at least for this family known for its specificity for gastropod host species. Correspondingly, based on morphology and genetic data, several authors have claimed that Gigantobilharzia is not a monophyletic group and should be revised and most species reassigned to new genera (Brackett, Reference Brackett1942; Farley, Reference Farley1971; Khalifa, Reference Khalifa1974; Brant et al., Reference Brant, Cohen, James, Hui, Hom and Loker2010; Schuster et al., Reference Schuster, Aldhoun and O'Donovan2014; Aldhoun and Horne, Reference Aldhoun and Horne2015; Sweazea et al., Reference Sweazea, Simperova, Juan, Gadau, Brant, Deviche and Jarrett2015; Pinto et al., Reference Pinto, Pulido-Murillo, de Melo and Brant2017).
Table 1 (Part a). Features and measurements of schistosome species described in the genus Gigantobilharzia, their localities, site of infections and hosts; accommodated in Groups A and B by the main features in an attempt to classify them by combinations of morphological characters.
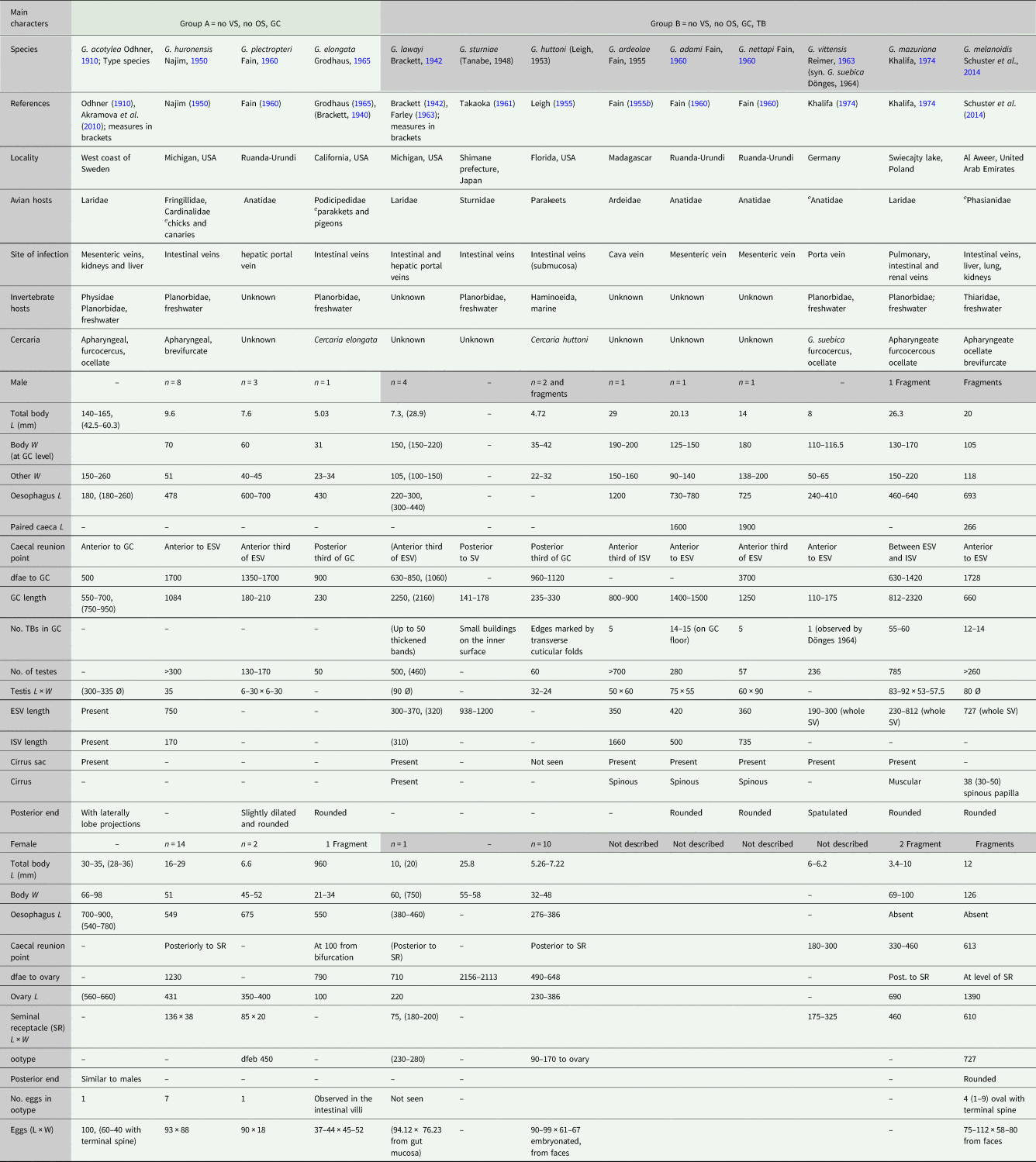
VS, ventral sucker; OS, oral sucker; GC, gynaecophoric canal; TB, transversal bands in the gynaecophoric canal; e, experimental host; L, length; W, width; dfae, distance from anterior end; dfeb, distance from oesophagus bifurcation; Ø, diameter; ESV, external seminal vesicle; ISV, internal seminal vesicle; SR, seminal receptacle.
In the last decade, taxonomists have suggested that delimiting species using solely morphology may be imprecise (Gagnon et al., Reference Gagnon, Kasl, Preisser, Belden and Detwiler2021), and increasingly encouraged an integrative paradigm (i.e. using all available data such as morphological and molecular data, host use and geographic distribution) for detecting and delimiting genera and species, and placing them within a phylogenetic context (e.g. Brower, Reference Brower2010; Cremonte et al., Reference Cremonte, Gilardoni, Pina, Rodrigues and Ituarte2015; Blasco-Costa et al., Reference Blasco-Costa, Cutmore, Miller and Nolan2016; Renner, Reference Renner2016, Bray et al., Reference Bray, Cutmore and Cribb2021; Gagnon et al., Reference Gagnon, Kasl, Preisser, Belden and Detwiler2021; Gonchar and Galaktionov, Reference Gonchar and Galaktionov2021). Such efforts have increased the identification of trematode species, or at least distinct lineages, when several of them could not be differentiated morphologically (e.g. Detwiler et al., Reference Detwiler, Bos and Minchella2010; Locke et al., Reference Locke, Mclaughlin and Marcogliese2010; Georgieva et al., Reference Georgieva, Selbach, Faltýnková, Soldánová, Sures, Skírnisson and Kostadinova2013; McNamara et al., Reference McNamara, Miller and Cribb2014; Gilardoni et al., Reference Gilardoni, Etchegoin, Cribb, Pina, Rodrigues, Diez and Cremonte2020). Furthermore, the inclusion of genetic data has increased that rate at which we can discover life cycles by connecting them with adult worms (Blasco-Costa et al., Reference Blasco-Costa, Cutmore, Miller and Nolan2016).
Table 1 (Part b). Features and measurements of schistosome species described in the genus Gigantobilharzia, their localities, site of infections, and hosts; accommodated in Groups C to F by the main features in an attempt to classify them by combinations of morphological characters.

VS, ventral sucker; OS, oral sucker; GC, gynaecophoric canal; TB, transversal bands in the gynaecophoric canal; e, experimental host; L, length; W, width; dfae, distance from anterior end; dfeb, distance from oesophagus bifurcation; Ø, diameter; ESV, external seminal vesicle; ISV, internal seminal vesicle; SR, seminal receptacle.
