INTRODUCTION
Accurate description and delimitation of parasite species has implications for biodiversity assessment, parasite-host evolutionary theory and the surveillance of parasites with medical and veterinary importance (de Leon and Nadler, Reference de Leon and Nadler2010). Molecular characterization of species can reveal morphology masking multiple distinct cryptic species. Re-evaluation and formal naming of such cryptic species reduces systematic confusion and improves understanding of diversity (Schlick-Steiner et al. Reference Schlick-Steiner, Seifert, Stauffer, Christian, Crozier and Steiner2007).
We have discovered, using molecular phylogenetics, 2 tentatively new myxosporean species in the gallbladders of frogs that have recently spread across eastern Australia and cause disease (Hartigan et al. Reference Hartigan, Fiala, Dyková, Jirků, Okimoto, Rose, Phalen and Šlapeta2011). Based on morphology, all myxospores from Australian frog gallbladders were assumed to represent an introduced species Myxidium immersum (=Cystodiscus immersus Lutz, 1889) originating from South America (Delvinquier, Reference Delvinquier1986). Retrospective analysis of museum specimens of frogs dating back to 1890 confirmed the emergence of this morphotype in Australia in the second half of the 20th century. No spores were found in introduced or native frog species until 30 years after the introduction of the Cane toad (Rhinella marina) (Hartigan et al. Reference Hartigan, Phalen and Šlapeta2010). Sequencing of small subunit (SSU) rDNA, internal transcribed spacer (ITS) and large subunit (LSU) rDNA of M. cf. immersum (=C. cf. immersus) spores from South American and Australian spores confirmed their distinct evolutionary trajectories (Hartigan et al. Reference Hartigan, Fiala, Dyková, Jirků, Okimoto, Rose, Phalen and Šlapeta2011). The 2 tentative new myxosporean species have been identified in a range of native frogs as well as the introduced Cane toad; however, the full extent of frog species they can infect and the degree of disease they cause in different species is yet unknown. It is deemed necessary to formally name these species to facilitate further research on myxosporean species affecting amphibians. Such action will facilitate establishment of baseline data and approaches to delineate parasite species in globally urgent conservation efforts of amphibian populations.
The aim of this study was to formally name the 2 myxosporean species affecting Australian frogs and to resurrect the genus Cystodiscus Lutz, 1889 for myxosporean species producing spores in the gallbladder of amphibians. We used morphological, ultrastructural and genetic techniques as a basis for our descriptions. We identified distinct differences in spore ultrastructure and present a species-specific multiplex PCR that unequivocally differentiates both species within a single DNA sample.
MATERIALS AND METHODS
Collection of individuals
Frogs and tadpoles collected for this study are listed in Table 1. Southern bell frog Litoria raniformis adults (n=7) were retrieved from the Australian Registry of Wildlife Health (ARWH). These animals were wild caught in Coleambally, NSW and kept in Sydney, NSW under laboratory conditions as part of a breeding study (Mann et al. Reference Mann, Hyne and Choung2010). All other frogs were wild-caught tadpoles and adult frogs collected under the University of Sydney animal ethics protocols (N00/9-2008/3/4855 and N00/9-2009/3/5134) and Australian National Parks and Wildlife Service scientific licenses (S12686/12969). All host names follow the recommended nomenclature of Frost (Reference Frost2011).
Table 1. Summary of host species parasitized by Cystodiscus axonis sp. n. and Cystodiscus australis sp. n.

* TEM (brain); &TEM (liver); ◊plasmodia without myxospores; n.a., material not available; +, PCR positive; −, PCR negative.
Light microscopy
Samples submitted for histological examination were fixed in fresh 10% buffered formalin and processed into paraffin blocks (Veterinary Pathology Diagnostic Services, University of Sydney). Blocks were sectioned (5 μm) and stained with haematoxylin and eosin (H&E) or Giemsa. Wet mounts of spores in phosphate-buffered solution (PBS, pH 7·2) or 70% ethanol were examined. Thirty spores were measured (except in the case of ARWH 6092.55b) according to the method of Lom and Arthur (Reference Lom and Arthur1989) and terminology used as defined in Fig. 1. Sections and wet mounts were viewed using a X100 oil-objective on an Olympus BX60 microscope equipped with a DP70 camera (Olympus Australia).
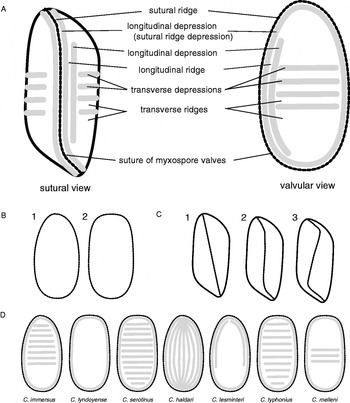
Fig. 1. Composite drawing of spores of Cystodiscus spp. (A) Major characteristics of the bivalved myxospore in sutural and valvular view. The suture is accompanied by a thickened ridge parallel to the suture and a longitudinal sutural ridge depression. (B) Depressions delineated by raised ridges. Valvular view shapes: ovoid (1) or ellipsoid (2). (C) Sutural view types indicating the course of the valve suture: straight (1), S-shaped (2), Z-shaped (3). (D) Characteristics of myxospores from valvular view.
Transmission electron microscopy
Materials for transmission electron microscopy (TEM) were fixed overnight in cacodylate buffered 2·5% glutaraldehyde (pH 7·0), post-fixed with 2% osmium tetroxide, dehydrated through graded ethanols (50–100%) and embedded in Spurr's resin. Ultrathin sections (70 nm) were stained with uranyl acetate and lead citrate. Copper mesh grids (200 nm) were viewed on a JEOL JEM 1010 transmission electron microscope at the Institute of Parasitology, Biology Centre of the ASCR, Czech Republic.
Scanning electron microscopy
Myxospores for scanning electron microscopy (SEM, see Table 1) were fixed in cacodylate-buffered 2·5% glutaraldehyde (pH 7·0), allowed to settle on poly-L-lysine (Sigma, Australia) coated coverslips and post-fixed with 2% osmium tetroxide in cacodylate buffer (pH 7·2). Specimens were dehydrated with a graded ethanol series (50–100%), critical-point dried and coated with a 0·2 nm layer of gold (Au) for 2 min. Specimens were observed on a Phillips XL-30 CP at the Australian Centre of Microscopy and Microanalysis, Australia.
Amplification of internal transcribed spacer rDNA
DNA from frozen (−20°C) frog brain and liver and from myxospores was extracted using the PureLink DNA Kit (Invitrogen Australia, Victoria, Australia) according to the manufacturer's instructions. Extracted DNA from all samples was resuspended in a final volume of 50–200 μl. DNA was quantified using Nanodrop 1000 spectrophotometer (Thermo Scientific, NSW, Australia). Amplification of the ITS rDNA region using myxosporean specific oligonucleotides S0108 (G-ITS-for) (5′-GGG ATC CGT TTC CGT AGG TGA ACC TGC-3′) and S0109 (ITS-Zschok-rev) (5′-GAT TCT CAT AGT AAC TGC GAG TG-3′) was carried out as previously described by Hartigan et al. (Reference Hartigan, Fiala, Dyková, Jirků, Okimoto, Rose, Phalen and Šlapeta2011). PCR products were purified with PCR Micro Kit (Invitrogen Australia, Victoria, Australia) and directly sequenced with amplification primers at the Australian Genomic Research Facility (Sydney, Australia). Chromatographs were assembled and aligned with related sequences using the CLC Main Workbench 5.5 (CLC bio, Denmark). The ITS rDNA region sequences were deposited into GenBank under the Accession numbers JN603234, JN603235.
Species-specific diagnostic PCR
Species-specific primer sets were designed with CLC Bio Workbench based on ITS region rDNA unit sequences described here and in Hartigan et al. (Reference Hartigan, Fiala, Dyková, Jirků, Okimoto, Rose, Phalen and Šlapeta2011), see Supplementary Fig. 1; online version only. Primer set 1 amplifying ITS region of C. axonis S0116 (Br150 forward) (5′-GTA ATG TGT TTT ACA GGC AAT TGA C-3′) and S0119 (Br760 reverse) (5′-TGC AGA GTA ACT AGA ATT GC-3′). Primer set 2 amplifying ITS region of C. australis S0110 (Liv200 forward) (5′-CTG AAC TTT CTA TGC CAG TTA C-3′) and S0113 (Liv720a reverse) (5′-CAT TAT AAG CAA TAT CCG TAA GA-3′). All primers were from Sigma-Aldrich (Australia).
