Introduction
Helminths are invertebrates morphologically characterized by elongated, flat or round bodies, and include trematodes, cestodes and nematodes (Kaliappan et al., Reference Kaliappan, George, Francis, Kattula, Sarkar, Minz, Mohan, George, Roy and Ajjampur2013). Among them, soil-transmitted helminths (STHs) and schistosomes are responsible for the most prevalent parasitic infection of humans living in resource-limited areas of developing countries. Studies indicate that STHs, such as Ascaris lumbricoides and Trichuris trichiura, as well as hookworms, affect over 1.5 billion people worldwide (WHO, 2019a). Schistosomiasis caused by the genus Schistosoma affects at least 220.8 million people worldwide (WHO, 2019b). Currently, it is still prevalent in 78 countries where 700 million people are at the risk of contracting schistosomiasis. The World Health Organization has already developed a roadmap to control and eliminate the related neglected tropical diseases caused by these helminths. The major control strategy against these helminth infections is mass drug administration (Warren et al., Reference Warren, Bundy, Anderson, Jamison, M., Measham and Bobadilla2001; Minetti et al., Reference Minetti, Lacourse, Reimer and Stothard2016). Consequently, it is critical to timely and accurately identify helminth infections to determine whether drug treatment is working efficiently and then deploy appropriate strategies for disease intervention. Currently, several ‘gold standard’ techniques are used for diagnosing human infection including the identification of parasites in blood (lymphatic filariasis (LF)) or skin snip biopsy (onchocerciasis), eggs or larvae in stools or urine (STHs and schistosomiasis) by microscopy and the detection of antibodies or pathogen antigens circulating in blood (onchocerciasis, LF and schistosomiasis) or urine (schistosomiasis) by immunological methods. However, these approaches usually have some limitations such as relatively low sensitivity (particularly for low and mid intensity infections), relatively low-throughput, increased time-consumption, skilled personnel being required for operation and lack of onsite applicability. In addition, serological methods for helminth diagnosis have limited ability to distinguish between ongoing and previous infections (Hinz et al., Reference Hinz, Schwarz, Hahn and Frickmann2017).
It is essential to develop rapid and sensitive techniques with high-throughput for diagnosing helminth infections. Immunodiagnostic techniques such as the detection of antibodies and antigens, and molecular diagnoses such as polymerase chain reaction (PCR), quantitative qPCR (qPCR), loop-mediated isothermal amplification (LAMP) and recombinase polymerase amplification (RPA) can fulfil these requirements. In addition, nucleic acid-based methods also offer the most rapid and sensitive detection with the potential to distinguish different species and to monitor potential genetic variation in pathogen populations owing to selection pressure caused by drug usage. Although the broad application of current nucleic acid-based detection methods for surveying, monitoring and evaluating helminth control programs have so far been hindered by some obvious flaws such as lack of standards of sample preparation and nucleic acid isolation, lack of suitable internal control for target deoxyribonucleic acid (DNA)/(ribonucleic acid) RNA amplification, potentially high background noise, potential amplification inhibition and lack of accurate data interpretation for intensity of infection, these techniques may best guide decision making for controlling helminth diseases because of their higher sensitivity, rapid and high-throughput (Corrado et al., Reference Corrado, James Lacourse, Lisa and Stothard2016). Hence, we mainly focused on nucleic acid-based diagnostic methods including PCR, qPCR, LAMP and RPA, reviewed their recent advances and highlighted the potential usage of biosensors, a modern technique, for the diagnosis of helminth infection.
Nucleic acid-based methods for detection of helminth infections
To date, several different nucleic acid-based methods have been available for the detection of helminth infections such as PCR, multiplex PCR, qPCR, LAMP and RPA. These methods offer considerable advantages such as precision, timeliness and sensitivity of parasite detection. We have briefly reviewed recent advances in each method and then discussed their utility in the diagnosis of helminth infections in the following sections.