Several authors argue that the focus for identification should be on diverse diagnosable features, which include genetic characters, that are informative in phylogenies for capturing species diversity and aid in erecting new families and genera (Brant et al., Reference Brant, Morgan, Mkoji, Snyder, Rajapakse and Loker2006; Brower, Reference Brower2010; Stentiford et al., Reference Stentiford, Bateman, Feis, Chambers and Stone2013; Félix et al., Reference Félix, Braendle and Cutter2014; Blasco-Costa et al., Reference Blasco-Costa, Cutmore, Miller and Nolan2016; Renner, Reference Renner2016; Gagnon et al., Reference Gagnon, Kasl, Preisser, Belden and Detwiler2021). Genetic characterizations have been shown to be a reasonable proxy for formulating a hypothesis of what might constitute a species. Furthermore, the molecular phylogenetic trees provide excellent frameworks within which to ascertain other types of variation, including additional morphology, pathology and host use. However, even if formal descriptions are not possible, it is still valuable to add as much of the morphology and host data as is possible (e.g. Brant et al., Reference Brant, Morgan, Mkoji, Snyder, Rajapakse and Loker2006; Cremonte et al., Reference Cremonte, Pina, Gilardoni, Rodrigues, Chai and Ituarte2013; Blasco-Costa et al., Reference Blasco-Costa, Cutmore, Miller and Nolan2016; Ebbs et al., Reference Ebbs, Loker, Davis, Flores, Veleizan and Brant2016; Laidemitt et al., Reference Laidemitt, Brant, Mutuku, Mkoji and Loker2019; Gilardoni et al., Reference Gilardoni, Etchegoin, Cribb, Pina, Rodrigues, Diez and Cremonte2020), as well as invaluable to voucher both the parasites and the hosts (Hoberg, Reference Hoberg2002; Turney et al., Reference Turney, Cameron, Cloutier and Buddle2015; Ebbs et al., Reference Ebbs, Loker, Davis, Flores, Veleizan and Brant2016; Haas et al., Reference Haas, Hoberg, Cook, Henttonen, Makarikov, Gallagher, Dokuchaev and Galbreath2020; Thompson et al., Reference Thompson, Phelps, Allard, Cook, Dunnum, Ferguson, Gelang, Khan, Paul, Reeder, Simmons, Vanhove, Webala, Weksler and Kilpatrick2021).
The type species for Gigantobilharzia is G. acotylea. According to Brackett (Reference Brackett1942), the generic diagnosis was inadequate. This species has recently been redescribed by Akramova et al. (Reference Akramova, Azimov and Shakarboev2010) that included the description of the larval stages and the experimental host involved in its freshwater life cycle. However, despite the detailed study, it did not resolve the taxonomic problem of diagnosis, there were a few questions: (a) it is not clear how they justified it was G. acotylea (e.g. the tail of the male type species is different from theirs, which looks like more of the other species of Gigantobilharzia reported from Eurasia), (b) measurements not compared to other species of Gigantobilharzia, (c) they obtained cercariae from two different families (Anisus spirorbis and Physa fontinalis) of experimentally exposed snails indicating the possibility that they had two species of schistosomes or a rare case in Schistosomatidae where one species uses multiple families of snails. This is a case where genetic characterization of the larval and adult stages would be useful to know that you have the same species, as co-infections are not uncommon and multiple birds were used a source material and (d) regrettably, the authors did not voucher any of their material, and so the specimens cannot be reexamined (Turney et al., Reference Turney, Cameron, Cloutier and Buddle2015) to verify the identity of the species. Thus, we could not compare genetic data to that study, but our specimens do not fit the morphology of G. acotylea.
There are a few species of Gigantobilharzia that have nucleotide data for additional comparisons. Currently, Gigantobilharzia huronensis Najim, Reference Najim1950 and Gigantobilharzia melanoidis Schuster, Aldhoun and Donovan, Reference Schuster, Aldhoun and O'Donovan2014 are the only nominal species of the genus both with adults morphologically and genetically described. Additionally, molecular data, and some morphology, of Gigantobilharzia vittensis Reimer, Reference Reimer1963 obtained from cercariae and eggs are available (Aldhoun et al., Reference Aldhoun, Podhorský, Holická and Horák2012; Pinto et al., Reference Pinto, Pulido-Murillo, de Melo and Brant2017). Furthermore, there are three species in the GenBank database that were not morphologically described but it was suggested they should be placed in Gigantobilharzia. The first one is from the African penguin, Spheniscus demersus from South Africa (Aldhoun and Horne, Reference Aldhoun and Horne2015), the second one is from a kelp gull, Larus dominicanus, from Argentina (Brant et al., Reference Brant, Loker, Casalins and Flores2017), and the last one is from a Magellanic penguin, Spheniscus magellanicus, from the Rio Grande estuary in Brazil (Vanstreels et al., Reference Vanstreels, Gardiner, Yabsley, Swanepoel, Kolesnikovas, Silva-Filho, Ewbank and Catão-Dias2018).
Under this context and as part of the results of a survey of gull parasites in Argentina, we describe below using morphological and nucleotide data two new genera and species of schistosomes parasitizing larids in two different coastal environments from Argentina. Morphological characters defining species of Gigantobilharzia were reviewed in order to identify the combination of morphological characters that do not correspond to those described for the genus or the type species. A summary of this review can help guide taxonomists in the future as well as allow us to assign the two new species and suggest new genera (Table 1). Hopefully, this effort is one step to alleviating the taxonomic problem of having such an inclusive generic diagnosis.
Materials and methods
Collection and morphological study of specimens
Different gull species were sampled during autumn, winter and spring between 2016 and 2018 in two localities from Argentina, South America: the Municipal Bowls of Puerto Madryn (42°76′ S–65°03′ W) where fish discards are deposited, on the north Patagonian coast, Chubut province, Southwestern Atlantic Ocean, and CEAMSE, Ensenada's sanitary landfill (34°51′ S–57°57′ W) where urban rubbish is treated, located in the northeast of Buenos Aires province, on the coast of the Río de La Plata (Fig. 1). Forty kelp gulls L. dominicanus and five brown-hooded gulls, Chroicocephalus maculipennis (Lichtenstein) were shot in the Municipal Bowls, whereas 30 L. dominicanus, 38 C. maculipennis and seven grey-hooded gulls, Chroicocephalus cirrocephalus (Vieillot) were shot in the CEAMSE. In the laboratory, birds were necropsied and their intestines and mesenteric veins were examined under a stereoscopic microscope for schistosomes. The schistosomes were found by looking for the presence of dark pigment in their caeca and live specimens were collected using fine needles and forceps. Worms obtained were transferred onto slides with saline solution, stained with neutral red dye and covered with coverslips and observed live for a better interpretation of their reproductive structures under an Olympus BX51® microscope (Olympus, Tokyo, Japan) and Leica DCM 2500® (Leica, Wetzlar, Germany). Some specimens were fixed in 10% formalin and preserved in 70% ethanol, stained with alum or hydrochloric carmine, dehydrated in a graded ethanol series, cleared in methyl salicylate and mounted in Canada balsam. Drawings were prepared using a lucid camera and photographs were obtained by using digital cameras (Olympus BX51® and Leica DMC 280®), both connected to the microscopes. In addition, two specimens from the Municipal Bowls were dried using the critical point method for scanning electron microscopy (SEM) (Jeol 6360LV®, JEOL, Tokyo, Japan) examination for fine features and photographs were obtained. Several worm fragments from each host were fixed in 100% ethanol for molecular analyses. The prevalence (P) was calculated according to Bush et al. (Reference Bush, Lafferty, Lotz and Shostak1997). Furthermore, cercariae from Siphonaria lessonii (Siphonariidae) and some adult specimens of schistosomes from L. dominicanus, collected in the estuary of the Deseado river (47°45′ S, 65°55′ W), at Puerto Deseado, Santa Cruz province, Argentina, Southwestern Atlantic Ocean (Fig. 1) by Gilardoni et al. (Reference Gilardoni, Di Giorgio, Bagnato and Cremonte2019) and Reference Gilardoni, Lorenti, Diaz, Leonardi and CremonteGilardoni et al. (in press), respectively, were used for the molecular analysis.