Myxozoan DNA from fish (n=39, see Supplementary Table 1; online version only), bryozoa (Tetracapsuloides bryosalmonae), amphibian (Sphaerospora ranae), reptile (Myxidium chelonarum) and mammal (Soricymyxum fegati) were used to test the specificity of the diagnostic primers. Species were selected to represent a diverse selection from phylogenetic clades in marine and freshwater Myxozoa (Bartošová et al. Reference Bartošová, Fiala and Hypša2009).
PCR reactions (25 μl) were performed with 2x EconoTaq PLUS GREEN MasterMix (Lucigen, USA) or 2x MyTaq RedMix (Bioline, Australia). Alternatively PCR was run using 1 U of Taq-Purple polymerase and a 5x PCR buffer (Top Bio, Czech Republic) with 250 μM of each dNTP (Invitek, Germany). Primer sets were used individually in species-specific PCR or in combination in species-specific multiplex PCR (each 10 pmol concentration). Positive and negative controls were run with each reaction. The PCR cycling conditions were initiated with 95°C for 5 min, then 35 cycles (95°C for 0·5 min, 57°C for 1 min, 72°C for 1 min) and concluded with 72°C for 7 min. Results were resolved on a 2% agarose gel stained with GelRed (Biotium) and visualized with a UV illuminator. Positive amplification for primer set 1 resulted in 595 bp amplicon and for primer set 2 resulted in 497 bp; mixed infections were identified by the presence of both amplicons.
Phylogenetic analysis
The dataset for phylogenetic analysis consisted of SSU rDNA sequences of selected species of the freshwater clade according to Fiala (Reference Fiala2006) and included all Cystodiscus spp. and Myxidium spp. Sequence of marine Chloromyxum leydigi was selected as an outgroup. The alignment of 27 sequences was constructed using MAFFT v6.626b (Katoh et al. Reference Katoh, Kuma, Toh and Miyata2005) with G-INS-i algorithm and default settings. Ambiguous characters were removed using program Gblocks v0.91b (Castresana, Reference Castresana2000) with less stringent parameters (14, 14, 8, 8 and with half). The final alignment consisted of 1853 positions (78% of the original alignment). Maximum likelihood (ML) analysis was carried out by program RAxML (Stamatakis, Reference Stamatakis2006) with GTRGAMMA model and 500 bootstrap replicates. Maximum parsimony (MP) was performed in program PAUP* 4.0b10 (Swofford et al. Reference Swofford, Waddell, Huelsenbeck, Foster, Lewis and Rogers2001) with heuristic search, random addition of taxa and Ts:Tv=1:2. Bootstrap support was calculated from 1000 replicates.
RESULTS
Scanning electron microscopy (SEM) and transmission electron microscopy (TEM) of myxosporean stages identified in Australian frogs have revealed yet undescribed myxosporean species. Our current ultrastructural investigation and the molecular characterization by Hartigan et al. (Reference Hartigan, Fiala, Dyková, Jirků, Okimoto, Rose, Phalen and Šlapeta2011) form the basis for a formal description of 2 new parasite species belonging to the genus Cystodiscus Lutz, 1889.
Myxozoa Grassé, 1881
Myxosporea Bütschli, 1881
Bivalvulida Shulman, 1959
Variisporina Lom and Noble, 1984
Myxidiidae Thélohan, 1982
Cystodiscus Lutz, 1889
Cystodiscus axonis sp. n.
Description: Ellipsoidal myxospores with 4–8 transverse ridges (3–7 transverse depressions) on the outside of 2 valves visible under light microscopy and SEM (Fig. 2A–E). Spore width and length 14·5 (13·0–15·0)×8·7 (8·0–10·0) μm and polar capsules 4·5 (4·0–6·0)×4·5 (4·0–5·0) μm with 4–5 turns of the polar filament (n=30, in PBS) (Table 2). Two spore valves joined by a raised ridge that varied from straight to curved (S-shaped in sutural view). A sutural depression accompanied the raised ridge (sutural ridge) from pole to pole but was not encircling. The sutural depression 0·8–1·0 μm tapered at the polar ends of the spore. The sutural ridge was thicker at the polar ends of the spore where the polar filament pore was situated (Fig. 2B–E). The depressions on the spore surface were centrally located and sometimes oriented diagonally rather than parallel (Fig. 2A). The depressions did not always extend across the entire valve surface (Fig. 2A and C), some depressions only covered one third to one half of the spore surface. The ridges or depressions were never observed to be branching or longitudinal. Filiform polar appendages (FPA) were attached to each end of the spore, these appeared to be delicate and were subject to breakage during SEM preparation (Fig. 2B). The FPA were visible using a bright-field microscope with a X100 objective, all spores observed had 1 or 2 FPA on each spore rounded end (Fig. 2A–E). Using SEM, of the spores with both polar ends seen, 64·3% (9/14) had 1 FPA at one end and 2 at the other (Fig. 2C). Smaller percentages had only 1 FPA seen at each end (21·4%, 3/14) or 2 FPA at each end (14·3%, 2/14) (Fig. 2B, E). The majority of spores with only the end available for observation had a single FPA (77·7%, 7/9) (Fig. 2E) compared to having 2 FPAs at one end (22·2%, 2/9) (Fig. 2A). The FPA were single stranded with a diameter of 200–300 nm along their entire length possessing a larger rounded terminus on SEM (Fig. 2C, D). The longest observed FPA possessing the rounded terminus was 20 μm, the shortest seen was 1·5 μm. However, in many cases, measurement of the FPA was obscured by their conformation on SEM, i.e. curled, twisted or broken. The FPA were present on spores with (Fig. 2C) and without ejected polar filaments (Fig. 2A, B, D–F). The polar filaments were easily distinguished from FPAs by greater length (>50 μm), width (0·5–0·7 μm) and the twisted rope appearance of the polar filament (Fig. 2C). Free spores were observed in the gallbladder, but no plasmodia containing spores were seen. Pre-sporogonic developmental stages seen in histology (H&E, Giemsa) appeared similar in CNS and meninges (Fig. 4C). The species identity has been confirmed using species-specific multiplex PCR (Fig. 3). The species was previously reported as the “Myxidium sp. brain genotype” by Hartigan et al. (Reference Hartigan, Fiala, Dyková, Jirků, Okimoto, Rose, Phalen and Šlapeta2011).

Fig. 2. Myxospore morphology of Cystodiscus axonis sp. n. from Litoria raniformis (A–F, hapantotype, ARWH 6985.6) and Cystodiscus australis sp. n. from Limnodynastes peronii (G–K, hapantotype, ARWH 6092.20f). (A–C) Scanning electron micrograph (SEM) of C. axonis sp. n. myxospores with filiform polar appendages (FPA) (white arrows) emerging from sutural ridges (A) or spore valve (C, D), polar filaments are distinctly thicker (grey arrows) than appendages. (D) Magnification of B showing attachment of the FPA to the valve surface and rounded terminal end of FPA (black arrow). (E) SEM of appendage showing the fine structure of FPAs. (F) Light microscopy C. axonis sp. n. showing FPAs (arrows) and polar filament coils in polar capsules (arrowhead). (G, H) SEM of C. australis sp. n. myxospores. (I) Light microscopy of C. australis sp. n. with polar filament coils in polar capsules (arrowhead). (J, K) High magnification view of myxospore end showing rounded ends of sutural depression and polar filament eversion pore (J) and myxospore showing everted polar filament with twisted rope appearance (K). Scale bars: A–E, G, H=2 μm; F, I=10 μm; J, K=1 μm.

