Introduction
Trichomonas vaginalis is the parasitic protozoan causing the sexually transmitted infection (STI) trichomoniasis. Trichomoniasis is the most common non-viral STI affecting an estimated 3.7 million U.S. women and men and 143 million people worldwide (Rowley et al., Reference Rowley, Toskin and Ndowa2012; Newman et al., Reference Newman, Rowley, Vander Hoorn, Wijesooriya, Unemo, Low, Stevens, Gottlieb, Kiarie and Temmerman2015). It is more common in women, particularly older women (Sutton et al., Reference Sutton, Sternberg, Koumans, McQuillan, Berman and Markowitz2007; Patel et al., Reference Patel, Gaydos, Packman, Quinn and Tobian2018). In addition, there is a pronounced racial disparity with African Americans having a higher prevalence than other racial groups (Patel et al., Reference Patel, Gaydos, Packman, Quinn and Tobian2018). Trichomoniasis is frequently asymptomatic in women; in a nationally representative sample of U.S. women aged 14–49 with T. vaginalis infection, only 9.5% reported vaginal discharge, 7.3% reported vaginal itch and 7.0% reported vaginal odour (Sutton et al., Reference Sutton, Sternberg, Koumans, McQuillan, Berman and Markowitz2007). Among women who are symptomatic, signs and symptoms may include a thin, frothy vaginal discharge, odour, genital inflammation, pruritus, dysuria and/or dyspareunia (Wolner-Hanssen et al., Reference Wolner-Hanssen, Krieger, Stevens, Kiviat, Koutsky, Critchlow, DeRouen, Hillier and Holmes1989; Swygard et al., Reference Swygard, Sena, Hobbs and Cohen2004). Trichomonas vaginalis is usually asymptomatic in men (Sena et al., Reference Sena, Miller, Hobbs, Schwebke, Leone, Swygard, Atashili and Cohen2007), although it can cause penile discharge and/or dysuria in the setting of non-gonococcal urethritis (Schwebke et al., Reference Schwebke, Rompalo, Taylor, Sena, Martin, Lopez, Lensing and Lee2011). It may also interfere with male and female fertility, although few studies have been conducted to directly investigate this (Meites et al., Reference Meites, Gaydos, Hobbs, Kissinger, Nyirjesy, Schwebke, Secor, Sobel and Workowski2015). Infected women and men with T. vaginalis also have an increased risk of acquisition and transmission of HIV (Kissinger and Adamski, Reference Kissinger and Adamski2013) whereas pregnant women have an increased risk of preterm delivery and other adverse birth outcomes (Silver et al., Reference Silver, Guy, Kaldor, Jamil and Rumbold2014).
Currently approved treatments for trichomoniasis include metronidazole (MTZ) and tinidazole (TDZ); 5-nitroimidazoles (WHO, 2017). Other drugs in this class include secnidazole (SEC), nimorazole, ornidazole and azanidazole. SEC is used outside the USA to treat T. vaginalis (Rossignol et al., Reference Rossignol, Maisonneuve and Cho1984), as well as other infections including Helicobacter pylori and Giardia lamblia (Ahuja et al., Reference Ahuja, Dhar, Bal and Sharma1998; Escobedo et al., Reference Escobedo, Canete, Gonzalez, Pareja, Cimerman and Almirall2003). SEC is currently FDA-approved for the treatment of bacterial vaginosis in the USA but not for trichomoniasis (Schwebke et al., Reference Schwebke, Morgan, Koltun and Nyirjesy2017). A phase 3 clinical trial to evaluate the safety and effectiveness of a single dose of SEC for the treatment of trichomoniasis in women is ongoing (NCT03935217).
MTZ is the 5-nitroimidazole most commonly used in clinical practice for Trichomonas treatment, as it is generic and inexpensive. MTZ was first introduced in 1959 (Cosar and Julou, Reference Cosar and Julou1959; Durel et al., Reference Durel, Couture, Collart and Girot1960); in vitro resistance in T. vaginalis was observed by 1962 (Robinson, Reference Robinson1962). Trichomonads with minimal lethal concentrations (MLCs) ≤25 μg mL−1 are considered MTZ-sensitive. An MLC of 50 μg mL−1 represents low-level resistance, MLCs of 100–200 μg mL−1 moderate-level resistance and MLCs ≥400 μg mL−1 high-level resistance. The prevalence of resistance to MTZ and TDZ ranges between 2.2 and 9.6% (Schmid et al., Reference Schmid, Narcisi, Mosure, Secor, Higgins and Moreno2001; Schwebke and Barrientes, Reference Schwebke and Barrientes2006; Krashin et al., Reference Krashin, Koumans, Bradshaw-Sydnor, Braxton, Evan Secor, Sawyer and Markowitz2010; Kirkcaldy et al., Reference Kirkcaldy, Augostini, Asbel, Bernstein, Kerani, Mettenbrink, Pathela, Schwebke, Secor, Workowski, Davis, Braxton and Weinstock2012) and 0 and 2% (Perez et al., Reference Perez, Fernandez-Verdugo, Perez and Vazquez2001; Schwebke and Barrientes, Reference Schwebke and Barrientes2006), respectively. Resistance to MTZ is associated with cross-resistance to other 5-nitroimidazoles. 5-Nitroimidazole-resistant trichomoniasis is concerning, as few alternative treatments exist.