PCR
The PCR is based on using the ability of DNA polymerase to synthesize a new strand of DNA complementary to the template strand. The technique has been successfully used for detecting schistosome DNA from host serum, urine and faeces as well as water containing cercaria and infected snails (Enk et al., Reference Enk, e Silva and Rodrigues2012; Sarhan et al., Reference Sarhan, Kamel, Saad and Ahmed2015; Sengupta et al., Reference Sengupta, Hellstrom, Kariuki, Olsen, Thomsen, Mejer, Willerslev, Mwanje, Madsen, Kristensen, Stensgaard and Vennervald2019). A new PCR-based approach has been shown to identify different Schistosoma species (S. mansoni, S. japonicum, S. haematobium, S. intercalatum and S. bovis) and is applicable to human urine samples with high specificity and sensitivity (Sandoval et al., Reference Sandoval, Siles-Lucas, Perez-Arellano, Carranza, Puente, Lopez-Aban and Muro2006). Recently, a study documented that PCR assay targeting the 121-bp tandem repeat sequence sensitive for S. mansoni and the Dra1 tandem repeat sequence specific for S. haematobium can distinguish Schistosoma species for diagnosis of early schistosomiasis (Bonnefond et al., Reference Bonnefond, Cnops, Duvignaud, Bottieau, Pistone, Clerinx and Malvy2019). For filarial pathogens, the amplification of genomic DNA repeat sequences (188 bp DNA repeat sequence) SspI from Wuchereria bancrofti (Zhong et al., Reference Zhong, McCarthy, Bierwert, Lizotte-Waniewski, Chanteau, Nutman, Ottesen and Williams1996), Hha I repeat region from Brugia malayi (Fischer et al., Reference Fischer, Supali, Wibowo, Bonow and Williams2000), LLMF72 or a repeat region (15r3) from Loa loa (Fink et al., Reference Fink, Kamgno and Nutman2011) and the O-150 repeat sequence from Onchocerca volvulus (Toé et al., Reference Toé, Boatin, Adjami, Back, Merriweather and Unnasch1998) by the PCR has been used to detect pathogen infection. In addition, the PCR can also amplify W. bancrofti DNA from host sputum, serum and urine with high diagnostic accuracy (Kagai et al., Reference Kagai, Mpoke, Muli, Hamburger and Kenya2008; Ximenes et al., Reference Ximenes, Brandao, Oliveira, Rocha, Rego, Medeiros, Aguiar-Santos, Ferraz, Reis, Araujo, Carvalho and Melo2014). Moreover, the detection of Taenia solium DNA from cerebrospinal fluid (CSF) samples by the PCR has diagnostic potential for neurocysticercosis, which cannot be detected using radiology or serology (Michelet et al., Reference Michelet, Fleury, Sciutto, Kendjo, Fragoso, Paris and Bouteille2011; Chaya and Parija, Reference Chaya and Parija2014). Furthermore, the PCR was used to amplify pathogen-specific DNA in host saliva and urine, which may become a new type of non-invasive biomarker for the diagnosis of schistosomiasis japonica (Weerakoon and McManus, Reference Weerakoon and McManus2016). In addition, this has been shown to have good potential for detecting early stage of schistosome infection by amplifying schistosome specific DNA from host sera using the PCR (Xia et al., Reference Xia, Rong, Lu, Shi, Xu, Zhang, Gong and Luo2009; Kato-Hayashi et al., Reference Kato-Hayashi, Kirinoki, Iwamura, Kanazawa, Kitikoon, Matsuda and Chigusa2010).
The traditional PCR can be modified by incorporating additional primers and amplification to increase the sensitivity for detecting helminth infection. For example, a two-step semi-nested PCR for amplifying Oesophagostomum bifurcum egg DNA in human faecal samples was shown to achieve a specificity of 100% (Verweij et al., Reference Verweij, Polderman, Wimmenhove and Gasser2000). In addition, the semi-nested PCR technique showed a 1 000 000-fold increase to the detection limit for W. bancrofti, compared with that of the conventional PCR (Ximenes et al., Reference Ximenes, Brandao, Oliveira, Rocha, Rego, Medeiros, Aguiar-Santos, Ferraz, Reis, Araujo, Carvalho and Melo2014).
By incorporating fluorescent dyes or fluorescently labelled oligonucleotide probes, the amplified DNA can be quantified. This is called a real-time qPCR, which is now one of the most widely used tools in research and clinical diagnosis. The qPCR has been used to detect schistosomes, STHs (e.g. S. stercoralis, T. trichiura, A. duodenale, N. americanus and A. lumbricoides) and O. volvulus infection (Morales-Hojas et al., Reference Morales-Hojas, Post, Shelley, Maia-Herzog, Coscarón and Cheke2001; Lier et al., Reference Lier, Simonsen, Wang, Lu, Haukland, Vennervald, Hegstad and Johansen2009; O'Connell and Nutman, Reference O'Connell and Nutman2016a; Prince-Guerra et al., Reference Prince-Guerra, Cama, Wilson, Thiele, Likwela, Ndakala, wa Muzinga, Ayebazibwe, Ndjakani and Pitchouna2018). In schistosomes, the qPCR was shown to identify considerably more positive cases than the Kato–Katz method and could be adopted as a suitable strategy for schistosomiasis elimination (He et al., Reference He, Gordon, Williams, Li, Wang, Hu, Gray, Ross, Harn and McManus2018).
Recently, microRNAs (miRNAs) have been considered as a new class of biomarkers for diagnosing many diseases, particularly for circulating miRNAs (Cheng, Reference Cheng2015). For example, Cheng and coworkers (Reference Cheng, Luo, Hu, Cao and Jin2013) identified five schistosome-specific miRNAs (Bantam, miR-3479, sja-miR-8185, miR-10 and miR-3096) in the plasma of rabbits infected with S. japonicum. Four out of these five miRNAs were shown to be significantly abundant in plasma of S. japonicum-infected mice, suggesting these miRNAs can serve as a potential biomarker for schistosomiasis diagnosis. More interestingly, several further studies demonstrated that parasite-derived miRNAs (miR-277, miR-3479-3p and bantam) could detect individuals infected with S. mansoni or S. haematobium from low and high-infection intensity sites by the real time qPCR (Hoy et al., Reference Hoy, Lundie, Ivens, Quintana, Nausch, Forster, Jones, Kabatereine, Dunne and Mutapi2014; Meningher et al., Reference Meningher, Lerman, Regev-Rudzki, Gold, Ben-Dov, Sidi, Avni and Schwartz2017).
