Introduction
Fibricola Dubois, 1932 (Diplostomidae Poirier, 1886) is a small genus of diplostomid digeneans distributed in North and South America, Africa, Asia and Australia (Barker, Reference Barker1915; Bisseru, Reference Bisseru1957; Seo et al., Reference Seo, Rim and Lee1964; Kifune and Uyema, Reference Kifune and Uyema1982; Cribb and Pearson, Reference Cribb and Pearson1993; Niewiadomska, Reference Niewiadomska, Gibson, Jones and Bray2002; Lima et al., Reference Lima, Hoppe, Tebaldi, Cruz, Barros Gomes and Nascimento2013). Members of Fibricola are often reported in ecological and parasite survey studies, most commonly from their frog second intermediate hosts (e.g. Ulmer, Reference Ulmer, MacInnis and Voge1970; Premvati and Bair, Reference Premvati and Bair1979; Gillilland and Muzzall, Reference Gillilland and Muzzall1999; Goldberg and Bursey, Reference Goldberg and Bursey2001; Goldberg et al., Reference Goldberg, Bursey and Gergus2001; Bolek and Coggins, Reference Bolek and Coggins2003; Richardson, Reference Richardson2013; Weinstein et al., Reference Weinstein, Van Wert, Kinsella, Tkach and Laffery2019). Although most members of Fibricola are known to parasitize intestines of mammals, some Fibricola species have also been reported from crocodilians (Bisseru, Reference Bisseru1957; Dubois, Reference Dubois1982).
In contrast to Fibricola spp., the currently accepted members of the morphologically similar Neodiplostomum Railliet, 1919 parasitize intestines of birds with few exceptions. The majority of Neodiplostomum spp. known from mammals were collected in the Old World and originally placed into Fibricola based on their parasitism in mammals (e.g. Neodiplostomum seoulensis (Seo, Rim and Lee, Reference Seo, Rim and Lee1964) and Neodiplostomum minor (Dubois, 1936)), and later transferred to Neodiplostomum (Seo et al., Reference Seo, Rim and Lee1964; Cribb and Pearson, Reference Cribb and Pearson1993; Hong and Shoop, Reference Hong and Shoop1994). Notably, Neodiplostomum vaucheri Dubois, 1983, described from the frog-eating big-eared woolly bat Chrotopterus auritus Peters in Peru, was the only member of Neodiplostomum from mammals originally assigned into the genus (Dubois, Reference Dubois1983). Noteworthily, N. seoulensis has been reported from humans in Korea (Huh et al., Reference Huh, Lee and Huh1994). Despite the general trends of parasitism in different groups of definitive hosts (birds vs mammals), adult Neodiplostomum spp. and Fibricola spp. are remarkably morphologically similar. In the most recent detailed taxonomic revision of the group, Niewiadomska (Reference Niewiadomska, Gibson, Jones and Bray2002) admitted that the two genera lack consistent morphological differences that can be used to reliably distinguish one from another. Although Niewiadomska (Reference Niewiadomska, Gibson, Jones and Bray2002) retained the traditional generic and subfamily status of Neodiplostomum and Fibricola, she emphasized that the resolution of the real relationship between these genera needs to be supported by both morphological and molecular evidence.
Currently, DNA sequences are available for six species of Neodiplostomum (Woodyard et al., Reference Woodyard, Rosser and Griffin2017; Heneberg et al., Reference Heneberg, Sitko and Těšínský2020; Lee et al., unpublished results), but DNA sequence data from morphologically identified adult Fibricola specimens are lacking. Herein, we provide partial sequences of the nuclear small ribosomal subunit (18S), internal transcribed spacer region (ITS1, 5.8S, ITS2) and large ribosomal subunit (28S) rRNA genes as well as a fragment of the mitochondrial cytochrome c oxidase subunit 1 (cox1) mtDNA gene for Fibricola cratera (Barker and Noll, 1915) and Fibricola lucidum (La Rue and Bosma, 1927) from mammals as well as five nominal species of Neodiplostomum and an unidentified Neodiplostomum species from a bird. We use newly generated and previously available DNA sequences to examine the phylogenetic interrelationships of Fibricola and Neodiplostomum species and re-evaluate their systematics.