To better understand treatment failure due to 5-nitroimidazole drug resistance, we performed a systematic literature review on mechanisms of 5-nitroimidazole resistance in T. vaginalis. An improved understanding of this resistance may inform development of novel therapeutic options outside of the 5-nitroimidazole class.
Methods
A systematic review of the literature focusing on original research on mechanisms of 5-nitroimidazole resistance in T. vaginalis was performed between January and August 2019 by authors KJG and PJK. The review included articles ranging from the introduction of MTZ as a treatment for trichomoniasis in 1959 to August 2019. The databases used included PubMed, ScienceDirect and EMBASE. Keywords were 5-nitroimidazole, metronidazole, tinidazole, secnidazole, drug resistance, Trichomonas vaginalis and trichomoniasis. Reference lists from selected publications identified by these keywords were also reviewed to identify any additional articles that may be relevant.
Evaluated articles had to contain original research on mechanisms of 5-nitroimidazole resistance in T. vaginalis. This included how resistance can arise, descriptions of proteins and enzymes involved in metabolic pathways affecting drug activation, enzymes altered in drug-resistant T. vaginalis isolates and whether or not clinical resistance was relative or absolute. Research aimed at describing genetic components of resistance, i.e. point mutations leading to inactivation or dysfunction of an enzyme involved in drug activation and other metabolic processes affecting resistance were also considered. Non-English language articles, case reports, case series or reviews were excluded. Research articles involving treatment of other microorganisms besides T. vaginalis, articles describing non-5-nitroimidazole treatments for T. vaginalis and articles focusing solely on susceptibility testing and prevalence of resistance were also excluded.
If it was unclear whether an article was appropriate for inclusion, author CAM assisted in an additional assessment. An Excel spreadsheet was created during the database search that contained all the papers that met the inclusion criteria. The spreadsheet was subsequently made available to all authors for analysis of the relevant literature.
Results
The search yielded 606 articles: 275 from EMBASE, 142 from PubMed and 189 from ScienceDirect (Fig. 1). Of these 606 articles, 550 were excluded for various reasons listed in Fig. 1. Thus, 58 articles relevant to 5-nitroimidazole resistance mechanisms in T. vaginalis were included in this review.
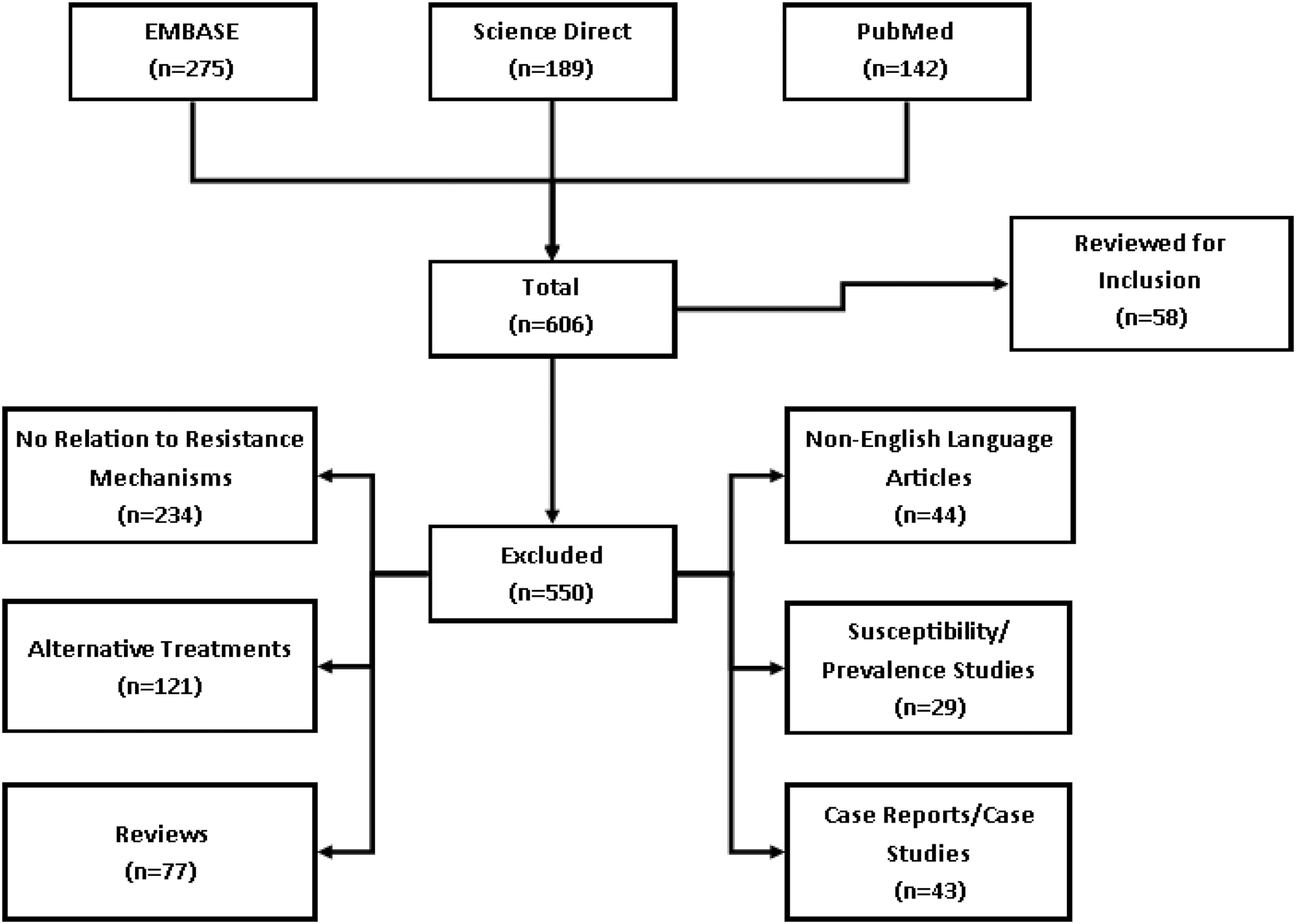
Fig. 1. Flow chart. Flow chart of the databases used to perform the systematic review of the literature. Included are the number of articles reviewed for study inclusion and those that were excluded for various reasons.