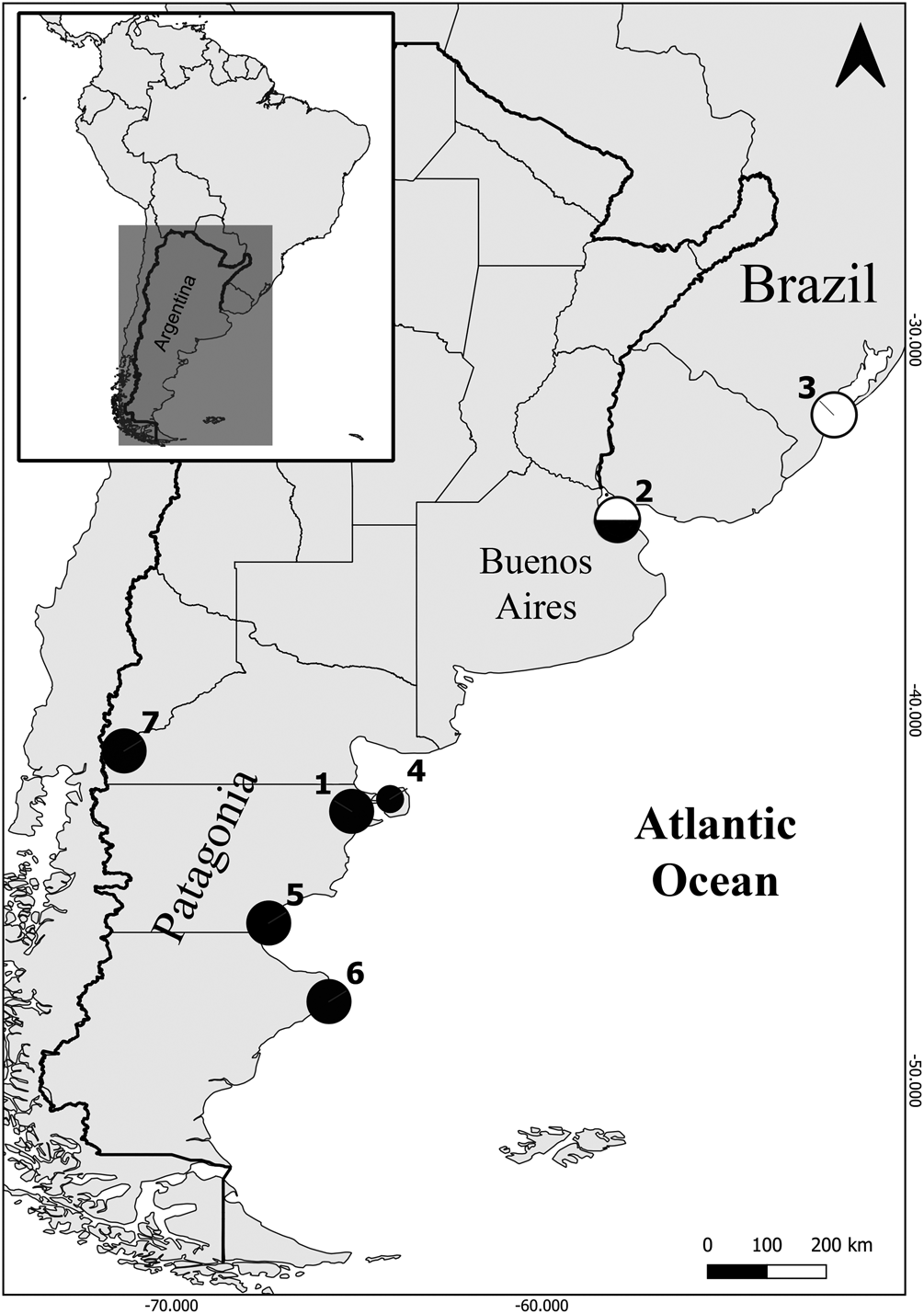
Fig. 1. Map showing the localities where Marinabilharzia patagonense n. g., n. sp. (black circles) and Riverabilharzia ensenadense n. g., n. sp. (white circles) where found in South America. (1) Puerto Madryn, Chubut (adults, current study); (2) Ensenada, Buenos Aires (adults, current study); (3) Rio Grande, Brazil (adults, Vanstreels et al., Reference Vanstreels, Gardiner, Yabsley, Swanepoel, Kolesnikovas, Silva-Filho, Ewbank and Catão-Dias2018); (4) Fracasso Beach, Chubut (cercaria, Bagnato et al., Reference Bagnato, Gilardoni, Di Giorgio and Cremonte2015); (5) Caleta Córdova and Comodoro Rivadavia, Chubut (cercaria, Alda and Martorelli, Reference Alda and Martorelli2009; Brant et al., Reference Brant, Loker, Casalins and Flores2017); (6) Puerto Deseado, Santa Cruz (cercaria and adults, Gilardoni et al., Reference Gilardoni, Di Giorgio, Bagnato and Cremonte2019, Reference Gilardoni, Lorenti, Diaz, Leonardi and Cremontein press); (7) National Nahuel Huapi Park, Rio Negro (adult fragment, Brant et al., Reference Brant, Loker, Casalins and Flores2017). Note that both species were found in Ensenada, Buenos Aires province.
The studied specimens were deposited in the Parasitological Collection (CNP-Par) of IBIOMAR (CCT CONICET-CENPAT) in Puerto Madryn, in the Museo de La Plata (MLP), La Plata, Argentina, and in the Museum of Southwestern Biology Division of Parasites, University of New Mexico (MSB:Para), Albuquerque, New Mexico, USA.
Bird scientific names were used following Birds of the Word, The Cornell Lab of Ornithology (www.birdsoftheworld.org).
Sequencing data and phylogenetic analysis
DNA was extracted from ten adult worm fragments with the QIAamp DNA micro kit (Qiagen, Valencia, California) according to the manufacturer's guidelines, except that samples were eluted with 30 μL of buffer to increase DNA concentration. The extracted DNA was amplified by polymerase chain reaction (PCR) (TaKara Ex Taq kit, Takara Biomedicals, Otsu, Japan) and sequenced with previously published primers [28S nDNA region (U178 and L1642), ITS1-5.8S-ITS2 nDNA region (BDF1, BDR2, 3S and 4S) and mtDNA region cox1 (Cox1_Schisto_5 and Cox1_Schisto_3); for details on primers, see Bowles and McManus (Reference Bowles and McManus1993), Bowles et al. (Reference Bowles, Blair and McManus1995), Lockyer et al. (Reference Lockyer, Olson, Østergaard, Rollinson, Johnston, Attwood, Southgate, Horák, Snyder, Le, Agatsuma, Mcmanus, Carmichael, Naem and Littlewood2003a), Brant et al. (Reference Brant, Morgan, Mkoji, Snyder, Rajapakse and Loker2006) and Brant and Loker (Reference Brant and Loker2009). The PCR products were purified with the E.Z.N.A. Cycle Pure Kit (Omega Bio-Tek, Norcross, GA, USA) and sequenced using the Applied Biosystems BigDye direct sequencing kit, version 3.1 (Applied Biosystems, Foster City, CA, USA). Chromatograms were edited in Sequencher v 5.0 (Gene Codes Corporation, Ann Arbor, MI, USA) and sequences were aligned by eye in Se-Al v 2.0a11 (http://tree.bio.ed.ac.uk). Phylogenetic analyses of the parasite nuclear 28S, ITS and mitochondrial cox1 datasets were carried out using Bayesian inference in MrBayes (Huelsenbeck and Ronquist, Reference Huelsenbeck and Ronquist2001) with default priors for 28S and ITS1-5.8S-ITS2 (Nst = 6, rates = gamma, ngammacat = 4) and cox1 (parameters un-linked so each partition by codon has its own set of parameters; Nst = 6 rates-invgamma). Partitions by codon evolved under different rates [preset applyto = (all) ratepr = variable]. Model selection was estimated using ModelTest (Posada and Crandall, Reference Posada and Crandall1998). Four chains were run simultaneously for 3 × 105 generations; the first 3000 trees with preasymptotic likelihood scores were discarded as burn-in, and the remaining trees were used to generate 50% majority-rule consensus trees and posterior probabilities. Outgroups used have been defined in previous analyses (see Brant and Loker, Reference Brant and Loker2013). The new sequences generated were deposited in GenBank (see accession numbers in Table 2).
Table 2. List of taxa included in the phylogenetic analyses, GenBank accession numbers and references

Results
Two new schistosome genera and species are morphologically and molecularly described below. Measurements from specimens in permanent mounts are given in micrometres unless otherwise indicated; the mean is followed by the range in parentheses.
Marinabilharzia n. g.
