Introduction
Among parasitic zoonoses, fasciolosis is particularly interesting due to its worldwide distribution, altitudinal transmission and the increasing impact on public and veterinary health that has come with its (re-)emergence. This parasitosis is caused by the liver flukes Fasciola hepatica and Fasciola gigantica (Trematoda: Digenea: Fasciolidae). The former has a major role in the global burden of the disease with the largest distribution (from temperate to tropical regions of all continents except Antarctica) and host range. More restricted, F. gigantica is largely distributed within the tropical and subtropical regions of Asia and Africa (Mas-Coma et al., Reference Mas-Coma, Bargues and Valero2009a). Incomplete reproductive isolating mechanisms have favoured interspecific cross-hybridization in regions of Asia and Africa where both species overlap, imposing an additional challenge for morphological and genetic identification of their intermediate forms, and for the epidemiological management of the disease (Itagaki et al., Reference Itagaki, Ichinomiya, Fukuda, Fusyuku and Carmona2011; Shoriki et al., Reference Shoriki, Ichikawa-Seki, Suganuma, Naito, Hayashi, Nakao, Aita, Mohanta, Inoue, Murakami and Itagaki2016). A third species, Fasciola nyanzae, infects only hippos generating scarce interest (Dinnik and Dinnik, Reference Dinnik and Dinnik1961), whereas genetic analysis currently locates former Fasciola jacksoni (significant parasite of Asian elephants), into the genus Fascioloides (Heneberg, Reference Heneberg2013; Rajapakse et al., Reference Rajapakse, Pham, Karunathilake, Lawton and Le2020). Therefore, in the present paper, only F. hepatica and F. gigantica will be discussed.
Both parasites present a similar two-host life cycle in which freshwater snails of the family Lymnaeidae and a large variety of mammals (i.e. domestic ruminants, wildlife, and humans) serve, respectively, as intermediate and definitive hosts. In the snail host, Fasciola spp. undergoes development and asexual reproduction; from the infective miracidium to sporocyst and then, to several generations of redia and to thousands of cercariae. The mammalian host gets infected after consuming edible plants or water contaminated with encysted cercariae (=metacercariae) and, once in the liver and biliary ducts of the host, the parasites reach adulthood and sexual maturity to produce thousands of eggs. The eggs embryonate and hatch in the environment after being expelled within the feces, and the resulting miracidia actively infect the snail host, continuing the life cycle (see Andrews, Reference Andrews and Dalton1999). The migration of the parasite to the liver and through the liver parenchyma in the mammal host coincides with the acute phase, whereas a chronic or obstructive phase may occur once the adult flukes are established within the bile ducts (see Behm and Sangster, Reference Behm, Sangster and Dalton1999 for details on the synthomatology and disease progression).
The progression of the disease and the number and intensity of the symptoms in the definitive host depend on the quantity of ingested cysts, the stage of the infection and the species involved, as well as the immunity and nutritional status of the host (Mas-Coma et al., Reference Mas-Coma, Bargues and Valero2009a). Morbidity is mostly unspecific and extremely variable; some people may have only mild symptomatology early in the infection or none at all, while others only feel sick later in the infection, during the chronic phase, when the damage to the liver and the gallbladder is significant (Kaya et al., Reference Kaya, Beştaş and Çetin2011; Ramachandran et al., Reference Ramachandran, Ajjampur, Chandramohan and Varghese2012). This is particularly important as it can have a direct epidemiological impact on both diagnosis (incidence and prevalence) and transmission (parasite circulation), as discussed further on. The death rate could be significant in both sheep and cattle, as they usually harbour higher numbers of parasites, but it is generally low among humans (Torgerson and Claxton, Reference Torgerson, Claxton and Dalton1999). Although significantly fewer, ectopic infections can occur and, in humans, adult flukes have been reported in intestines and peritoneal cavity, skin and subcutaneous tissues, eyes, brain, pancreas, neck and lymph node, lung and dorsal spine (see Taghipour et al., Reference Taghipour, Zaki, Rostami, Foroutan, Ghaffarifar, Fathi and Abdoli2019 for review).
Long considered mostly of veterinary concern, the global increase in the prevalence and distribution of fasciolosis in livestock is worrying (Pritchard et al., Reference Pritchard, Forbes, Williams, Salimi-Bejestani and Daniel2005; Rojas et al., Reference Rojas, Vázquez, Domenech and Robertson2010; Caminade et al., Reference Caminade, van Dijk, Baylis and Williams2015; Kelley et al., Reference Kelley, Rathinasamy, Elliott, Rawlin, Beddoe, Stevenson and Spithill2020). Similarly, at the public health level, the pattern of fasciolosis shifted from an occasional zoonotic disease, to today's estimations from 2.4 to 50 million of infected people from all inhabited continents (World Health Organization, 2013; Nyindo and Lukambagire, Reference Nyindo and Lukambagire2015). In this sense, some recent papers have reviewed different aspects of fasciolosis epidemiology and its transmission (e.g. Beesley et al., Reference Beesley, Caminade, Charlier, Flynn, Hodgkinson, Martinez-Moreno, Martinez-Valladares, Perez, Rinaldi and Williams2018; Mas-Coma et al., Reference Mas-Coma, Valero and Bargues2019), whilst independent studies have been made relating fasciolosis impact to factors such as climate change or direct human activities (Mas-Coma et al., Reference Mas-Coma, Valero and Bargues2009b; Caminade et al., Reference Caminade, van Dijk, Baylis and Williams2015; Sabourin et al., Reference Sabourin, Alda, Vázquez, Hurtrez-Boussès and Vittecoq2018). Nonetheless, given the complexity of fasciolosis, it is essential to examine the (re)-emergence of fasciolosis through a more integrative scrutiny of the concomitant factors that affect transmission and may have contributed to such significant increase.
In this review, we attempt an integrative and multicentred analysis of the disease considering both species of Fasciola and all elements involved in transmission (natural or anthropic) at a global scale. It is aimed at providing a comprehensive overview of fasciolosis (re)emergence from all accountable aspects underlying the transmission. To accomplish this goal, we bring forward the various types of factors impacting the burden of fasciolosis and the interrelations that may exist between them to dissect the realities and complexities behind this phenomenon. From biological and ecological to social and political factors, past and current trends of fasciolosis dynamics are analysed at the light of adaptation for parasitism and ecology of transmission, and further contextualized in past and current trends of environmental degradation and of scientific awareness.
Fasciolosis (re-)emergence: an overview
Overall, animal fasciolosis is highly prevalent in livestock worldwide, particularly in bovines, and accounts for most transmissions causing 29% of zoonoses (Nyindo and Lukambagire, Reference Nyindo and Lukambagire2015). An estimate of over 600 million domestic ruminants are infected with Fasciola parasites (Toet et al., Reference Toet, Piedrafita and Spithill2014), causing worldwide losses in animal production of US$3.2 billion per year (Mehmood et al., Reference Mehmood, Zhang, Sabir, Abbas, Ijaz, Durrani, Saleem, Ur Rehman, Iqbal, Wang, Ahmad, Abbas, Hussain, Ghori, Ali, Khan and Li2017). Such economic losses are mainly due to direct death (Fiss et al., Reference Fiss, de Lourdes Adrien, Marcolongo-Pereira, Assis-Brasil, Sallis, Riet-Correa, Ruas and Schild2013), liver condemnation (Nyirenda et al., Reference Nyirenda, Sakala, Moonde, Kayesa, Fandamu, Banda and Sinkala2019), reduced milk, wool and meat production (Charlier et al., Reference Charlier, Vercruysse, Morgan, van Dijk and Williams2014; Arenal et al., Reference Arenal, García, Quesada, Velázquez, Sánchez, Peña, Suárez, Díaz, Sánchez, Casaert, van Dijk, Vercruysse and Charlier2018), and as a result of reduced fertility and high costs of drug therapy (Schweizer et al., Reference Schweizer, Braun, Deplazes and Torgerson2005; Mehmood et al., Reference Mehmood, Zhang, Sabir, Abbas, Ijaz, Durrani, Saleem, Ur Rehman, Iqbal, Wang, Ahmad, Abbas, Hussain, Ghori, Ali, Khan and Li2017). Traditionally, it has been a serious veterinary health concern in areas with diverse socio-economic scenarios and epidemiological contexts such as Europe (Beesley et al., Reference Beesley, Caminade, Charlier, Flynn, Hodgkinson, Martinez-Moreno, Martinez-Valladares, Perez, Rinaldi and Williams2018), East Africa (Gryseels, Reference Gryseels1988), South Asia (Gupta and Singh, Reference Gupta and Singh2002) and the Bolivian Altiplano (Ueno et al., Reference Ueno, Arandia, Morales and Medina1975). Moreover, increasing trends in the prevalence and/or spreading of animal fasciolosis are presumed at a global scale; in the Americas and the Caribbean (Rojas et al., Reference Rojas, Vázquez, Domenech and Robertson2010; Molento et al., Reference Molento, Bennema, Bertot, Pritsch and Arenal2018; Villa-Mancera and Reynoso-Palomar, Reference Villa-Mancera and Reynoso-Palomar2019), in Europe (Pritchard et al., Reference Pritchard, Forbes, Williams, Salimi-Bejestani and Daniel2005; Caminade et al., Reference Caminade, van Dijk, Baylis and Williams2015), in Africa (Jajaa et al., Reference Jajaa, Mushongab, Greenc and Muchenje2017), in Asia (Nguyen et al., Reference Nguyen, Le, Vo, Van Cao, Nguyen, Ho, Nguyen, Tran and Matsumoto2017; Meshgi et al., Reference Meshgi, Majidi-Rad, Hanafi-Bojd and Fathi2019) and in Australia and Oceania (Haydock et al., Reference Haydock, Pomroy, Stevenson and Lawrence2016; Kelley et al., Reference Kelley, Rathinasamy, Elliott, Rawlin, Beddoe, Stevenson and Spithill2020).
A retrospective analysis of human fasciolosis carried out in the early 1990s over a 20-year period of published literature computed a prevalence of merely 2594 clinical cases from 42 countries (Chen and Mott, Reference Chen and Mott1990). Such numbers contrast with the highest current estimates of around 50 million people infected and 180 million living at risk (World Health Organization, 2013; Nyindo and Lukambagire, Reference Nyindo and Lukambagire2015). In addition, a median number of total disability-adjusted life years due to human fasciolosis is nowadays calculated to be 90 041 (95% uncertainty interval; range 58 050–209 097; Torgerson et al., Reference Torgerson, Devleesschauwer, Praet, Speybroeck, Willingham, Kasuga, Rokni, Zhou, Fèvre, Sripa, Gargouri, Fürst, Budke, Carabin, Kirk, Angulo, Havelaar and de Silva2015). Importantly, even though the highlands of South America, the Nile valley, the Caspian Sea basin and East and Southeast Asia are recognized hyperendemic areas of human fasciolosis, human cases occur all over the globe and probably no country can be considered free from the risk of fascioliasis (World Health Organization, 2013).
Previous data show an alarming increase of fasciolosis burden in the last decades. Interestingly, the disease has likely been sub-diagnosed, resulting in lower past and present prevalence (Toet et al., Reference Toet, Piedrafita and Spithill2014), and due to its complex transmission, the rapid rise of fasciolosis as a global health concern is most likely a consequence of different concomitant factors. Therefore, as it was previously mentioned, it should be revised in an integrative manner. In 2012, Cabada and White reviewed the epidemiological data on fasciolosis at the time and suggested that the current burden was related to two (non-exclusive) hypotheses: (i) either the endemic areas are expanding and/or (ii) the disease is being identified in areas where it was not previously observed (Cabada and White, Reference Cabada and White2012). Here, we discuss this topic from both perspectives to give a comprehensive and integrative overview of this phenomenon. On the one hand, the increase of fasciolosis burden lies on the high adaptability of the causative parasites, and has been promoted by the expansion of the livestock industry and the increasing environmental degradation associated with the plethora of human activities, whereas social factors will ultimately define the extent of infection risks, especially within human populations. On the other hand, while a neglected problem in the past, increased scientific and public health awareness have drawn attention to human and animal fasciolosis. The latter has boosted the significance and global burden of this parasitosis as it has promoted epidemiological surveys and improvements in diagnosis (see Fig. 1 for details).

Fig. 1. Factors that have contributed to the rise of fasciolosis burden in the last decades. The biological features of Fasciola parasites determining their high adaptability together with the increased environmental degradation and social risk-related factors are in the core of faciolosis (re-)emergence. The increasing scientific awareness and the boosting of health policies aiming at recognizing and investigating the so-called neglected tropical diseases concomitantly with improvements in diagnosis have contributed to highlight the global burden of this largely ignored disease.
Biological factors: a glance at highly evolved and adaptable parasites
Wide range of and adaptation to hosts
The earliest recorded observation of F. hepatica dates back to 1379 when leaf-shaped parasites found in the liver of a sheep were mistaken for a ‘nuysant et dommageuse herbe’ called dauve that was believed to adhere to the liver after its consumption by the animal and to cause disease (De Brie, Reference De Brie1379). Further works from different European scientists rendered more accurate morphological and biological descriptions of the parasite as well as the elucidation of its complex life cycle. The snail Galba truncatula and domestic herbivores, particularly cattle and sheep, were respectively referenced as the typical intermediate and definitive hosts (details on timeline discovery of F. hepatica in Andrews, Reference Andrews and Dalton1999). Today, the number of reports of different species serving as hosts of F. hepatica and F. gigantica is significant. Overall, around 30 species of freshwater lymnaeid snails are recognized as intermediate hosts (reviewed in Vázquez et al., Reference Vázquez, Alda, Lounnas, Sabourin, Alba, Pointier and Hurtrez-Boussès2018). Furthermore, more than 50 species of mammals from seven different orders, mainly Artiodactyla but also Diprotodontia, Lagomorpha, Perissodactyla, Rodentia, and two examples from Primate and Carnivora, can get naturally infected and transmit at least one of the two species (see Table 1 for details). Even if certain regions may have their own local intermediate and definitive host species, it is evident that fasciolosis can be transmitted almost anywhere in the world (see Table 1 in Vázquez et al., Reference Vázquez, Alda, Lounnas, Sabourin, Alba, Pointier and Hurtrez-Boussès2018).
Table 1. Animal species reported to naturally host adult stages of Fasciola hepatica (F. hep) and F. gigantica (F. gig).

*regions where the host species is introduced.
Concerning snails, besides the family Lymnaeidae (usual intermediate hosts), successful infection (i.e. intramolluscan developed parasite larvae) was observed in Bulinus truncatus (Bulinidae) and Biomphalaria alexandrina (Planorbidae) (Dar et al., Reference Dar, Rondelaud and Dreyfuss2005; Hamed et al., Reference Hamed, Hammami, Khaled, Rondelaud and Ayadi2009). The possible role of Anisus leucostoma (Planorbidae) as an intermediate host of F. hepatica in France has also been suggested, but only when co-infected with Calicophoron daubneyi (Abrous et al., Reference Abrous, Rondelaud, Dreyfuss and Cabaret1998). However, the transmission capacity of these snail species is not clear as infection events may occur at random but do not necessarily translate into successful cercarial shedding and metacercarial development.