Metabolic pathways of T. vaginalis and their effects on 5-nitroimidazole resistance
Energy production pathways in T. vaginalis
Trichomonas vaginalis obtains most of its energy through carbohydrate metabolism, which involves breaking down glucose to produce lactate, acetate, molecular hydrogen and adenosine triphosphate (ATP). Malate can also serve as an energy source. Figures 2a and b depict the structure (Honigberg and King, Reference Honigberg and King1964; Honigberg and Brugerolle, Reference Honigberg, Brugerolle and Honigberg1990; Bouchemal et al., Reference Bouchemal, Bories and Loiseau2017) and energy production pathways of T. vaginalis. One pathway begins in the cytoplasm where glucose undergoes glycolysis to form pyruvate, which is then passively transported into hydrogenosomes (Fig. 2a). Inside the hydrogenosome (Fig. 2b), the enzyme pyruvate:ferredoxin oxidoreductase (PFOR) facilitates oxidation (loss of electrons) of pyruvate by ferredoxin (Fdx) to produce acetyl-CoA. The reduced Fdx is oxidized by the enzyme hydrogenase (HYD), which uses the electrons gained from Fdx to produce molecular hydrogen (H2). Figure 2b depicts a second energy production pathway in the hydrogenosomes that uses malate (Dolezal et al., Reference Dolezal, Vanacova, Tachezy and Hrdy2004; Hrdy et al., Reference Hrdy, Cammack, Stopka, Kulda and Tachezy2005). This pathway utilizes malate dehydrogenase (MDH) and nicotinamide adenine dinucleotide:ferredoxin oxidoreductase (NADH:FOR). Malate is decarboxylated by MDH to yield pyruvate and CO2, transferring electrons to NAD+ whereas pyruvate enters the first pathway to produce acetyl-CoA and ATP. Similar to PFOR, NADH:FOR facilitates the transfer of electrons to Fdx, allowing the reaction to proceed to H2 production. ATP and acetate production in the hydrogenosomes occurs through a substrate-level phosphorylation catalysed by two enzymes: acetate:succinate CoA-transferase (ASCT) and succinyl-CoA synthetase (SCS), called the ASCT/SCS cycle (Fig. 2b; pathway 3).

Fig. 2. MTZ activation through the energy production pathway in T. vaginalis. (a) Overview of T. vaginalis and the MTZ drug activation pathway. (1) MTZ enters the cell by passive diffusion and is subsequently metabolized in the hydrogenosome. (2) Pyruvate is produced from glucose through glycolysis in the cytosol. (3) Inside the hydrogenosome, PFOR facilitates oxidative decarboxylation of pyruvate by reducing and transferring electrons to Fdx. Fdx then reduces the nitro group of MTZ, which creates a cytotoxic nitro radical anion. (4) Activated MTZ then interacts with T. vaginalis DNA causing damage and death of the organism. (b) Detailed energy production and MTZ drug activation pathway in the hydrogenosome. Solid line: Major energy production pathway: Pyruvate is the major intermediate product produced from glucose through the glycolytic pathway (pathway 1) as well as through an alternative malate-dependent pathway (pathway 2). PFOR then transfers electrons from pyruvate to the electron acceptor Fdx, producing acetyl-CoA, which is through ASCT/SCS cycle reduced to acetate, producing ATP (pathway 3). The reduced Fdx is used to produce H2 by the HYD enzyme. Dashed line: Drug activation pathway – Once MTZ enters the hydrogenosome, it competes with HYD for the electron carrying Fdx. MTZ is reduced to a nitro radical anion that interacts with T. vaginalis DNA causing damage and death of the organism. PFOR, pyruvate:ferredoxin oxidoreductase; Fdx, ferredoxin; HYD, hydrogenase; H2, hydrogen; CO2, carbon dioxide; MTZ, metronidazole; MDH, malate dehydrogenase; NADPH, nicotinamide adenine dinucleotide phosphate; NADH:FOR, nicotinamide adenine dinucleotide:ferredoxin oxidoreductase; PEPCK, phosphoenolpyruvate carboxykinase; GTP/GDP, guanosine triphosphate/guanosine diphosphate; PDC, pyruvate decarboxylase; LDH, lactate dehydrogenase; ADH, alcohol dehydrogenase; ASCT, acetate:succinate CoA-transferase; SCS, succinyl CoA synthetase; OX, oxidized; RED, reduced.