Fig. 3. Cystodiscus species-specific multiplex diagnostic PCR based on ITS rDNA region. Presence of single approximately 600 bp (primers set 1) and 500 bp (primer set 2) product is indicative of C. axonis sp. n. and C. australis sp. n., respectively (see Materials and Methods section). Presence of both products indicates mixed infection. (A) Negative control (water). (B) C. axonis sp. n. from brain tissue of type host Litoria raniformis (ARWH 6985.6). (C) C. axonis sp. n. from gallbladder myxospores of type host Litoria raniformis (ARWH 6985.6). (D) C. axonis sp. n. from tadpole liver L. aurea (ARWH 6092.14a). (E) Brain tissue of Rhinella marina (adult, ARWH 6092.45.7) (F) Gallbladder myxospore sample showing mixed Cystodiscus infection (G–H). Mixed Cystodiscus spp. infection in tadpole liver L. aurea (ARWH 6092.5a (G) and ARWH 6092.10a (H)). (I) C. australis sp. n. from immature gallbladder plasmodium in L. aurea tadpole (ARWH 6092.37a). (J) Rhinella marina (adult, ARWH 6092.USC10) with C. australis sp. n. infection. (K) Brain tissue of type host of C. australis sp. n. (Limnodynastes peronii, adult, ARWH 6092. 20f). (L) C. australis sp. n. myxospores from type host Limnodynastes peronii, adult, ARWH 6092. 20f. Size DNA ladder is on the left. Agarose gel (2%) stained with GelRed (Biotium).
Table 2. Summary of morphological characteristics of Cystodiscus spp. myxospores in Australian frogs

Type host: The Southern bell frog, Litoria raniformis Keferstein, 1867 (Hylidae, Anura), carcass not available for deposition.
Type locality: Wild animal collected in Coleambally, New South Wales, Australia (−34° 47′ 49″ S,145° 59′ 39″ E) and kept captive in Sydney, New South Wales until May, 25th 2009.
Species and genus type material (hapantotype): G.17758. Histological slides of the liver, CNS, gut and gonad (H&E stain), SEM stubs and spores in 70% ethanol deposited in the Australian Museum, Sydney, New South Wales, Australia.
Additional material: Formalin-fixed paraffin-embedded blocks, unfixed tissues in −80°C of the type host L. raniformis at the Australian Registry of Wildlife Health, Mosman, NSW, Australia (ARWH 6985.6); the type belongs to a cohort of adult L. raniformis ARWH 6985.1-7.
Nucleotide signature sequenced data: HQ822168, HQ822169, HQ822171 – SSU rDNA; JN603234, JN603235 – ITS1 rDNA, 5·8S rDNA, ITS2 rDNA (GenBank Accession numbers); “Myxidium sp. brain genotype” sensu Hartigan et al. (Reference Hartigan, Fiala, Dyková, Jirků, Okimoto, Rose, Phalen and Šlapeta2011).
Etymology: The species epithet is derived from the anatomical term ‘axon’ to indicate the location of the developmental stages of this species in the host. The name is derived as Latin singular genitive.
Other hosts and locality of Cystodiscus axonis sp. n.:Table 1 lists frog species infected with C. axonis sp. n. Adults of L. raniformis from the same cohort as the type host (ARWH 6985.6) were infected with C. axonis sp. n. (ARWH 6985.1-5, 7). Myxosporean stages were identified in the brain and CNS, occasionally associated with glial inflammation (Hartigan et al. Reference Hartigan, Fiala, Dyková, Jirků, Okimoto, Rose, Phalen and Šlapeta2011). Myxosporean developmental stages within myelinated axons were identified in the CNS and dorsal nerve roots (Fig. 4) of 3 native frogs in Riverstone, outskirts of Sydney, NSW and considered conspecific with stages detected in L. raniformis (ARWH 6985.1-7). Numerous C. axonis sp. n. plasmodia were seen throughout the brain and CNS of Litoria peronii (adult, ARWH 6092.29a) (Fig. 4A), the pre-sporogonic plasmodia were found on the periphery of the CNS and were associated with microgliosis (Fig. 4B). The immature stages in L. peronii ARWH 6092.29a were indistinguishable from C. axonis sp. n. in the meninges of L. raniformis (type host ARWH 6985.6) (Fig. 4C) and the dorsal root nerve of Litoria aurea (adult, ARWH 6092.36a) (Fig. 4D and E). The CNS stages in L. peronii (adult, ARWH 6092.29a) and L. aurea (adult, ARWH 6092.36a) were confirmed to be C. axonis sp. n. with species-specific PCR and nucleotide sequencing (Hartigan et al. Reference Hartigan, Fiala, Dyková, Jirků, Okimoto, Rose, Phalen and Šlapeta2011). A third frog from Riverstone NSW Limnodynastes peronii (adult, ARWH 6092.55b) was identified with C. axonis sp. n. developmental stages in the brain and spores in the gallbladder (Table 2) (confirmed with species-specific PCR) (Fig. 3). Pre-sporogonic plasmodia in the bile ducts of L. aurea (tadpoles ARWH 6092.14a) (Fig. 4) were confirmed to be C. axonis. sp. n. with species-specific multiplex PCR (Fig. 3). Infection with C. axonis sp. n. of this tadpole specimen was associated with lymphoplasmocytic inflammation and hyperplasia of the biliary system.

Fig. 4. Histology of nervous tissue with pre-sporogonic development of Cystodiscus axonis sp. n. (A) Distribution of myxosporean plasmodial stages (arrows) within spinal cord of Litoria peronii (adult, ARWH 6092.29a). Note concentration of myxosporean development at the periphery. (B) Higher magnification of the spinal cord of L. peronii (adult, ARWH 6092.29a). (C) Pre-sporogonic plasmodium in the meninges of an adult Litoria raniformis (type host, ARWH 6985.6). (D) Dorsal root nerve of Litoria aurea (adult, ARWH 6092.36a) showing glial infiltration associated with the presence of Myxosporea. (E) Higher magnification of the pre-sporogonic plasmodia in the dorsal root nerve of L. aurea (adult, ARWH 6092.36a). Scale bars: A=500 μm, B–C=20 μm, D=50 μm, E=20 μm.
An adult R. marina (ARWH 6092.45.7) from Lismore, NSW was identified with a mixed infection of Cystodiscus species. The brain stages were unequivocally confirmed as C. axonis sp. n. only using species-specific multiplex PCR (Fig. 3). Mixed infection was confirmed for spores in the gallbladder using species-specific multiplex PCR (Fig. 3). Similarly, tadpole livers (ARWH 6092.5a and ARWH 6092.10a) of L. aurea were identified by species-specific multiplex PCR to contain a mixed infection of Cystodiscus species (Fig. 3).
Ultrastructure of intra-axonal plasmodia of Cystodiscus axonis sp. n.: Brain tissue of L. aurea (adult ARWH 6092.36a), confirmed to be infected with C. axonis sp. n. by PCR, showed myxosporean stages in semi-thin sections. The stages were characterized by a large plasmodium with numerous daughter cells within, located exclusively within myelinated axons (Fig. 5C). Brain tissue samples from L. raniformis (ARWH 6985.4-5), L. aurea (ARWH 6092.10a), and Lim. peronii (ARWH 6092.55b) were processed for TEM but no myxosporean development was detected. Using TEM, multiple myxosporean development stages were observed throughout the sample of L. aurea (ARWH 6092.36a). The myxosporean stages observed included a single cell enveloping one daughter cell or more complex myxosporean cell formations, including secondary and tertiary cell development (Fig. 5D and E). All stages observed were pre-sporogonic. The secondary cells had only mitochondria and glycogen granules and the tertiary cells appeared to have very little cytoplasm with 1 or 2 mitochondria (Fig. 5E). Microtubules were seen throughout the large primary cell cytoplasm and numerous mitochondria with tubular cristae (Fig. 5F). More than 1 plasmodial body was observed in an axon, often one was significantly smaller than the other (Fig. 5G). The individual plasmodia were never connected to each other or the axonal sheath. No pseudopodia were observed on the external surfaces of these stages. The primary cells had a high degree of multicellularity, the cytoplasm contained numerous vacuoles, glycogen granules and Golgi apparatus (Fig. 5G). Despite the complexity of these developmental stages no sporogonic activity was observed.

Fig. 5. Histological and ultrastructural morphology of Cystodiscus axonis sp. n. in L. aurea tadpole (ARWH 6092.14a) and adult (ARWH 6092. 36a). (A) Histological section of C. axonis sp. n. pre-sporogonic plasmodia (arrows) in bile ducts of L. aurea tadpole (ARWH 6092.14a). (B) Biliary plasmodia at higher magnification (ARWH 6092.14a). (C) Transmission electron micrograph of C. axonis sp. n. plasmodium in myelinated axon of L. aurea adult (ARWH 6092.36a) showing secondary and tertiary daughter cells within the primary cell. (D) Secondary cell (Sc) and nucleus (Sn) with internal tertiary cell (Tc) of C. axonis sp. n. (ARWH 6092.36a). (E) Microtubules (arrow) throughout the cytoplasm of the primary of C. axonis sp. n. plasmodium (ARWH 6092.36a). (F) A plasmodium budding off another plasmodium within a single axon, note absence of attachment to the axonal sheath (ARWH 6092.36a). (G) A primary cell seen with tubular cristae mitochondria (m), Golgi apparatus (arrow), and glycogen granules (Gg) in the cytoplasm of the cell. Scale bars: A=100 μm, B=20 μm, C=5 μm, D–E=1 μm, F=2 μm, G=1 μm.