In the case of definitive hosts, several examples exist in the literature about the substantial contribution of different species on Fasciola parasites circulation. Such examples vary from local endemic species such as marsupials in Australia (Spratt and Presidente, Reference Spratt and Presidente1981), to wide-range wildlife serving as reservoirs, and worldwide introduced domestic mammals (Haamond, Reference Haamond1972; Ménard et al., Reference Ménard, L'Hostis, Leray, Marchandeau, Pascal, Roudot, Michel and Chauvin2000; Rondelaud et al., Reference Rondelaud, Vignoles, Abrous and Dreyfuss2001; Issia et al., Reference Issia, Pietrokovsky, Sousa-Figueiredo, Stothard and Wisnivesky-Colli2009). As an add-on, effective experimental infections of small (mice, hamsters, Winstar rats, rabbits; Itagaki et al., Reference Itagaki, Sakamoto, Tsutsumi and Itagaki1994; Terasaki et al., Reference Terasaki, Noda, Shibahara, Itagaki, Fukuda and Tsuchiya2003; Phalee et al., Reference Phalee, Wongsawad, Rojanapaibul and Chai2015; Khan et al., Reference Khan, Shareef, Rehman, Ullah, Rehman and Abidi2017) and large mammals (e.g. bighorn sheep; Foreyt, Reference Foreyt2009, the American bison; Foreyt and Drew, Reference Foreyt and Drew2010) are also indistinctively reported for both parasites. There are also isolated reports of the presence of F. hepatica adults and eggs in ratite birds (Palaeognathae), particularly among native South American rheas (Soares et al., Reference Soares, da Silva, Nizoli, Felix and Schild2007; Martínez-Díaz et al., Reference Martínez-Díaz, Martella, Navarro and Ponce-Gordo2013) and emus (Vaughan et al., Reference Vaughan, Charles and Boray1997). Overall, F. hepatica is considered more successful than F. gigantica in exploiting diverse species of mammals and lymnaeid snails (see Table 1 in Vázquez et al., Reference Vázquez, Alda, Lounnas, Sabourin, Alba, Pointier and Hurtrez-Boussès2018), a fact that has certainly contributed to its spread and global burden.
Overall, both Fasciola parasites can be considered generalists in terms of host choice. However, the landscape of parasite specificity differs between intermediate and definitive hosts and across species. The specificity of the intermediate host is narrower considering that all reports of successful Fasciola transmission are only linked to certain snail species within a single family. In addition, several studies have pointed out the occurrence of differential affinities of each Fasciola species to distinct genus and species within the Lymnaeidae at phenotypical (e.g. higher susceptibility in F. gigantica–Radix s.l. combinations; Dar et al., Reference Dar, Vignoles, Rondelaud and Dreyfuss2004; Kaset et al., Reference Kaset, Eursitthichai, Vichasri-Grams, Viyanant and Grams2010; Ashrafi and Mas-Coma, Reference Ashrafi and Mas-Coma2014), eco-epidemiological (differential distribution of Fasciola spp. in relation with snail distribution; Walker et al., Reference Walker, Makundi, Namuba, Kassuku, Keyyu, Hoey, Prödohl, Stothard and Trudgett2008; Mas-Coma et al., Reference Mas-Coma, Bargues and Valero2009a) and population levels (e.g. local acclimation and adaptation; Vázquez et al., Reference Vázquez, Sánchez, Pointier, Théron and Hurtrez-Boussès2014; Alba et al., Reference Alba, Vázquez, Sánchez, Duval, Hernández, Sabourin, Vittecoq, Hurtrez-Boussés and Gourbal2018).
While it is suggested that F. gigantica prefers bovids as definitive hosts, F. hepatica's infectivity, life span, egg shedding and immunity data in ovine species are proposed as evidence of higher adaptability of F. hepatica in the latter (Mas-Coma et al., Reference Mas-Coma, Bargues and Valero2009a). In fact, higher parasite prevalence and intensity are documented in F. hepatica-infected in relation to F. gigantica-infected ovine hosts, with also an enhanced ability to evade and modulate the immune response in sheep (Zhang et al., Reference Zhang, Moreau, Hope, Howard, Huang and Chauvin2005; Raadsma et al., Reference Raadsma, Kingsford, Suharyanta, Spithill and Piedrafita2007). On the other hand, the lateral transfer of F. hepatica to small rodents in the island of Corsica constitutes an example of F. hepatica's significant adaptation to small non-ruminant mammals driven by the insular enlarging of the ecological niche of the wild rat (highly abundant and markedly herbivorous in the island; Magnanou and Morand, Reference Magnanou, Morand, Morand, Krasnov and Poulin2006). The latter, as well as other examples such as the finding of natural infection of F. hepatica in the carnivore Lutra lutra (Shimalov and Shimalov, Reference Shimalov and Shimalov2000), suggest an ecological rather than phylogenetical compatibility, driving leap and acclimation to new definitive hosts in Fasciola spp., particularly F. hepatica.
High polymorphism of virulence factors
Part of the success of Fasciola species relies on a fast and critical ‘sensing’ of the different environments along their life cycle, which is followed by rapid and highly effective metabolic and physiological adjustments to the new conditions (including hosts; Cwiklinski et al., Reference Cwiklinski, Dalton, Dufresne, La Course, Williams, Hodgkinson and Paterson2015; Cwiklinski et al., Reference Cwiklinski, Jewhurst, McVeigh, Barbour, Maule, Tort, O'Neill, Robinson, Donnelly and Dalton2018; Zhang et al., Reference Zhang, Cwiklinski, Hu, Zheng, Sheng, Zhang, Elsheikha, Dalton and Zhu2019b). The genomes of F. hepatica and F. gigantica are among the largest pathogen genomes known (around 1.1–1.3 Gb; Cwiklinski et al., Reference Cwiklinski, Dalton, Dufresne, La Course, Williams, Hodgkinson and Paterson2015; McNulty et al., Reference McNulty, Tort, Rinaldi, Fischer, Rosa, Smircich, Fontenla, Choi, Tyagi, Hallsworth-Pepin, Mann, Kammili, Latham, Dell'Oca, Dominguez, Carmona, Fischer, Brindley and Mitreva2017; Choi et al., Reference Choi, Fontenla, Fischer, Le, Costábile, Blair, Brindley, Tort, Cabada and Mitreva2020). Non-repeating genome sizes are similar to other trematodes but there are genomic regions containing interspersed repetitive elements that are exceptionally long in Fasciola spp. compared to other digenean, even within the Fasciolidae (Choi et al., Reference Choi, Fontenla, Fischer, Le, Costábile, Blair, Brindley, Tort, Cabada and Mitreva2020). Fasciola spp. diverged from the phylogenetically related Fascioloides buski (planorbid snails, and pigs and human as intermediate and definitive hosts), through adaptive radiation that involved genomic changes mediated by transposable elements (Choi et al., Reference Choi, Fontenla, Fischer, Le, Costábile, Blair, Brindley, Tort, Cabada and Mitreva2020). According to the authors, they appear to be lineage-specific and may have played a central role in Fasciola spp. genome size evolution and adaptation capacity to different hosts (Choi et al., Reference Choi, Fontenla, Fischer, Le, Costábile, Blair, Brindley, Tort, Cabada and Mitreva2020). Moreover, substantial genome-wide polymorphism is noticeable in F. hepatica (48% of genes exhibited at least one non-synonymous SNP; Cwiklinski et al., Reference Cwiklinski, Dalton, Dufresne, La Course, Williams, Hodgkinson and Paterson2015). Comparative analysis with other parasitic species reveals phylogenetic conservation and diversification of orthologous protein families among trematodes (McNulty et al., Reference McNulty, Tort, Rinaldi, Fischer, Rosa, Smircich, Fontenla, Choi, Tyagi, Hallsworth-Pepin, Mann, Kammili, Latham, Dell'Oca, Dominguez, Carmona, Fischer, Brindley and Mitreva2017), with high non-synonymous polymorphism found in genes related to other parasitic taxa and involved in infection and survival within the host (Cwiklinski et al., Reference Cwiklinski, Dalton, Dufresne, La Course, Williams, Hodgkinson and Paterson2015).
In this sense, a unique feature of Fasciola trematodes is their almost exclusive reliance on a family of secreted–excreted papain-like cysteine peptidases, called cathepsins (L and B) to parasitize the hosts, facilitating tissue migration, food intake and immune evasion/modulation (Cwiklinski et al., Reference Cwiklinski, Donnelly, Drysdale, Jewhurst, Smith, De Marco Verissimo, Pritsch, O'Neill, Dalton and Robinson2019). They are among the most significantly over-expressed genes in the different developmental stages of Fasciola parasites (McNulty et al., Reference McNulty, Tort, Rinaldi, Fischer, Rosa, Smircich, Fontenla, Choi, Tyagi, Hallsworth-Pepin, Mann, Kammili, Latham, Dell'Oca, Dominguez, Carmona, Fischer, Brindley and Mitreva2017; Zhang et al., Reference Zhang, Cwiklinski, Hu, Zheng, Sheng, Zhang, Elsheikha, Dalton and Zhu2019b) and, whilst within Trematoda they have expanded, the differential expansion and diversification that occurred in Fasciola resulted in more families and gene copy numbers of proteins with overlapping but broad functions (McNulty et al., Reference McNulty, Tort, Rinaldi, Fischer, Rosa, Smircich, Fontenla, Choi, Tyagi, Hallsworth-Pepin, Mann, Kammili, Latham, Dell'Oca, Dominguez, Carmona, Fischer, Brindley and Mitreva2017; Cwiklinski et al., Reference Cwiklinski, Donnelly, Drysdale, Jewhurst, Smith, De Marco Verissimo, Pritsch, O'Neill, Dalton and Robinson2019). So far, 37 and 24 transcript clusters have been, respectively, recognized as cathepsin L and B sequences from transcriptomic analyses in different stages of F. gigantica, including intramolluscan larvae (Zhang et al., Reference Zhang, Cwiklinski, Hu, Zheng, Sheng, Zhang, Elsheikha, Dalton and Zhu2019b). In F. hepatica, at least 11 sequence clusters of cathepsin B and 13 of cathepsin L comprising 29 and 44 sequences, respectively, have been identified from only 13 adult F. hepatica (McNulty et al., Reference McNulty, Tort, Rinaldi, Fischer, Rosa, Smircich, Fontenla, Choi, Tyagi, Hallsworth-Pepin, Mann, Kammili, Latham, Dell'Oca, Dominguez, Carmona, Fischer, Brindley and Mitreva2017). In both species, cathepsins L and B are strictly regulated during development (Di Maggio et al., Reference Di Maggio, Tirloni, Pinto, Diedrich, Yates Iii, Benavides, Carmona, da Silva Vaz and Berasain2016; McNulty et al., Reference McNulty, Tort, Rinaldi, Fischer, Rosa, Smircich, Fontenla, Choi, Tyagi, Hallsworth-Pepin, Mann, Kammili, Latham, Dell'Oca, Dominguez, Carmona, Fischer, Brindley and Mitreva2017; Zhang et al., Reference Zhang, Cwiklinski, Hu, Zheng, Sheng, Zhang, Elsheikha, Dalton and Zhu2019b). Different clades of cathepsin proteases have evolved distinctive peptidolytic activity specific to the requirements of different lifecycle stages (Robinson et al., Reference Robinson, Tort, Lowther, Donnelly, Wong, Xu, Stack, Padula, Herbert and Dalton2008), which highlights the biological significance of these molecules for infection and survival, and their occurrence in host-specific and stage-specific ‘winning combinations’.
Furthermore, a wide-genome comparison of F. hepatica and F. gigantica has underlined the differential expansion and positive selection, along with cathepsins, of the fatty-acid-binding proteins and protein disulphide-isomerases. Such molecular compounds are also significant for infection and survival of the parasites within the hosts along with G-protein-coupled receptors, which may have key roles in physiological and behavioural adaptation to new ecological niches (Choi et al., Reference Choi, Fontenla, Fischer, Le, Costábile, Blair, Brindley, Tort, Cabada and Mitreva2020). These pronounced differential expansions in biologically relevant excretory–secretory proteins leading to lineage-specific adaptation occurred at or after the split between Fasciolinae (Fasciola spp.) and Fasciolopsinae (F. buski) and have been associated with host switch capabilities and to the wide range of hosts related to Fasciola spp. (Choi et al., Reference Choi, Fontenla, Fischer, Le, Costábile, Blair, Brindley, Tort, Cabada and Mitreva2020).
Genetic diversity of parasite populations
In addition to the high genomic polymorphism, perhaps one of the most advantageous features of Fasciola spp. is its ability to generate and maintain an above-fair amount of intra-population genetic diversity (Hurtrez-Boussès et al., Reference Hurtrez-Boussès, Durand, Jabbour-Zahab, Guégan, Meunier, Bargues, Mas-Coma and Renaud2004). For instance, F. hepatica has shown high multilocus genotypic diversity using highly polymorphic markers (e.g. microsatellites) in analysed populations from the UK (Beesley et al., Reference Beesley, Williams, Paterson and Hodgkinson2017), Spain (Vilas et al., Reference Vilas, Vázquez-Prieto and Paniagua2012), Bolivia (Hurtrez-Boussès et al., Reference Hurtrez-Boussès, Durand, Jabbour-Zahab, Guégan, Meunier, Bargues, Mas-Coma and Renaud2004), France (Vázquez et al., Reference Vázquez, Sabourin, Alda, Leroy, Leray, Carron, Mulero, Caty, Hasfia, Boisseau, Saugné, Pineau, Blanchon, Alba, Faugère, Vittecoq and Hurtrez-Boussès2020) and Cuba (Vázquez et al., Reference Vázquez, Lounnas, Sánchez, Alba, Milesi and Hurtrez-Boussés2016). Similar results in this species were observed when other variable markers were explored (e.g. mitochondrial or nuclear) in Spain (Thang et al., Reference Thang, Vázquez-Prieto, Vilas, Paniagua, Ubeira and Ichikawa-Seki2020), Austria (Husch et al., Reference Husch, Sattmann, Haefeli, Prosl and Walochnik2020), Armenia (Aghayan et al., Reference Aghayan, Gevorgian, Ebi, Atoyan, Addy, Mackenstedt, Romig and Wassermann2019), Iran (Bozorgomid et al., Reference Bozorgomid, Rouhani, Harandi, Ichikawa-Seki and Raeghi2020) and Australia (Elliott et al., Reference Elliott, Muller, Brockwell, Murphy, Grillo, Toet, Anderson, Sangster and Spithill2014). In the case of F. gigantica, high genetic diversity is also reported from Pakistan (Rehman et al., Reference Rehman, Zahid, Rashid, Ali, Akbar, Oneeb, Shehzad, Ashraf, Sargison and Chaudhry2020), Cambodia (Loeurng et al., Reference Loeurng, Ichikawa-Seki, Wannasan, Sothyra, Chaisowwong and Tiwananthagorn2019) and Nigeria (Ichikawa-Seki et al., Reference Ichikawa-Seki, Tokashiki, Opara, Iroh, Hayashi, Kumar and Itagaki2017b) using mitochondrial markers. However, other countries have shown lower haplotype diversity generally associated with recent introductions from particular regions, such as in Indonesia (Hayashi et al., Reference Hayashi, Ichikawa-Seki, Allamanda, Wibowo, Mohanta, Sodirun, Guswanto and Nishikawa2016).
In any case, in both F. hepatica and F. gigantica, although a globally high genetic diversity is observed, a lack of between-population differentiation within the same country or region has been found. This is generally explained by a continuous movement of livestock that facilitates the mixing of populations (Vázquez et al., Reference Vázquez, Lounnas, Sánchez, Alba, Milesi and Hurtrez-Boussés2016; Beesley et al., Reference Beesley, Williams, Paterson and Hodgkinson2017; Ichikawa-Seki et al., Reference Ichikawa-Seki, Tokashiki, Opara, Iroh, Hayashi, Kumar and Itagaki2017b). However, several studies assert that some structuration may exist in the definitive hosts, which raise questions on host specificity and the general epidemiology of fasciolosis. For example, significant differentiation between F. hepatica isolates was observed in Spain and Iran when hosts such as cattle, buffalo or sheep were explored (Meshgi et al., Reference Meshgi, Karimi and Shayan2008; Vilas et al., Reference Vilas, Vázquez-Prieto and Paniagua2012).