Materials and methods
Adult specimens belonging to genera Fibricola and Neodiplostomum were collected from a variety of mammalian and avian definitive hosts as well as amphibian intermediate hosts in North and South America (Table 1). Live digeneans removed from hosts were briefly rinsed in saline, killed with hot water and preserved in 80% ethanol. Dead digeneans were immediately preserved in 80% ethanol. Specimens for light microscopy were stained with aqueous alum carmine and mounted permanently according to Lutz et al. (Reference Lutz, Tkach, Weckstein and Webster2017). Specimens were measured using an Olympus® BX53 microscope (Olympus America, Center Valley, Pennsylvania, USA) equipped with a digital imaging system. Voucher specimens are deposited in the collection of the Harold W. Manter Laboratory (HWML), University of Nebraska State Museum, Lincoln, NE, USA and the Museo de Zoología, Pontificia Universidad Católica del Ecuador (QCAZI), Quito, Ecuador. Due to the inconsistent reporting of morphological characteristics in the descriptions of Neodiplostomum spp., we re-measured type and voucher specimens of Neodiplostomum americanum Chandler and Rausch, 1947, Neodiplostomum banghami Penrod, 1947 and Neodiplostomum reflexum Chandler and Rausch, 1947 (syn. Neodiplostomum delicatum Chandler and Rausch, 1947) for comparison with specimens collected in the current study. Type and voucher specimens were borrowed for our study from the Natural History Museum of Geneva and the Smithsonian Institution Museum of Natural History. We use the terms prosoma and opisthosoma as explained by Achatz et al. (Reference Achatz, Curran, Patitucci, Fecchio and Tkach2019a) and Tkach et al. (Reference Tkach, Achatz, Pulis, Junker, Snyder, Bell, Halajan and Melo2020).
Table 1. Hosts, geographic origin, GenBank and museum accession numbers of Neodiplostomum (syn. Fibricola) spp. used in this study

HWML, Harold W. Manter Laboratory; QCAZI, Museo de Zoología, Pontificia Universidad Católica del Ecuador.
*Species previously considered to be within Fibricola.
For comparative purposes, specimens of the following species have been examined from the collection of the Natural History Museum, London (NHM): Neodiplostomum australiense (Dubois, 1937) from Australia (co-types, NHM 1950.12.6.18–22), Neodiplostomum ramachandrani (Betterton, 1976) from Malaysia (paratypes: NHM 1979.8-3.36, 44–46; NHM 1976.4.21.74; vouchers: NHM 1976.8.4.7–8) and Neodiplostomum spathula (Creplin, 1829) from Minnesota (vouchers: NHM 1975.1.7.35-42).
Genomic DNA was extracted from either fragments (in case of larger specimens) or whole individuals of each species according to the methods described by Tkach and Pawlowski (Reference Tkach and Pawlowski1999). An approximately 1800 bp long fragment at the 5′ end of the 18S rRNA gene and a 1300 bp long fragment at the 5′ end of the 28S rRNA gene were amplified by polymerase chain reactions (PCRs) in a T100™ thermal cycler (Bio-Rad, Hercules, CA, USA). The 18S fragment was amplified using the forward primer WormA (5′–GCG AAT GGC TCA TTA AAT CAG–3′) and the reverse primer WormB (5′–ACG GAA ACC TTG TTA CGA CT–3′), whereas 28S was amplified using the forward primer digL2 (5′–AAG CAT ATC ACT AAG CGG–3′) and the reverse primer 1500R (5′–GCT ATC CTG AGG GAA ACT TCG–3′) (Littlewood and Olson, Reference Littlewood, Olson, Littlewood and Bray2001; Tkach et al., Reference Tkach, Littlewood, Olson, Kinsella and Swiderski2003). In addition, fragments of the ribosomal internal transcribed spacer region (ITS1 + 5.8S + ITS2) were amplified for some of the studied taxa using the forward primer ITSf (5′–CGC CCG TCG CTA CTA CCG ATT G–3′) and the reverse primer 300R (5′–CAA CTT TCC CTC ACG GTA CTT G–3′) (Littlewood and Olson, Reference Littlewood, Olson, Littlewood and Bray2001; Snyder and Tkach, Reference Snyder and Tkach2007). A fragment of the mitochondrial cox1 gene was amplified using the previously published forward primer Dipl_Cox_5′ (5′-ACK TTR GAW CAT AAG CG-3′) and the reverse primer Dipl_Cox_3′ (5′-WAR TGC ATN GGA AAA AAA CA–3′) (Achatz et al., Reference Achatz, Brito, Fecchio and Tkach2021b). For a subset of taxa collected in Mississippi and Arkansas (USA), the molecular methods described by Woodyard et al. (Reference Woodyard, Rosser and Griffin2017) were used.