Apart from diagnosis, the PCR and qPCR can also be used to distinguish between species of parasites. For example, a copro-PCR method targeting a segment of the internal transcribed spacer (ITS) region and COX1 gene was shown to distinguish between O. viverrini and C. sinensis; results suggested that C. sinensis was present in an area in central Thailand where it had not been previously detected (Traub et al., Reference Traub, Macaranas, Mungthin, Leelayoova, Cribb, Murrell and Thompson2009; Cai et al., Reference Cai, Yu, Li, Yue, Liu, Bai, Deng, Qiu and Zhu2014). In addition, the PCR and qPCR for diagnosis of STH infections and for monitoring genetic variation of helminths under mass drug administration have been elegantly reviewed (Corrado et al., Reference Corrado, James Lacourse, Lisa and Stothard2016; O'Connell and Nutman, Reference O'Connell and Nutman2016b; Papaiakovou et al., Reference Papaiakovou, Gasser and Littlewood2019). The qPCR was able to detect and distinguish filarial infection in domestic cats among B. malayi, D. immitis and D. repens as well as A. (Dipetalonema) reconditum, showing more sensitivity than microfilariae detection (Wongkamchai et al., Reference Wongkamchai, Nochote, Foongladda, Dekumyoy, Thammapalo, Boitano and Choochote2014).
Unlike the conventional qPCR, the Droplet Digital™ PCR (ddPCR) can perform absolute quantification of PCR products without the need for generating standard curves (Elmahalawy et al., Reference Elmahalawy, Halvarsson, Skarin and Hoglund2018). In principle, the ddPCR can fractionate a loading sample into thousands to millions of droplets, and PCR amplification of the template molecules occurs in each individual droplet, followed by a real time or endpoint detection of the amplification. The distribution of target sequences into droplets is described by the Poisson distribution, thus allowing accurate and absolute quantification of the target without using reference materials with known target concentrations (Gutierrez-Aguirre et al., Reference Gutierrez-Aguirre, Racki, Dreo and Ravnikar2015). The ddPCR has been successfully used to detect W. bancrofti DNA (targeting the long DNA repeat (LDR) element) and B. malayi DNA (targeting the Hha I element) in host blood samples and mosquito vectors with high sensitivity and specificity (Jongthawin et al., Reference Jongthawin, Intapan, Lulitanond, Sanpool, Thanchomnang, Sadaow and Maleewong2016). In gastrointestinal (GI) nematode parasitic infection, the ddPCR can detect and distinguish major GI nematodes by targeting the internal transcribed spacer region 2 (ITS2) of the ribosomal RNA gene in ovine parasites such as Haemonchus contortus, Teladorsagia circumcincta and Trichostrongylus species (Elmahalawy et al., Reference Elmahalawy, Halvarsson, Skarin and Hoglund2018). In addition, the ddPCR assay exhibited a high level of diagnostic performance compared with Kato–Katz and antibody-based ELISA for diagnosis of Schistosoma infection although it is expensive and requires specialized equipment (Weerakoon et al., Reference Weerakoon, Gordon, Cai, Gobert, Duke, Williams and McManus2017a,Reference Weerakoon, Gordon, Williams, Cai, Gobert, Olveda, Ross, Olveda and McManusb; Cai et al., Reference Cai, Weerakoon, Mu, Olveda, Ross, Olveda and McManus2019).
Multiplex PCR
The multiplex PCR can amplify several different DNA sequences simultaneously using multiple primers (Touron et al., Reference Touron, Berthe, Pawlak and Petit2005; Hawkins and Guest, Reference Hawkins and Guest2017). Using selected target sequences for amplification, this PCR approach can not only detect the pathogen's genotype, but also distinguish among species. For example, S. mansoni and S. haematobium from a set of duplicate stool samples can be distinguished using primers for species-specific amplification of cytochrome C oxidase genes by the multiplex PCR, and the results showed a significant correlation with microscopic egg counts for S. mansoni in stool and S. haematobium in urine (ten Hove et al., Reference ten Hove, Verweij, Vereecken, Polman, Dieye and van Lieshout2008). In addition, the multiplex PCR was also shown to distinguish between differential infections by T. solium and T. asiatica (Wicht et al., Reference Wicht, Yanagida, Scholz, Ito, Jiménez and Brabec2010; Jeon and Eom, Reference Jeon and Eom2013). Moreover, the multiplex PCR has been shown to discriminate N. americanus, A lumbricoides and T. trichiura infections from faecal samples (Phuphisut et al., Reference Phuphisut, Yoonuan, Sanguankiat, Chaisiri, Maipanich, Pubampen, Komalamisra and Adisakwattana2014). In the Philippines, a study indicated that the multiplex PCR could simultaneously detect two cestode parasites T. saginata and T. solium alongside A. lumbricoides and hookworms in stool samples (Gordon et al., Reference Gordon, McManus, Acosta, Olveda, Williams, Ross, Gray and Gobert2015). Moreover, a recent study indicated that a multiplex single-tube PCR assay can specifically discriminate trematode, cestode and nematode species by targeting the 28S rRNA gene (trematode), COX1 gene (cestode) and COX1 gene (nematode) (Wong et al., Reference Wong, Poon, To, Chan, Lu, Xing, Cheng and Yuen2019).