Antioxidant defense pathway in T. vaginalis
The antioxidant defense pathway takes place in the cytosol of T. vaginalis. Figure 3a illustrates this pathway under normal conditions, i.e. in the absence of a 5-nitroimidazole. The reactive oxygen species (ROS), superoxide (O2−), is reduced to molecular oxygen (O2) and hydrogen peroxide (H2O2) by the enzyme superoxide dismutase (SOD). The O2 is then reduced by either nicotinamide adenine dinucleotide (NADH) oxidase to H2O or by flavin reductase 1 (FR1) to H2O2. The H2O2 produced by these processes must be further reduced to avoid its cytotoxic side-effects. This is accomplished indirectly by the activity of the flavin enzyme thioredoxin reductase (TrxR) and its accompanying protein and enzyme thioredoxin (Trx) and thioredoxin peroxidase (TrxP), respectively. TrxR facilitates the reduction of Trx by nicotinamide adenine dinucleotide phosphate (NADPH). Reduced Trx subsequently activates enzymes involved in other metabolic pathways. Importantly, Trx activates TrxP, which then reduces H2O2 to H2O.

Fig. 3. Aerobic resistance to MTZ arises from deficient O2 scavenging pathways. (a) Normal T. vaginalis antioxidant defense pathways – (1) ROS, such as O2−, can be harmful to trichomonads, and multiple pathways can control and ‘neutralize’ ROS. SOD reduces O2− to molecular O2 while also producing water and H2O2. (2) Intracellular O2 can be reduced to H2O2 by FR1 or by (3) NADH oxidase into H2O. The cytotoxic H2O2 produced by these pathways further metabolized by the trichomonad. (4) H2O2 can be indirectly reduced to H2O, through the activation of the flavin-containing enzyme TrxR by NADPH. Electrons from NADPH are transferred from TrxR to Trx, which activates thioredoxin-dependent peroxidases such as TrxP to reduce H2O2 to H2O. (b) Aerobic resistance pathway – (1) TrxR, like Fdx and NTR, can reduce MTZ. Once reduced, MTZ can form covalent adducts with TrxR and Trx. These adducts inhibit the activities of the enzymes, leading to increased levels of cytotoxic H2O2. (2) FR1 and NADH oxidase activities are significantly decreased or absent in MTZ resistant strains. (3) Intracellular O2 levels increase in the absence of normally functioning O2-scavenging pathways, resulting in the inactivation of MTZ from its nitro radical anion in a process known as futile cycling. ROS, reactive oxygen species; O2−, superoxide; SOD, superoxide dismutase; O2, oxygen; H2O2, hydrogen peroxide; FR1, flavin reductase 1; NADH, nicotinamide adenine dinucleotide; TrxR, thioredoxin reductase; NTR, nitroreductase; NADPH, nicotinamide adenine dinucleotide phosphate; Trx, thioredoxin; TrxP, thioredoxin peroxidase; MTZ, metronidazole; OX, oxidized; RED, reduced.
Drug activation of 5-nitroimidazoles in T. vaginalis
MTZ or other 5-nitroimidazoles used for Trichomonas treatment enter the parasites in an inactive form through passive diffusion (Muller, Reference Muller1986). 5-Nitroimidazoles need to be reduced in order to actively interfere with T. vaginalis processes (Fig. 2a). MTZ is activated in the hydrogenosomes by the energy-production pathway through competition with HYD for the electrons of Fdx (Fig. 2b) (Lloyd and Kristensen, Reference Lloyd and Kristensen1985, Lloyd et al., Reference Lloyd, Yarlett and Yarlett1986). Both Fdx and nitroreductase (NTR) can reduce MTZ to its active nitro radical anion form (Yarlett et al., Reference Yarlett, Gorrell, Marczak and Muller1985; Yarlett et al., Reference Yarlett, Yarlett and Lloyd1986a, Reference Yarlett, Yarlett and Lloyd1986b; Pal et al., Reference Pal, Banerjee, Cui, Schwartz, Ghosh and Samuelson2009). In this active form, MTZ interferes with, and disrupts, DNA synthesis and repair, leading to cell death (Declerck and De Ranter, Reference Declerck and De Ranter1986).
TrxR, such as Fdx and NTR, can activate MTZ (Leitsch et al., Reference Leitsch, Kolarich, Binder, Stadlmann, Altmann and Duchene2009). This provides an alternative theory to the mechanism of action and target metabolic mechanisms that 5-nitroimidazoles affect. Upon activation, MTZ can form a ‘covalent adduct’ with TrxR and other enzymes and proteins involved in the antioxidant defense pathway. The inactivation of TrxR prevents Trx activation which in turn blocks TrxP from reducing H2O2, which is cytotoxic. Left unchecked, this will lead to death of the organism. Thus, antioxidant defenses (i.e. oxygen scavenging mechanisms) could be the main mechanism of action of MTZ instead of DNA disruption.