An ExoSAP-IT PCR clean-up enzymatic kit from Affymetrix (Santa Clara, California, USA) was used to clean-up the PCR products following the manufacturer's protocol. PCR products were cycle-sequenced directly using BrightDye® Terminator Cycle Sequencing Kit chemistry (MCLAB, San Francisco, California, USA), alcohol precipitated and run on an ABI 3130 automated capillary sequencer (Life Technologies, Grand Island, New York, USA). PCR primers were used for sequencing of 18S, 28S and cox1 genes as well as the ribosomal ITS region. In addition, internal forward primer 18S-8 (5′-GCA GCC GCG GTA ATT CCA GC-3′) and internal reverse primer WB1 (5′-CTT GTT ACG ACT TTT ACT TCC-3′) were used for sequencing of the 18S fragment; internal forward primer DPL600F (5′-CGG AGT GGT CAC CAC GAC CG-3′) and internal reverse primer DPL700R (5′-CAG CTG ATT ACA CCC AAA G-3′) were used for sequencing of 28S PCR reactions; internal forward primer d58F (5′-GCG GTG GAT CAC TCG GCT CGT G-3′ was used to sequence the ITS region (Littlewood and Olson, Reference Littlewood, Olson, Littlewood and Bray2001; Kudlai et al., Reference Kudlai, Stunžėnas and Tkach2015; Achatz et al., Reference Achatz, Pulis, Junker, Tran, Snyder and Tkach2019d). Contiguous sequences were assembled using Sequencher version 4.2 software (GeneCodes Corp., Ann Arbor, Michigan, USA). Our newly generated sequences are deposited in GenBank (Table 1).
Phylogenetic interrelationships among the members of Fibricola and Neodiplostomum and other members of the Diplostomoidea Poirier, 1886 were analysed using the 18S, 28S and cox1 sequence data, in part, to match the data published by Heneberg et al. (Reference Heneberg, Sitko and Těšínský2020). Interrelationships among the members of the genus-level clades of Fibricola/Neodiplostomum were studied using two cox1 datasets based on the presence of two distinct clades of Neodiplostomum as observed from the results of our analyses of 18S and 28S as well as the suprageneric analysis of cox1 data. Sequences were aligned with the assistance of ClustalW as implemented in MEGA7 (Kumar et al., Reference Kumar, Stecher and Tamura2016); alignments were trimmed to the length of the shortest respective sequence. Cyathocotyle prussica Mühling, 1896 was used as the outgroup for the analysis of 18S and Strigea strigis (Schrank, 1788) was used as the outgroup for the suprageneric analysis of cox1 to be comparable with the analysis of Heneberg et al. (Reference Heneberg, Sitko and Těšínský2020). Suchocyathocotyle crocodili (Yamaguti, 1954) was selected as the outgroup for the 28S analysis based on the topology presented by Achatz et al. (Reference Achatz, Pulis, Junker, Tran, Snyder and Tkach2019d). On the basis of the results of the broader phylogenetic analyses (see ‘Results’ section), we opted to not use an outgroup in the phylogenetic analyses of interrelationships within the clade uniting Fibricola spp. with the majority of Neodiplostomum spp. clade based on cox1 data, because of the high level of genetic divergence between the members of this clade and other diplostomoidean taxa.
The 18S alignment included newly generated sequences of Fibricola spp. (n = 3) and Neodiplostomum spp. (n = 5) as well as previously published sequences of Neodiplostomum spp. (n = 3) and other members of the Diplostomidae (n = 21). The 28S alignment included newly generated sequences of Fibricola (n = 3) and Neodiplostomum spp. (n = 5) along with a previously published sequence of N. americanum. The 28S analysis also included previously published sequences of members of the Diplostomidae (n = 17), the Proterodiplostomidae Dubois, 1936 (n = 2) and Strigeidae Railliet, 1919 (n = 12). The suprageneric cox1 alignment included new sequences of Fibricola (n = 2) and Neodiplostomum spp. (n = 6) as well as previously published sequences of Neodiplostomum spp. (n = 7). This alignment also included previously published sequences of other members of the Diplostomidae (n = 15). The cox1 alignment limited to the members of the Fibricola/Neodiplostomum clade (clade I) included 21 newly generated sequences. The second cox1 alignment limited to the second clade of Neodiplostomum species (clade II) included nine newly generated sequences and nine previously published sequences.
Independent phylogenetic analyses were conducted using Bayesian inference (BI) as implemented in MrBayes Ver. 3.2.6 software (Ronquist and Huelsenbeck, Reference Ronquist and Huelsenbeck2003). The general time-reversible model with estimates of invariant sites and gamma distributed among site variation (GTR + I + G) was identified as the best-fitting nucleotide substitution model for the datasets using MEGA7 (Kumar et al., Reference Kumar, Stecher and Tamura2016). BI analyses of 18S, 28S and cox1 of the Diplostomidae were carried out with the following settings: Markov chain Monte Carlo chains run for 3 000 000 generations with a sample frequency of 1000, log-likelihood scores were plotted and only the final 75% of trees were used to produce the consensus trees. The cox1 analyses of clades I and II were carried out with identical settings, but sequence data were analysed as codons. The number of generations was considered sufficient when the s.d. value reduced well below 0.01. Due to limited representation, the ITS region sequences were not used for phylogenetic inference; however, we provided a pairwise sequence comparison for all isolates that have a complete ITS1 + 5.8S + ITS2 fragment sequenced.