By incorporating Luminex beads or fluorescent dye, the multiplex PCR can be modified as qPCR to improve its sensitivity and specificity. For example, several helminths including A. lumbricoides, A. duodenale, N. americanus and S. stercoralis can be quantitatively co-detected using a Luminex bead-based multiplex qPCR, showing high sensitivity (90–100%) and specificity (95–100%) from 319 stool samples (Taniuchi et al., Reference Taniuchi, Verweij, Noor, Sobuz, Lieshout, Petri, Haque and Houpt2011). In addition, a multiplex qPCR for detecting N. americanus and A. duodenale also showed 100% specificity and 98.5 to 100% sensitivity (Verweij et al., Reference Verweij, Brienen, Ziem, Yelifari, Polderman and Van Lieshout2007). Moreover, a pentaplex qPCR was not only able to simultaneously detect A. duodenale, N. americanus, A. lumbricoides and S. stercoralis but also to show significantly increased sensitivity of detection (up to 62.3% compared with the detection of microscopic examination of 7.8%) for one or more helminth species (Basuni et al., Reference Basuni, Muhi, Othman, Verweij, Ahmad, Miswan, Rahumatullah, Aziz, Zainudin and Noordin2011). Recent studies also indicated that a multiplex TaqMan qPCR assay can specifically identify encapsulated Trichinella species in North America (Almeida et al., Reference Almeida, Bishop, Nascimento, Mathison, Bradbury and Silva2018), and a multiplex qPCR can detect and quantify DNA from F. hepatica and the intermediate snail host, A. tomentosa, in water samples (Rathinasamy et al., Reference Rathinasamy, Hosking, Tran, Kelley, Williamson, Swan, Elliott, Rawlin, Beddoe and Spithill2018).
Loop-mediated isothermal amplification (LAMP)
Although the PCR and multiplex PCR offer significant advantages for detecting helminth infections such as more sensitivity and specificity, high-throughput and cost-effectiveness, both methods are heavily dependent on a particular instrument or elaborate detection methods. Alternatively, LAMP can amplify target DNA by DNA polymerase based on a set of specific primers under a constant temperature of 60–65°C without a thermocycler and accumulated 109 copies of target DNA in less than an hour, with a detection limit of a few copies. More importantly, the amplification endpoint could be observed using agarose gel electrophoresis for visual observation of DNA under UV light and using turbidity determination that is more suitable for field research. As a pyrophosphate ion is released once a nucleotide is added to the DNA strands, a visible white precipitate of magnesium pyrophosphate is formed and used to determine whether the target nucleic acid was amplified or not.
The use of LAMP for helminth detection has been recently summarized and reviewed (Amoah et al., Reference Amoah, Singh, Stenstrom and Reddy2017; Deng et al., Reference Deng, Zhong, Kamolnetr, Limpanont and Lv2019). Here, we highlight only the studies related to schistosomes. LAMP has been successfully used to amplify the SjR2 gene of S. japonicum from the blood samples of infected rabbits at 3rd day post-infection, indicating the potential of early detection of S. japonicum infection (Xu et al., Reference Xu, Guan, Zhao, Wang, Cao, Zhang, Zhu, He and Xia2015). In addition, by targeting S. haematobium ribosomal intergenic spacer DNA, LAMP was shown to detect S. haematobium DNA in heated pellets from patients’ urine samples without requiring a complicated procedure for DNA extraction with 100% sensitivity and 86.67% specificity (Gandasegui et al., Reference Gandasegui, Fernández-Soto, Carranza-Rodríguez, Pérez-Arellano, Vicente, López-Abán and Muro2015). Moreover, LAMP can detect miracidium DNA at the first day of snail exposure (intermediate hosts) to Schistosoma (Abbasi et al., Reference Abbasi, King, Muchiri and Hamburger2010) and also detect infection in pooled samples of field-collected snails (Tong et al., Reference Tong, Chen, Zhang, Yang, Kumagai, Furushima-Shimogawara, Lou, Yang, Wen, Lu, Ohta and Zhou2015). Furthermore, LAMP was shown to be an effective means to detect low-intensity infection of schistosomiasis (Lodh et al., Reference Lodh, Mikita, Bosompem, Anyan, Quartey, Otchere and Shiff2017). Overall, these studies suggested that LAMP might become a new surveillance and response tool for schistosomiasis control.