Diagnosis
Schistosomatidae. Thin filiform worms, entire body surface covered by striations. Several papillae distributed mainly around the mouth opening and extended posteriorly some distance. Sexes similar in shape, females slenderer. Body cylindrical, anterior end rounded in both sexes, posterior rounded end in males and often spatulated in females. Mouth subterminal. Oral and ventral suckers absent. Female genital pore at anterior end of body. Erection of this new genus is proposed based upon the morphological combinations of characters relative to other species as well as genetic characterization of the specimens found and described in this study, and also of a worm fragment recovered from a South African penguin (KM023789). Marinabilharzia can be differentiated from all other known genera within the family by lack of oral and ventral sucker, short gynaecophoric canal, oesophagus bifurcation and caecal reunion near anterior end, absence of intestinal diverticula (further morphological discussion detailed below), as well as comparison of partial sequence data for the nuclear 28S and mitochondrial cox1 gene; these specimens form a clade and do not group within any of the named genera in the tree that also have morphological justifications, nor any of the unnamed lineages.
Etymology: The genus name refers to the ‘marine’ environment where it was first discovered.
Type species: Marinabilharzia patagonense n. g., n. sp.
Marinabilharzia patagonense n. g., n. sp.
Description
Male (measurements based on nine stained specimens, Figs 2–4): Body filiform 21.93 (13.45–31.40) mm long (n = 6), almost uniform width, 169 (128–190) at oesophagus bifurcation level, widest 268 (215–320) at gynaecophoric canal level and 138 (83–195) (n = 8) at testicular field. Oesophagus 533 (395–750) (n = 9) long, bifurcating to short paired caeca 520 (420–700) (n = 3), joining mid-level of cirrus sac to form unique caecum. Common caecum running zigzag inside gynaecophoric canal until near end of body. Gynaecophoric canal 1056 (650–1590) long (n = 8), starting at 1255 (1020–1500) (n = 8) from anterior end. Transversal bands absent. A delicate superficial lateral junction observed at end of gynaecophoric canal (Fig. 4A). Testicular field 20.48 (11.06–30.06) mm in length (n = 5), starting immediately end of gynaecophoric canal or some distance 65 (0–170) (n = 5) from its end; testes rounded to oval, 60 (53–69) long by 68 (49–89) wide (n = 6), 286 (185–387) (n = 2) in number, smaller at start of testicular field and enlarging posteriorly. Vas deferens leads forward from testes, bends posteriorly and forms seminal vesicle elongated and sinuous. External seminal vesicle starting at 172 (110–225) (n = 8) from oesophagus bifurcation, 280 (170–370) long by 67 (48–110) wide (n = 8); internal seminal vesicle enclosed in cirrus sac and surrounded by prostatic cells. Cirrus sac 319 (225–460) long by 80 (50–125) wide (n = 8), ending in genital pore located in papilla; ejaculatory duct 63 (42–95) (n = 7) long connects to spinous cirrus 47 (42–52) in diameter, located at beginning of gynaecophoric canal. Posterior end of body rounded without protrusions.
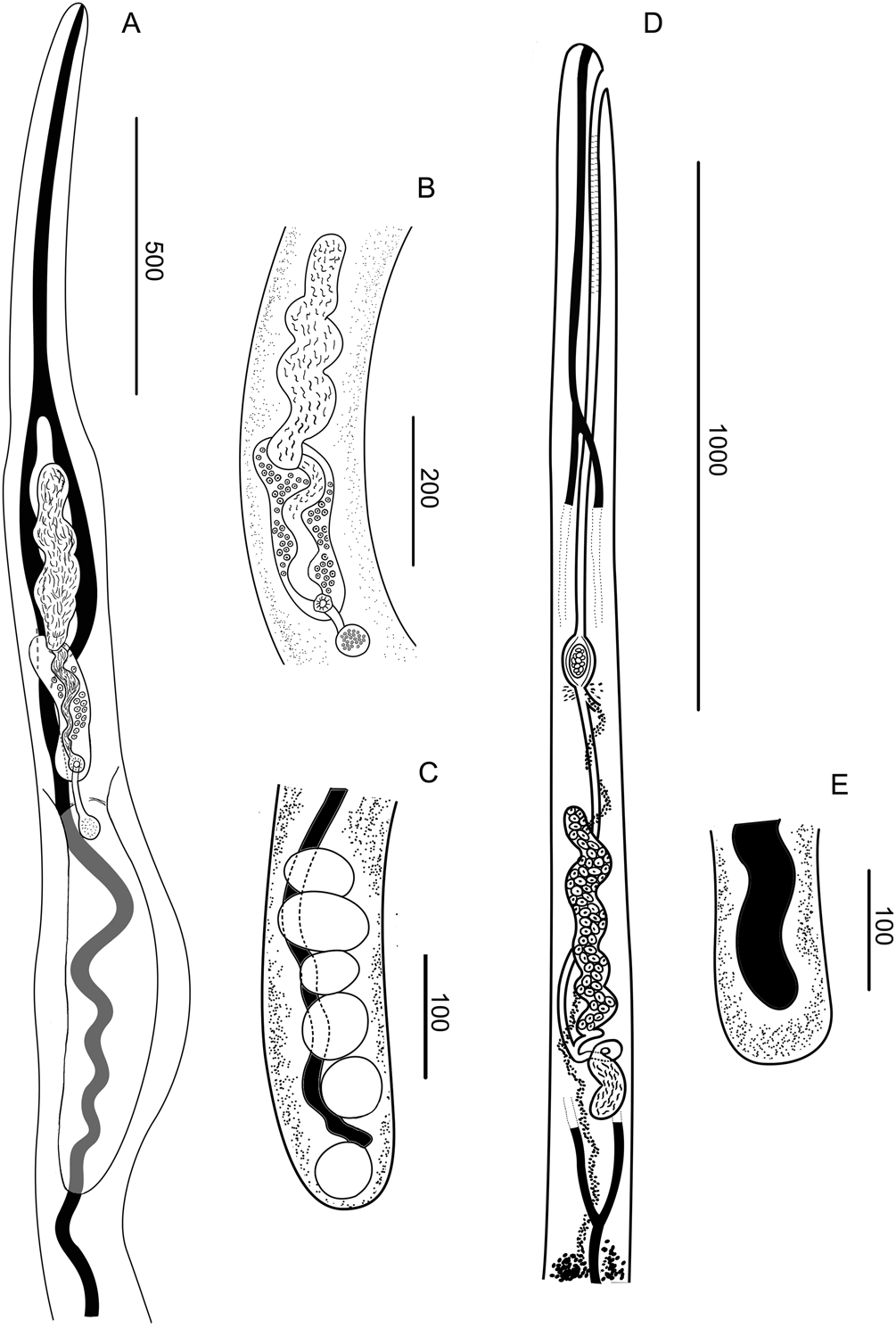
Fig. 2. Drawings of M. patagonense n. g., n. sp. from Argentina. (A–C) Male. (A) Anterior end. (B) Detail of the terminal genitalia. (C) Posterior end. (D and E). Female. (D) Anterior end. (E) Posterior end.