Cystodiscus australis sp. n.
Description: Ellipsoidal myxospores 5–11 transverse ridges (4–12 transverse depressions) on the outside each of the 2 valves visible under light microscopy LM and SEM (Fig. 2G–I). Spore width and length 16·0 (15·0–18·0)×8·7 (8·0–10·0) μm and polar capsules 5·3 (5·0–6·0)×4·8 (4·0–5·5) μm with 5–6 turns of the polar filament (n=30, in PBS) (Table 2). The 2 spore valves were joined by a raised ridge that appeared straight or slightly curved (S-shaped in sutural view) along the medial axis of the spore. The raised sutural ridge was accompanied by a depression that varied in thickness and depth from 0·5 to 1·5 μm at its widest point and tapered towards the poles (Fig. 2H, J, K). Transverse depressions on the surface of the spores varied in depth and length and appeared connected to the sutural ridge depression. The majority of the depressions seen were parallel. No FPAs were observed on any spores in either light microscopy or SEM. The polar filament eversion pores were approximately 500 nm in diameter (Fig. 2J). Polar filaments are 500 nm thick and had an appearance of 3 or more single filaments twisted around each other (Fig. 2 K). Polysporic gallbladder plasmodia ranged from 0·5 to 1·5 mm in diameter, <0·3 mm in thickness and were circular or oval in shape (Fig. 6C). The plasmodia were unattached to the gallbladder epithelium and could be seen without a microscope when the gallbladder was held against direct light. Spores were free floating or attached to the plasmodium. The species identity has been confirmed using species-specific multiplex PCR (Fig. 3). The species was previously reported as the “Myxidium sp. liver genotype” sensu Hartigan et al. (Reference Hartigan, Fiala, Dyková, Jirků, Okimoto, Rose, Phalen and Šlapeta2011).

Fig. 6. Histological and ultrastructural morphology of Cystodiscus australis sp. n. (A–C) Biliary and gallbladder development of Myxosporea from Litoria aurea tadpole (ARWH 6092.37a). (A) Lymphoplasmocytic inflammation of the bile ducts and proliferation of the bile tree associated with myxosporean development (arrows), H&E. (B) Immature pre-sporogonic developmental stages (arrows) in the biliary system, H&E. (C) An immature pre-sporogonic plasmodium from the gallbladder on black background. (D–K) Transmission electron microscopy of sporogenesis and myxospores within plasmodia from Rhinella marina (adult, ARWH 6092.USC10). (D) Early stage of sporogenesis, differentiation of sporoplasm (Sp), capsulogenic cell (Cc) and valve cell (Vc), (ARWH 6092.USC10). (E) Myxospore with defined valvogenic cell (n=valve cell nuclei) and sporoplasm, note clearly defined sutural ridge and depression (arrow indicating depression) (ARWH 6092.USC10). (F) Internal structure of the myxospore sutural ridge, note the microtubules lining the sutural depression (ARWH 6092.USC10). (G) Early definition of myxospore polar capsule, spiral pattern of the polar capsule wall is an artefact caused by sectioning angle. The external tube of capsular primordium is visible (*), this is prior to the formation of polar filaments. (H) Polar capsule wall thickened in a later stage of myxospore development, club-shaped polar filaments seen within the capsule. (I) Polar capsule development of the polar eversion pore (arrow), polar filaments appear S-shaped due to sectioning angle. (J) Cross-section of gall bladder plasmodium epithelium showing elongated projections on surface (arrow), and myxospore positioning adjacent to the primary cell wall. (K) Mature myxospore showing binucleate sporoplasm with the developed polar capsule, transverse ridges and depressions on the valve surface. Scale bars: A=20 μm, B–C=100 μm, D–E=1 μm, F=500 nm, G–I=1 μm.
Type host: Striped Marsh Frog, Limnodynastes peronii Dumeril and Bibron, 1841 (Myobatrachidae, Anura), carcass was deposited at the Australian Museum, Sydney, New South Wales, Australia (R.175753).
Type locality: Wild animal caught in Riverstone, New South Wales, Australia (−33°41′7″, 150°50′53″) on 19 September 2008.
Species type material (hapantotype): G.17756. Formalin-fixed spores in 70% ethanol deposited in the Australian Museum, Sydney, New South Wales, Australia.
Additional material: Myxospores in 70% ethanol and SEM stubs of Lim. peronii (AWRH 6092.34b) deposited at the Australian Museum (G.17757) and formalin-fixed paraffin-embedded blocks, unfixed tissues in −80°C at the Australian Registry of Wildlife Health, Mosman, NSW, Australia.
Nucleotide signature sequenced data: HQ822152 – SSU rDNA; HQ822193, HQ822194, HQ822195, HQ822196, HQ822203, HQ822208, HQ822211, HQ822212, HQ822215, HQ822216, HQ822221, HQ822222, HQ822223 – ITS1 rDNA, 5·8S rDNA, ITS2 rDNA; HQ822178, HQ822179 – LSU rDNA (GenBank Accession numbers); “Myxidium sp. liver genotype” sensu Hartigan et al. (Reference Hartigan, Fiala, Dyková, Jirků, Okimoto, Rose, Phalen and Šlapeta2011).
Etymology: The species epithet is derived from a noun in the genitive case, i.e. australis, from the geographical name Australia.
Other hosts and locality of C. australis sp. n.:Table 1 lists frogs infected with C. australis sp. n. Myxosporean plasmodia in the bile ducts of a L. aurea tadpole (ARWH 6092.37a) were confirmed to be C. australis sp. n. only using species-specific multiplex PCR (Fig. 3). The pre-sporogonic stages of C. australis sp. n. were associated with lymphoplasmocytic inflammation and proliferation of the biliary tree of the liver (Fig. 6A). The gallbladder contained pre-sporogonic plasmodia confirmed by species-specific multiplex PCR as C. australis sp. n. The pre-sporogonic plasmodial stages were free floating in the bile, appeared highly vacuolated and ranged in diameter from 20–150 μm (Fig. 6B). An adult R. marina (ARWH 6092.USC10) from Lismore, NSW was identified with plasmodia and spores in the gallbladder. The spores were morphologically identified as C. australis sp. n. and confirmed with species-specific multiplex PCR (Fig. 3).
Species-specific multiplex PCR was used to detect the presence of spores of both species (C. axonis sp. n. and C. australis sp. n.) in R. marina (ARWH 6092.45.7) and L. aurea tadpoles (ARWH 6092.5a and ARWH 6092.10a) (Fig. 3).
Ultrastructure of gall bladder C. australis sp. n. plasmodia with spores: Gall bladder plasmodia of R. marina (adult, ARWH 6092.USC10) were confirmed as C. australis sp. n. by species-specific multiplex PCR (Fig. 3). The plasmodia were fixed, embedded and sectioned to observe the ultrastructure of C. australis sp. n. The plasmodia contained various stages of spore development (sporogony) including spores identified throughout the cytoplasm. Early stages of sporogenesis were observed where valve, sporoblast and capsular cells had differentiated and started to envelope one another (Fig. 6D). Valve cells with thick cytoplasm and nuclei still present were seen covering sporoblast cells and capsulogenic primordium (Fig. 6D). Sporoblast cells developed a thick electron-dense layer, which defined the cellular boundary between sporoblast, valve cell, and capsular cell (Fig. 6D). The valve cells enlarged to envelop the sporoplasm and began to develop structural features (Fig. 6E). The sutural ridge and depression of the spore could be seen before the capsular primordium had begun to differentiate (Fig. 6E). The sutural ridge and adjacent depression was lined with microtubules (Fig. 6F). There was no indication of any FPAs or other external features to the spore valve. The capsular primordium or polar capsule development formed a circular structure with thick rings in its cell wall (Fig. 6G). The angle of sectioning could make the capsular wall appear spiralled, the external tube was still visible before development of the polar filaments (Fig. 6G). The polar filaments appeared S shaped (Fig. 6I), the definition of the polar filaments coincided with a thickening of the polar capsule wall (Fig. 6H–K) and development of a discharge pore (Fig. 6I, arrow). The surface of plasmodia was covered in villus-like projections that may represent pseudopodia (Fig. 6J). A lattice of electron-dense material and microtubules connected the plasmodial walls, spores were found along the lattice and close to the primary cell wall (Fig. 6J). Ridges and depressions on the surface of the spore valve were easily recognizable (Fig. 6K).