Such a marked tendency towards maintaining very high genetic diversity is usually sustained by a preferential outcrossing over a self-mating strategy of the hermaphroditic adult flukes (Hurtrez-Boussès et al., Reference Hurtrez-Boussès, Durand, Jabbour-Zahab, Guégan, Meunier, Bargues, Mas-Coma and Renaud2004; Beesley et al., Reference Beesley, Williams, Paterson and Hodgkinson2017). The existence of high within-population diversity translates into greater plasticity and fast adaptability to new environmental (intra or extra-hosts) variations and constraints, and thus, to higher chances of initiating and maintaining transmission, even in unusual hosts. In a worldwide scenario of fasciolosis (re-)emergence, genetically diversified parasite populations may also facilitate the appearance of resistance to anthelmintic drugs, further hindering disease control (Dreyfuss and Rondelaud, Reference Dreyfuss and Rondelaud2008).
Parasitic strategies
Experimental infections of intermediate hosts indicate that their interaction with Fasciola parasites is usually characterized by relatively high survival of infected snails during the mid-late days of pre-patent infection and early-patent period (Sorensen and Minchella, Reference Sorensen and Minchella2001; Dar et al., Reference Dar, Vignoles, Rondelaud and Dreyfuss2004; Ashrafi and Mas-Coma, Reference Ashrafi and Mas-Coma2014; Alba et al., Reference Alba, Vázquez, Sánchez, Duval, Hernández, Sabourin, Vittecoq, Hurtrez-Boussés and Gourbal2018; Vázquez et al., Reference Vázquez, de Vargas, Alba, Sánchez, Alda, Sabourin, Vittecoq, Alarcón-Elbal, Pointier and Hurtrez-Boussès2019). In the definitive hosts, particularly in humans, fasciolosis is frequently non-fatal and clinically mild especially in the case of low-intensity infections (Behm and Sangster, Reference Behm, Sangster and Dalton1999). As a result, the lifespan of most infected hosts is long enough for Fasciola trematodes to develop into rediae and shed cercariae (in the snail) or into egg-producing sexually mature parasites (in the definitive hosts).
The predominant infection strategy of fasciolids involves the immune evasion/modulation of the immune system of the hosts starting at early infection, which derives into a parasite-driven hyporesponsive or inhibitory immune phenotype in both definitive and intermediate hosts. Overall, polarized antigen-specific Th (lymphocytes T helper) 2 pattern and/or downregulation of Th1 and Th17 immune responses are detected in splenocytes within 7 days in F. hepatica-murids/ruminants and in F. gigantica-buffalo models, and are featured by a mixed pattern of elevated anti-inflammatory cytokines [e.g. interleukin (IL)-10, tumour growth factor (TGF)-β]. Higher IL-4/interferon-γ ratio can be also observed with the increase of the parasitic burden and/or infection progress (Mulcahy et al., Reference Mulcahy, O'Connor, Clery, Hogan, Dowd, Andrews and Dalton1999; O'Neill et al., Reference O'Neill, Brady, Callanan, Mulcahy, Joyce, Mills and Dalton2000; Donnelly et al., Reference Donnelly, Stack, O'Neill, Sayed, Williams and Dalton2008; Walsh et al., Reference Walsh, Brady, Finlay, Boon and Mills2009; Shi et al., Reference Shi, Wei, Elsheikha, Zhang, Sheng, Lu, Wang, Huang and Zhu2017; Sheng et al., Reference Sheng, Li, Wang, Kang, Wei, Zhang, Zhu, Luo and Huang2019). Towards chronicity, immunomodulation of inflammation and a significant cytokine dysregulation are also present as Th2/Treg immune responses become more dominant, with increasing levels of Treg lymphocytes, TGF-β and IL-10 and neutralizing inflammatory Th1/Th2 cytokines in different Fasciola-mammal models (Walsh et al., Reference Walsh, Brady, Finlay, Boon and Mills2009; Escamilla et al., Reference Escamilla, Zafra, Pérez, McNeilly, Pacheco, Buffoni, Martínez-Moreno, Molina-Hernández and Martínez-Moreno2016; Shi et al., Reference Shi, Wei, Elsheikha, Zhang, Sheng, Lu, Wang, Huang and Zhu2017). On the intermediate host side, a few hours following infection, there is no significant haemocyte (snail immune cells) proliferation. In addition, lower expression of the proliferative cytokine granulin and lower levels of in vitro encapsulation activity (=protective immune response towards Fasciola) of haemocytes against F. hepatica larvae have been found in susceptible over resistant Pseudosuccinea columella snails (Alba et al., Reference Alba, Duval, Sánchez, Pérez, Pinaud, Galinier, Vázquez and Gourbal2020).
These are some of the fine strategies aimed at avoiding/diminishing the defence response of the hosts that not only support the parasite's successful establishment but also could directly contribute to the balance of energetic trade-offs between the two partners, mitigating the fitness costs of the infection for the host and building up host tolerance. Furthermore, both Fasciola species also appear to induce wound-healing immune mechanisms in their hosts (Ruiz-Campillo et al., Reference Ruiz-Campillo, Molina-Hernández, Pérez, Pacheco, Pérez, Escamilla, Martínez-Moreno, Martínez-Moreno and Zafra2018; Zhang et al., Reference Zhang, Mei, Liang, Zhu, Sheng, Shi, Wang and Huang2020). Controlling inflammation and inducing tissue repair mechanisms are also certainly needed to assure the migratory activity of Fasciola trematodes without seriously compromising vital tissues and thus, supporting the overall long-term survival of the parasites (Adelman and Hawley, Reference Adelman and Hawley2017; Motran et al., Reference Motran, Silvane, Chiapello, Theumer, Ambrosio, Volpini, Celias and Cervi2018).
The rapid and significant modulation of host immunity exerted by the parasites is possibly linked to the lack of concomitant immunity following Fasciola primo infection. In this sense, as pre-exposure to fasciolids does not confer complete protection in trickle/challenge infection of intermediate (Alba et al., Reference Alba, Vázquez, Sánchez, Duval, Hernández, Sabourin, Vittecoq, Hurtrez-Boussés and Gourbal2018) or definitive hosts (Bossaert et al., Reference Bossaert, Jacquinet, Saunders, Farnir and Losson2000; Wiedosari et al., Reference Wiedosari, Hayakawa and Copeman2006), it is certainly an add-on factor for transmission.
Finally, the longevity of adult parasites, which could range from 9–12 months up to 11 years depending on the combination of host species-Fasciola (Mas-Coma et al., Reference Mas-Coma, Bargues and Valero2009a), the large egg outputs (Walker et al., Reference Walker, Hoey, Fletcher, Brennan, Fairweather and Trudgett2006) and the environmental resilience of eggs and metacercariae (Luzón-Peña et al., Reference Luzón-Peña, Rojo-Vázquez and Gómez-Bautista1994; Suhardono et al., Reference Suhardono, Roberts and Copeman2006a) are also pivotal strategies for parasitism. Furthermore, the significant clonal expansion within the snail host in which tens of rediae and thousands of cercariae can be generated from single miracidium infection (Rondelaud et al., Reference Rondelaud, Belfaiza, Vignoles, Moncef and Dreyfuss2009; Ashrafi and Mas-Coma, Reference Ashrafi and Mas-Coma2014; Hodgkinson et al., Reference Hodgkinson, Cwiklinski, Beesley, Hartley, Allen and Williams2018) is another aspect to consider when discussing transmission success of Fasciola parasites.
Environmental degradation: ecological impact of human activities and climate factors as major determinants of fasciolosis dynamics
The transmission of a parasite by its hosts is a probabilistic event that depends on biological determinants as well as on environmental constraints (suitable ecological conditions, availability of compatible hosts, etc.; Sandland and Minchella, Reference Sandland and Minchella2003). Therefore, different environments in which hosts and parasites develop strongly affect specificity and genotype selection and determine the close link between host–parasite relationship and natural or anthropic changes (Wolinska and King, Reference Wolinska and King2009). Particularly, human activities and the unsustainable exploitation of natural resources that have dramatically increased over the past decades are leading to a serious environmental degradation that influences current and future epidemiological patterns of infectious diseases including that of Fasciola spp. transmission.
Livestock production and land use
Since the 1960s, livestock systems have increasingly developed to respond to the food demands of an ever-growing human population. Today, livestock occupies between 22 and 26% of the ice-free terrestrial surface of the planet (Phelps and Kaplan, Reference Phelps and Kaplan2017), and it is increasingly affected by fasciolosis (Mehmood et al., Reference Mehmood, Zhang, Sabir, Abbas, Ijaz, Durrani, Saleem, Ur Rehman, Iqbal, Wang, Ahmad, Abbas, Hussain, Ghori, Ali, Khan and Li2017). Changes in distribution and/or increase of animal fasciolosis over the last decades have been discussed elsewhere (see Mehmood et al., Reference Mehmood, Zhang, Sabir, Abbas, Ijaz, Durrani, Saleem, Ur Rehman, Iqbal, Wang, Ahmad, Abbas, Hussain, Ghori, Ali, Khan and Li2017; Beesley et al., Reference Beesley, Caminade, Charlier, Flynn, Hodgkinson, Martinez-Moreno, Martinez-Valladares, Perez, Rinaldi and Williams2018 for review on animal fasciolosis). Reports of active transmission foci of the parasites and naturally infected snails occurring in lands associated with farming and agricultural activities are also frequent (e.g. Schweizer et al., Reference Schweizer, Meli, Torgerson, Lutz, Deplazes and Braun2007; Nguyen et al., Reference Nguyen, Nguyen, Van Nguyen, Huynh, Le, Fukuda and Nakai2012; Grabner et al., Reference Grabner, Mohamed, Nachev, Méabed, Sabry and Sures2014; Vázquez et al., Reference Vázquez, Sánchez, Alba, Pointier and Hurtrez-Boussés2015). Other authors have informed of an increase in parasite prevalence among field populations of lymnaeid hosts over time (Dreyfuss et al., Reference Dreyfuss, Vignoles and Rondelaud2003; Alba et al., Reference Alba, Vázquez, Sánchez, Fraga, Martínez, Hernández, Marcet, Figueredo and Sarracent2016).
Livestock-related land use has drastically modified terrestrial ecosystems into extensive pasture fields that include natural water bodies but also drains, ditches and large-scale irrigation systems. These systems are often built to manage water supply and/or flash floods. Indeed, flood-based practices are occasionally used to support grazing and bolster the food industry (Robinson et al., Reference Robinson, Thornton, Franceschini, Kruska, Chiozza, Notenbaert, Cecchi, Herrero, Epprecht, Fritz, You, Conchedda and See2011). This anthropomorphic degradation of natural habitats impacts fasciolosis transmission as it fosters lymnaeid snails-prone ecosystems in proximity to definitive hosts. Ecological features within the Lymnaeidae family vary between genus and species. For instance, several Galba species (generally highly associated with F. hepatica transmission) can thrive from cold to more tropical regions and present a considerably amphibious nature, whilst Radix snails are aquatic species. Thus, Galba species are frequently found in shallower and/or temporary waters such as ditches, puddles, banks of slowly-moving streams, spring swamps, reeds, cattle watering tanks and irrigation channels (Hammami and Ayadi, Reference Hammami and Ayadi1999; de Kock et al., Reference De Kock, Wolmarans and Bornman2003; Kleiman et al., Reference Kleiman, Pietrokovsky, Prepelitchi, Carbajo and Wisnivesky-Colli2007; Schweizer et al., Reference Schweizer, Meli, Torgerson, Lutz, Deplazes and Braun2007; Vázquez et al., Reference Vázquez, Hevia and Sánchez2009). Nevertheless, beyond the differences, a general pattern is evident within the family Lymnaeidae: a preference for stagnant, slow-flowing water ecosystems where they usually reach high densities and occur close to the shoreline (Utzinger and Tanner, Reference Utzinger and Tanner2000; de Kock et al., Reference De Kock, Wolmarans and Bornman2003; Dreyfuss et al., Reference Dreyfuss, Vignoles and Rondelaud2003; Kleiman et al., Reference Kleiman, Pietrokovsky, Prepelitchi, Carbajo and Wisnivesky-Colli2007; Schweizer et al., Reference Schweizer, Meli, Torgerson, Lutz, Deplazes and Braun2007; Vázquez et al., Reference Vázquez, Hevia and Sánchez2009; Dida et al., Reference Dida, Gelder, Anyona, Matano, Abuom, Adoka, Ouma, Kanangire, Owuor and Ofulla2014). In particular, different authors report a positive association between the presence and abundance of different lymnaeid host species in anthropized sites such as farms and pasture lands (Pfukenyi et al., Reference Pfukenyi, Mukaratirwa, Willingham and Monrad2006; Kleiman et al., Reference Kleiman, Pietrokovsky, Prepelitchi, Carbajo and Wisnivesky-Colli2007; Schweizer et al., Reference Schweizer, Meli, Torgerson, Lutz, Deplazes and Braun2007; Vázquez et al., Reference Vázquez, Hevia and Sánchez2009; Novobilský et al., Reference Novobilský, Kašný, Beran, Rondelaud and Höglund2013; Alba et al., Reference Alba, Vázquez, Sánchez, Fraga, Martínez, Hernández, Marcet, Figueredo and Sarracent2016), and man-made irrigation systems (Diaw et al., Reference Diaw, Vassiliades, Seye and Sarr1990; Hammami and Ayadi, Reference Hammami and Ayadi1999; Esteban et al., Reference Esteban, Gonzalez, Bargues, Angles, Sanchez, Naquira and Mas-Coma2002; Chlyeh et al., Reference Chlyeh, Dodet, Delay, Khallaayoune and Jarne2006; Novobilský et al., Reference Novobilský, Kašný, Beran, Rondelaud and Höglund2013; Grabner et al., Reference Grabner, Mohamed, Nachev, Méabed, Sabry and Sures2014). Therefore, under such conditions, the probability of contact between Fasciola parasites and their intermediate and definitive hosts increases.
Increase land use has led to deforestation and land overexploitation, significantly increasing the risk of floods and/or drought in the different areas (Food and Agriculture Organization, 2020). Therefore, the growing need to install infrastructure to manage freshwater supplies for the livestock industry has resulted in 20% increase of global land area equipped for irrigation from 1995 to 2016 (Food and Agriculture Organization, 2020). These conditions can further promote the establishment, growth and development of lymnaeid snail populations and the survival of parasite free-living stages (even stating certain seasonality of transmission). Furthermore, man-made irrigation systems can also greatly influence the genetic structure of snail populations as snails can be frequently dispersed throughout the hydrological network (Sabourin et al., Reference Sabourin, Alda, Vázquez, Hurtrez-Boussès and Vittecoq2018). The relationship between the drainage basin structure and genetic population structure of lymnaeid snails was studied on G. truncatula. Significant genetic differentiation increasing with hydrographic distance and consistent with a dispersion dependent on the direction of water flow has been observed (Hurtrez-Boussès et al., Reference Hurtrez-Boussès, Hurtrez, Turpin, Durand, Durand, De Meeüs, Meunier and Renaud2010). This can affect the epidemiology of fasciolosis transmission as the ability for Fasciola parasites to infect and develop in the snail hosts can vary among populations (e.g. Vignoles et al., Reference Vignoles, Dreyfuss and Rondelaud2012; Vázquez et al., Reference Vázquez, Sánchez, Pointier, Théron and Hurtrez-Boussès2014). The installation of artificial irrigation networks may favour the rapid spread of snail genotypes sensitive to F. hepatica and may therefore be a concomitant factor on the (re-)emergence of fasciolosis in certain areas (Hurtrez-Boussès et al., Reference Hurtrez-Boussès, Hurtrez, Turpin, Durand, Durand, De Meeüs, Meunier and Renaud2010).