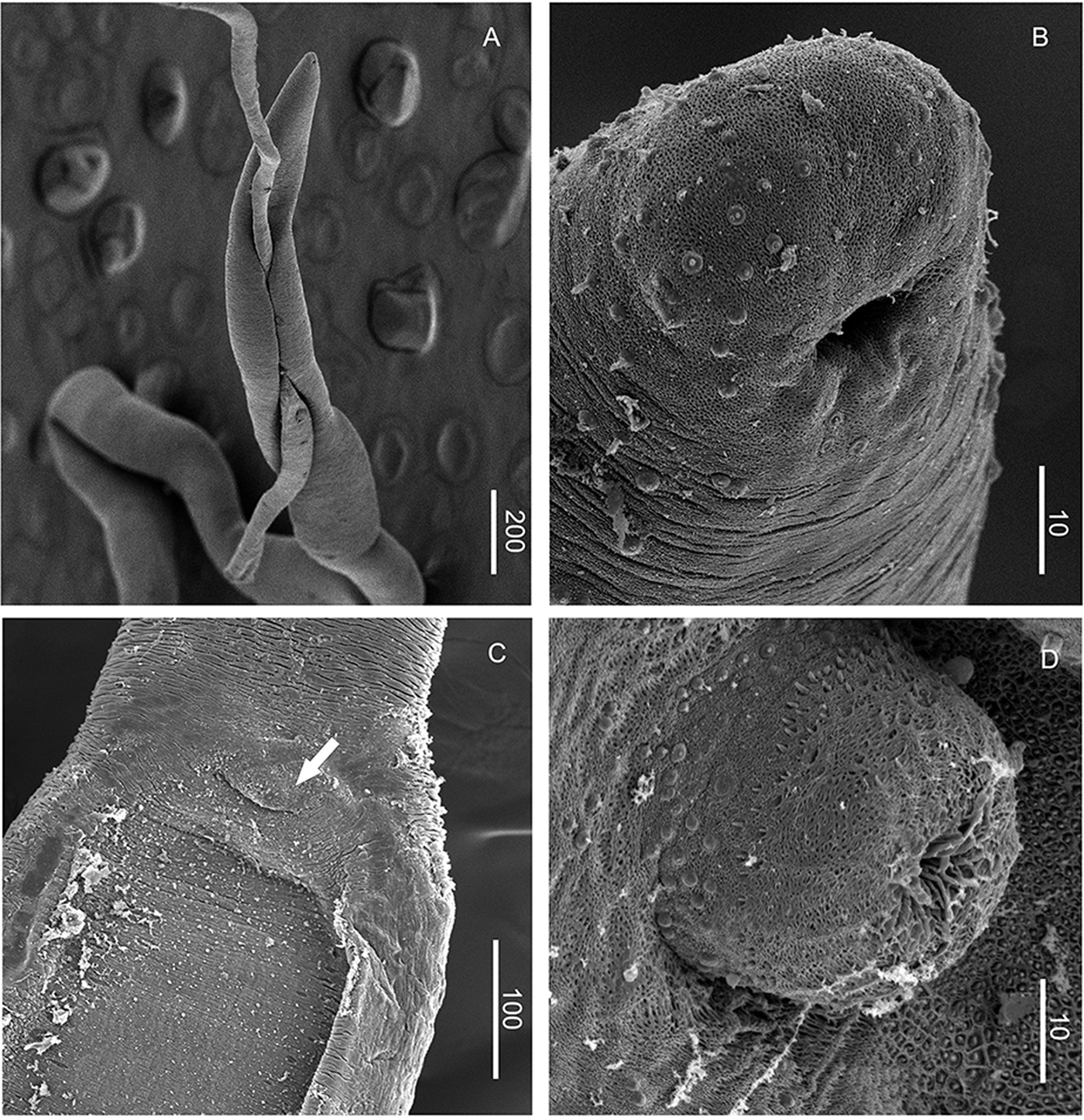
Fig. 3. SEM photographs of M. patagonense n. g., n. sp. from Argentina. (A) Female inside gynaecophoric canal of male. (B) Anterior end of male showing mouth and papillae surrounding it. (C) Anterior end of gynaecophoric canal showing the everted cirrus (arrow). (D) Details of the everted cirrus.
Female (measurements based on ten stained specimens; Fig. 2): Body filiform 14.26 (10.70–19.30) (n = 3) mm long, maximum width at vitellaria level 130 (95–180) (n = 7). Oesophagus 790 (620–1200) (n = 7) long, bifurcating to paired caeca 1080 (640–1400) (n = 3), joining immediately or at short distance posteriorly to seminal receptacle, to form common caecum ending at end of body. Ovary 526 (340–690) long by 54 (42–63) wide (n = 8), located at 1289 (1110–1590) (n = 7) from anterior end of body and at 659 (570–730) (n = 6) from oesophagus bifurcation; coiled in 7 (4–9) loops, depending on the contraction state of specimen. Seminal receptacle 143 (110–230) long (n = 6), just posterior to ovary. Oviduct rising dorsally from posterior end of ovary, bends in a loop and receives duct from seminal receptacle, turns forward. Mehlis' gland immediately anterior to ootype. Ootype at 1020 (630–1320) from anterior end. Vitellaria field starts at 103 (50–130) (n = 4) from end of seminal receptacle. Vitelloduct enters oviduct immediately anterior to Mehlis' gland. Uterus long, opening ventrally at anterior end of body, near oral opening. One egg in development was observed (n = 6), occasionally already formed 63 (55–70) long by 24 (22–25) wide (n = 3), with a minute terminal spine. Eggs never observed in intestinal villi. Posterior body end rounded, often spatulated, lacks protrusions.
Taxonomic summary
Type host: Larus dominicanus Lichtenstein (Aves: Laridae).
Other hosts: Chroicocephalus maculipennis (Lichtenstein) (Aves: Laridae), Spheniscus demersus (Linnaeus) (Aves: Spheniscidae).
Site of infection: Mesenteric veins of posterior intestine, penetrating the serosa.
Intermediate host: Siphonaria lessonii Blainville (Gastropoda: Siphonariidae).
Type locality: Puerto Madryn (42°76′ S–65°03′ W), Chubut province, Argentina.
Other localities: Ensenada (34°51′ S–57°57′ W), Buenos Aires province, Fracasso Beach (42.42° S, 064.12° W), Comodoro Rivadavia (MSB:Para: 18934; 45.87° S, 067.48° W), and Caleta Córdova (MSB:Para: 18938; 45°45′ S, 67°22′ W) Chubut province; Puerto Deseado (47°52′ S, 65°45′ W) Santa Cruz province, National Park Nahuel Huapi (41°6′ S, 71°17′ W) Río Negro province, Argentina (Alda and Martorelli, Reference Alda and Martorelli2009; Bagnato et al., Reference Bagnato, Gilardoni, Di Giorgio and Cremonte2015; Brant et al., Reference Brant, Loker, Casalins and Flores2017 and current study), South Africa (Aldhoun and Horne, Reference Aldhoun and Horne2015).
Prevalences: L. dominicanus (P = 68%), C. maculipennis (P = 50%) from Puerto Madryn.
Specimens deposited: Holotype (MLP-He 4828), allotype (MLP-He 4829), paratypes (MLP-He 4830, CNP-Par 196) and paragenophores (MSB:Para: 32121, 32124, 32128, 32129, 32130, 32131) (sensu Pliejel et al., Reference Pliejel, Jondelius, Norlinder, Nygren, Oxelman, Schander, Sundberg and Thollesson2008).
ZooBank registration: 901E1AFB-CAE4-4FAB-AD32-8B505B9C90C1.
Etymology: The species name refers to the ‘Patagonian’ region, a geographical area where the species was first found.
Riverabilharzia n. g.
Diagnosis
Schistosomatidae. Thin filiform worms, entire body surface covered by striations and papillae. Sexes similar in shape, females slenderer. Body cylindrical, tapered anterior end, rounded or spatulated posterior end without protrusions. Mouth subterminal. Oral and ventral suckers absent. Female genital pore at anterior end of body. Erection of this new genus is proposed based upon the morphological combinations of characters relative to other species as well as genetic characterization of the specimens found and described in this study, and a worm recovered from a Brazilian penguin (MG670449) using partial sequence data for the nuclear 28S and mitochondrial cox1 gene. Riverabilharzia can be differentiated from all other known genera within the family by lack of oral and ventral sucker, short gynaecophoric canal, oesophagus bifurcation and caecal reunion near anterior end, absence of intestinal diverticula (further morphological discussion detailed below), as well as comparison of partial sequence data for the nuclear 28S and mitochondrial cox1 gene; these specimens form a clade and do not group within any of the named genera in the tree that also have morphological justifications, or any of the unnamed lineages.
Type species: Riverabilharzia ensenadense n. g., n. sp.
Etymology: The genus name refers to the ‘river’ environment where it was first found.
Riverabilharzia ensenadense n. g., n. sp.
Description
Male (measurements based on ten stained specimens; no entire worm was recovered, total length is estimated; Figs 4 and 5): Body extremely thin, 18.1 mm long, almost uniform width, 79 (60–100) (n = 7) at oesophagus bifurcation, widest at gynaecophoric canal 112 (80–160) (n = 7) and 87 (70–115) (n = 7) at testicular field. Oesophagus 541 (410–700) long (n = 5), bifurcating into two short caeca 584 (500–585) (n = 3), joining anteriorly to external seminal vesicle to form unique caecum. Common caecum zigzags ending near end of body. Gynaecophoric canal (very) short 281 (240–340) long (n = 7), starting posteriorly to cirrus sac and 1485 (850–1780) (n = 6) from the anterior end. Transverse bands absent. Testicular field starting short distance from posterior end of gynaecophoric canal 136 (50–200) (n = 5) and ending near end of body. Testes rounded, 39 (28–48) long, 39 (33–55) wide (n = 6), more than 200 (n = 6) in number, arranged alternately either of common caecum, smaller at start of testicular field and enlarging posteriorly. Vas deferens leads forward from testes, bends posteriorly and forms seminal vesicle elongated and sinuous, anterior to gynaecophoric canal. External seminal vesicle starting 680 (610–790) (n = 5) from oesophagus bifurcation, 88 (70–100) long (n = 6), 22 (20–25) wide; internal seminal vesicle enclosed within conspicuous cirrus sac 307 (130–410) (n = 7) and surrounded by prostatic cells. Thinner ejaculatory duct ending in a genital pore situated in a genital papilla covered with minute spines located at anterior edge of gynaecophoric canal. Cirrus not observed. Posterior body end spatulated without any protrusion.