Remarks: Only 2 Myxidium-like species (=Cystodiscus spp.) were detected in Australian frogs (Hartigan et al. Reference Hartigan, Fiala, Dyková, Jirků, Okimoto, Rose, Phalen and Šlapeta2011). Both species have been identified in 3 major frog families (Hylidae, Myobatrachidae and Bufonidae). The spores of C. axonis sp. n. are distinguished from C. australis sp. n. by the presence of FPAs and pre-sporogonic stages in the CNS of affected frogs.
Ambiguous biliary stages of Cystodiscus in tadpoles
Cystodiscus species development in the bile ducts of tadpoles were deemed to be morphologically indistinguishable (tadpole livers of L. aurea, ARWH 6092.5a and ARWH 6092.10a, see Table 1). Histology of liver showed myxosporean developmental stages, multinucleated plasmodia, in the biliary tree (Fig. 7A, B; ARWH 6092.10a). The plasmodia appeared unattached to the biliary epithelium, oval to round and 5–50 μm in diameter. A single bile duct could contain multiple pre-sporogonic plasmodia. The myxosporean developmental stages were associated with inflammation and proliferation of the bile ducts (Fig. 7A, B). Morphologically similar stages were observed in L. aurea tadpoles ARWH 6092.14a (Fig. 5A and B) and ARWH 6092.37a (Fig. 6A and B) confirmed with species-specific multiplex PCR (Fig. 3) to be single species infections of C. axonis sp. n. and C. australis sp. n respectively. No morphological features were noted to be species specific based on histology. Identical myxosporean stages were seen in tadpoles of L. aurea (ARWH 6092.42 m), L. peronii (ARWH 6092.63i) and Lim. peronii (ARWH 6092.57e), the liver tissues of these individuals were not available for PCR.

Fig. 7. Cystodiscus species developmental stages in the bile ducts of tadpoles. (A–B) Myxosporean development in bile ducts of Litoria aurea (tadpole, ARWH 6092.10a). (A) Histological section of biliary ducts showing multiple immature free-floating plasmodial forms of Cystodiscus spp. n. (arrows), H&E. (B) Higher magnification of multinucleated plasmodia in bile ducts, H&E. (C–F) Transmission electron microscopy of myxosporean development in bile ducts of L. aurea (tadpole, ARWH 6092.42 m). (C) Early plasmodial stage with primary cell (Pc) and nucleus (Pn) with a single internal secondary cell (Sc) with nucleus (Sn). (D) Bile duct filled with myxosporean plasmodium with numerous internal secondary cells, the plasmodium has expanded to the limits of the biliary duct (arrow). (E) Plasmodium in a bile duct showing cellular complexity of primary cell, numerous vacuoles (v), mitochondria (arrows) and secondary cells within the cytoplasm. (F) Secondary cell at higher magnification, internal organization included nucleus, mitochondria with tubular cristae (m) and vacuoles (arrow). Scale bars: A=50 μm, B=20 μm, C=1 μm, D=5 μm, E=2 μm, F=1 μm.
Transmission electron microscopy was used to investigate the ultrastructure of Cystodiscus spp. developmental stages of L. aurea tadpole liver (ARWH 6092.42 m) which was not available for PCR. Plasmodia were only observed within the bile duct. No pseudopodia or other attachment structures were observed to connect the plasmodial cell to the biliary epithelium. The plasmodial development was asynchronous in ARWH 6092.42 m, stages observed ranged from a single cell enveloping a cell to more complex cellular differentiation (Fig. 7C–F). The early stages of development were simple primary cells with a single secondary cell within (Fig. 7C). These early stages were small and free floating within a bile duct. In contrast, the primary cells in advanced developmental stages became more complex with an increased number of secondary cells, the plasmodium expanded to the limits of the bile duct (Fig. 7D). Sometimes there was very little intercellular space between the plasmodial primary cell and the host's bile duct (Fig. 7D). Larger plasmodia were observed with higher levels of cellular organization, the primary cells in addition to secondary cells, had numerous mitochondria with tubular cristae, electron-dense lipids and vacuoles (Fig. 7E). Secondary cells were relatively simpler in cellular organization. The cytoplasm of secondary cells mostly contained mitochondria (Fig. 7F) and vacuoles containing granules (Fig. 7F arrow). Despite the detail and intracellular proliferation seen within myxosporean primary cells in the bile ducts, no tertiary cells or sporogenesis were observed.
Phylogenetic position of the genus Cystodiscus
Sequences of all Cystodiscus species clustered together in both ML and MP analyses with a maximum bootstrap support for the clade monophyly (Fig. 8). The sequence of C. axonis sp. n. is a well-supported sister branch to the rest of the Cystodiscus spp. sequences. Relationships of C. australis sp. n., C. melleni, and 2 sequences of C. cf. immersus had moderate or low bootstrap support but their positions were stable in both analyses. The Cystodiscus spp. group clustered inside the freshwater gallbladder clade according to Fiala (Reference Fiala2006) with a close relationship to the representative of the marine genus Sphaeromyxa in ML (bootstrap <50%) and to the terrestrial Soricimyxum fegati (bootstrap <50%) in MP. The SSU rDNA alignment did not robustly resolve the phylogenetic position of the monophyletic Cystodiscus spp. clade within the myxosporean tree, as indicated by low bootstrap values (Fig. 8).

Fig. 8. Phylogenetic position of Cystodiscus spp. within the myxosporean freshwater clade. Maximum likelihood tree (−ln=14644.0851) with GTRGAMMA model of evolution. Numbers at the nodes represent bootstrap values (ML/MP) for the nodes gaining more than 50% support. GenBank Accession numbers are next to the species names. Scale bar is given under the tree. Schematic drawings illustrate the diversity of spore morphology of Myxidium and Cystodiscus spp.
DISCUSSION
The description of these 2 new myxosporean parasites was based on a large collection of native and exotic frog material from eastern Australia. The combination of histological, ultrastructural and genetic characterization has unequivocally discriminated 2 Australian frog species from each other and from described myxosporean taxa from other continents. Our investigation led to the re-examination of frog gallbladder Myxosporea and subsequent resurrection of the genus Cystodiscus Lutz, 1889.
The coupling of traditional myxospore morphological description with molecular analysis has aided the diagnostic methods to differentiate between these species as suggested by Fiala and Bartošová (Reference Fiala and Bartošová2010). In addition, the examination of in situ tissue development provided more information about the biology and development of these parasites. The developmental stages of C. axonis sp. n. within the axons of tadpoles are considered to be extrasporogonic, because no stages were observed to progress to sporogenesis. Extrasporogonic stages are suggested to exist for proliferation of the parasite only (Lom, Reference Lom1987; Lom and Dyková, Reference Lom and Dyková1992). For example, extrasporogonic stages in the blood are thought to amplify and spread infection of Sphaerospora dykovae (syn. S. renicola) in carp (Lom et al. Reference Lom, Pavlásková and Dyková1985). Multiple attempts to identify blood cell stages were unsuccessful in any of the animals infected with C. axonis sp. n. or C. australis sp. n. (A.H., unpublished data). Without blood cell stages, the presence of extrasporogonic plasmodia in myelinated axons raises at least two significant questions. It is yet unknown, if the stages form a tissue pool that continually contributes to the terminal bile duct development. It is yet to be discovered, if the swollen parasitized axons have decreased capacity for signal transmission and therefore an effect on motor function of frogs compared to unaffected frogs.
Significant disease has been observed in Myxosporea affecting CNS in fish, such as Myxobolus cerebralis, Myxobolus neurobius, and Myxobolus cottis. However, the development of these species is restricted entirely to CNS and associated cartilage and is dissimilar to the parasite development observed in frogs. The axonal stages are ultrastructurally very similar to parasite stages in the axons of the Bullhead Cottus gobio (Lom et al. Reference Lom, Feist, Dyková and Kepr1989), a lungfish Polypterus endlicheri (Marquet and Sobel, Reference Marquet and Sobel1970) and a toad Bufo arenarum (Stensaas et al. Reference Stensaas, Stensaas and Sotelo1967). The significance of the axonal development of Myxosporea remains unknown. Therefore, it is likely that axonal development is not restricted to C. axonis sp. n., and complete histopathology including CNS may reveal that axon tropism of myxosporeans is more common than previously recognized. It remains unknown, if the axonal stages described by Stensaas et al. (Reference Stensaas, Stensaas and Sotelo1967) in South American toads represent C. axonis sp. n. or a closely related new species.