Concerning transmission to humans, sporadic cases and epidemic outbreaks of human fasciolosis usually occur where the presence of infected local livestock is also acknowledged, mainly in association with rural environments (e.g. Mas-Coma et al., Reference Mas-Coma, Anglés, Esteban, Bargues, Buchon, Franken and Strauss1999; Esteban et al., Reference Esteban, Gonzalez, Bargues, Angles, Sanchez, Naquira and Mas-Coma2002, Reference Esteban, Gonzalez, Curtale, Muñoz-Antoli, Valero, Bargues, Mabrouk, el-Wakeel, Abdel-Wahab, Montresor, Engels, Savioli and Mas-Coma2003; Parkinson et al., Reference Parkinson, O'Neill and Dalton2007; Rojas et al., Reference Rojas, Vázquez, Domenech and Robertson2010; Nguyen et al., Reference Nguyen, Nguyen, Van Nguyen, Huynh, Le, Fukuda and Nakai2012; Chen et al., Reference Chen, Chen, Ai, Xu, Jiao, Zhu, Su, Zang, Luo, Guo, Lv and Zhou2013; Ashrafi et al., Reference Ashrafi, Valero, Peixoto, Artigas, Panova and Mas-Coma2015). Therefore, the ongoing circulation of Fasciola parasites in domestic animals is associated with higher risks of human infection. Nonetheless, a highly significant prevalence of animal fasciolosis does not necessarily associate with areas where human infection is also a highly significant problem (Mas-Coma et al., Reference Mas-Coma, Bargues and Valero2009a). The latter highlights the significance of social factors as the ultimate drivers of Fasciola spp. transmission, which will be further discussed.
Climate change and global warming
The impact of human activities is driving drastic changes in long-term weather patterns with global warming being one of its more patent effects. The livestock industry is a major contributor of heat-trapping gases to the atmosphere, accounting for around 20% of total anthropogenic emissions, and more CO2 production than the entire world's transportation systems combined (Thornton et al., Reference Thornton, van de Steeg, Notenbaert and Herrero2008). The impacts of climate change throughout the globe will vary from region to region but overall, mean temperature will rise, as well as the frequency of extreme weather events (e.g. droughts, rainfalls), all of which will affect the spatial and temporal dynamics of infectious diseases such as fasciolosis (Utaaker and Robertson, Reference Utaaker and Robertson2015).
In the current global climate scenario, it is crucial to delineate the causality between climate change and the transmission of parasites, particularly of those like Fasciola spp. which have heteroxenous life cycles and development stages both in the environment and/or in ectothermic hosts or vectors (Mas-Coma et al., Reference Mas-Coma, Valero and Bargues2009b). Climate can influence population dynamics (e.g. host/parasite abundance, population genetic structure and diversity, adaptation), the parasite's life cycle (e.g. physiology, metabolism, development rate) and disease transmission (e.g. host/parasite distribution and behaviour, transmission windows, susceptibility/virulence, prevalence, intensity, morbidity and mortality). In turn, such changes may lead to community-wide impacts such as shifts in species composition of host and parasite faunas (Polley and Thompson, Reference Polley and Thompson2009; Mas-Coma et al., Reference Mas-Coma, Valero and Bargues2009b).
The presence and abundance of lymnaeid host populations are dependent on environmental factors such as water velocity, soil evapotranspiration, rainfall and temperature, depicting different seasonality patterns (Goumghar et al., Reference Goumghar, Vignoles, Rondelaud, Dreyfuss and Benlemlih2001; Prepelitchi et al., Reference Prepelitchi, Pietrokovsky, Kleiman, Rubel, Issia, Moriena, Racioppi, Álvarez and Wisnivesky-Colli2011). Even in compatible snail–digenean systems, changes in ecological variables such as temperature and droughts may directly and indirectly alter the outcome of parasite exposure. Average daily temperatures above 10 or 16°C combined with high moisture levels are required for the reproduction of F. hepatica or F. gigantica within the snail; thus, if these conditions are not met, the transmission is halted (Mas-Coma et al., Reference Mas-Coma, Bargues and Valero2009a). Furthermore, the dependency of temperature and moisture levels in egg development and metacercarial infectivity span is widely acknowledged for Fasciola spp. (Andrews, Reference Andrews and Dalton1999). Therefore, an increase in temperature within the optimal range for parasites and snail hosts is associated with a shorter pre-patent infection (the period before cercarial shedding) and increase in cercarial output and metacercariae production (Polley and Thompson, Reference Polley and Thompson2009; Mas-Coma et al., Reference Mas-Coma, Valero and Bargues2009b).
Extreme drought/rainfall events will also probably increase in frequency and yet its effect on transmission efficacy of Fasciola spp. may be difficult to ascertain. Even when extreme precipitations may result in deleterious effects for snail populations and wash away free-living stages (reducing the infection risk per individual host), they can also lead to extensive relocation of hosts and parasitic larvae in nearby lands through flooding and thus, to spatially expand transmission foci (Utaaker and Robertson, Reference Utaaker and Robertson2015). In East Anglia (UK), the emergence of fasciolosis in cattle at the beginning of the 21st century was associated with increased precipitation levels during summer months, favouring the thriving of snail hosts and the development of parasite free-living stages along with wetter grazing conditions (Pritchard et al., Reference Pritchard, Forbes, Williams, Salimi-Bejestani and Daniel2005). In Cuba, one of the largest outbreaks of human fasciolosis resulted from the consumption of lettuce contaminated with F. hepatica cysts following pastures run-off into the crop field due to heavy rainfall events (Rojas et al., Reference Rojas, Vázquez, Domenech and Robertson2010). Moderate droughts may impose a patchier distribution of transmission foci and higher risk for infection per individual hosts. These may result from an increase in the density of parasites and hosts as metacercariae-free herbage becomes sparse and the number and volume of remnant water bodies are reduced, promoting contact of all elements involved in transmission (Fox et al., Reference Fox, White, McClean, Marion, Evans and Hutchings2011; Vázquez et al., Reference Vázquez, Sánchez, Alba, Pointier and Hurtrez-Boussés2015). Prolonged drought is intuitively related to a reduced risk of infection because free-living parasite stages and snail hosts may succumb to desiccation. However, during a severe water shortage, man-made irrigation systems, watering tanks and other artificial water sources might supply the necessary conditions to at least keep transmission afloat within domestic animals, and creating ‘artificial oasis’ for lymnaeid snails to colonize. The construction of man-made water resources to support farming and agriculture in a region within the Peruvian Altiplano during the third quarter of the 20th century resulted in the permanent transmission foci of F. hepatica, a current hot spot of human fasciolosis (Esteban et al., Reference Esteban, Gonzalez, Bargues, Angles, Sanchez, Naquira and Mas-Coma2002).
Of note, even when major effects on transmission dynamics are expected to occur at the snail/parasite interface, it is already accepted that global warming, in combination with an increase in the frequency of weather extremes, also has serious direct and indirect effects on vertebrates including domestic animals. Even in moderate climate zones, such effects impact grassland production, fodder quality and nutrition of definitive hosts while also increasing environmental stress, and pathogens and vector loads. These, in turn, directly affect animal physiology, behaviour, welfare, health, and increase susceptibility to infections (Gauly and Ammer, Reference Gauly and Ammer2020).
The influence of environmental factors on the distribution and dynamics of fasciolosis in different regions has been evidenced within recent years. For instance, the intensification and spreading of animal fasciolosis in the UK and acute fasciolosis outbreaks in southern Italy were related to simultaneous increases in temperature and rainfall (Pritchard et al., Reference Pritchard, Forbes, Williams, Salimi-Bejestani and Daniel2005; Fox et al., Reference Fox, White, McClean, Marion, Evans and Hutchings2011; Bosco et al., Reference Bosco, Rinaldi, Musella, Amadesi and Cringoli2015). Other examples include the diminishing of fasciolosis incidence in cattle after a heat wave in southwestern France (Thomas et al., Reference Thomas, Jacquiet and Dorchies2007) or the emergence of human fasciolosis in Pakistan related to climate change and man-made irrigation systems (Afshan et al., Reference Afshan, Fortes-Lima, Artigas, Valero, Qayyum and Mas-Coma2014). Due to a growing understanding and acceptance of the effects of climate change (particularly global warming and rainfall patterns) on the incidence and spreading of fasciolosis, several models have attempted to assess (Malone et al., Reference Malone, Gommes, Hansen, Yilma, Slingenberg, Snijders, Nachtergaele and Ataman1998; Fuentes et al., Reference Fuentes, Sainz-Elipe, Nieto, Malone and Mas-Coma2005; Afshan et al., Reference Afshan, Fortes-Lima, Artigas, Valero, Qayyum and Mas-Coma2014) and forecast (Fox et al., Reference Fox, White, McClean, Marion, Evans and Hutchings2011; Caminade et al., Reference Caminade, van Dijk, Baylis and Williams2015; Haydock et al., Reference Haydock, Pomroy, Stevenson and Lawrence2016) the risk for transmission based on climate data (latitude, altitude, local rainfall and temperature patterns, soil evapotranspiration, etc.). An overall increase of risk for transmission related to global warming in regions such as Europe (Caminade et al., Reference Caminade, van Dijk, Baylis and Williams2015), Britain (Fox et al., Reference Fox, White, McClean, Marion, Evans and Hutchings2011) and New Zealand (Haydock et al., Reference Haydock, Pomroy, Stevenson and Lawrence2016) is expected to occur in future years.
Ecological invasions of alien species and biodiversity loss
Host populations displaying high genetic diversity and occurring on stable environments are supposed to decrease the chances of parasite transmission (Sandland et al., Reference Sandland, Foster, Zavodna and Minchella2007; Tavalire et al., Reference Tavalire, Blouin and Steinauer2016). However, the arrival of new invaders with different genotypes and usually depleted genetic diversity could drastically modify parasite success (Meunier et al., Reference Meunier, Tirard, Hurtrez-Boussès, Durand, Bargues, Mas-Coma, Pointier, Jourdane and Renaud2001; King and Lively, Reference King and Lively2012). Therefore, processes related to human activity and to the effects of climate change such as the introduction and successful invasion of alien species and the loss of biodiversity are additional factors to consider when discussing the (re-)emergence of fasciolosis.
In addition to the global spread of production animals, the introduction of wild mammal species in new areas (e.g. South American rodent Myocastor coypus in Europe or the European hare Lepus europeaus in South America), along with successful parasite spillbacks, has concomitantly enlarged the repertoire of definitive hosts in the invaded regions (Ménard et al., Reference Ménard, L'Hostis, Leray, Marchandeau, Pascal, Roudot, Michel and Chauvin2000; Cuervo et al., Reference Cuervo, Cataldo, Fantozzi, Deis, Isenrath, Viberti, Artigas, Peixoto, Valero, Sierra and Mas-Coma2015). However, given the generalized presence of livestock and wild native reservoirs of Fasciola spp. worldwide, the distribution and transmission of fasciolosis will ultimately be determined by the distribution of Lymnaeidae snails.
Several species of lymnaeid snails that are highly compatible with local liver flukes have been incidentally introduced out of their native ranges, promoting fasciolosis occurrence. In this sense, two of the most globally distributed and epidemiologically significant lymnaeids, i.e. G. truncatula and P. columella, are spread out of their native range (see Lounnas et al., Reference Lounnas, Correa, Vázquez, Dia, Escobar, Nicot, Arenas, Ayaqui, Dubois, Gimenez, Gutiérrez, González-Ramírez, Noya, Prepelitchi, Uribe, Wisnivesky-Colli, Yong, David, Loker, Jarne, Pointier and Hurtrez-Boussès2017; Alda et al., Reference Alda, Lounnas, Vázquez, Ayaqui, Calvopiña, Celi-Erazo, Dillon, González Ramírez, Loker, Muzzio-Aroca, Nárvaez, Noya, Pereira, Martini Robles, Rodríguez-Hidalgo, Uribe, David, Jarne, Pointier and Hurtrez-Boussès2020), definitely boosting fasciolosis transmission all over the world. For example, G. truncatula is known for transmitting F. hepatica at very high altitudes not only in the Andean Altiplano (Esteban et al., Reference Esteban, Gonzalez, Bargues, Angles, Sanchez, Naquira and Mas-Coma2002) but also in the highlands of southern Tanzania (Walker et al., Reference Walker, Makundi, Namuba, Kassuku, Keyyu, Hoey, Prödohl, Stothard and Trudgett2008) and in the alpine and subalpine ecosystems of the Pyrenees (Roldán et al., Reference Roldán, Begovoeva, López-Olvera, Velarde, Cabezón, Molinar, Pizzato, Pasquetti, Fernández, Mentaberre, Serrano, Puig, Espunyes, Castillo-Contreras, Estruch and Rossi2020). It is long recognized as the main intermediate host of F. hepatica in Europe (Mas-Coma et al., Reference Mas-Coma, Bargues and Valero2009a) and in Africa; G. truncatula is believed to be a significant host of F. gigantica as natural infection and significant larval production in experimental exposures have been recorded (Dar et al., Reference Dar, Rondelaud and Dreyfuss2005). The long-distance flash invasion of P. columella from North to South America and the Caribbean, Africa, Australia and the Pacific Islands, and Europe (see Lounnas et al., Reference Lounnas, Correa, Vázquez, Dia, Escobar, Nicot, Arenas, Ayaqui, Dubois, Gimenez, Gutiérrez, González-Ramírez, Noya, Prepelitchi, Uribe, Wisnivesky-Colli, Yong, David, Loker, Jarne, Pointier and Hurtrez-Boussès2017) in the last 200 years is accompanied by reports of infection status or transmission capacity of the local F. hepatica (Prepelitchi et al., Reference Prepelitchi, Kleiman, Pietrokovsky, Moriena, Racioppi, Alvarez and Wisnivesky-Colli2003; Dar et al., Reference Dar, Vignoles, Rondelaud and Dreyfuss2015; Alba et al., Reference Alba, Vázquez, Sánchez, Lounnas, Pointier, Hurtrez-Boussès and Gourbal2019) and F. gigantica (Grabner et al., Reference Grabner, Mohamed, Nachev, Méabed, Sabry and Sures2014; Malatji and Mukaratirwa, Reference Malatji and Mukaratirwa2019).
On the other hand, changes in pathogen complexes can also result from biological invasions and other human-related processes, and can alter the composition, abundance and timing of pathogen infection within the hosts. As co-infection by multiple parasites is the normal state in host populations, such modification of the already established equilibrium can drastically alter the effect of every single pathogen, increasing the complexity of transmission dynamics (Viney and Graham, Reference Viney and Graham2013). There is scarce information on how exposure and susceptibility to other parasitic (or microbiota) organisms relate to F. hepatica transmission and surely further studies are needed to clarify this. However, as an example, the dual infection of F. hepatica and the rumen fluke C. daubneyi may decrease the mean number of metacercariae and the patent period for both digenean compared to single parasite infections in the snail P. columella (Dreyfuss et al., Reference Dreyfuss, Vignoles and Rondelaud2016).