Fig. 4. Light microscope photographs of M. patagonense n. g., n. sp. (A–C) and R. ensenadense n. g., n. sp. (D–F) males from Argentina. (A) Anterior end showing gynaecophoric canal (CG). (B) Details of the gynaecophoric canal, showing cirrus (arrow). (C) Posterior end. (D) Anterior end showing gynaecophoric canal (CG). (E) Details of the gynaecophoric canal, showing cirrus (arrow). (F) Posterior end.
Female (measurements based on 11 stained specimens; Figs 5 and 6). Body filiform, until 14.8 mm long, maximum width at vitellaria 89 (50–170) (n = 8). Oesophagus 644 (530–830) (n = 4) long, bifurcating 557 (370–840) (n = 7) from anterior end to form paired-caeca 1525 (1400–1650) long (n = 2), joining immediately posterior to seminal receptacle to form common caecum, ending at end of body. Ovary coiled, 554 (350–790) (n = 11) long, located 1182 (850–1500) (n = 10) from anterior end and 649 (480–800) (n = 9) from oesophagus bifurcation. Seminal receptacle 147 (90–200) (n = 9) long, situated immediately posterior to ovary. Oviduct arises from posterior end of ovary, bends in a loop and receives a branch from seminal receptacle. Mehlis' gland immediately anterior to ootype. Ootype 329 (270–400) (n = 8) distant from caecal bifurcation. Vitellaria field start just posterior to seminal receptacle and continued to end of body along each side of common caecum. Vitelloduct enters oviduct immediately anterior to Mehlis' gland. Uterus long, opening ventrally at anterior end of body, near oral opening. Usually one egg observed (n = 8), oval to rounded, 58 (40–75) by 41 (30–55) (n = 6), with a minute terminal spine. Eggs usually observed in intestinal villi. Posterior body end rounded.

Fig. 5. Drawings of R. ensenadense n. g., n. sp. from Argentina. (A–C) Male. (A) Anterior end. (B) Detail of terminal genitalia. (C) Posterior end. (D and E) Female. (D) Anterior end. (E) Posterior end.

Fig. 6. Light microscope photographs of R. ensenadense n. g., n. sp. female, from Argentina. (A) Anterior end, showing oesophagus bifurcation (eb), ootype (o), ovary (o) and seminar receptacle (sr). (B) Details of anterior end showing mouth (m) and uterus opening (uo). (C) Egg inside ootype. (D) Seminal receptacle (sr) and ovary loops (o). (E) Vitellaria field. (F) Egg recovered from intestinal villi.
Taxonomical summary
Type host: Larus dominicanus Lichtenstein (Aves, Laridae).
Other hosts: Chroicocephalus maculipennis (Lichtenstein), C. cirrocephalus (Vieillot), (Aves: Laridae), Spheniscus magellanicus (Forster) (Aves, Spheniscidae).
Site of infection: Mesenteric veins of intestine, penetrating deeply into the mucosa.
Intermediate host: unknown
Type locality: Ensenada (34°51′ S, 57°57′ W), Buenos Aires Province, Argentina.
Other locality: Rio Grande, Rio Grande do Sul State (32°01′34″ S, 52°06′21″ W), Brazil (Vanstreels et al., Reference Vanstreels, Gardiner, Yabsley, Swanepoel, Kolesnikovas, Silva-Filho, Ewbank and Catão-Dias2018).
Prevalences: L. dominicanus (P = 70%), C. maculipennis (P = 50%), C. cirrocephalus (29%) from Ensenada.
Specimens deposited: Holotype (MLP 4831), allotype (MLP 4832), paratypes (MLP-He 4833, 4834, 4835), and paragenophores (MSB:Para: 32122, 32123, 32125, 32126, 32127) (sensu Pleijel et al., Reference Pliejel, Jondelius, Norlinder, Nygren, Oxelman, Schander, Sundberg and Thollesson2008).
ZooBank registration: BA43EA94-A6D3-4493-B405-164E2EE5BA6D.
Etymology: The species name refers to ‘Ensenada’ city, a geographical area where the species was first found.
Taxonomic remarks
The two new species described here are different from all other schistosome genera that are parasites of birds (Austrobilharzia, Ornithobilharzia, Macrobilharzia, Bilharziella, Allobilharzia, Anserobilharzia, Trichobilharzia and Jilinobilharzia) by the absence of the oral and ventral sucker, except for Dendritobilharzia. They differ from Dendritobilharzia in overall body shape (leaf-like vs long and thin), habitat (arterial vs venous system) and shape of common cecum (dendritic vs straight). Our specimens fit more closely with Gigantobilharzia as currently defined (Khalil, Reference Khalil, Gibson, Jones and Bray2002) but not as much the type of Odhner (Reference Odhner1910). However, Odhner (Reference Odhner1910) did not make a formal diagnosis for the genus and very little comparison to other schistosomes, although at that time there were not several described. When he examined the gulls he collected, he found both Bilharzia kowalewskii Parona and Ariola, 1896 and G. acotylea. Distilling his text, he noted the two schistosomes were not the same and concluded that the lack of suckers and protrusions of the posterior end of body distinguished it from B. kowalewskii. Eggs can also be somewhat distinguishing, and his paper notably did not include the eggs, because, as he writes, he lost the paper with the measurements and thus his measurements are inferred from the in utero eggs. Furthermore, he did not designate where the type specimens were deposited. We have found that they are in the Invertebrates Collection of the Swedish Museum of Natural History [Gigantobilharzia Odhner, Reference Odhner1910 in GBIF Secretariat (2021). GBIF Backbone Taxonomy. Checklist dataset https://doi.org/10.15468/39omei accessed via GBIF.org on 2022-01-22]. Thus, that is the generic diagnosis. Likely this inadequate diagnosis was, in part, what contributed to the later confusion on what defines Gigantobilharzia.
The two new species are morphologically different from each other by the length of the gynaecophoric canal; in M. patagonense it is much longer (1056 vs 281) and it is located at a shorter distance from the anterior end than in R. ensenadense (1255 vs 1485). Also, the caecal reunion in males is at the level of the middle of the cirrus sac in M. patagonense, whereas in R. ensenadense it occurs anteriorly to the external seminal vesicle.
According to Khalil (Reference Khalil, Gibson, Jones and Bray2002), the morphological diagnosis of Gigantobilharzia includes the following characters: body thread-like, presence of a short gynaecophoric canal (GC), oral sucker (OS) absent or weakly developed, ventral sucker (VS) absent and a common caecum without lateral diverticula. However, after the description of the type species G. acotylea (no oral or ventral sucker, presence of gynaecophoric canal), the 18 species included in this genus do not fully correspond with either the type description or the amended one. But species of Gigantobilharzia could be grouped based on combination of characters that were more valid (see Table 1). Marinabilharzia patagonense and R. ensenadense (both have no OS, no VS, short GC without TBs) are different from Gigantobilharzia egreta because it last lacks both OS and GC; from Gigantobilharzia monocotylea because it has an OS but no GC; from Gigantobilharzia tantali because it has a weak OS and the GC has TBs; from Gigantobilharzia gyrauli and Gigantobilharzia aegypti because they have an OS; from Gigantobilharzia sturniae, Gigantobilharzia huttoni, Gigantobilharzia ardeolae, Gigantobilharzia adami, Gigantobilharzia nettapi, G. vittensis, Gigantobilharzia lawayi, Gigantobilharzia mazuriana and G. melanoidis, because despite no OS and no VS, all of them have GC with TBs.