Examination of host tissues and the developing stages of C. australis sp. n. and C. axonis sp. n. offered important information about the significance of infection to the host and revealed a significant species-specific diagnostic feature. These features would otherwise be missed if only molecular data and spore measurements were used for species identification. Spore ultrastructure revealed the presence of FPAs on the spores of C. axonis sp. n. and serves as the distinguishing feature between otherwise similar looking spores in Australian frogs. To our knowledge no other myxosporeans possess such fine appendages. Appendages such as those described for Tetrauronema desqualis or Henneguya adiposa are thicker and easily observed in light microscopy (Azevedo and Matos, Reference Azevedo and Matos1996; Griffin et al. Reference Griffin, Wise and Pote2009). While the FPA of C. axonis sp. n. were observed using light microscopy, they may often remain unnoticed. It is likely that Delvinquier (Reference Delvinquier1986) observed C. axonis sp. n. during his extensive frog parasite survey and SEM myxospore studies. Therefore, thorough morphological re-evaluation of parasitological specimens is imperative for accurate species identification and classification.
The taxonomy and systematics of Myxosporea is largely based upon the spore morphology and host species (Lom and Dyková, Reference Lom and Dyková2006). More recently, molecular characterization became a complementary part of species descriptions. Such combination of molecular phylogenetics and morphology is serving as the basis for improved systematics of Myxosporea (Fiala and Bartošová, Reference Fiala and Bartošová2010). One of the most diverse myxosporean genera is Myxidium Bütschli, 1882 which is now comprising numerous phylogenetically unrelated clades sharing similar spore morphology (Fiala, Reference Fiala2006; Lom and Dyková, Reference Lom and Dyková2006). Molecular phylogenetics based on SSU rDNA and LSU rDNA laid the foundation for several robust clades within Myxidium sensu lato that await taxonomic reclassification (Fiala, Reference Fiala2006; Fiala and Bartošová, Reference Fiala and Bartošová2010; Hartigan et al. Reference Hartigan, Fiala, Dyková, Jirků, Okimoto, Rose, Phalen and Šlapeta2011). Here, we re-classify one such clade embracing amphibian gallbladder species and resurrect the genus Cystodiscus Lutz, 1889 from the synonymy of Myxidium Bütschli, 1882.
Lutz (Reference Lutz1889) defined the genus Cystodiscus based on the large disc-like appearance of the gallbladder plasmodia “Für das Genus möchte ich zur Bezeichnung der regelmässigen Form und des zelligen Baus den Namen Cystodiscus und für die Species die Bezeichnung immersus vorschlagen” (=for the genus, I would like to propose a name Cystodicus referring to its regular form and the cellular construction, and for the species the name immersus) of the species Cystodiscus immersus Lutz, 1889 from Cane toads in Brazil. The genus name was later abandoned and fell into the synonymy of Myxidium Bütschli, 1882 (Kudo and Sprague, Reference Kudo and Sprague1940). The available molecular phylogenetic data clearly place Cystodiscus Lutz, 1889 as a distinct monophyletic lineage termed “Myxidium-frog clade” (Hartigan et al. Reference Hartigan, Fiala, Dyková, Jirků, Okimoto, Rose, Phalen and Šlapeta2011). Due to the polyphyletic nature of the genus Myxidium we propose to resurrect the genus with the following emendations and provide a synopsis for named species.
Cystodiscus Lutz,1889emend.
Type species: Cystodiscus immersus Lutz, 1889
Bivalved myxospores with 2 polar capsules at opposite poles and a central binucleate sporoplasm. Spores oval or ellipsoidal with raised sutural ridge between the valves. The sutural ridge straight or curved along the medial axis of the spore. One or more longitudinal ridges parallel to the sutural ridge. Transverse ridges across the valve surface absent or present. Disc-like plasmodia with spore development in gallbladders of members of Amphibia.
Synopsis of Cystodiscus Lutz,1889emend.
Cystodiscus immersus Lutz,1889
Syn. Cystodiscus immersus Lühe, 1899; Cystodiscus immersus Cordero, Reference Cordero1919; Myxidium immersum Kudo and Sprague, Reference Kudo and Sprague1940
Myxospore morphology: 12·0–14·0×9·0–10·0 μm with sutural ridge at 45° angle from lateral view, but no ridges are mentioned or illustrated (Lutz, Reference Lutz1889). Later, Cordero (Reference Cordero1919) noted the presence of transverse ridges. Spores are re-described as “broadly fusiform with bluntly rounded extremities in front view […] rounded rectangular in side view. The slightly curved sutural line of the shell-valves makes an acute angle with the longitudinal axis of the spore and extends to the diagonally opposite points at the extremities” (Kudo and Sprague, Reference Kudo and Sprague1940). Re-description: 11·8–13·3×7·5–8·6 μm, S-shaped suture, 1 longitudinal depression encircling the valve parallel to the sutural ridge, 7–9 transverse ridges (6–8 transverse depressions) situated toward the smaller end not reaching the larger end of the shell valve. Polar capsules broadly piriform 3·5–4·2 μm. No FPA. Spores in the gallbladder.
Type host: Bufo agua=Cane toad Rhinella marina Linnaeus, 1758 syn. Bufo marinus (Bufonidae, Anura) (Lutz, Reference Lutz1889). Also described in captive Bufo agua=Bufo marinus by Lühe (1899). Re-described from R. marina (Kudo and Sprague, Reference Kudo and Sprague1940).
Type locality: Brazil.
Type material: Material described by Lutz (Reference Lutz1889) and the re-description based on material that consisted of several sporulating trophozoites “from the gallbladder of a Bufo marinus from Brazil in August 1937” (Kudo and Sprague, Reference Kudo and Sprague1940). No material deposited.
Other hosts: Lutz (Reference Lutz1889) noted that the same parasite was found in Cystignathus ocellatus=Leptodactylus bolivianus Boulenger, 1898 (Leptodactylidae, Anura). The list of frog species infected with C. immersus by Cordero (Reference Cordero1919) is treated with caution because Hartigan et al. (Reference Hartigan, Fiala, Dyková, Jirků, Okimoto, Rose, Phalen and Šlapeta2011) demonstrated the presence of multiple distinct genotypes within one morphotype. The species was assumed to be translocated to Australia (Delvinquier, Reference Delvinquier1986), but now all Australian gallbladder Cystodiscus spp. are considered distinct from this species (Hartigan et al. Reference Hartigan, Fiala, Dyková, Jirků, Okimoto, Rose, Phalen and Šlapeta2011).
Remarks: Kudo and Sprague (Reference Kudo and Sprague1940) wrote about C. immersus that “when the spore is seen in front view, transverse ridges occupy about two-thirds of the shell valve and the remaining one-third is without any transverse ridges” and use it to distinguish it from C. serotinus. Kudo and Sprague (Reference Kudo and Sprague1940) mentioned that the numbers and arrangement were remarkably constant for spores they had observed. Genetic analysis of spores consistent with Cystodiscus cf. immersus from R. marina collected in Brazil revealed the presence of 2 distinct genotypes (Hartigan et al. Reference Hartigan, Fiala, Dyková, Jirků, Okimoto, Rose, Phalen and Šlapeta2011). These Cystodiscus cf. immersus spores were characterized ultrastructurally and therefore they remain unassigned until morphological analysis coupled with genetic characterization is conducted. Etymology not given.
Cystodiscus lyndoyense Carini,Reference Carini1932
Syn. Myxidium lyndoyense Kudo and Sprague, Reference Kudo and Sprague1940
Myxospore morphology: Broadly oval shaped and fusiform in sutural view, 11–12×7·5–8 μm, with rounded polar capsules 4 μm in diameter. The suture S-shaped with a sutural ridge and an additional longitudinal ridge running parallel present (2 longitudinal depressions). No transverse ridges/depressions noted (Carini, Reference Carini1932). Transverse ridges considered present in the revision by Kudo and Sprague (Reference Kudo and Sprague1940). No FPA. Spores in the gallbladder.