Lastly, man-driven environmental degradation and introduction/spreading of alien species are also associated with biodiversity loss (Cardinale et al., Reference Cardinale, Duffy, Gonzalez, Hooper, Perrings, Venail, Narwani, Mace, Tilman, Wardle, Kinzig, Daily, Loreau, Grace, Larigauderie, Srivastava and Naeem2012). In particular, the livestock industry plays an important role in the current biodiversity crisis at local and global levels. For instance, between 1900 and 2016, records show a 4-fold increase of human population, and with it, an equal increase of biomass of domestic animals and a biomass reduction of wild animals of a similar extent (Pozio, Reference Pozio2020). Furthermore, the domestication and inbreeding of livestock to increase production yield have led to a continuous drop of the genetic diversity within production animals, particularly in intensive production systems (Gauly and Ammer, Reference Gauly and Ammer2020). The overall result of both effects is a decline in the genetic variability of wild and domestic animal populations, which directly render them more susceptible to changes in environmental conditions and to parasite infections (King and Lively, Reference King and Lively2012). At the snail host level, the consequences of a reduced gene pool for fasciolosis transmission was observed in the Bolivian Altiplano as the hyperendemicity of human fasciolosis reported in this region is supported by the transmission capacity of the single genotype (at six polymorphic microsatellite loci) occurring in local G. truncatula populations (Meunier et al., Reference Meunier, Tirard, Hurtrez-Boussès, Durand, Bargues, Mas-Coma, Pointier, Jourdane and Renaud2001). Additionally, the relatively recent global spreading of a unique invasive P. columella genotype/haplotype illustrates the consequences of biological invasion in infectious diseases dynamics as it is supposed to be highly susceptible to F. hepatica infection and thus, to expand the epidemiological risk of fasciolosis transmission (Lounnas et al., Reference Lounnas, Correa, Vázquez, Dia, Escobar, Nicot, Arenas, Ayaqui, Dubois, Gimenez, Gutiérrez, González-Ramírez, Noya, Prepelitchi, Uribe, Wisnivesky-Colli, Yong, David, Loker, Jarne, Pointier and Hurtrez-Boussès2017).
Social factors: human behaviour as ultimate driver of fasciolosis transmission
As in the case of other food-borne zoonoses, human infection with Fasciola spp. is directly and ultimately linked to livestock management and agricultural practices, environmental resources, population income and living conditions as well as consumption and cultural habits (Macpherson, Reference Macpherson2005). Therefore, it is necessary to discuss not only the biological and environmental factors that promote Fasciola spp. circulation in nature but also the social factors that relate to the increased risk of fasciolosis.
Livestock management and agricultural practices
Livestock is a significant global asset providing many benefits and opportunities associated with rapid sector transformation and growth (Thornton, Reference Thornton2010). However, it also brings social, environmental and public health risks that differ among production systems, management strategies, and environmental and economic vulnerabilities (Robinson et al., Reference Robinson, Thornton, Franceschini, Kruska, Chiozza, Notenbaert, Cecchi, Herrero, Epprecht, Fritz, You, Conchedda and See2011), determining current and future trends on fasciolosis dynamics (Bennema et al., Reference Bennema, Ducheyne, Vercruysse, Claerebout, Hendrickx and Charlier2011). Alongside climatic and environmental variations, management factors also affect the spatial distribution and transmission dynamics of Fasciola, and should always be considered, particularly in forecasting models (Bennema et al., Reference Bennema, Ducheyne, Vercruysse, Claerebout, Hendrickx and Charlier2011).
Parasite control in livestock systems is largely based on the use of chemoprophylactic anthelmintic agents, grazing management or a combination of both. On the one hand, proper pasture management (e.g. rotation, fencing, draining) is essential, especially in stationary husbandry and organic production, to diminish prevalence, to avoid (re-)infection and the high parasite burden that usually correlates with acute fasciolosis, and to contribute to a responsible use of flukicides (Knubben-Schweizer et al., Reference Knubben-Schweizer, Rüegg, Torgerson, Rapsch, Grimm, Hässig, Deplazes and Braun2010). Nevertheless, in high-risk areas, general guidelines of parasite control should be adapted to the specific conditions of each farm (infectious status, husbandry practices, wet habitats, etc.). In such areas, it is also recommended to organize the movement of livestock throughout the different pasture sections, according to the probability of occurrence of transmission foci. Here, it is also essential to consider the presence of lymnaeid snails and the characteristics of Fasciola spp. life cycle such as seasonality and length of the prepatent period within the mammal host (see Knubben-Schweizer et al., Reference Knubben-Schweizer, Rüegg, Torgerson, Rapsch, Grimm, Hässig, Deplazes and Braun2010; Knubben-Schweizer and Torgerson, Reference Knubben-Schweizer and Torgerson2015 for details). A study carried out in Switzerland puts theory into practice demonstrating the efficacy of this approach: a decrease in F. hepatica prevalence (from 30.7 to 9.3% during 3–4 years period) was solely observed in the herds where proper pasture management following these recommendations was applied (Knubben-Schweizer et al., Reference Knubben-Schweizer, Rüegg, Torgerson, Rapsch, Grimm, Hässig, Deplazes and Braun2010). Despite its advantages in the control of fasciolosis and other pasture-borne parasitoses, the design and application of appropriate (lymnaeid snail-based) pasture management can have some practical drawbacks that often threaten compliance. Technical (e.g. insufficient knowledge/information, insufficient suitable pastures for rotations) and behavioural (e.g. insufficient risk perception and/or motivation) are among the most cited challenges (Knubben-Schweizer et al., Reference Knubben-Schweizer, Rüegg, Torgerson, Rapsch, Grimm, Hässig, Deplazes and Braun2010; Knubben-Schweizer and Torgerson, Reference Knubben-Schweizer and Torgerson2015).
On the other hand, treatment with anthelmintics differently relates to the incidence of the disease, depending on the type of drug, its effect on the different parasite stages, the number and period of the applications in relation to the seasonality patterns of transmission (Bloemhoff et al., Reference Bloemhoff, Danaher, Forbes, Morgan, Mulcahy, Power and Sayers2014; Munita et al., Reference Munita, Rea, Martinez-Ibeas, Byrne, McGrath, Munita-Corbalan, Sekiya, Mulcahy and Sayers2019), and to the history of chemotherapeutic pressure exerted on the parasite (McMahon et al., Reference McMahon, Edgar, Hanna, Ellison, Flanagan, McCoy, Kajugu, Gordon, Irwin, Barley, Malone, Brennan and Fairweather2016). Thus, knowledge gaps between the scientific and farming communities could challenge the efficiency of this practice. Also, even when it can be relatively easy to accomplish and should reduce parasite load within the herds, the application of flukicides by itself does not prevent reinfections and other interventions are needed to lower the level of pasture infestation with parasite metacercariae (Knubben-Schweizer et al., Reference Knubben-Schweizer, Rüegg, Torgerson, Rapsch, Grimm, Hässig, Deplazes and Braun2010; Greter et al., Reference Greter, Batil, Alfaroukh, Grimm, Ngandolo, Keiser, Utzinger, Zinsstag and Hattendorf2016). Furthermore, the high selective pressure caused by the traditional overreliance on the use of triclabendazole in livestock has resulted in the appearance and spreading of resistant F. hepatica genotypes. This poses a major problem for the control of both human and animal fasciolosis and may significantly alter the epidemiology and transmission of the parasite over the coming decades (for review, see Kelley et al., Reference Kelley, Elliott, Beddoe, Anderson, Skuce and Spithill2016). Fluke resistance to other anti-flukicide treatments (albendazole, clorsulon) and to multiple treatments has also been reported (see Fairweather et al., Reference Fairweather, Brennan, Hanna, Robinson and Skuce2020 for review).
Along with globalization and a growing demand for food, livestock movement (within or outside its current pasture lands) is also increasing. Movements may facilitate the dispersion of the parasites and the entanglement of ongoing transmission. For instance, this occurred during the New World colonization through the introduction of infected cattle and sheep from Europe (Mas-Coma et al., Reference Mas-Coma, Bargues and Valero2009a). Beyond mediating flash invasions of hosts and parasites into new areas, reports of pastural practices such as nomadism and transhumance as well as livestock trade (local or international) also promote genetic diversity of parasites through an increased genetic flow between domestic animals and/or wildlife. This immediately translates into an increase in parasite adaptability through a more diverse gene pool and has been also linked to the spreading of hybrid/introgressed forms of Fasciola spp. to new areas (see Vázquez et al., Reference Vázquez, Lounnas, Sánchez, Alba, Milesi and Hurtrez-Boussés2016; Beesley et al., Reference Beesley, Williams, Paterson and Hodgkinson2017; Amor et al., Reference Amor, Farjallah, Merella, Alagaili and Mohammed2020; Calvani et al., Reference Calvani, Ichikawa-Seki, Bush, Khounsy and Šlapeta2020 for some examples). This is particularly troublesome if we considered that resistance to available anti-flukicide drugs is on the rise worldwide, and can spread through improper cattle management (Beesley et al., Reference Beesley, Caminade, Charlier, Flynn, Hodgkinson, Martinez-Moreno, Martinez-Valladares, Perez, Rinaldi and Williams2018; Fairweather et al., Reference Fairweather, Brennan, Hanna, Robinson and Skuce2020). Furthermore, such movement of hosts and parasites could facilitate contact with more compatible Fasciola–lymnaeid snail combinations, as it has been recorded from certain allopatric snail–parasite systems (high snail survival, infection rate and parasite burden; Gasnier et al., Reference Gasnier, Rondelaud, Abrous, Carreras, Boulard, Diez-Baños and Cabaret2000; Vázquez et al., Reference Vázquez, Sánchez, Pointier, Théron and Hurtrez-Boussès2014) and hence, can increase the risk of liver fluke transmission.
Aside from livestock movements, other factors and management activities are highlighted as significant risk-increasing factors: (i) using unsafe water resources and prolonged grazing (for review, see Sabourin et al., Reference Sabourin, Alda, Vázquez, Hurtrez-Boussès and Vittecoq2018); (ii) feeding on wetland pastures vs feeding on forage/dry-land crop residues (Suon et al., Reference Suon, Hol, Siek, McLean and Copeman2006; Khan et al., Reference Khan, Sajid, Khan, Iqbal and Iqbal2009; Takeuchi-Storm et al., Reference Takeuchi-Storm, Denwood, Hansen, Halasa, Rattenborg, Boes, Enemark and Thamsborg2017); (iii) increased proportion of grazed grass and length of grazing season (Bennema et al., Reference Bennema, Ducheyne, Vercruysse, Claerebout, Hendrickx and Charlier2011); (iv) mixed farming of small and large ruminants; and (v) the use of stagnant pond bathing and river/canal bathing vs river/tap water bathing (Khan et al., Reference Khan, Sajid, Khan, Iqbal and Iqbal2009). In some cases, lower levels of literacy of farmers have been associated with higher infection risk, as proper knowledge and comprehension of control strategies in relation to the parasite cycle are essential for decreasing transmission in high-risk areas (Villa-Mancera and Reynoso-Palomar, Reference Villa-Mancera and Reynoso-Palomar2019). Current trends have also led to an increase and expansion of organic ruminant production. In this context, parasite management remains a challenge as mandatory outdoor access for all age groups can increase exposure to pasture-borne parasites, whereas restrictions in the prophylactic use of anthelmintic drugs can limit parasite control (Takeuchi-Storm et al., Reference Takeuchi-Storm, Moakes, Thüer, Grovermann, Verwer, Verkaik, Knubben-Schweizer, Höglund, Petkevičius, Thamsborg and Werne2019).
It is worth considering that even when the majority of world's livestock is under exclusive livestock farming (occupying more than 60% of the land devoted to livestock activities), mixed crop–livestock systems occur particularly in areas with the highest population density. This allows sharing and re-utilizing resources such as space and water supplies, with livestock frequently providing draught and manure for crops and control for herbs, whereas the residues and by-products of crops can be used for feeding livestock (Robinson et al., Reference Robinson, Thornton, Franceschini, Kruska, Chiozza, Notenbaert, Cecchi, Herrero, Epprecht, Fritz, You, Conchedda and See2011). Wetland rice-based farming systems have been associated with animal fasciolosis transmission, particularly in regions of South and Southeast Asia where livestock manure is used to fertilize rice fields and animals are then fed with contaminated rice straws (Mahato and Harrison, Reference Mahato and Harrison2005; Suon et al., Reference Suon, Hol, Siek, McLean and Copeman2006; Nguyen et al., Reference Nguyen, Le, Dao, Tran, Praet, Speybroeck, Vercruysse and Dorny2011). In addition, the spatial proximity of infected animals, flooded vegetables usually consumed raw by humans (whether crops fields or small gardens) and shared water supplies may facilitate cross-contamination of crops and/or irrigation waters with parasite free-living stages leading to human infections in both high- and low-income countries (Esteban et al., Reference Esteban, Gonzalez, Bargues, Angles, Sanchez, Naquira and Mas-Coma2002; Nguyen et al., Reference Nguyen, Le, Dao, Tran, Praet, Speybroeck, Vercruysse and Dorny2011; Chen et al., Reference Chen, Chen, Ai, Xu, Jiao, Zhu, Su, Zang, Luo, Guo, Lv and Zhou2013; Milas et al., Reference Milas, Rossi, Philippart, Dorny and Bottieau2020). Traditional practices such as the use of livestock manure as fertilizers might be risky if manure is not properly managed and stored to decrease the viability of Fasciola eggs (Suhardono et al., Reference Suhardono, Roberts and Copeman2006b). Similarly, anecdotal reports of disease outbreaks in housed livestock in high-risk areas have documented the importance of appropriate management of forage and wetland crop residues to prevent livestock infection with metacercariae (John et al., Reference John, Davies, Williams and Hodgkinson2019). In Indonesia, metacercarial attached to submerged rice plants showed the longest survival (5 weeks in water temperatures of 25–30°C); thus, the drying-out of rice fields for at least 2 weeks prior to harvest is needed to affect metacercariae infectivity as well as snail abundance (Suhardono et al., Reference Suhardono, Roberts and Copeman2006a). The importance of maintaining a proper anaerobic ensiling environment to eliminate the risk of F. hepatica transmission from silage has been recently demonstrated (John et al., Reference John, Davies, Howell, Williams and Hodgkinson2020).
Poverty and social inequities
Even though fasciolosis distribution, incidence and emergence are not exclusive of regions within low-income countries, it is considered an infectious disease of poverty (Zhou, Reference Zhou2012). Current hot spots of human fasciolosis are located in the Americas, North of Africa and Southern Asia (Mas-Coma et al., Reference Mas-Coma, Bargues and Valero2018), whereas animal fasciolosis is also more prevalent in some African, Asian and American countries with infection rates comparatively higher in developing than in developed countries (Mehmood et al., Reference Mehmood, Zhang, Sabir, Abbas, Ijaz, Durrani, Saleem, Ur Rehman, Iqbal, Wang, Ahmad, Abbas, Hussain, Ghori, Ali, Khan and Li2017).
Various factors determine the increased prevalence of fasciolosis in low- and middle-income countries. Agriculture and livestock production make important contributions to food security and poverty reduction and it is estimated that about 70% of the world's 1.4 billion persons living in extreme poverty depend on livestock (Food and Agriculture Organization, 2020). Nevertheless, conditions for livestock husbandry between developed and developing countries are usually different, with riskier conditions for transmission in terms of fasciolosis (and other infectious diseases) prevailing in low-income countries. In developed countries, each farmer usually grazes a large number of animals, has more control on the access to pasture and water, produces high-value products, operates applied strategic and tactical drenching programmes and anthelminthic treatments based on knowledge about the parasites (Roberts and Suhardono, Reference Roberts and Suhardono1996). Contrastingly, in low-income countries, scattered smallholder livestock systems usually have low- or no-input management with the animals of usually poor genetic breeds foraging for themselves, feeding on plants or waste that otherwise would not be used. Traditional and out-dated practices are usually coupled with a lack of knowledge among farmers, as literacy is usually lower in rural communities within developing countries. Limited capacity and resources, including limited services for disease detection and control, and unaffordable anthelmintic treatments hinder farmers' ability to control parasites in such settings (Roberts and Suhardono, Reference Roberts and Suhardono1996; Randolph et al., Reference Randolph, Schelling, Grace, Nicholson, Leroy, Cole, Demment, Omore, Zinsstag and Ruel2007; Nguyen et al., Reference Nguyen, Le, Dao, Tran, Praet, Speybroeck, Vercruysse and Dorny2011).