The combination of some key features (absence of both OS and VS and a short GC without TBs) in the two new species are like those observed in G. acotylea (type species), Gigantobilharzia elongata, G. huronensis and Gigantobilharzia plectropteri. However, the two new species can be distinguished from G. acotylea by the length of the GC that is longer in M. patagonense and shorter in R. ensenadense than that in G. acotylea (1056, 281 vs 550–700); also the GC starts much further posterior in both new species than that in G. acotylea (1255, 1485 vs 500); by the position of the caecal reunion [at the middle of internal seminal vesicle (ISV), anterior to external seminal vesicle (ESV) vs posterior to ESV], also the eggs are smaller in both new species than that in G. acotylea (63, 58 vs 100). Marinabilharzia patagonense and R. ensenadense can be distinguished from G. elongata because in both of the new species the GC starts much further posterior from the anterior end (1255, 1485 vs 900) and by the position of the caecal reunion (middle of ISV, anterior to ESV vs anterior third of GC). In M. patagonense, the GC begins formerly from the anterior end compared to G. huronensis (1255 vs 1700) and the position of the caecal reunion is different (middle ISV vs anterior to ESV). Riverabilharzia ensenadense can be distinguished from G. huronensis because its GC is shorter (281 vs 1084 respectively). A redescription of G. huronensis is in dire need because the measurements in the description do not correspond to those shown in the figures. Marinabilharzia patagonense and R. ensenadense have a longer GC compared to G. plectropteri (1056 and 281 vs 180–210) and the position of the caecal reunion is different (middle ISV, anterior to ESV vs anterior third of ESV), respectively.
Phylogenetic analyses
The 28S, ITS and cox1 trees (Fig. 7A, B and C, respectively) reconstructed here show that M. patagonense and R. ensenadense grouped into different clades and are neither conspecific nor are they sister taxa. The specimens of M. patagonense found in L. dominicanus, mainly in Puerto Madryn but also in Ensenada, grouped in a clade with the previously published sequences of a schistosome fragment recovered from L. dominicanus in an Andean lake plus with cercariae from the pulmonate false limpet S. lessonii (Fig. 7). Thus, the life cycle occurs in the marine environment, in the intertidal rocky shores of the Patagonian coast. Additionally, a morphologically undescribed schistosome, but sequenced (28S and ITS), from a penguin collected in South Africa (S. demersus) also grouped in the same clade with M. patagonense specimens (see discussion in Brant et al., Reference Brant, Loker, Casalins and Flores2017).

Fig. 7. Phylogenetic trees placing the new taxa described here among the available sequences of avian schistosome taxa, based on (A) 28S sequences, (B) ITS sequences and (C) cox1 sequences. Specimens from this study are in bold and the new taxa are highlighted in grey boxes. The ‘*’ represent significant posterior probability support for the Bayesian analysis, values lower than 0.95 are not indicated. GenBank accession numbers follow the taxon names.
The second new genus and species, R. ensenadense, parasitizing L. dominicanus, C. maculipennis and C. cirrocephalus from Ensenada, grouped in a clade with the previously published sequences of a schistosome fragment recovered from Magellanic penguins in a rehabilitation centre from Brazil (Fig. 7A).
The average genetic distance of cox1 between these two new species was 19.1%, which is higher than the p-distance value (<5%) as suggested by Vilas et al. (Reference Vilas, Criscione and Blouin2005) for species differentiation. Furthermore, the average genetic distance for the same gene region between M. patagonense with G. huronensis and G. melanoidis was in both cases 18.3%; and between R. ensenadense with G. huronensis and G. melanoidis was 19.1 and 16.9%, respectively (see Table 3). As for comparisons among other avian schistosome genera, Allobilharia and Anserobilharzia are average 13.5% and either of those two genera from Trichobilharzia is average 14.5% (Table 3). The intraspecific variation of M. patagonense (that includes Schistosomatidae sp. M2) was 0.4% (Fig. 7C). As mitochondrial DNA is more variable compared to nuclear DNA (Vilas et al., Reference Vilas, Criscione and Blouin2005), these genetic distances, in combination with the phylogenetic tree and morphological evaluation, also support the erection of the new genera and species, M. patagonense and R. ensenadense.
Table 3. Average genetic distances of cox1 (p-distances) among some key taxa

Discussion
This study describes both morphologically and genetically two new genera and species of avian schistosomes from gulls in Argentina. Nowadays, morphological descriptions are supplemented with molecular characterization (Blasco-Costa et al., Reference Blasco-Costa, Cutmore, Miller and Nolan2016; Gonchar and Galaktionov, Reference Gonchar and Galaktionov2021). This combination is useful for species determination whose morphological characters may not reveal enough variation, e.g. cryptic species or lack of sufficient material (Gagnon et al., Reference Gagnon, Kasl, Preisser, Belden and Detwiler2021).
After the original description of the type genus and species, G. acotylea, which is characterized by the absence of an OS and VS and the presence of a GC, and a posterior end of body with protrusions, several other species were later identified as Gigantobilharzia, despite not having the defined morphological characteristics of the genus described by Odhner (see Table 1). Because of this, several authors have proposed that a revision of the genus is needed and would likely result in reassigning Gigantobilharzia species into several genera (e.g. Brackett, Reference Brackett1942; Farley, Reference Farley1971; Khalifa, Reference Khalifa1974; Schuster et al., Reference Schuster, Aldhoun and O'Donovan2014). After a detailed review of the currently defined species of Gigantobilharzia, we agree with previous authors that a revision is needed. Furthermore, we consider that six different groups can be morphologically distinguished by a combination of some features (see Tables 1 and 4). There are 13 species (G. acotylea, G. adami, G. ardeolae, G. elongata, G. huttoni, G. huronensis, G. lawayi, G. mazuriana, G. melanoidis, G. nettapi, G. sturniae, G. plectropteri and G. vittensis) that agree with the original generic diagnosis in the absence of OS and VS and the presence of GC, but not the protrusions in the posterior end of body (see Table 1). However, unlike the description for the type species, nine of these species (G. adami, G. ardeolae, G. huttoni, G. lawayi, G. mazuriana, G. melanoidis, G. nettapi, G. sturniae and G. vittensis) have TBs in their GC (Table 1). Although morphologically (broadly speaking), six groups can be proposed based on combinations of characters, we do not have the molecular information necessary to test the validity of these character combinations as synapomorphies or to determine the interrelationships within the genus Gigantobilharzia and among other schistosomes (Schuster et al., Reference Schuster, Aldhoun and O'Donovan2014). As was mentioned, G. huronensis, G. melanoidis and G. vittensis have associated sequence data. These species share (with each other and with G. acotylea) the absence of OS and VS and the presence of GC, but all differ in the position of the caecal reunion and lack of posterior end of body protrusions. However, according to this study, Schuster et al. (Reference Schuster, Aldhoun and O'Donovan2014) with G. melanoidis and Pinto et al. (Reference Pinto, Pulido-Murillo, de Melo and Brant2017) with G. vittensis, none of these species grouped in phylogenetic analyses with G. huronensis, nor to each other, indicating that Gigantobilharzia is not monophyletic as defined. Even more, Pinto et al. (Reference Pinto, Pulido-Murillo, de Melo and Brant2017) emphasized that G. vittensis does not belong to Gigantobilharzia based on egg morphology and molecular data from cercariae. This scenario is repeated in this study, where morphological characterizations of the two new species do not allow differentiation (mostly because of the generic description for Gigantobilharzia) but the molecular phylogenetic tree shows that they correspond to different genera. All mentioned results confirm that the current diagnosis for Gigantobilharzia is not taxonomically useful and does not correspond thus far with any genetic results.