Type host: Cane toad Rhinella marina Linnaeus, 1758 syn. B. marinus (Bufonidae, Anura).
Type locality: Lindóia – São Paulo, Brazil.
Type material: Material described by Carini (Reference Carini1932). No material deposited.
Other hosts: Carini (Reference Carini1932) has recorded this species in at least 4 species of frogs in 3 families (Bufonidae, Hylidae, Leiuperidae).
Remarks: Kudo and Sprague (Reference Kudo and Sprague1940) and all future authors treat this name as a synonym of C. immersus Lutz, 1889. We treat this name cautiously as nomen dubium/species inquirendae until further data on the diversity of Cystodiscus spp. in South American frogs is available (see remarks for C. immersus Lutz, 1889). Species named after the type locality – Lindóia.
Cystodiscus serotinus (Kudo and Sprague,Reference Kudo and Sprague1940) comb. n.
Syn. Myxidium serotinum Kudo and Sprague, Reference Kudo and Sprague1940
Myxospore morphology: Ellipsoidal, broad ended, 16·0–18·0×9·0 μm, with 2–4 longitudinal ridges (3–5 longitudinal depressions) which are parallel to the sutural ridge. The suture curves (S-shape) before the end of the spore. The valve surface is covered with 10–13 transverse ridges (9–14 transverse depressions), some ridges are fused. The orientation of ridges and depressions was variable according to Kudo and Sprague (Reference Kudo and Sprague1940); some spores were seen with branching transverse ridges and depressions, some with both transverse and longitudinal patterns. The nearly spheroidal polar capsules measure 5·0–5·5 μm with 3–5 polar filament coils. No FPA. Spores in the gallbladder.
Type host: Northern Leopard Frog Rana pipiens Schreber, 1782 (Ranidae, Anura).
Type locality: First seen in a frog in a “class room”, at The University of Illinois, USA; an additional survey by Kudo and Sprague (Reference Kudo and Sprague1940) revealed that “11·3 per cent of a group of 150 Rana pipiens which were collected from Wisconsin, Iowa, Minnessota, etc.”, were infected with the Myxosporea.
Type material: Material described by Kudo and Sprague (Reference Kudo and Sprague1940). No material deposited.
Other hosts: Rana sp. “15·4% of 26 undetermined species of Rana of Louisiana” (Kudo and Sprague, Reference Kudo and Sprague1940). In the later study by Kudo (Reference Kudo1943) the parasite was identified in 25/41 (60·9%) of the Southern toad Bufo terrestris=Anaxyrus terrestris Bonnaterre, 1789, 6/21 (28·6%) of Rana clamitans=Lithobates clamitans Latreille, 1801 and 33/88 (37·5%) of Rana sphenocephala=Lithobates sphenocephalus Cope, 1886. The species was considered identical to the one found in the gallbladder of the Two lined salamander Eurycea bislineata Green, 1818 (Plethodontidae, Caudata) from Cabell and Wayne countries, West Virginia, USA in high (87·9%, 51/58, spring of 1969) prevalence (Clark and Shoemaker, Reference Clark and Shoemaker1973). While the illustration (Clark and Shoemaker, Reference Clark and Shoemaker1973) and SEM (Clark, Reference Clark1982) of the spores of salamanders appear to show spores to be identical to those seen by Kudo and Sprague (Reference Kudo and Sprague1940), further work using molecular typing is deemed necessary to confirm identify of these parasites in frogs and salamanders. In addition, a large number of North American frogs and salamanders have tentatively been considered to be infected by this species (McAllister and Trauth, Reference McAllister and Trauth1995; McAllister and Bursey, Reference McAllister and Bursey2005; McAllister et al. Reference McAllister, Bursey and Trauth2008, Reference McAllister, Crawford and Kuhns2009).
Remarks: Kudo and Sprague (Reference Kudo and Sprague1940) distinguished this species by its size and the large number of ridges and depressions (covering entire spore surface) from the only other known myxosporean species they recognized valid in frog gallbladders of the time, C. immersus. Etymology not given.
Cystodiscus haldari (Sarkar,Reference Sarkar1982) comb. n.
Syn. Myxidium haldari Sarkar, Reference Sarkar1982
Myxospore morphology: ‘Fusiform or cylindriobiconical in sutural view’, spindle to oval shaped, 10·8 (10·0–12·0)×6·7 (6·5–7·0) μm with 8–10 longitudinal (end to end) ridges (7–11 longitudinal depressions) on the surface without transverse ridges. Polar capsules round, 3·6 (3·0–4·0) μm. No FPA. Spores in the gallbladder.
Type host: European tree frog Hyla arborea Linnaeus, 1758 (Hylidae, Anura), 20% (1/5) frogs (Sarkar, Reference Sarkar1982). As this frog is absent in India, we speculate that it was Jerdon's Tree Frog Hyla annectans Jerdon, 1870, because it is the only representative of the genus Hyla in the Hyla arborea group in India (Faivovich et al. Reference Faivovich, Haddad, Garcia, Frost, Campbell and Wheeler2005).
Type locality: Chakdaha, West Bengal, India.
Type material: Specimens on slides (H–M 6) stained with Giemsa described by Sarkar (Reference Sarkar1982) as “to be deposited soon” in the National Collection of the Zoological Survey of India, Calcutta (http://www.envfor.nic.in/zsi/).
Remarks: This species is distinct from all other Cystodiscus spp. by the presence of 8–10 longitudinal ridges (9–11 longitudinal depressions) and no transverse ridges (Sarkar, Reference Sarkar1982). Named after Dr D. P. Haldar.
Cystodiscus lesminteri (Delvinquier, Markus and Passmore,Reference Delvinquier, Markus and Passmore1992) comb. n.
Syn.Myxidium lesminteri Delvinquier, Markus and Passmore,Reference Delvinquier, Markus and Passmore1992
Myxospore morphology: Ovoid, 12·5 (9·5–15·0)×6·5 (5·7–8·0) μm with 1 longitudinal depression encircling the valve parallel to the sutural ridge. Additional longitudinal depressions (1–2 seen in SEM) occupying two-thirds of spore valve, no transverse depressions. Polar capsule measurements not given. No FPAs. Spores in the gallbladder.
Type host: Knocking Sand Frog, Tomopterna krugerensis Passmore and Carruthers, 1975 (Ranidae, Anura), 20% (1/5) of adult frogs collected in February 1989.
Type locality: Naboomspruit District,Transvaal, Mosdene Nature Reserve, South Africa.
Type material: SEM stub with spores and record image deposited in the Queensland Museum, PO Box 3300, South Brisbane BC, Queensland 4101, Australia under collection number GL13041 (http://www.qm.qld.gov.au/Collections/Collection+Online) (Delvinquier et al. Reference Delvinquier, Markus and Passmore1992).
Other hosts: Eastern Olive Toad Amietophrynus garmani=Bufo garmani Meek, 1897 (Bufonidae, Anura); Natal Ghost Frog Heleophryne natalensis Hewitt, 1913 (Heleophrynidae, Anura); Common Sand Frog Tomopterna cryptotis Boulenger, 1907 (McAllister and Freed, Reference McAllister and Freed1996).
Remarks: The only Cystodiscus species described from Africa. Delvinquier et al. (Reference Delvinquier, Markus and Passmore1992) distinguished this species from all known gallbladder spores in frogs by the absence of transverse spore surface ridges. The shape and low number of longitudinal ridges distinguishes this species from Cystodiscus haldari Sarkar, Reference Sarkar1982 which is devoid of transverse ridges. Named after Mr Les Minter.
Cystodiscus typhonius (Gray,Reference Gray1993) comb. n.
Syn. Myxidium typhonius Gray, Reference Gray1993
Myxospore morphology: Ellipsoidal, 10·9 (9·8–12·2)×7·2 (5·7–8·9) μm with a single straight sutural ridge extending from pole to pole, accompanied with a sutural depression. The valve surface with 9–11 non-branching transverse ridges (8–10 transverse depressions) parallel to each other. Polar capsules 3·8 (2·5–5·5)×3·6 (3·3–5·2) μm with 4–5 polar filament coils. No FPA. Spores in the gallbladder.
Type host: Bufo typhonius=Trachycephalus typhonius Linnaeus, 1758, 88% (30/34) of adult frogs collected in December 1989 – January 1990.
Type locality: Puerto Maldonaro, Peru, South America.