While high-income countries are experiencing certain stagnation in the demand for livestock products and progress in efficient production systems that reduce environmental impact, low-income countries are reporting an increase in production mostly under low-resource livestock-keeping systems (Thornton, Reference Thornton2010). Furthermore, losses related to agriculture and livestock industry are a major factor in extending the cycle of poverty, driving low-resource farmers to overexploit the environment in the attempt to maximize production in already ecologically vulnerable areas (Thornton et al., Reference Thornton, van de Steeg, Notenbaert and Herrero2008). As adaptation and mitigation could be significantly challenging in these settings, all these translate into further environmental degradation of certain areas (Thomas et al., Reference Thomas, Hardy, Lazrus, Mendez, Orlove, Rivera-Collazo, Roberts, Rockman, Warner and Winthrop2019) and into a vicious cycle that perpetuates the ecological, economic and social conditions that favour fasciolosis transmission. In this scenario, low-income countries are expected to continue to experience a greater impact of fasciolosis and other infectious diseases (Roberts and Suhardono, Reference Roberts and Suhardono1996; Piedrafita et al., Reference Piedrafita, Spithill, Smith and Raadsma2010).
In poor smallholder livestock communities, and particularly in developing countries, resources constrain, poor living conditions and low literacy level hinder the implementation of proper management practices and educational campaigns to increase awareness of food and water security as well as the risks of fasciolosis (Gray et al., Reference Gray, Copland and Copeman2008; Robinson and Dalton, Reference Robinson and Dalton2009; Villa-Mancera and Reynoso-Palomar, Reference Villa-Mancera and Reynoso-Palomar2019). Even if treatment-based interventions are applied and are proved effective (e.g. Curtale et al., Reference Curtale, Hassanein and Savioli2005; Zhang et al., Reference Zhang, Si, Zhou, Shang, Li and Zhang2019a), reinfection of animals and/or human communities is likely to occur if not coupled with education on the aspects of parasite transmission, risky practices and infection sources, and on the importance of separating animals from crops (Robinson and Dalton, Reference Robinson and Dalton2009; Greter et al., Reference Greter, Batil, Alfaroukh, Grimm, Ngandolo, Keiser, Utzinger, Zinsstag and Hattendorf2016). Therefore, it has become increasingly clear that options for effective management and sustainable control need to be developed locally with an understanding of the science underlying control mechanisms (Gray et al., Reference Gray, Copland and Copeman2008). Furthermore, resource-poor farmers and communities are more likely to need financial, resource assistance and other incentives to participate in control programmes and to adopt new practices. Thus, any control strategy needs to be accompanied by political commitment, ensuring mobilization of resources and multisectoral approaches (Curtale et al., Reference Curtale, Hassanein and Savioli2005; Gray et al., Reference Gray, Copland and Copeman2008). This represents an additional challenge for developing countries experiencing economic instability and/or political crises.
Water and food security
Estimates indicate that 60% of the world's population lives in ecologically vulnerable areas in low-income countries where the adverse effects of climate change on health may manifest through direct pathways related to the shifting of climate patterns and weather extremes, and through indirect pathways like increasing social instability and inequality, such as food/water insecurity (Thornton et al., Reference Thornton, van de Steeg, Notenbaert and Herrero2008). Even though access to safe food and water has increased in the last decades, around 30% of the world's population still suffers from food and water insecurity (Food and Agriculture Organization, 2020). This social inequality can also positively associate with fasciolosis transmission.
In addition to the pre-existing high probabilities of food/water contamination in rural communities from endemic areas (Esteban et al., Reference Esteban, Gonzalez, Bargues, Angles, Sanchez, Naquira and Mas-Coma2002; Nguyen et al., Reference Nguyen, Le, Dao, Tran, Praet, Speybroeck, Vercruysse and Dorny2011), scarce access to sufficient, safe and nutritious food may promote the consumption of wild edible plants to supplement domestic diets. This increases the risk of infection by Fasciola and other food-borne parasites, particularly in endemic areas (McGarry and Shackleton, Reference McGarry and Shackleton2009; Shumsky et al., Reference Shumsky, Hickey, Pelletier and Johns2014). Furthermore, selling wild plants in non-controlled places is a common practice also associated with the human transmission of fasciolosis and one that extends the epidemiological risk to urban dwellers (see Mas-Coma et al., Reference Mas-Coma, Bargues and Valero2018 for detail). To note, some of these risks have been also described in developed countries, which highlight the potential threat of fasciolosis transmission worldwide. Reports of fasciolosis in Europe through khat leaves consumption after non-controlled importation exist (Doherty et al., Reference Doherty, Price, Moody, Wright and Glynn1995; Chand et al., Reference Chand, Herman, Partridge, Hewitt and Chiodini2009). In addition, cases or outbreaks of human fasciolosis have been reported from eating locally-produced contaminated plants commercialized in urban markets within endemic areas of France (Mailles et al., Reference Mailles, Capek, Ajana, Schepens, Ilef and Vaillant2006) and Australia (Hughes et al., Reference Hughes, Spithill, Smith, Boutlis and Johnson2003), or even cultivated in small house gardens (e.g. Milas et al., Reference Milas, Rossi, Philippart, Dorny and Bottieau2020 in Belgium).
Water-borne transmission might represent another challenge to parasite control as a portion of shed cercariae can exist as free-floating metacercariae (Dreyfuss and Rondelaud, Reference Dreyfuss and Rondelaud1997; Rondelaud et al., Reference Rondelaud, Vignoles, Vareille-Morel, Abrous, Mage, Mouzet and Dreyfuss2004). The significance of secure water sources is pointed out by a study in Pakistan that aimed at assessing the contamination of different water sources with F. hepatica DNA and showed the lowest records from tap water (1.6%) over tube (10%) and open wells (8%), respectively (Khan et al., Reference Khan, Khan, Ayaz, Khan, Anees and Khan2012). Indirect evidence of water-borne transmission has been indicated in particular epidemiological contexts where endemicity and infection rates of the Fasciola parasites are high among animals and snails, and access to safely managed drinking water is scarce (e.g. Esteban et al., Reference Esteban, Gonzalez, Bargues, Angles, Sanchez, Naquira and Mas-Coma2002; Zumaquero-Ríos et al., Reference Zumaquero-Ríos, Sarracent-Pérez, Rojas-García, Rojas-Rivero, Martínez-Tovilla, Valero and Mas-Coma2013; Cabada et al., Reference Cabada, Morales, Webb, Yang, Bravenec, Lopez, Bascope, White and Gotuzzo2018). In the hyperendemic areas of the Northern Andean Altiplano, water and food security is a significant issue as inhabitants lack basic services such as piped potable water, sewage and waste disposal. On the Peruvian side of the Titicaca Lake, drinking water appears to be the predominant human infection source (Esteban et al., Reference Esteban, Gonzalez, Bargues, Angles, Sanchez, Naquira and Mas-Coma2002), as the consumption of raw aquatic plants is uncommon. In contrast, eating contaminated vegetables is supposed to play a more important role than drinking unsafe water in the Bolivian part, eastward from Lake Titicaca (Parkinson et al., Reference Parkinson, O'Neill and Dalton2007). In such a setting, the possible differential epidemiological significance between water and food as the main vehicle of parasite transmission is yet to be linked to other social factors: cultural and dietary habits.
Cultural and dietary habits and human movements
Human behaviour is influenced by psycho-social factors such as ethnicity, culture and religion, which relate to every aspect of our lives including food choice and preparation, and determine the range and level of exposure to parasites (Macpherson, Reference Macpherson2005). Several freshwater plants may be carriers of Fasciola metacercariae but their role as infection sources to humans will ultimately depend on the diet and traditions of the different groups (see Mas-Coma et al., Reference Mas-Coma, Bargues and Valero2018 for review on infection sources). Watercress (both common Nasturtium officinale and N. microphyllum and wild watercress Rorippa sylvestris and R. amphibia) are perennial aquatic or semi-aquatic herbs globally distributed and frequently consumed raw in salads. They are commonly associated with fasciolosis infection in humans worldwide (Mas-Coma et al., Reference Mas-Coma, Bargues and Valero2018) and have been found carrying large numbers of parasite metacercariae compared to those carried by other sympatric aquatic plants (Rondelaud et al., Reference Rondelaud, Vignoles and Dreyfuss2020). It is the most cited infection source of liver flukes to humans in endemic regions of Europe (e.g. Garcia et al., Reference Garcia, Martin, Fernandez and Garcia1985; Mailles et al., Reference Mailles, Capek, Ajana, Schepens, Ilef and Vaillant2006; Vignoles et al., Reference Vignoles, Hourdin, Dreyfuss and Rondelaud2019) and the Americas (e.g. Rojas et al., Reference Rojas, Vázquez, Domenech and Robertson2010; Mera y Sierra et al., Reference Mera y Sierra, Agramunt, Cuervo and Mas-Coma2011; Zumaquero-Ríos et al., Reference Zumaquero-Ríos, Sarracent-Pérez, Rojas-García, Rojas-Rivero, Martínez-Tovilla, Valero and Mas-Coma2013) and several field studies following fasciolosis outbreaks have reported lymnaeid snails in watercress beds (Ferrer et al., Reference Ferrer, Perera, Yong and Amador1989; Rondelaud et al., Reference Rondelaud, Vignoles, Abrous and Dreyfuss2001). Nevertheless, other edible plants have been also pointed out as infection sources in different regions. For instance, in Tunisia, the plant Apium nodiflorum (Fool's watercress) is recorded as the vehicle of parasite metacercariae into humans (Hammami et al., Reference Hammami, Hamed and Ayadi2007), whereas in Southwest China, a human outbreak was associated with the consumption of contaminated fish mint Houttuynia cordata (Chen et al., Reference Chen, Chen, Ai, Xu, Jiao, Zhu, Su, Zang, Luo, Guo, Lv and Zhou2013). In Iran, besides watercress, the species Mentha logifolia (mint) and M. spicata (spearmint) have been implicated in transmission as they are frequently eaten fresh in local traditional foods (Sarkari et al., Reference Sarkari, Ghobakhloo, Moshfea and Eilami2012; Hosseini et al., Reference Hosseini, Sarkari, Moshfe, Motazedian and Abdolahi Khabisi2015). Eating dandelion leaves (Taraxacum dens leonis or T. officinale) has been associated with human infection in France and Argentina (Mera y Sierra et al., Reference Mera y Sierra, Agramunt, Cuervo and Mas-Coma2011; Vignoles et al., Reference Vignoles, Hourdin, Dreyfuss and Rondelaud2019). In several regions of Latin America, the customary drinking of a beverage made from Alfalfa (Medicato sativa), particularly in rural populations, has been also related to an increased risk of infection (Mas-Coma et al., Reference Mas-Coma, Anglés, Esteban, Bargues, Buchon, Franken and Strauss1999; Zumaquero-Ríos et al., Reference Zumaquero-Ríos, Sarracent-Pérez, Rojas-García, Rojas-Rivero, Martínez-Tovilla, Valero and Mas-Coma2013). Other vegetables such as lettuce (Lactuca sativa), spinach (Spinacia oleracea), garden rocket (Eruca vesicaria syn. E. sativa) and leek (Allium porrum) have been also associated with infection (see Mas-Coma et al., Reference Mas-Coma, Bargues and Valero2018).
Cultural habits other than direct consumption of edible plants can also influence fasciolosis transmission in humans. For instance, sucking and/or chewing wild aquatic or semiaquatic plants has been pointed out as one of the possible explanations for the high infection rates in Aymara children in Bolivia (Mas-Coma et al., Reference Mas-Coma, Anglés, Esteban, Bargues, Buchon, Franken and Strauss1999). Several patients from Ethiopia and Yemen have become infected through the traditional chewing of khat (Catha edulis) leaves (Cats et al., Reference Cats, Scholten, Meuwissen and Kuipers2000; Chand et al., Reference Chand, Herman, Partridge, Hewitt and Chiodini2009). The involvement in household and farm work is also considered a risky behaviour and it has been linked to a differential infection burden between women and men in certain human fascioliasis hyperendemic areas. Such differential risks have been observed in the Nile delta, Egypt (Esteban et al., Reference Esteban, Gonzalez, Curtale, Muñoz-Antoli, Valero, Bargues, Mabrouk, el-Wakeel, Abdel-Wahab, Montresor, Engels, Savioli and Mas-Coma2003) and in the Bolivian Altiplano (Curtale et al., Reference Curtale, Hassanein, Barduagni, Yousef, Wakeel, Hallaj and Mas-Coma2007), as these roles are usually assigned to women and girls in these regions and are presumed to increase exposure to the parasite. A common habit among farmers of the Nile Delta region that consists of picking vegetables and then leaving them immersed in the canals to keep them fresh while they continue picking has been identified as a possible reason for the high prevalence of fasciolosis in the area (Hotez et al., Reference Hotez, Savioli and Fenwick2012).
Demographic movements towards metropolitan areas can also influence the incidence of human fasciolosis as Fasciola parasites mostly circulate within rural environments. The significant decrease in human cases in central France, from hundreds being diagnosed between 1956 and 1986 to a few tens between 1986 and 2006, has been associated with two factors: (i) an increased migration of the population towards highly urbanized cities (Vignoles et al., Reference Vignoles, Hourdin, Dreyfuss and Rondelaud2019) and (ii) changes in dietary and cultural habits such as the decrease of recollection and consumption of wild watercress among younger generations (Rondelaud et al., Reference Rondelaud, Dreyfuss, Bouteille and Dardé2000). Nonetheless, even though human infection in city settlements occurs only sporadically, usually due to consumption of metacercariae-carrying vegetables acquired in urban establishments (see e.g. Hughes et al., Reference Hughes, Spithill, Smith, Boutlis and Johnson2003; Mailles et al., Reference Mailles, Capek, Ajana, Schepens, Ilef and Vaillant2006), infection of urban dwellers is also possible during country field trips, as observed in Argentina (Mera y Sierra et al., Reference Mera y Sierra, Agramunt, Cuervo and Mas-Coma2011), or through international travel (Figtree et al., Reference Figtree, Beaman, Lee, Porter, Torey, Hugh and Hudson2015; Salzer and Schmiedel, Reference Salzer and Schmiedel2015). Similarly, human migration can also influence the prevalence of fasciolosis and its distribution. In Southwest Iran, a higher seroprevalence of fasciolosis (2.6%) was found among nomad communities that travel with their flocks twice a year between summer highlands and lower warmer winter pastures in comparison to the settled population (1.8%; Zoghi et al., Reference Zoghi, Emami, Shahriarirad, Vahedi, Cheraghi, Zamiri, Arefkhah, Ghorbani and Sarkari2019). In the USA and Germany where the incidence of human fasciolosis is relatively low, current trends show that, although some cases are local, most are associated with travellers or migrant populations (Chand et al., Reference Chand, Herman, Partridge, Hewitt and Chiodini2009; Fried and Abruzzi, Reference Fried and Abruzzi2010; Salzer and Schmiedel, Reference Salzer and Schmiedel2015).
Figure 2 summarizes, in an integrative diagram, the interrelation of biological, environmental and social factors discussed so far at the view of Fasciola spp. transmission.