Aerobic vs anaerobic resistance to 5-nitroimidazoles in T. vaginalis
5-Nitroimidazole resistance in T. vaginalis can be either aerobic (in vivo clinical resistance) or anaerobic (laboratory-induced, in vitro resistance) (Kulda et al., Reference Kulda, Tachezy and Cerkasovova1993; Tachezy et al., Reference Tachezy, Kulda and Tomkova1993). Aerobic resistance is the most commonly observed form of resistance and may be a potential first step in the development of anaerobic resistance (Kulda, Reference Kulda1999). Proposed mechanisms for aerobic and anaerobic resistance to 5-nitroimidazoles in T. vaginalis include multiple enzymes and proteins involved in energy production as well as oxygen-scavenging mechanisms (Coombs, Reference Coombs1978; Land et al., Reference Land, Clemens and Johnson2001; Rasoloson et al., Reference Rasoloson, Tomkova, Cammack, Kulda and Tachezy2001; Leitsch et al., Reference Leitsch, Kolarich and Duchene2010; Paulish-Miller et al., Reference Paulish-Miller, Augostini, Schuyler, Smith, Mordechai, Adelson, Gygax, Secor and Hilbert2014).
Aerobic resistance
Aerobic resistance arises due to deficiencies associated with oxygen-scavenging mechanisms of the antioxidant-defense pathway (Lloyd and Pedersen, Reference Lloyd and Pedersen1985; Yarlett et al., Reference Yarlett, Yarlett and Lloyd1986a, Reference Yarlett, Yarlett and Lloyd1986b; Ellis et al., Reference Ellis, Yarlett, Cole, Humphreys and Lloyd1994). Decreased or absent FR1, TrxR and possibly NADH oxidase and SOD activity leads to increased intracellular oxygen levels (Fig. 3b) (Muller and Gorrell, Reference Muller and Gorrell1983; Rasoloson et al., Reference Rasoloson, Tomkova, Cammack, Kulda and Tachezy2001; Leitsch et al., Reference Leitsch, Kolarich and Duchene2010). Downregulated expression of FR1 in response to toxic TrxR-MTZ adducts limits the production of H2O2 and increases intracellular O2 leading to inactivation (oxidation) of MTZ (Leitsch et al., Reference Leitsch, Drinic, Kolarich and Duchene2012; Leitsch et al., Reference Leitsch, Janssen, Kolarich, Johnson and Duchene2014). Although increased O2 levels and MTZ inactivate cytosolic metabolic pathways, the hydrogenosomal metabolic pathways are still functional in aerobically resistant T. vaginalis strains. This is characterized by increased rates of glucose consumption and increased production of acetate and CO2 (Ellis et al., Reference Ellis, Cole and Lloyd1992).
Anaerobic resistance
Trichomonas vaginalis isolates that exhibit anaerobic resistance tend to have much higher in vitro MLC values compared to aerobically-resistant isolates. Anaerobic resistance has mainly been observed in vitro and induced under laboratory conditions rather than arising clinically. It is characterized by the disruption of enzymes that participate in the energy production pathway (Land et al., Reference Land, Clemens and Johnson2001; Land et al., Reference Land, Delgadillo and Johnson2002). Decreased levels of hydrogenosomal enzymes have been associated with smaller hydrogenosomes in laboratory-induced resistant strains compared to MTZ-sensitive strains (Wright et al., Reference Wright, Webb, O'Donoghue, Upcroft and Upcroft2010). However, loss of PFOR activity alone does not lead to resistance as Fdx functions in the malate-dependent pathway through NADH:FOR and MDH (Fig. 2b) (Rasoloson et al., Reference Rasoloson, Vanacova, Tomkova, Razga, Hrdy, Tachezy and Kulda2002). Thus, full resistance only occurs when both PFOR and NADH:FOR activities are lost. Another alternative energy pathway proposed in resistant T. vaginalis strains bypasses PFOR and the need to pass on electrons to Fdx. It involves the enzyme keto acid oxidoreductase (KOR), which, like PFOR, can reduce pyruvate and other metabolites to meet the energy demands of T. vaginalis (Brown et al., Reference Brown, Upcroft, Dodd, Chen and Upcroft1999).
Genetics of 5-nitroimidazole resistance
The role of genetic diversity and differential gene expression in 5-nitroimidazole resistance
Many techniques have been used to characterize and analyse the genotypic, phenotypic and genetic relatedness of T. vaginalis strains from different geographical regions. These techniques involve analysing random-amplified polymorphic DNA patterns, restriction fragment length polymorphisms, multi-locus sequence typing and internal transcribed spacer (ITS) region sequences (Vanacova et al., Reference Vanacova, Tachezy, Kulda and Flegr1997; Snipes et al., Reference Snipes, Gamard, Narcisi, Beard, Lehmann and Secor2000; Stiles et al., Reference Stiles, Shah, Xue, Meade, Lushbaugh, Cleary and Finley2000; Hampl et al., Reference Hampl, Vanacova, Kulda and Flegr2001; Abdel-Magied et al., Reference Abdel-Magied, El-Kholya, Abou El-Khair, Abdelmegeed, Hamoudaa, Mohamed and El-Tantawy2017). Genotypic and phenotypic analyses demonstrate the genetic relatedness of MTZ-resistant T. vaginalis strains and suggest T. vaginalis can be divided into two distinct populations (Cornelius et al., Reference Cornelius, Mena, Lushbaugh and Meade2010; Rabiee et al., Reference Rabiee, Bazmani, Matini and Fallah2012). Type 1 populations are the more ancestral lineage whereas type 2 populations appear to have diverged more recently (Conrad et al., Reference Conrad, Gorman, Schillinger, Fiori, Arroyo, Malla, Dubey, Gonzalez, Blank, Secor and Carlton2012), with type 2 having higher mean MLCs for MTZ (Bradic et al., Reference Bradic, Secor, Conrad and Carlton2012; Conrad et al., Reference Conrad, Gorman, Schillinger, Fiori, Arroyo, Malla, Dubey, Gonzalez, Blank, Secor and Carlton2012). Some genes involved in 5-nitroimidazole resistance possess mutations and are differentially expressed in resistant strains (Mead et al., Reference Mead, Fernadez, Romagnoli and Secor2006; Bradic et al., Reference Bradic, Warring, Tooley, Scheid, Secor, Land, Huang, Chen, Lee, Tang, Sullivan and Carlton2017).