Type material: Formalin preserved plasmodia containing spores deposited into the United States National Museum, 10th St. and Constitution Ave. NW, Washington, DC 20560, Helminth collection number 81272.
Other hosts: A survey of Peruvian amphibians in December 1989 – January 1990 showed: Rhinella marina Linnaeus, 1758, 72·7% (8/11) (Bufonidae, Anura); Dull Rocket Frog, Colostethus marchesians=Allobates marchesianus Melin, 1941 90·9% (10/11) (Aromobatidae, Anura); Crump Treefrog, Hyla brevifrons=Dendropsophus brevifrons Duellman and Crump, 1974 100% (12/12) (Hylidae, Anura), Koechlin's Treefrog Hyla koechlini=Dendropsophus koechlini Duellman and Trueb, 1989, 92·8% (26/28) (Hylidae, Anura); White leaf frog Hyla leucophyllata group=Dendropsophus leucophyllatus Beireis, 1783 71·4% (10/14) (Hylidae, Anura); Dendropsophus leali Bokermann, 1964 93·8% (15/16) (Hylidae, Anura); Wagner's White-lipped Frog, Leptodactylus wagneri Peters, 1862 100% (3/3) (Leptodactylidae); Pointedbelly Frog, Leptodactylus podicipinus Cope, 1862 100% (1/1) (Leptodactylidae, Anura); Basin White-lipped Frog, Leptodactylus mystaceus Spix, 1824 85·7% (6/7) (Leptodactylidae, Anura); Leptodactylus bolivianus Boulenger, 1898 75·0% (9/12) (Leptodactylidae, Anura); Perez's Snouted Frog, Edalorhina perezi Jiménez de la Espada, 1870 50·0% (3/6) (Leiuperidae, Anura); Bolivian Bleating Frog, Hamptophryne boliviana Parker, 1927 92·0% (23/25) (Microhylidae, Anura) (Gray, Reference Gray1993).
Remarks: The size of C. typhonius spore is similar to the spores of C. immersus, both species are described from sympatric toads in South America. Compared to C. typhonius (entire spore is covered with depressions and ridges), the spore of C. immersus has ridges only in 2/3 of the spores. While C. typhonius is not recorded in North America, it shares similar spore morphology with C. serotinus. The spore of C. serotinus is larger. The spore suture of C. serotinus is S-curved compared to C. typhonius straight suture and the ridges on the valve of C. typhonius are transverse and parallel, compared to C. serotinus which is noted to have branched ridges and varied ridge orientation. Named after the type host.
Cystodiscus melleni (Jirků, Bolek, Whipps, Janovy, Kent and ModrýReference Jirků, Bolek, Whipps, Janovy, Kent and Modrý2006) comb. n.
Syn. Myxidium melleni Jirků, Bolek, Whipps, Janovy, Kent and ModrýReference Jirků, Bolek, Whipps, Janovy, Kent and Modrý2006
Myxospore morphology: “Mature spores, broadly ellipsoidal with slightly flattened ends in valvular view […] elongate and rhomboidal with rounded edges in sutural view. Sutural ridge turns at angle of approximately 90° near each pole” (suture Z-shaped) (Jirků et al. Reference Jirků, Bolek, Whipps, Janovy, Kent and Modrý2006), 12·3 (12·0–13·5)×7·6 (7·0–9·0) μm with 1 longitudinal depression encircling the valve parallel to the sutural ridge, 2–5 transverse ridges (1–4 transverse depressions) situated centrally. Polar capsules 5·2 (4·8–5·5)×4·2 (3·8–4·5) μm with 6–7 polar filament coils. No FPAs. Spores in the gallbladder.
Type host: Western Chorus Frog Pseudacris triseriata triseriata Wied-Neuwied, 1838 (Hylidae, Anura), 65% (20/31) frogs during March 2004.
Type locality: Pawnee Lake near Lincoln, Lancaster County, Nebraska, USA (40° 51.18′ N, 96° 53.11′ W).
Type material: Myxospores, SEM stub and slides deposited in the Harold W. Manter Laboratory of Parasitology collection, University of Nebraska State Museum (http://manter.unl.edu/hwml/) under collection numbers 48167–48171 and phototypes as collection number 48172; SSU rDNA in GenBank: DQ003031.
Other hosts: Recorded from 1/9 (11%) Blanchard's cricket frog, Acris crepitans blanchardi Conant, 1958 (Hylidae, Anura) and 1/6 (16·7%) Upland chorus frog Pseudacris feriarum feriarum Hedges, 1986 (Hylidae, Anura) (Jirků et al. Reference Jirků, Bolek, Whipps, Janovy, Kent and Modrý2006; McAllister et al. Reference McAllister, Bursey and Trauth2008).
Remarks: This species is distinct from all Cystodiscus spp. based on its size, centrally positioned transverse ridges and depressions and sutural ridge shape. In particular the sympatric but larger C. serotinus whose spore is entirely covered with transverse ridges. See C. serotinus and C. immersus remarks regarding genetic identification.
Cystodiscus axonis sp. n.
Syn. Myxidium immersum Delvinquier, Reference Delvinquier1986pro parte; Myxidium immersum Hartigan et al. (Reference Hartigan, Fiala, Dyková, Jirků, Okimoto, Rose, Phalen and Šlapeta2011) pro parte
Myxospore morphology: Ellipsoid to ovoid, straight or S-shaped suture, 14·5 (13·0–15·0)×8·7 (8·0–10·0) μm with 4–8 centrally located transverse ridges (5–9 depressions). FPA present.
Type host: Southern bell frog Litoria raniformis Keferstein, 1867 (Hylidae, Anura).
Type locality: Coleambally, New South Wales, Australia (−34°47′60″, 145°46′00″).
Type material: G.17758. Australian Museum, Sydney, New South Wales, Australia.
Other hosts: Green and golden bell frog Litoria aurea Lesson, 1826 (Hylidae, Anura); Striped marsh frog Limnodynastes peronii Duméril and Bibron, 1841 (Limnodynastidae, Anura); Peron's tree frog Litoria peronii Tschudi, 1838 (Hylidae, Anura); Cane toad Rhinella marina Linneaus, 1758 (Bufonidae, Anura).
Cystodiscus australis sp. n.
Syn. Myxidium immersum Delvinquier, Reference Delvinquier1986pro parte; Myxidium immersum Hartigan et al. (Reference Hartigan, Fiala, Dyková, Jirků, Okimoto, Rose, Phalen and Šlapeta2011) pro parte
Myxospore morphology: Ellipsoid to ovoid, straight or S-shaped suture, 16·0 (15·0–18·0)×8·7 (8·0–10·0) μm with 5–11 transverse ridges (5–12 depressions). No FPA.
Type host: Striped marsh frog Limnodynastes peronii Duméril and Bibron, 1841 (Limnodynastidae, Anura).
Type locality: Riverstone, New South Wales, Australia (−33°41′7″, 150°50′53″).
Type material: G.17756. Australian Museum, Sydney, New South Wales, Australia.
Other hosts: Green and golden bell frog Litoria aurea Lesson, 1826 (Hylidae, Anura); Striped marsh frog Limnodynastes peronii Duméril and Bibron, 1841 (Limnodynastidae, Anura); Cane toad Rhinella marina Linneaus, 1758 (Bufonidae, Anura).
ACKNOWLEDGEMENTS
We thank Tomáš Scholz and Astrid Holzer (Institute of Parasitology, Biology Centre of the ASCR, Czech Republic) for their support. We acknowledge the Australian Key Centre for Microscopy and Microanalysis at The University of Sydney and the Electron Microscopy unit at the Institute of Parasitology, Biology Centre of the ASCR, Czech Republic. A.H. was the recipient of the J.D. Smyth Travel Award from the Australian Society for Parasitology and the ARC/NHMRC Research Network for Parasitology. We thank Elaine Chew for expert histopathology processing, Glenn Shea, Lance Jurd, David Newell for their insight and two anonymous reviewers for constructive suggestions. This study was supported by the Australian Academy of Science, Dr William Richards Award in Veterinary Pathology and in part by the Grant Agency of the Academy of Sciences of the Czech Republic (KJB600960701) and the Grant Agency of the Czech Republic (204/09/P519). The Australian Registry of Wildlife Health acknowledges the Australian Biosecurity Intelligence Network (ABIN) for information management and communication systems that contributed to this research. ABIN is a project of the National Collaborative Research Infrastructure Strategy (NCRIS).