Compared to the PCR, LAMP has better sensitivity that could enhance the effectiveness of surveillance and mass drug administration of control programs for helminth diseases. However, primer design is a critical part of LAMP assay, because the use of multiple primers for LAMP assay may increase the risk of primer–primer hybridization, resulting in template-free amplification that needs to further evaluate false-positive outcomes (Hsieh et al., Reference Hsieh, Mage, Csordas, Eisenstein and Soh2014). Similar to the PCR, LAMP cannot be used for detecting unknown or unsequenced targets of pathogens since specific DNA sequences are required for primer design (Mori and Notomi, Reference Mori and Notomi2020).
Recombinase polymerase amplification (RPA)
RPA is another fast and sensitive isothermal amplification-based method for amplifying target DNA or RNA using a bacteriophage derived recombinase in combination with a polymerase (Piepenburg et al., Reference Piepenburg, Williams, Stemple and Armes2006; Euler et al., Reference Euler, Wang, Nentwich, Piepenburg, Hufert and Weidmann2012). RPA comprises three core enzymes including a recombinase, a single-stranded DNA-binding protein (SSB) and a strand-displacing polymerase. Recombinases pair oligonucleotide primers with a homologous sequence, and the SSB binds to displaced strands to prevent primer displacement (Piepenburg et al., Reference Piepenburg, Williams, Stemple and Armes2006). Finally, the strand displacing polymerase begins DNA synthesis where the primer has bound to the target DNA (Euler et al., Reference Euler, Wang, Nentwich, Piepenburg, Hufert and Weidmann2012).
Studies have demonstrated that RPA can detect small amounts of viral, bacterial and parasitic DNA with high sensitivity and specificity (Shahin et al., Reference Shahin, Gustavo Ramirez-Paredes, Harold, Lopez-Jimena, Adams and Weidmann2018). In helminths, most of these studies focused on schistosomes. For example, RPA was used to amplify the SjR2 gene of S. japonicum, and the result indicated that this assay could detect 0.9 fg S. japonicum DNA within 15 min and distinguish S. japonicum from other worm species (Xing et al., Reference Xing, Yu, Feng, Sun, Fu, Wang, Zou, Xia, Luo, He, Li and Xu2017). In addition, RPA combined with oligochromatographic lateral flow strips was shown to detect low levels of S. haematobium DNA (Dra1) from crude urine (Rosser et al., Reference Rosser, Rollinson, Forrest and Webster2015). Similarly, an RPA-based lateral flow was also developed in S. mansoni by amplifying the 28S rDNA region and the result indicated that the lower limit of detection for this assay was 10 pg DNA with the lower test parameters permitting sufficient amplification being 6 min and 25°C (Poulton and Webster, Reference Poulton and Webster2018).
RPA can amplify as low as 1–10 DNA/RNA target copies with a rapid turnaround time (less than 20 min) and simple set-up at a single low temperature (37–42°C). Consequently, it provides significant advantages compared with other isothermal methods such as LAMP. In addition, RPA has been shown to amplify diverse targets, including RNA, miRNA, ssDNA and dsDNA from a wide variety of organisms and samples (Euler et al., Reference Euler, Wang, Nentwich, Piepenburg, Hufert and Weidmann2012). It may be necessary to develop an RPA assay to detect pathogen-specific small RNAs such as miRNAs in future for diagnosing helminth infections. Moreover, RPA has good potential for field application and the use of this method can reduce diagnostic expenses in resource-limited countries (Ajibola et al., Reference Ajibola, Gulumbe, Eze and Obishakin2018). Although PCR, LAMP and RPA methods can also be ‘sensed’ by incorporating a fluorescent dye or probe and the amplified signal can be detected and presented by an electrical device, these methods usually carry disadvantages such as requiring a multi-step set up, being time-consuming, requiring a trained operator for use and being difficult to apply in the field.
Biosensors for nucleic acid biomarker detection
With the development of modern electrical and chemical techniques, biosensors, a powerful and innovative analytical device shows excellent characterizations, being highly sensitive, rapid, simple, low-cost and field-applicable that can be widely used for drug discovery, diagnosis, biomedicine, food safety, processing and environmental monitoring.
The first biosensor invented by Clark and Lyons (Reference Clark and Lyons1962); Cheng and coworkers (Reference Cheng, Luo, Hu, Cao and Jin2013) for measuring glucose levels in biological samples was based on electrochemical detection of oxygen or hydrogen peroxide (Fracchiolla et al., Reference Fracchiolla, Artuso and Cortelezzi2013). Since then, incredible progress has been made in terms of both the technology and applications of biosensors. Generally, biosensors consist of at least three elements, namely, a biological capture molecule (bioreceptor), a method of converting capture molecule-target interactions into a signal (transducer) and a data output system. A bioreceptor is an immobilized sensitive biological element (antibody, DNA probe or enzyme) recognizing the analytic (antigen, complementary DNA or enzyme substrate). A transducer is used to convert the (bio) chemical signal resulting from the interaction of the analytic with the bioreceptor into an electronic signal. The intensity of signals generated corresponds to the analyte concentration.