Fdx gene mutations
Some MTZ-resistant T. vaginalis isolates have decreased fdx gene transcription (Quon et al., Reference Quon, d'Oliveira and Johnson1992). Two different point mutations have been identified at nucleotide positions −178 and −239 upstream of the transcription start site. The −239 mutation is more relevant and is characterized by an A→T transversion (adenine to thymidine). One study observed a 28-bp region surrounding the −239 mutation that binds a 23-kDa DNA-binding protein thought important for transcription of the fdx gene (Quon et al., Reference Quon, d'Oliveira and Johnson1992). MTZ-resistant isolates with this mutation have a lower binding affinity for DNA-binding protein that could lead to reduced transcription. This −239 A→T mutation has been observed more recently (Heidari et al., Reference Heidari, Bandehpour, Seyyed-Tabaei, Valadkhani, Haghighi, Abadi and Kazemi2013). An additional study analysed the amino-acid sequence of Fdx and determined regions coding for amino-acids 43–48 are more susceptible to mutation (Wiwanitkit, Reference Wiwanitkit2008).
NTR 4/6 gene mutation
NTR is one of the enzymes responsible for the reduction of 5-nitroimidazoles (Pal et al., Reference Pal, Banerjee, Cui, Schwartz, Ghosh and Samuelson2009). A recent study found single-nucleotide polymorphisms (SNPs) in two NTR genes (ntr4 and ntr6) (Paulish-Miller et al., Reference Paulish-Miller, Augostini, Schuyler, Smith, Mordechai, Adelson, Gygax, Secor and Hilbert2014). These SNPs were nonsense mutations (introduction of a stop codon) associated with MTZ resistance. Isolates with either mutation or both were more likely to belong to the type 2 population, which is associated with greater MTZ resistance (Paulish-Miller et al., Reference Paulish-Miller, Augostini, Schuyler, Smith, Mordechai, Adelson, Gygax, Secor and Hilbert2014).
ITS1 point mutation
The ITS regions that flank the 5.8S subunit of the ribosomal DNA gene of T. vaginalis are not as highly conserved as the ribosomal coding sequences they flank, leading to a greater frequency of genetic polymorphisms between organisms of the same species. A point mutation at the 66th nucleotide of the ITS region consisting of a cysteine being replaced with a thymidine (C66T) is associated with higher levels of MTZ resistance (Snipes et al., Reference Snipes, Gamard, Narcisi, Beard, Lehmann and Secor2000; Xiao et al., Reference Xiao, Xie, Fang, Gao, Zhu, Song, Zhong and Lun2006; Kazemi et al., Reference Kazemi, Hooshyar, Zareikar, Bandehpour, Arbabi, Talari, Alizadeh and Kazemi2010).
Iron metabolism affects gene expression in T. vaginalis
Iron plays an important role in the metabolic processes (as a cofactor in many enzymes) and transcriptional regulation of genes for key enzymes in T. vaginalis (Argaez-Correa et al., Reference Argaez-Correa, Alvarez-Sanchez, Arana-Argaez, Ramirez-Camacho, Novelo-Castilla, Coral-Martinez and Torres-Romero2019). The restriction of iron in T. vaginalis leads to the down-regulation of genes important for energy metabolism and drug activation like PFOR and Fdx (Horvathova et al., Reference Horvathova, Safarikova, Basler, Hrdy, Campo, Shin, Huang, Huang, Lin, Tang and Tachezy2012). Decreased expression leads to development of 5-nitroimidazole resistance. Iron is a key structural and metabolic component of PFOR and Fdx; decreasing iron stores available to T. vaginalis isolates decrease their ability to synthesize these critically important enzymes. Supplemental addition of iron with MTZ treatment decreases MLCs under both aerobic and anaerobic conditions in MTZ-sensitive strains (Elwakil et al., Reference Elwakil, Tawfik, Alam-Eldin and Nassar2017).
Expression of surface carbohydrate genes
Trichomonas vaginalis membranes contain glycoproteins and glycolipids that act as cell receptors and are involved in host–parasite interactions. The plasma membranes of MTZ-resistant and MTZ-sensitive T. vaginalis strains differ in their surface carbohydrate structures (Dias Filho et al., Reference Dias Filho, Andrade, de Souza, Esteves and Angluster1992). Trichomonas vaginalis also has a P-glycoprotein gene (Tvpgp1) that codes for an ATP binding cassette (ABC) transmembrane protein (Johnson et al., Reference Johnson, Schuck and Delgadillo1994). ABC proteins are involved in the transport of drugs out of cells. Tvpgp1 is expressed 2–20-fold higher in MTZ-resistant T. vaginalis strains compared to sensitive strains (Johnson et al., Reference Johnson, Schuck and Delgadillo1994).