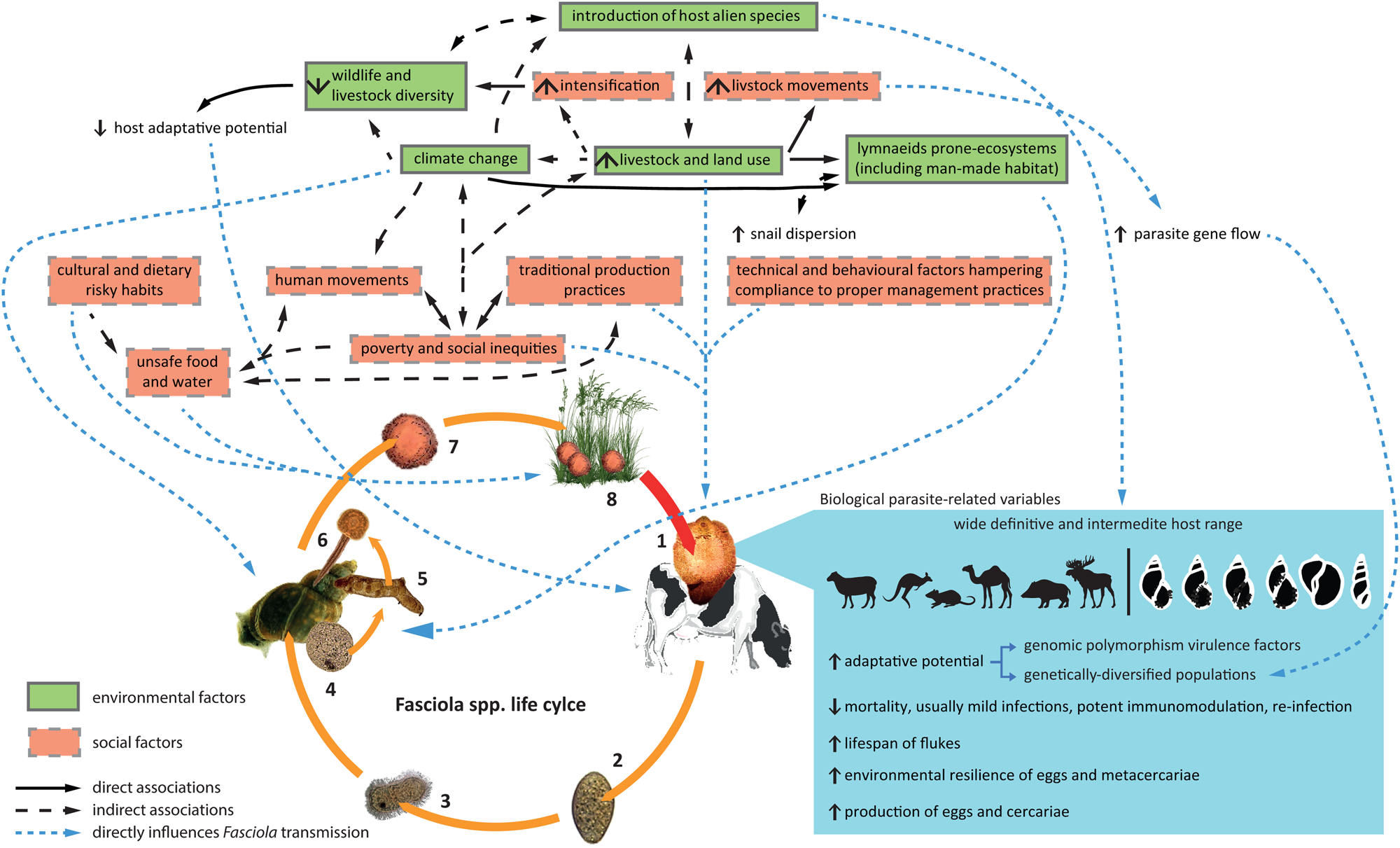
Fig. 2. Integrative diagram showing the interrelation between biological (parasite-related), environmental and social factors directly influencing the different components participating in Fasciola spp. transmission. (1) Fasciola adult in the definitive host, (2) egg, (3) miracidium, (4, 5, 6) sporocyst development, and rediae and cercariae production in the snail host, (7) metacercariae formation following cercariae encystation, (8) water and vegetation contaminated with metacercariae.
Scientific and political factors: changing the view from sporadic diseases in humans to a significant (re)emergent public health problem
Scientific and public health awareness
Archaeological studies showing adult fragments and eggs of Fasciola within an Egyptian mummy (David, Reference David1997) indicate the historical incidence of human fasciolosis. Nevertheless, even when records of the parasite in humans became more evident after their description in the 14th century, fasciolosis was traditionally (and unfortunately still is) considered a veterinary disease. For instance, whereas infection of human European population with Fasciola parasites during medieval and Renaissance periods is accountable today through paleoparasitological studies (Graff et al., Reference Graff, Bennion-Pedley, Jones, Ledger, Deforce, Degraeve, Byl and Mitchell2020), by late 19th century, the following statement by Wilson (Reference Wilson1879): ‘cases of its occurrence as a human parasite were by no means plentifully met with either in purely medical records on the one hand, or in helminthological treatises on the other’, clearly reflects that fasciolosis has been largely overlooked in humans. However, in the 20th century, this zoonosis began to acquire a new connotation within the scientific community due to indistinctive reports of significant numbers of infected people and/or epidemic outbreaks from different regions of the world. Some registered records are, for example, four outbreaks in Cuba between 1943 and 1983 with over 2000 people infected (Rojas et al., Reference Rojas, Vázquez, Domenech and Robertson2010), around 10 000 diagnosed cases between 1956 and 1982 (Gaillet, Reference Gaillet1983) and one epidemic outbreak in 1989 in France (Chandenier et al., Reference Chandenier, Bastard, Merioua, Combes and Thiebault1989), or a major outbreak in North Iran in 1988 affecting around 10 000 people (Rokni, Reference Rokni2008).
In 1990, Chen and Mott extensively reviewed the existing reports of clinical cases of human fasciolosis over a 20-year period (1970–1990) computing, for the first time, a worldwide prevalence of 2594 built on data from 42 countries of all continents (Chen and Mott, Reference Chen and Mott1990). This investigation was highly significant as it redirected the attention towards human fasciolosis at a global scale and discussed the possibility of a higher overlooked impact on human populations. It raised awareness of fasciolosis in terms of its public health impact and preconized the (re-)emergence of fasciolosis in temperate and tropical regions of the world, triggering growth in epidemiological studies and a consequential drastic increase in the rate of case reports in the literature (Parkinson et al., Reference Parkinson, Dalton, O'Neill, Palmer, Soulsby, Torgerson and Brown2011).
In parallel, the 1990s were characterized by an expansion of public health policies led by the World Health Organization (WHO) on diseases such as malaria, filariasis, lepra but also on other less notorious tropical diseases. Such policies promoted a greater emphasis on the provision of assistance for research and control in endemic countries, encouraging biomedical, social and science research institutions to devote greater attention to tropical diseases (World Health Organization, 1990). Particularly among these diseases, the significance of food-borne trematodoses was recognized in 1993 when a Study Group on the Control of Food-borne Trematode Infections held a debate about the burden of food-borne diseases and called for collaboration and potential strategies for their control. The report of the meeting officially recognized human fasciolosis, enlisted as part of the food-borne trematodoses, as a cosmopolitan infection. With an expanding distribution and clinical cases reported from 61 countries, a worldwide estimation of over 1.9 million people infected with fasciolosis was calculated but only counting the potential burdens of six countries: Bolivia, Ecuador, Peru, Egypt and Iran (World Health Organization, 1995). The WHO considered fasciolosis, along with the rest of food-borne trematode infections, as an emerging human disease of increasing impact and a severe public health problem ‘that made action imperative’. From this point forward, the WHO encouraged the establishment of integrative control programmes alongside agricultural and public health strategies, particularly within endemic countries, as well as the collection, monitoring and surveillance of epidemiological data at a global scale (World Health Organization, 1995). With more inclusive assessments, global prevalence quickly rose to 2.4 million (Rim et al., Reference Rim, Farag, Sornmani and Cross1994) or to 17 million (Hopkins, Reference Hopkins1992), and up to 50 or 72 million if underestimation is accounted for (Nyindo and Lukambagire, Reference Nyindo and Lukambagire2015), whereas 180 million people are currently considered to live at risk (World Health Organization, 2013). The lack of extensive public awareness of the transmission and impact of fasciolosis, the gaps in knowledge about the distribution and epidemiology of food-borne infections, the poorly assessed biology and ecology of the snails hosts and the particular features of the disease caused by the parasites were soon considered major constraints for the feasibility of control strategies (World Health Organization, 1995).
Additionally, in 1992, the Food and Agriculture Organization (FAO) also reviewed the geographic distribution of helminth infections (including Fasciola parasites) in livestock in developing countries based on the selection of relevant data from the literature published during the previous 15 years. Given the accumulated material, it considered liver fluke infections 'as an important regional threat to animal production in many developing countries’ (Over et al., Reference Over, Jansen and van Olm1992). Furthermore, with fasciolosis showing the greatest distribution of all zoonotic trematode infections and with predictably negative effects on the productivity of animals and in human health as a result of massive infection, FAO stated ‘that control measures should be introduced immediately’ (Over et al., Reference Over, Jansen and van Olm1992).
The 2000s have been characterized by a ‘momentum’ of the so-called neglected tropical diseases (NTD). Since then, largely ignored diseases have been given priority in the global health agenda. The first meeting of WHO on the control of NTD held in 2005 emphasized the urgency of gathering evidence and gaining understanding of particular NTDs including fasciolosis and other foodborne trematodoses (World Health Organization, 2011). These meetings also led to increased collaboration and concerted efforts with FAO in the development and research of common strategies and investments of the zoonoses. Such actions set the example for the need for coordinated and synergistic actions between animal and human health sectors when dealing with infections like fasciolosis to achieve ‘One Health’. Overall, the increasing scientific awareness and research evidence served as a call for attention to settle fasciolosis within the global landscape as a significant disease, and to stimulate research on Fasciola and fasciolosis.
To illustrate the worldwide interest in fasciolosis within the scientific community, we carried out a rapid search on Scopus and PubMed databases. We used (i) ‘Fasciola OR Fasciolosis OR Fascioliasis’ as the basic search criteria, and we also complemented it with: (ii) AND human, (iii) AND ruminants, (iv) AND epidemiology, (v) AND survey, (vi) AND diagnostic method. The compiled data evidence a growing trend of scientific interest and commitment that started rising in the 1960s and saw an exponential increase at the beginning of 2000s which continues to be present today (see Fig. 3A and B). The enhanced scientific production experienced in the last decades indirectly shows the interest from funding agencies and investors that followed the inclusion of fasciolosis in the international health agenda. A special mention ought to be given to the growing percentage of research within Fasciola and fasciolosis devoted to epidemiological surveys and detection methods (Fig. 3C and D). Given the long pre-patent period and normally insidious sub-clinical nature of the disease in the definitive hosts and the sensitivity issues of traditional parasitological diagnosis, these surveys and methods have significantly contributed to identifying endemic areas that were previously neglected.
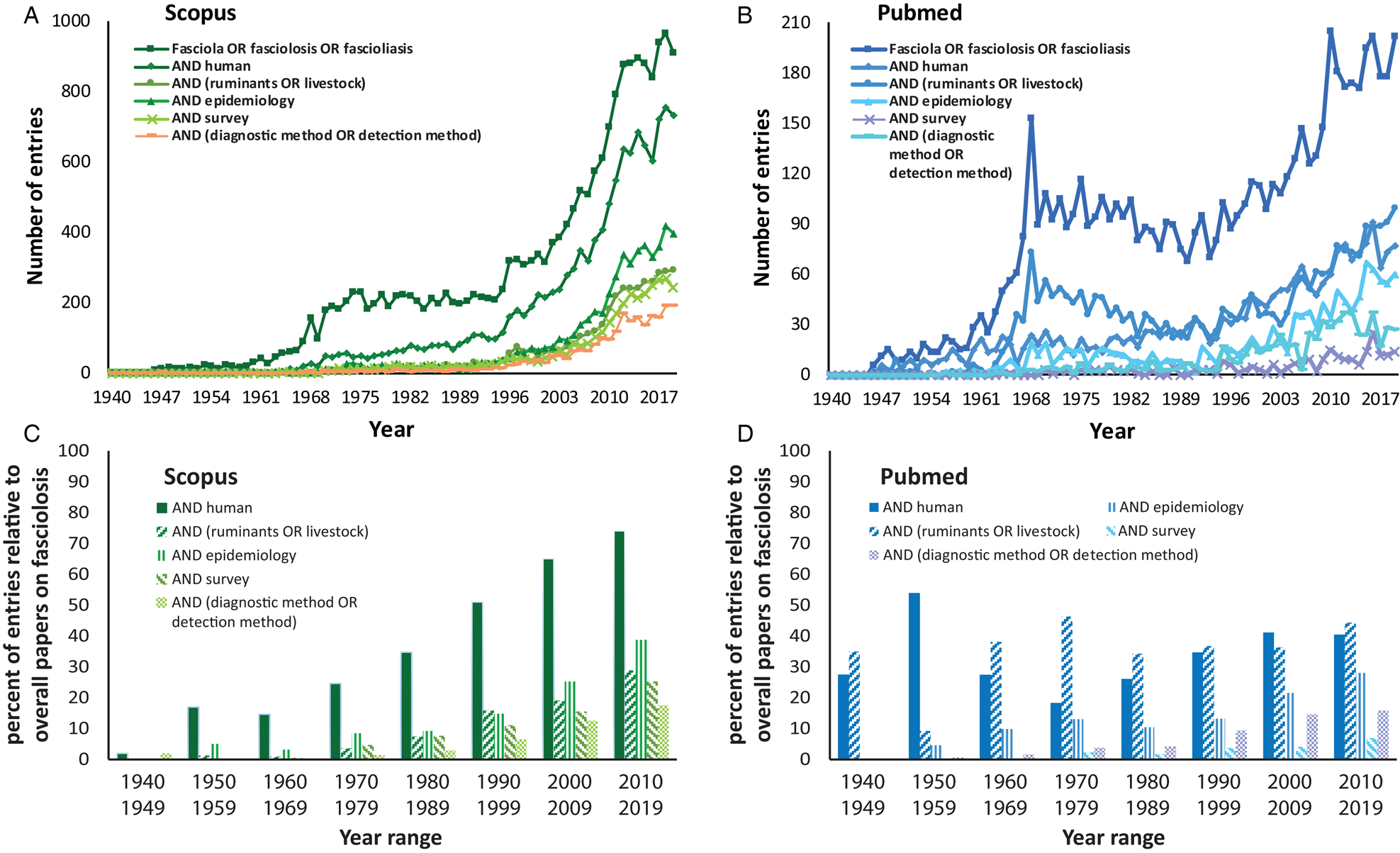
Fig. 3. The data compilation from searching results on Scopus (A, C) and Pubmed (B, D) databases using (i) Fasciola OR Fasciolosis OR Fascioliasis as basic search criterium, and complemented it with: (ii) AND human, (iii) AND ruminants OR livestock, (iv) AND epidemiology, (v) survey, (vi) diagnostic method OR detection method. (A, B) The absolute number of entries per year, between 1940 and 2019, for each search criteria. (C, D) The percentage of entries for each complementary criterion (from ii to vi) over the total of entries corresponding to the basic search criterion (i) in 10-year intervals.