Table 4. Proposed generic groups for species of Gigantobilharzia that share main characters with the type species G. acotylea, taking into account the morphological and genetic features

As was mentioned in the ‘Results’ section, genetic distances can be used as a proxy for delineating species and genera. Genetic distance among most avian schistosome genera is 16–19% for the 5′ half of cox1 gene (Brant and Loker, Reference Brant and Loker2009; Brant et al., Reference Brant, Pomajbíková, Modry, Petrželková, Todd and Loker2013, Reference Brant, Loker, Casalins and Flores2017; Pinto et al., Reference Pinto, Pulido-Murillo, de Melo and Brant2017). Our results are within this range (Table 3), which also supports the erection of the two new genera and species herein. Considering the above and based on the phylogenetic results, we suggest that several Gigantobilharzia species could be reassigned into at least four different genera, which together with Marinabilharzia and Riverabilharzia would represent six new genera into the family (Table 4). wThis might include G. huronensis (1), an unidentified schistosome cercaria parasitizing Haminoea japonica which was suggested to correspond to G. huttoni (2) (marine life cycle; also see Brant et al., Reference Brant, Cohen, James, Hui, Hom and Loker2010), G. melanoidis (3), G. vittensis (4), the marine species described herein, M. patagonense (5) and the freshwater species described herein, R. ensenadense (6) (Table 4; Fig. 7).
As currently defined, the avian definitive hosts of Gigantobilharzia spp. are diverse and include Pelecaniformes, Anseriformes, Podicipediformes, Charadriiformes, Columbiformes, Sphenisciformes and Passeriformes. Gulls (Charadriiformes) as definitive hosts for schistosome taxa that resemble or were identified as Gigantobilharzia have been found in North America (Farley, Reference Farley1963; Ulmer, Reference Ulmer1968), Europe (Odhner, Reference Odhner1910; Nicoll, Reference Nicoll1914; Bykhovskaya-Pavlovskaya, Reference Bykhovskaya-Pavlovskaya1953, Reference Bykhovskaya-Pavlovskaya1962; Sulgostowska, Reference Sulgostowska1960; Reimer, Reference Reimer1963; Macko, Reference Macko1964; Sitko, Reference Sitko1968; Khalifa, Reference Khalifa1974; Akramova et al., Reference Akramova, Azimov and Shakarboev2010) and Africa (Appleton, Reference Appleton1986). But, schistosomes resembling the species of Gigantobilharzia and occurring in Laridae do not seem to be a common feature for conspecifics or even congeners. For example, other than this study, Pinto et al. (Reference Pinto, Pulido-Murillo, de Melo and Brant2017) found a lineage of schistosomes based on cercariae that genetically grouped with G. vittensis and another clade of schistosomes from USA, both using small freshwater Planorbidae gastropods as intermediate hosts (Fig. 7B). The habitat preferences of each gull species likely determine type of gastropod they contact, and thus the schistosome species they can potentially harbour (e.g. Ebbs et al., Reference Ebbs, Loker, Davis, Flores, Veleizan and Brant2016). Similarly, there is a diverse group of gastropods capable of hosting schistosome genera. These include freshwater gastropod families Physidae, Planorbidae, Lymnaeidae and Thiaridae, and the marine families Haminoeidae and Siphonariidae.
Marinabilharzia patagonense was characterized originally from genetic data from a small fragment, but not morphologically described, parasitizing a kelp gull collected in a freshwater Andean lake in Patagonia (Brant et al., Reference Brant, Loker, Casalins and Flores2017). Using genetic markers, the authors determined that this fragment was conspecific with the cercaria found in the marine limpet S. lessonii from the Atlantic coast of Patagonia, described previously by Alda and Martorelli (Reference Alda and Martorelli2009) and Gilardoni et al. (Reference Gilardoni, Etchegoin, Diaz, Ituarte and Cremonte2011). These results suggested that this species has a marine life cycle, such as G. huttoni, the only other Gigantobilharzia species reported currently with a marine life cycle within the large mostly freshwater clade of avian schistosomes (Brant et al., Reference Brant, Cohen, James, Hui, Hom and Loker2010, Reference Brant, Loker, Casalins and Flores2017). We mentioned previouslya report of a specimen from a penguin off the coast of South Africa that based on genetic data, which was conspecific with M. patagonense. The distribution of parasite species across a wide geographic range is likely facilitated by the ring distribution of L. dominicanus being one of the most abundant gulls in the southern hemisphere including a wide habitat-use distribution.
The life cycle of R. ensenadense is thus far only known from its definitive hosts, Laridae. This schistosome species was found in two gull genera: Larus and Chroicocephalus. As was mentioned, L. dominicanus is widely distributed and has generalist and opportunist behaviours, allowing them to frequent different environments, most of them related to human activities. Chroicocephalus maculipennis is endemic to South America and is predominantly found in freshwater lakes, marshes, river banks and open fields. Chroicocephalus cirrocephalus is distributed in both southern South America and southern Africa and is predominantly found in coastal or estuarine habitats. Given the habitat preferences of these gull species, it could be hypothesized that the gastropod host of Riverabilharzia is likely freshwater, brackish or both, rather than marine. A specimen recovered from one S. magellanicus in Brazil is likely a conspecific of R. ensenadense (Fig. 7A). However, although S. magellanicus is marine, the penguin examined in Vanstreels et al. (Reference Vanstreels, Gardiner, Yabsley, Swanepoel, Kolesnikovas, Silva-Filho, Ewbank and Catão-Dias2018) had been housed in a rehabilitation centre in brackish water filled from a nearby estuary. Perhaps, gulls also frequent this place near the estuary containing appropriate habitat for the putative gastropod host.
Currently, ~70% of avian schistosome species known may cause HCD (see Horák et al., Reference Horák, Mikeš, Lichtenbergová, Skála, Soldánová and Brant2015). In Argentina, there are some records of HCD caused by different avian schistosome larvae from freshwater environments (see Szidat, Reference Szidat1958; Martorelli, Reference Martorelli1984; Ostrowski de Núñez, Reference Ostrowski de Núñez and de Castellanos1992; Veleizán et al., Reference Veleizán, Flores and Viozzi2016). The recreational use of costal environments has been suggested as one of the risk factors for the occurrence, incidence and severity of HCD in some places (Verbrugge et al., Reference Verbrugge, Rainey, Reimink and Blankespoor2004; Selbach et al., Reference Selbach, Soldánová and Sures2016; Veleizán et al., Reference Veleizán, Flores and Viozzi2016). The coasts of the Rio de La Plata and Argentinean sea, frequented by laird species, are also increasingly used by people in the summer months as recreational areas. Considering the population growth of common gull species favoured by garbage dump resources, the presence of these two schistosome species, could constitute an increased risk of HCD in the future.
The results detailed in this study allow us to improve the knowledge about schistosomes parasitizing aquatic birds and supports the idea that DNA diagnoses should be used in combination with morphology, since much of the time morphology is not enough to distinguish cryptic species (see Nadler and Pérez-Ponce de León, Reference Nadler and Pérez-Ponce de León2011; Félix et al., Reference Félix, Braendle and Cutter2014; Blasco-Costa et al., Reference Blasco-Costa, Cutmore, Miller and Nolan2016). Two new species and genera are described here and a discussion to revise the taxonomy of species of Gigantobilharzia is presented. Based on the species of Gigantobilharzia for which we have genetic data, we have proposed a justification for at least another four new genera.
Acknowledgements
The authors gratefully thank Hernán Améndola, Mariano Dorrego and our laboratory partners for their help in the fieldwork. We thank fauna and flora secretary of Buenos Aires province (Disposition Nos. 43/16, 30/17 and 132/18) and Chubut province (Disposition Nos. 15/16, 60/17, 03/18 and 78/19) for permits granted. We thank Patricia Sarmiento of the Servicio de Microscopía Electrónica de Barrido from Museo de La Plata and Graciela T. Navone for her constant support, and to Dr Ben Hanelt (University of New Mexico) for translating and interpreting the German papers.
Author contributions
E. L., J. I. D. and F. C. conceived the idea; E. L., C. G., J. I. D. and F. C. carried out the samplings; E. L., J. I. D., C. G. and F. C. carried out the morphological study; S. V. B. carried out the molecular and phylogenetic analyses. All the authors contributed to the results and discussion as well as both the draft and final versions of the manuscript; J. I. D. prepared the artwork and S. V. B. performed the English language check; J. I. D. and F. C. supervised and contributed equally to the study.
Financial support
This study was supported by CONICET (J. I. D., PIP 0698), Universidad Nacional de La Plata (J. I. D., grant number N859). The University of New Mexico supported the work done in New Mexico through a National Science Foundation grant (S. V. B., DEB 1021427). Technical assistance at UNM Molecular Biology Facility was supported by the Institute Development Award programme of the National Center for Research Resources (NIH 1P20RR18754).
Conflict of interest
The authors declare there are no conflicts of interest.