Currently, most reported biosensors have been designed to detect nucleic acid biomarkers and protein biomarkers related to pathogen infection. Nucleic acid biomarker-based biosensors show good potential for the detection and diagnosis of various diseases such as cancer, diabetes, cardiovascular diseases, tuberculosis, hepatitis, dengue and food-borne diseases such as diarrhea, cholera and salmonellosis (Bora et al., Reference Bora, Sett and Singh2013). Based on transducer methods, these biosensors can be divided into electrochemical biosensors and optical biosensors.
Electrochemical detection techniques
Various electrochemical biosensors have been reported to detect nucleic acid biomarkers during parasite infection, particularly protozoan infections. For example, Mohan and coworkers used a kind of sol–gel synthesized nickel oxide (NiO) film deposited onto an indium tin oxide (ITO) coated glass plate to immobilize a 23-mer DNA sequence (oligonucleotide) from 18S rRNA gene sequences from L. donovani for the development of a DNA biosensor. Response studies of the ss-DNA/NiO/ITO bioelectrode in the presence of methylene blue redox dye as a redox mediator indicated a linear response in the wide concentration range of 2 pg/mL to 2 μg/mL of complementary target genomic DNA (parasite DNA), suggesting the potential of this biosensor for diagnosis of Visceral leishmaniasis (Mohan et al., Reference Mohan, Srivastava, Maheshwari, Sundar and Prakash2011). In addition, a gold nanoleaf-based biosensor was constructed by immobilizing Leishmania major-specific DNA for detecting parasite infection. Further analysis indicated that the biosensor could detect a synthetic DNA target in the range of 1.0 × 10−10 to 1.0 × 10−19 mol L−1 with a detection limit of 1.8 × 10−20 mol L−1, and genomic DNA in the range of 0.5–20 ng μL−1 with a detection limit of 0.07 ng μL−1. More importantly, the biosensor could not only detect L. major in patient samples but also distinguish L. major from a non-complementary-sequence oligonucleotide and L. tropica with high selectivity (Moradi et al., Reference Moradi, Sattarahmady, Rahi, Hatam, Sorkhabadi and Heli2016).
Recently, several electrochemical biosensors were also developed for detecting nucleic acid biomarkers for helminth infection. For example, Santos et al. used AuNPs that are the most commonly used nanomaterial in biosensor development through biomolecule conjugation and then obtained compelling sol–gel derivative films to immobilize AuNPs using the organosilane, 3-mercaptopropyltrimethoxysilane. Further analyses indicated that the biosensor could recognize the S. mansoni genome sequence at different concentrations in samples of urine, CSF and serum with the limit detection of 0.6 pg μL−1 (Santos et al., Reference Santos, Caldas, Melo, Bruscky, Silva, Wanderley, Andrade and Oliveira2019). The authors also reported the development of another biosensor by using a self-assembled monolayer of mercaptobenzoic acid immobilizing nanostructures composed of gold nanoparticles (AuNPs) and magnetite nanoparticles (Fe3O4_NPs) to recognize specific nucleotide sequence of S. mansoni present in CSF and serum samples, and this biosensor showed a DNA detection limit of 0.781 and 0.685 pg μL−1 (Santos et al., Reference Santos, Andrade, Bruscky, Wanderley, Melo and Oliveira2017).
Apart from the detection of nuclear acid biomarkers, some biosensors were also developed to detect specific antibodies/antigens related to helminth infection. For example, a piezoelectric immunosensor assay was developed with immobilizing immunoglobulin G (IgG) as a probe to detect S. japonicum circulating antigens. By immobilizing purified IgG from infected rabbit's sera with S. japonicum, the developed biosensor was shown to specifically detect S. japonicum circulating antigens (Cheng et al., Reference Cheng, Chen, Chen and Chen2008). In addition, a superhydrophobic surface-based magnetic electrochemical immunoassay for the detection of S. japonicum antibodies was also developed and the results indicated that the biosensor is quantitatively sensitive to SjAb concentrations ranging from 2 ng mL−1 to 15 μg mL−1, with a detection limit of ~1.3 ng mL−1 (Nie et al., Reference Nie, Zhang, Wang, Wang and Shen2012). Moreover, an electrochemical immunosensor was also developed by immobilizing W. bancrofti epitope antigens (Wb/ALT2-A5) on a screen-printed carbon electrode by their amine groups via chitosan film by coupling with glutaraldehyde as the crosslinker. The detection limitation of this biosensor was 0.002 μg mL−1 for human serum with a sensitivity of 1.86 μA (Prado et al., Reference Prado, Mendes, Souza, Dutra and De-Simone2018).