Extracellular ATP and ADP level modulation by T. vaginalis
Extracellular ATP is involved in host–parasite interactions such as signal transduction, cytoadherence, immune response and inflammation. The enzymes that T. vaginalis employs for the hydrolysis of extracellular ATP, adenosine diphosphate (ADP) and adenosine monophosphate, are NTPDase1 and ecto-5′-nucleotidase; these enzymes can be incorporated into the plasma membrane of T. vaginalis and are involved in signal transduction and cytoadhesion. Prolonged exposure/treatment with MTZ or TDZ results in the inhibition of these enzymes in MTZ-sensitive strains (Tasca et al., Reference Tasca, Borges, Bonan, De Carli, Battastini and Sarkis2003). Thus, modulation of extracellular ATP and ADP levels may be a defense strategy of T. vaginalis in response to unfavourable environmental conditions (i.e. drug pressure) (Tasca et al., Reference Tasca, Borges, Bonan, De Carli, Battastini and Sarkis2003).
Relative vs absolute resistance in T. vaginalis
Compared to resistance mechanisms in many bacteria, resistance to 5-nitroimidazoles in Trichomonas appears to be relative rather than absolute. Based upon how quickly resistance to MTZ appeared after its introduction (Robinson, Reference Robinson1962), the mechanism(s) for drug resistance may have already existed and clinical treatment failure may be more of a function of drug tolerance rather than developed drug resistance. For example, T. vaginalis infections unresponsive to currently recommended doses of MTZ (i.e. 2 g single oral dose or 500 mg orally twice daily for 7 days) can often be treated by increasing dosages (i.e. 2–4 g of MTZ orally daily for 3–14 days) (Lossick et al., Reference Lossick, Muller and Gorrell1986). This may be because the enzymes that activate MTZ are important in other critical cellular functions and complete loss of these enzymes would result in parasite death. In addition, some T. vaginalis isolates have been found to be clinically more resistant than others with similar MLC values to MTZ (Lossick et al., Reference Lossick, Muller and Gorrell1986). This suggests that complex interactions between drug levels in the vaginal mucosa, the intra-vaginal redox potential (which may regulate the amount of drug taken up by the parasites), and the composition of the vaginal microbiota (which may modify the amount of available drug) may contribute to the level of drug resistance (Lossick et al., Reference Lossick, Muller and Gorrell1986). The major limiting factor of high dose treatment in such cases is the amount of drug that the patient can safely tolerate. Significant nausea, metallic taste, sensorium changes and peripheral neuropathy have occurred in patients receiving 4 g of MTZ for 14 days (Lossick et al., Reference Lossick, Muller and Gorrell1986). Thus, the risks and benefits of treating patients with MTZ-resistant trichomoniasis with daily doses of MTZ exceeding 3 g should be carefully considered; alternative treatments outside of 5-nitroimidazoles may need to be considered in these cases (Workowski et al., Reference Workowski, Bolan and Centers for Disease and Prevention2015).
Other factors supporting the assertion that Trichomonas resistance is relative rather than absolute are the similar prevalence of the two genotypes (type 1 and type 2) in different regions of the world (Conrad et al., Reference Conrad, Gorman, Schillinger, Fiori, Arroyo, Malla, Dubey, Gonzalez, Blank, Secor and Carlton2012) and the apparent lack of ‘outbreaks’ of drug resistance that might be expected if resistance developed in response to drug pressure.
The role of T. vaginalis co-infections and 5-nitroimidazole resistance
Early treatment failures to MTZ were not readily attributed to resistance but instead to the presence of a diverse population of micro-organisms that may metabolize MTZ before it can reach T. vaginalis for uptake in sufficient concentrations to kill the parasite (Edwards et al., Reference Edwards, Thompson, Tomusange and Shanson1979; Ingham et al., Reference Ingham, Sisson and Selkon1982). Some data suggest that 5-nitroimidazole resistance may also be associated with co-infection of T. vaginalis by Mycoplasma hominis or T. vaginalis virus (TVV) (Wang, Reference Wang1984; Fichorova et al., Reference Fichorova, Fraga, Rappelli and Fiori2017).
Trichomonas vaginalis virus (TVV)
TVV is a 4.5 kbp dsRNA virus that infects some T. vaginalis isolates (Wang and Wang, Reference Wang and Wang1985). Four strains of TVV have been described since its discovery in the mid-1980s with TVV1 being the most common strain, followed by TVV2 and TVV3, with TVV4 being least common (Graves et al., Reference Graves, Ghosh, Kissinger and Muzny2019). TVV2 and TVV3 are associated with expression of the surface immunogen P270. Some studies found no clear associations of TVV presence on MTZ resistance (Wang and Wang, Reference Wang and Wang1985; Flegr et al., Reference Flegr, Cerkasov, Kulda, Tachezy and Stokrov1987), whereas another study found increased MTZ susceptibility in TVV-infected T. vaginalis (Malla et al., Reference Malla, Kaul, Sehgal and Gupta2011); additional research is needed to clarify the effect of TVV infection on 5-nitroimidazole resistance in T. vaginalis.