Improved detection and increased surveys
The finding of Fasciola eggs in stool and/or adults in liver, gall bladder or biliary ducts is the classical and most widely used principle to diagnose infection and thus, it has been a significant contributor to prevalence assessments. However, parasitological detection has numerous drawbacks inherent to the different methods available, to the biology of Fasciola parasites, the progression of the infection and to the different epidemiological situations (see Mas-Coma et al., Reference Mas-Coma, Bargues and Valero2014 for review). Therefore, the incorporation of immunoassays, whether serological methods since the 1980s such as in-house methods (Chen and Mott, Reference Chen and Mott1990) or commercial variants (e.g. Fas2-ELISA: Espinoza et al., Reference Espinoza, Maco, Marcos, Saez, Neyra, Terashima, Salmavides, Gotuzzo, Chavarry, Huaman, Bargues, Valero and Mas-Coma2007; Svanovir®: Charlier et al., Reference Charlier, De Cat, Forbes and Vercruysse2009), and of Fasciola antigen capture-based immunodetection since the 1990s (e.g. FasciDig: Espino et al., Reference Espino, Díaz, Pérez and Finlay1998; Zumaquero-Ríos et al., Reference Zumaquero-Ríos, Sarracent-Pérez, Rojas-García, Rojas-Rivero, Martínez-Tovilla, Valero and Mas-Coma2013; MM3-ELISA: Mezo et al., Reference Mezo, González-Warleta, Carro and Ubeira2004; Ubeira et al., Reference Ubeira, Muiño, Valero, Periago, Pérez-Crespo, Mezo, González-Warleta, Romarís, Paniagua, Cortizo, Llovo and Mas-Coma2009), has allowed faster, more sensible and complementary diagnoses of fasciolosis. This has proven to be particularly useful in non-endemic or hypo-endemic areas and/or for large-scale surveys (Mas-Coma et al., Reference Mas-Coma, Bargues and Valero2014). Lastly, the expansion of molecular techniques in the 2000s added a new dimension to the screening of fasciolosis transmission. Molecular tools solve the parasitological method's drawbacks of sensitivity and specificity, allow to discriminate both Fasciola species and the hybrid forms in a reliable way and to characterize genetically the different parasite and host populations (Martínez-Valladares and Rojo-Vázquez, Reference Martínez-Valladares and Rojo-Vázquez2016; Amor et al., Reference Amor, Farjallah, Merella, Alagaili and Mohammed2020; Moghadamizad et al., Reference Moghadamizad, Hosseini-Safa, Mohebali, Heydarian, Aryaeipour and Rokni2020).
Different techniques within each type of non-classical detection method have been applied indistinctively in the monitoring of infection within definitive hosts such as ELISAs (Espino et al., Reference Espino, Díaz, Pérez and Finlay1998), rapid immunochromatographic tests (Xifeng et al., Reference Xifeng, Mengfan, Kai, Guowu, Jing, Lixia, Jun, Qingling, Shasha, Yunfu and Xuepeng2019), conventional PCR, loop-mediated isothermal amplification (Martínez-Valladares and Rojo-Vázquez, Reference Martínez-Valladares and Rojo-Vázquez2016) and high-resolution melting analysis (Moghadamizad et al., Reference Moghadamizad, Hosseini-Safa, Mohebali, Heydarian, Aryaeipour and Rokni2020). In addition, detection of snail infections also evolved from the classical parasitological dissection to immunoenzymatic assays (Alba et al., Reference Alba, Hernández, Marcet, Vázquez, Figueredo, Sánchez, Otero and Sarracent2015a) and molecular methods (multiplex PCR, Alba et al., Reference Alba, Vázquez, Hernández, Sánchez, Marcet, Figueredo, Sarracent and Fraga2015b; real-time PCR, Schweizer et al., Reference Schweizer, Meli, Torgerson, Lutz, Deplazes and Braun2007; etc.). All these techniques have demonstrated improved diagnostic sensitivity and specificity (see e.g. Zumaquero-Ríos et al., Reference Zumaquero-Ríos, Sarracent-Pérez, Rojas-García, Rojas-Rivero, Martínez-Tovilla, Valero and Mas-Coma2013; Alba et al., Reference Alba, Vázquez, Sánchez, Fraga, Martínez, Hernández, Marcet, Figueredo and Sarracent2016). Furthermore, other types of samples beyond blood, stool (definitive host) and snails (intermediate host) have been added to the panel of possibilities for active screening; from meat juice and bulk milk for serological assessment in livestock (Charlier et al., Reference Charlier, De Cat, Forbes and Vercruysse2009; Villa-Mancera and Reynoso-Palomar, Reference Villa-Mancera and Reynoso-Palomar2019) to water for environmental DNA detection of the parasite and its snail hosts (Jones et al., Reference Jones, Brophy, Davis, Davies, Emberson, Rees Stevens and Williams2018; Davis et al., Reference Davis, Tyson, Cutress, Davies, Jones, Brophy, Prescott, Rose, Williams, Williams and Jones2020).
Most of these newly developed methods have been implemented in epidemiological surveys during scientific investigations, and the conjunction of both factors has undoubtedly contributed to building up knowledge on fasciolosis burden in specific areas and its transmission by the different host systems. As the occurrence of most human endemic areas within poor rural populations is usually characterized by low attendance to health centres, frequent co-infection with other diseases, and a tricky diagnosis if it is based solely on clinical description, in the absence of sounded symptoms and/or active screening with sensitive methods, fasciolosis could go unnoticed. As reviewed by Chen and Mott (Reference Chen and Mott1990), before 1990, most scientific reports of human fasciolosis consisted of a small series of acute clinical cases seeking hospital attention. Furthermore, the highest numbers were reported from countries with strengthened public health systems and scientific tradition (e.g. France, Spain, Portugal; Chen and Mott, Reference Chen and Mott1990). The authors alerted about the possibility of a serious underestimation of the global infection as only a few low-scale community-based surveys carried out at the time and applying serological screenings (in Peru, France, Portugal, Egypt and Puerto Rico) demonstrated improved capacity for assessing the impact of the disease (Chen and Mott, Reference Chen and Mott1990). In this sense, a meta-analysis of epidemiological surveys from 38 communities in the Bolivian Altiplano established that fasciolosis is endemic there since at least 1984 (Parkinson et al., Reference Parkinson, O'Neill and Dalton2007). However, it was not until extensive surveys were conducted in the 1990s that it was pinpointed as a significant hot spot where the highest human prevalence was and continue to be reported (Esteban et al., Reference Esteban, Flores, Angles and Mas-Coma1999; Mas-Coma et al., Reference Mas-Coma, Anglés, Esteban, Bargues, Buchon, Franken and Strauss1999; Parkinson et al., Reference Parkinson, O'Neill and Dalton2007). Later, other significant endemic foci of human fasciolosis have been also found based on large-scale or community-based epidemiological studies, for example, in regions within Egypt (Esteban et al., Reference Esteban, Gonzalez, Curtale, Muñoz-Antoli, Valero, Bargues, Mabrouk, el-Wakeel, Abdel-Wahab, Montresor, Engels, Savioli and Mas-Coma2003; Curtale et al., Reference Curtale, Hassanein, Barduagni, Yousef, Wakeel, Hallaj and Mas-Coma2007), Iran (Sarkari et al., Reference Sarkari, Ghobakhloo, Moshfea and Eilami2012), Peru (Esteban et al., Reference Esteban, Gonzalez, Bargues, Angles, Sanchez, Naquira and Mas-Coma2002) and Mexico (Zumaquero-Ríos et al., Reference Zumaquero-Ríos, Sarracent-Pérez, Rojas-García, Rojas-Rivero, Martínez-Tovilla, Valero and Mas-Coma2013).
It should be mentioned that even though efforts have been increased, extensive epidemiological studies, especially in high-risk communities, remain scarce in most countries and the overall rates of infection are still being extrapolated from sporadic reports of cases or outbreaks (Parkinson et al., Reference Parkinson, Dalton, O'Neill, Palmer, Soulsby, Torgerson and Brown2011). Factors such as: (i) fasciolosis can cause mild symptoms or go unnoticed for a long time; (ii) current diagnostic tools have sensitivity, reproducibility or availability and cost-associated issues; (iii) it is a disease of non-obligatory declaration in many countries; and (iv) coordinated and updated epidemiological information is usually scarce especially in low-income countries, imply that the real number of animals and humans infected with fasciolosis in some countries is presumed to be higher than their reports (Toet et al., Reference Toet, Piedrafita and Spithill2014). At present, worldwide disease prevalence is still uncertain (Parkinson et al., Reference Parkinson, Dalton, O'Neill, Palmer, Soulsby, Torgerson and Brown2011) and concerns on the real extent of human fasciolosis in certain geographical areas such as Argentina (Mera y Sierra et al., Reference Mera y Sierra, Agramunt, Cuervo and Mas-Coma2011), Nepal (Sah et al., Reference Sah, Khadka, Lakhey, Pradhan, Shah, Singh and Mas-Coma2018) and China (Chen et al., Reference Chen, Chen, Ai, Xu, Jiao, Zhu, Su, Zang, Luo, Guo, Lv and Zhou2013) have been augmenting.
Realities and challenges towards the future
Herein we have exposed the different factors affecting fasciolosis dynamics and incidence in the context of its current (re)emergence but it is difficult to say with certainty up to when, where and for how long this trend will continue in the future. Global livestock population is predicted to almost double by 2050, especially in low- and middle-income countries and the intensification of livestock production in the humid and subhumid grazing systems of the world is also projected to increase (Thornton, Reference Thornton2010). The relationship between livestock production–environmental degradation–climate change (especially under poor management practices) may continue to do so in the near future. However, it is important to note that the effects of climate change are not homogeneous and straightforward in all regions and they are not altogether necessarily enhancers of transmission (see Mas-Coma et al., Reference Mas-Coma, Valero and Bargues2009b). Therefore, while it may be assumed that the current climate change has contributed to the overall spreading of fasciolosis and increasing prevalence in certain regions, a constant trend and a global homogeneous pattern cannot, by any chance, be expected. Therefore, regional projections and screenings must be put in place to assess the future impact of fasciolosis in different areas.
In addition, the increase in livestock production could translate into economic growth. However, economic growth without consideration for social inequality does not automatically translate into human development. Therefore, poverty risks must be always considered because fasciolosis as other infectious diseases usually affects in low-income regions the most. This increased risk further constrains development at different levels (e.g. economic, environmental, social), reinforcing social inequities, environmental degradation and unsustainable exploitation of resources and condemning the population to a vicious cycle that perpetuates these very same socio-economic, ecological and epidemiological conditions. It should be mentioned that fasciolosis is a disabling disease (Torgerson et al., Reference Torgerson, Devleesschauwer, Praet, Speybroeck, Willingham, Kasuga, Rokni, Zhou, Fèvre, Sripa, Gargouri, Fürst, Budke, Carabin, Kirk, Angulo, Havelaar and de Silva2015) and, whilst all age groups can be affected, in human hyperendemic areas, children appear to be the most infected (Esteban et al., Reference Esteban, Gonzalez, Bargues, Angles, Sanchez, Naquira and Mas-Coma2002, Reference Esteban, Gonzalez, Curtale, Muñoz-Antoli, Valero, Bargues, Mabrouk, el-Wakeel, Abdel-Wahab, Montresor, Engels, Savioli and Mas-Coma2003; Zumaquero-Ríos et al., Reference Zumaquero-Ríos, Sarracent-Pérez, Rojas-García, Rojas-Rivero, Martínez-Tovilla, Valero and Mas-Coma2013). Hence, it directly constrains the development of the youngest generation.
With fasciolosis (re-)emergence and a growing livestock industry, the lack of vaccines places immediate pressure on the use of anthelmintic treatments to control F. hepatica infection leading to a significant problem to consider for the near future, such as parasite resistance (Kelley et al., Reference Kelley, Elliott, Beddoe, Anderson, Skuce and Spithill2016). Indeed, numerous reports of parasite resistance to triclabendazole, albendazole and clorsulon in infected livestock are growing in different regions of the world (Kelley et al., Reference Kelley, Elliott, Beddoe, Anderson, Skuce and Spithill2016; Fairweather et al., Reference Fairweather, Brennan, Hanna, Robinson and Skuce2020), whereas the report of closantel failure (Novobilský and Höglund, Reference Novobilský and Höglund2015) is an additional wakeup call. The list of effective drugs to treat animal fasciolosis and decrease parasite circulation in nature may indeed quickly grow thinner. Even when the availability of treatment, and hence, the selective pressure, is expected to be higher in high-income countries, the worldwide trading of livestock and the F. hepatica–F. gigantica hybridization leave no region out of risk. Treatment failure with triclabendazole in human patients is already being documented in developed (Branco et al., Reference Branco, Ruas, Nuak and Sarmento2020; in Portugal) as well as developing countries (Cabada et al., Reference Cabada, Lopez, Cruz, Delgado, Hill and White2016; in Peru).
Other tendencies must be considered as they might become significant in increasing transmission risks, particularly in high-income regions where environmental education is usually higher. In this sense, future trends of livestock industry, such as those comprising the increase in intensification of pastoral animal production and organic ruminant production, will have positive and negative effects on animal welfare, including disease susceptibility. Here, the balance will be determined by the quality of management and stockmanship, as well as by the pressure on businesses to be profitable (Stafford and Gregory, Reference Stafford and Gregory2008; Takeuchi-Storm et al., Reference Takeuchi-Storm, Moakes, Thüer, Grovermann, Verwer, Verkaik, Knubben-Schweizer, Höglund, Petkevičius, Thamsborg and Werne2019). Concerning infection sources to humans through wild vegetables, whether directly collected from the field or from in-house gardens or acquired in urban markets, the recent drive to ‘go green’ as a healthy approach to the modern lifestyle in today's developed societies poses evident problems as it underlies an increase in the consumption of fresh, raw/green fruits and vegetables (Mas-Coma et al., Reference Mas-Coma, Bargues and Valero2018).
The complexity and heterogeneity of Fasciola spp. transmission make it difficult to know and forecast its burden with certainty across different regions. In addition, four aspects offer serious problems for the analysis of the epidemiological situations of this disease in the different countries; (i) diagnosis is still tricky in both developed and developing countries; (ii) as awareness of the disease in non-endemic areas and/or access to health or sensitive detection methods need to be improved in primary health centres (see e.g. Ramachandran et al., Reference Ramachandran, Ajjampur, Chandramohan and Varghese2012; Perrodin et al., Reference Perrodin, Walti, Gottstein, Kim-Fuchs, Candinas and Banz2019); (iii) fasciolosis' usually mild or unspecific symptomatology; and (iv) the classification of fascioliasis as a non-reportable disease (Mas-Coma et al., Reference Mas-Coma, Bargues and Valero2014, Reference Mas-Coma, Bargues and Valero2018). Regional and international awareness remains fundamentally biased if human infections go unreported, keeping an underestimation of the real situation, about the risky areas and sources for human infection and, with it, deprioritizing much needed multi-sectoral approaches to control transmission.
Concluding remarks
Some zoonotic parasitic infections such as fasciolosis do not enjoy great notoriety among the general population, as they are far less understood and mostly unknown or forgotten. Regardless, they significantly affect or threat not only the poor long-neglected populations but also high-income countries. This review illustrates the different aspects involved in the transmission of fasciolosis and its current increasing burden within veterinary medicine and public health worldwide. It further demonstrates how interconnected biological, environmental and social factors have shaped the epidemiology of human and animal fasciolosis and how the shifting trends in scientific awareness and health policies have been pivotal to recognize the phenomenon of fasciolosis (re-)emergence.
Whereas simplification is usually essential to approach scientific topics, the complexity of fasciolosis and the numerous interacting aspects influencing transmission implies that all the different factors should be always pondered. Hypo-, meso- and hyperendemic regions of animal and human fasciolosis occur even within countries (Mas-Coma et al., Reference Mas-Coma, Bargues and Valero2009a), and such heterogeneity is determined by differences at biological, environmental, social and/or political levels. Considering them all at the view of each epidemiological context would be the best initial approximation to comprehend fasciolosis impact, to forecast parasite circulation and to successfully act upon it. Finally, through the integrative overview of the present review, the bridge among human and animal health and the environment becomes evident and, with it, the necessity to bring all these elements together in a single platform, i.e. One Health approach, to conduct interdisciplinary, collaborative, and socially relevant research and to develop control strategies that efficiently tackle fasciolosis and its (re-)emergence.
Acknowledgements
The authors would like to thank Dr Luis Fonte for insightful comments. We thank Dr Camila Perera for proofreading the manuscript. We are also grateful to the editor and two anonymous reviewers for proper corrections, suggestions and remarks that greatly helped to improve the manuscript.
Financial support
None.
Conflict of interest
None.