Optical detection techniques
Optical detection techniques are those that can sense phenomena related to the interaction of target compounds with the analytes. Usually, these biosensors can measure luminescence, fluorescence or colour changes. Several biosensors have used optical detection techniques to detect nucleic acid biomarkers for parasite infection, particularly for malaria. For example, Ittarat and coworkers described a genosensor based on quartz crystal microbalance (QCM) to detect malaria infection. In this method, the QCM surface was immobilized with a malaria biotinylated probe. Specific DNA fragments of malaria-infected blood were amplified. Hybridization between the amplified products and the immobilized probe resulted in quartz frequency shifts that were measured by an in-house frequency counter. Further analysis indicated that this biosensor can differentially diagnose blood infected with P. falciparum from that infected with P. vivax and no cross reaction with human DNA (Ittarat et al., Reference Ittarat, Chomean, Sanchomphu, Wangmaung, Promptmas and NgrenngarmLert2013). In helminths, a diffraction-based optical biosensor was developed although it detects a protein biomarker (a 31-kDa recombinant antigen called NIE derived from S. stercoralis) to diagnose strongyloidiasis. The biosensor showed excellent agreement (κ = 0.98) with results obtained by an NIE-based ELISA method (Pak et al., Reference Pak, Vasquez-Camargo, Kalinichenko, Chiodini, Nutman, Tanowitz, McAuliffe, Wilkins, Smith, Ward, Libman and Ndao2014).
In addition, as stated above, miRNAs are recognized a novel kind of biomarker for disease diagnosis, particularly for cancer, for example in early diagnostics, predicting responses to therapy, monitoring relapse and molecular classification of tumours. A recent study documented that circulating miRNAs associated with cancer derived from serum can be detected with a biosensor using DNA concatemer amplification (Hong et al., Reference Hong, Chen, Liu, Li, Yang, Chen and Chen2013). If sensitive and effective nucleic acid biomarkers can be identified during helminth infection, and suitable and effective probe layers can be immobilized on a transducer surface, we believe that electrochemical biosensors can become a next generation technique for diagnosis of helminth infections.
All the nucleic acid-based methods discussed and the related targets for detecting helminth infections are summarized and listed in Table 1.
Table 1. Nucleic acid-based methods for the detection of helminth infections
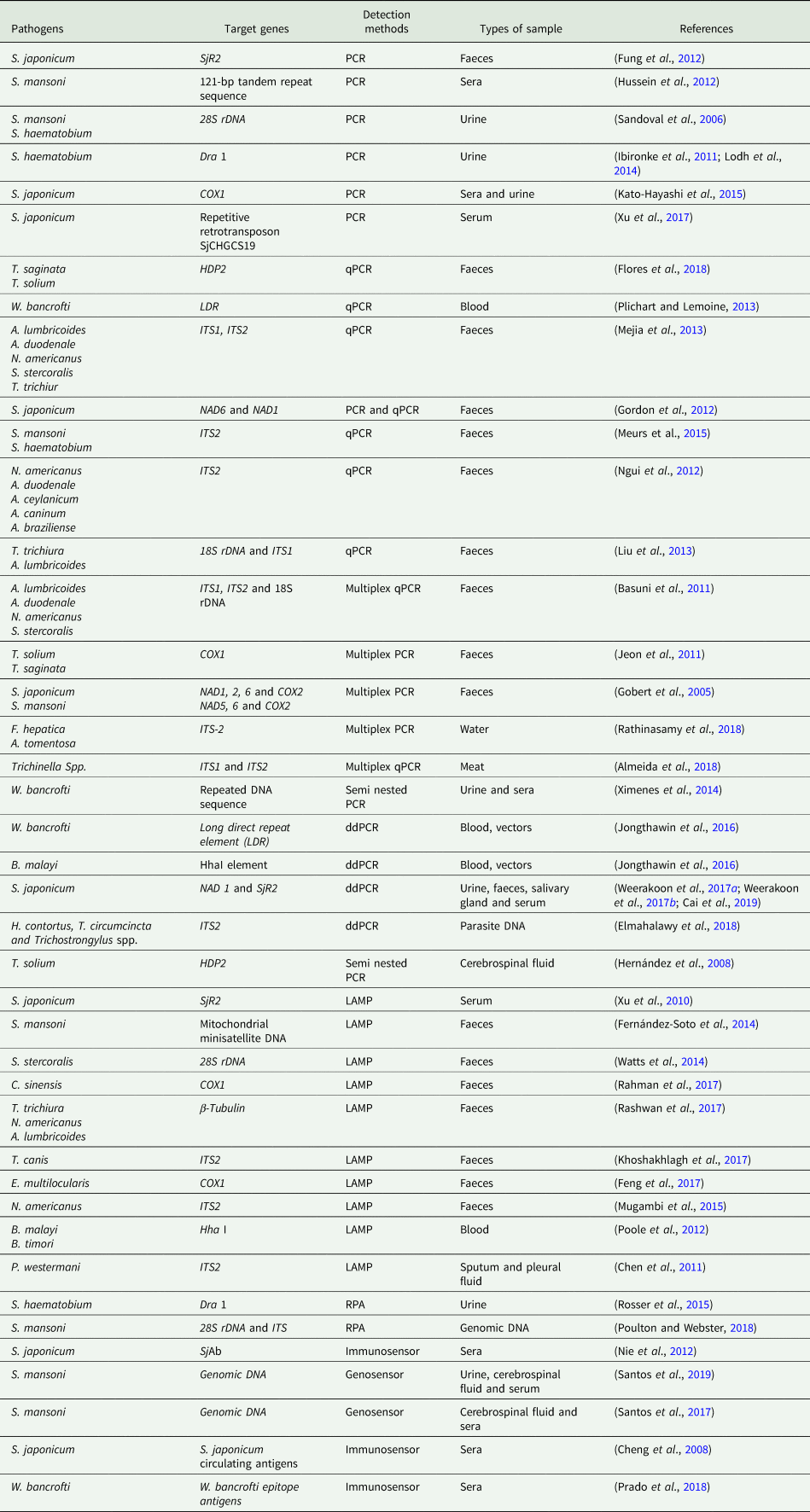
Advantages and limitations of nucleic acid-based methods for detection of helminth infections
Each nucleic acid-based method has its own advantages and limitations. We have summarized them as follows. The PCR is one of the most promising approaches to detect parasite DNA or RNA in human, animal or vector samples owing to its higher diagnostic sensitivity and specificity compared to microscopic examination or immunodiagnostics. However, it has limitations, such as the high cost of the instrumentation required for thermal cycling and signal detection, and the need for well-equipped laboratories (Verweij and Stensvold, Reference Verweij and Stensvold2014). Both qPCR and ddPCR methods can quantitatively determine helminth infection as they are amenable for automation and have relatively high-throughput, whereas the ddPCR can increase the accuracy of quantification at low-target concentrations. Unlike the PCR, LAMP offers sensitivity, high efficiency, rapidity and simplicity for identifying certain infectious diseases, although the specificity is yet to be validated. The main shortcoming of LAMP is the false-positive production caused by non-specific amplification resulting from primers, which could be improved by optimization of the reaction conditions, primer design, detection approaches, etc. RPA offers several advantages such as operation at a low