Mycoplasma hominis
Mycoplasma hominis is a bacterial pathogen of the lower genital tract that naturally infects T. vaginalis (van Belkum et al., Reference van Belkum, van der Schee, van der Meijden, Verbrugh and Sluiters2001). Some studies have found that M. hominis infection of T. vaginalis was associated with increased MTZ resistance (Xiao et al., Reference Xiao, Xie, Fang, Gao, Zhu, Song, Zhong and Lun2006; Furnkranz et al., Reference Furnkranz, Henrich and Walochnik2018). However, other studies indicate M. hominis infection has no clinical significance (Butler et al., Reference Butler, Augostini and Secor2010; Fraga et al., Reference Fraga, Rodriguez, Fernandez, Mondeja, Sariego, Fernandez-Calienes and Rojas2012; da Luz Becker et al., Reference da Luz Becker, dos Santos, Frasson, de Vargas Rigo, Macedo and Tasca2015). Mycoplasma hominis can downregulate expression of Fdx, FR1 and PFOR genes involved in MTZ resistance mechanisms (Furnkranz et al., Reference Furnkranz, Henrich and Walochnik2018); however, similar to TVV, how M. hominis affects T. vaginalis susceptibility to MTZ needs further investigation.
Discussion
We found that resistance is facilitated by altered expression and activation of enzymes and proteins involved in T. vaginalis energy production and oxygen scavenging. Increased intracellular oxygen concentrations, due to deficient oxygen scavenging, enables development of aerobic resistance, the major form of resistance encountered in clinical isolates. Anaerobic resistance is rarely observed clinically but has been induced under laboratory conditions and is characterized by altered energy metabolism due to reduced expression of enzymes and proteins involved in the main and alternative energy-production pathways. There is no history of ‘outbreaks’ of drug-resistant trichomoniasis and the relative frequency of the two genotypes of T. vaginalis is consistent throughout the world, suggesting that insensitivity to 5-nitroimidazoles may naturally occur rather than being treatment-induced.
Currently recommended treatments in the setting of 5-nitroimidazole resistance are 2 g of oral MTZ or TDZ daily for 7 days (Workowski et al., Reference Workowski, Bolan and Centers for Disease and Prevention2015). Even higher doses of these medications for longer periods of time have been previously used to overcome drug resistance (Lossick et al., Reference Lossick, Muller and Gorrell1986). However, patients may be at increased risk of adverse drug effects, as previously described (Lossick et al., Reference Lossick, Muller and Gorrell1986). For patients failing to respond to higher doses or longer courses of oral MTZ or TDZ, two additional treatments are available, although not rigorously studied. The first is high dose oral TDZ 2 g daily plus intra-vaginal TDZ 500 mg twice daily for 14 days (Sobel et al., Reference Sobel, Nyirjesy and Brown2001). If this regimen fails, a second option is high dose oral TNZ 1 g three times daily plus intra-vaginal paromomycin (4 g of 6.25% intra-vaginal paromomycin cream nightly) for 14 days (Nyirjesy et al., Reference Nyirjesy, Gilbert and Mulcahy2011). Paromomycin is an aminoglycoside with a different mechanism of action (destruction of ribosomal RNA) than 5-nitroimidazoles (inhibition of nucleic acid synthesis by DNA disruption and/or inhibition of enzymes involved in antioxidant defense) (Leitsch et al., Reference Leitsch, Kolarich, Binder, Stadlmann, Altmann and Duchene2009; Van Gerwen and Muzny, Reference Van Gerwen and Muzny2019); combination use of these medications may provide a synergistic effect. It is important to note that all intra-vaginal formulations need to be made at a compounding pharmacy and may be costly (Muzny et al., Reference Muzny, Van Gerwen and Kissinger2020). In addition, intra-vaginal paromomycin can cause vaginal ulceration and should be discontinued if this occurs, at which time ulcerations may spontaneously regress (Van Gerwen and Muzny, Reference Van Gerwen and Muzny2019).
The primary limitation of this study is that non-English language articles were excluded. Because of this we may have missed some pertinent information that should have been included. Future reviews on this topic should include addition of non-English language articles. Despite this limitation, we were able to find a moderately sized body of English-language literature to include in this review. In summary, the data reviewed suggest that alternative methods of treatment using medications outside of the 5-nitroimidazole class are urgently needed as well as a more complete understanding of the mechanisms of 5-nitroimidazole resistance in T. vaginalis.
Acknowledgements
This paper was presented as a poster presentation at the 2019 Infectious Diseases Society of Obstetrics and Gynecology (IDSOG) Annual Meeting in Big Sky, Montana on 8 August 2019 (#12).
Financial support
This work was supported by a Developmental Award to Keonte Graves, MS from the University of Alabama at Birmingham Sexually Transmitted Infections Cooperative Research Center (U19AI113212).
Conflict of interest
Christina A. Muzny, MD, MSPH is a consultant for Lupin Pharmaceuticals, BioFire Diagnostics and Cepheid. She has also received research funding support from Lupin as well as speaker honoraria from Abbott Molecular, Cepheid, Roche Diagnostics and Becton Dickinson. All other authors declare no conflicts of interest.
Disclaimer
The findings and conclusions in this report are those of the authors and do not necessarily represent the views of the Centers for Disease Control and Prevention.
Ethical standards
Not applicable.