and constant temperature, without the need for an initial denaturation step or the use of multiple primers. Biosensor-based methods have the potential to deliver rapid, accurate and affordable diagnosis of parasite infection. In addition, biosensors can improve diagnosis and follow-up treatment in terms of rapidity, real-time performance and expense compared to current detection technologies such as PCR and ELISA. Furthermore, the use of biosensors offers significant advantages such as small fluid volume manipulation and high-integration capability that facilitates the development of portable devices and ease of use, allowing use by non-specialized personnel in non-centralized laboratories (Sin et al., Reference Sin, Mach, Wong and Liao2014). The time-consuming preparative steps of gene probe evaluation make it hard to consider these as the basis of biosensors for in situ detection of pathogenic microorganisms. Although extensive efforts have been expended to develop biosensors, a relatively small number of analytics, particularly toxic materials, can be detected using commercially available devices (Alhamoud et al., Reference Alhamoud, Yang, Kenston, Liu, Liu, Zhou, Ahmed and Zhao2019). Although recent advancements in nanotechnology are facilitating the development of smaller, less-expensive, multiplex, easy-to-use and fully integrated biosensors for diagnostics and food and water safety (Bouguelia et al., Reference Bouguelia, Roupioz, Slimani, Mondani, Casabona, Durmort, Vernet, Calemczuk and Livache2013; Verbarg et al., Reference Verbarg, Plath, Shriver-Lake, Howell, Erickson, Golden and Ligler2013; Chung et al., Reference Chung, Kang, Jurng, Jung and Kim2015), future research must also focus on simplifying their fabrication techniques, miniaturizing devices and developing the biocompatibility and environmental friendliness of the nanomaterials. Unfortunately, the preparation and manipulation of nanomaterials in a reproducible and economic fashion is still premature. More importantly, the development of point of care devices is the ultimate goal, particularly for human patients, which still faces a significant challenge. Consequently, techniques that employ and combine with chemistry, electrics, optics, material science and mechanical passivation approaches could speed up the development of biosensors for commercial requirements. The advantages and limitations of each nucleic acid-based method are summarized in Table 2.
Table 2. Summary of advantages and limitations of each nucleic acid-based method

Conclusions
Traditional pathogen detection methods are usually time consuming, and associated with low sensitivity, selectivity or accuracy. Therefore, sensitive and specific methods for diagnosing parasitic diseases are urgently required. Recent advances in PCR, qPCR, ddPCR, LAMP and RPA are proving to be highly sensitive and specific diagnostic approaches. By identifying effective biomarkers during helminth infection, these approaches could be further improved by increasing the sensitivity of detection and diagnosis. Current biosensors have the potential to provide rapid, accurate and affordable diagnoses of parasite infection by offering significant advantages such as small fluid volume manipulation, high integration capability that facilitates the development of portable devices and ease of use. Therefore, the combination of these outcomes with advanced technologies such as nucleic acid-based biosensors may change the current scenario of clinical diagnosis.
Financial support
This study was, in part or in whole, supported by the National Key Research and Development Program of China (2017YFD0501306-3), the National Natural Science Foundation of China (Grants No. 31472187 and 31672550) and the Science & Technology Innovation Program of the Chinese Academy of Agricultural Sciences.
Ethical standards
Not applicable.
Conflict of interest
The authors declare that there are no conflicts of interest