Results
Molecular phylogenies
To maintain continuity and consistency in presenting and discussing our results, we are stating herein that we consider Fibricola to be a junior synonym of Neodiplostomum (see results of 18S, 28S and suprageneric cox1 analyses and discussion below). We refer to F. cratera and F. lucidum as Neodiplostomum cratera n. comb. (Barker and Noll, 1915) and Neodiplostomum lucidum La Rue and Bosma, 1927, respectively, throughout the remainder of the text. Justification for the synonymization is provided in the discussion.
The 18S alignment was 1619 bp long; 22 bases were excluded from the analysis due to ambiguous homology. The phylogenetic tree resulting from the BI analysis of 18S (Fig. 1) demonstrated a similar topology to that presented by Heneberg et al. (Reference Heneberg, Sitko and Těšínský2020). Neodiplostomum spp. were positioned in two distinct clades within a larger polytomy of diplostomids. Clade I (100% supported) of Neodiplostomum spp. contained Neodiplostomum cf. cratera 3 (Barker and Noll, 1915) (former type-species of Fibricola; see discussion below), Neodiplostomum cf. lucidum La Rue and Bosma, 1927, Neodiplostomum spathula (Creplin, 1829) (former type-species of Conodiplostomum Dubois, 1937) + Neodiplostomum attenuatum (Linstow, 1906) + Neodiplostomum microcotyle Dubois, 1937 + N. reflexum + N. vaucheri + Neodiplostomum sp. VVT1. Clade II (100% supported) of Neodiplostomum spp. only contained N. americanum + N. banghami.

Fig. 1. Phylogenetic interrelationships among the Diplostomidae including Neodiplostomum (syn. Fibricola) based on BI analysis of partial 18S rRNA gene sequences. Members of Neodiplostomum are indicated by the shaded rectangles. BI posterior probability values lower than 80% are not shown. The new sequences are indicated in bold. The scale bar indicates the number of substitutions per site. GenBank accession numbers are provided after the names of species.
The 28S alignment was 1135 bp long; three bases were excluded from the analysis due to ambiguous homology. The phylogenetic tree resulting from the BI analysis of 28S demonstrated the non-monophyly of the Diplostomidae and Strigeidae and monophyly of the Proterodiplostomidae (Fig. 2), similar to previous molecular phylogenetic analyses of the Diplostomoidea (e.g. Blasco-Costa and Locke, Reference Blasco-Costa and Locke2017; Hernández-Mena et al., Reference Hernández-Mena, García-Varela and Pérez-Ponce de León2017; Achatz et al., Reference Achatz, Dmytrieva, Kuzmin and Tkach2019b, Reference Achatz, Pulis, Fecchio, Schlosser and Tkach2019c, Reference Achatz, Martens, Kostadinova, Pulis, Orlofske, Bell, Fecchio, Oyarzún-Ruiz, Syrota and Tkach2022, Reference Achatz, Pulis, González-Acuña and Tkach2020, Reference Achatz, Bell, Melo, Fecchio and Tkach2021a; Queiroz et al., Reference Queiroz, López-Hernández, Locke, Pinto and Anjos2020; Tkach et al., Reference Tkach, Achatz, Pulis, Junker, Snyder, Bell, Halajan and Melo2020; Locke et al., Reference Locke, Drago, López-Hernández, Chibwana, Núñez, Van Dam, Achinelly, Johnson, Assis, Melo and Pinto2021). All sequences of taxa/lineages representing Fibricola formed a 99% supported clade (clade I) with four Neodiplostomum species: N. microcotyle, N. reflexum and N. vaucheri as well as unidentified species-level lineage Neodiplostomum sp. VVT1. This clade was separated into two strongly supported sub-clades. The first sub-clade (97%) included sequences of Fibricola from mammals + N. reflexum from birds. The second sub-clade (96%) contained Neodiplostomum sp. VVT1 from great horned owl Bubo virginianus (Gmelin) + a weakly supported assemblage of [N. microcotyle + N. vaucheri]. Clade II of Neodiplostomum spp. (100% supported) contained N. americanum + N. banghami (Fig. 2).
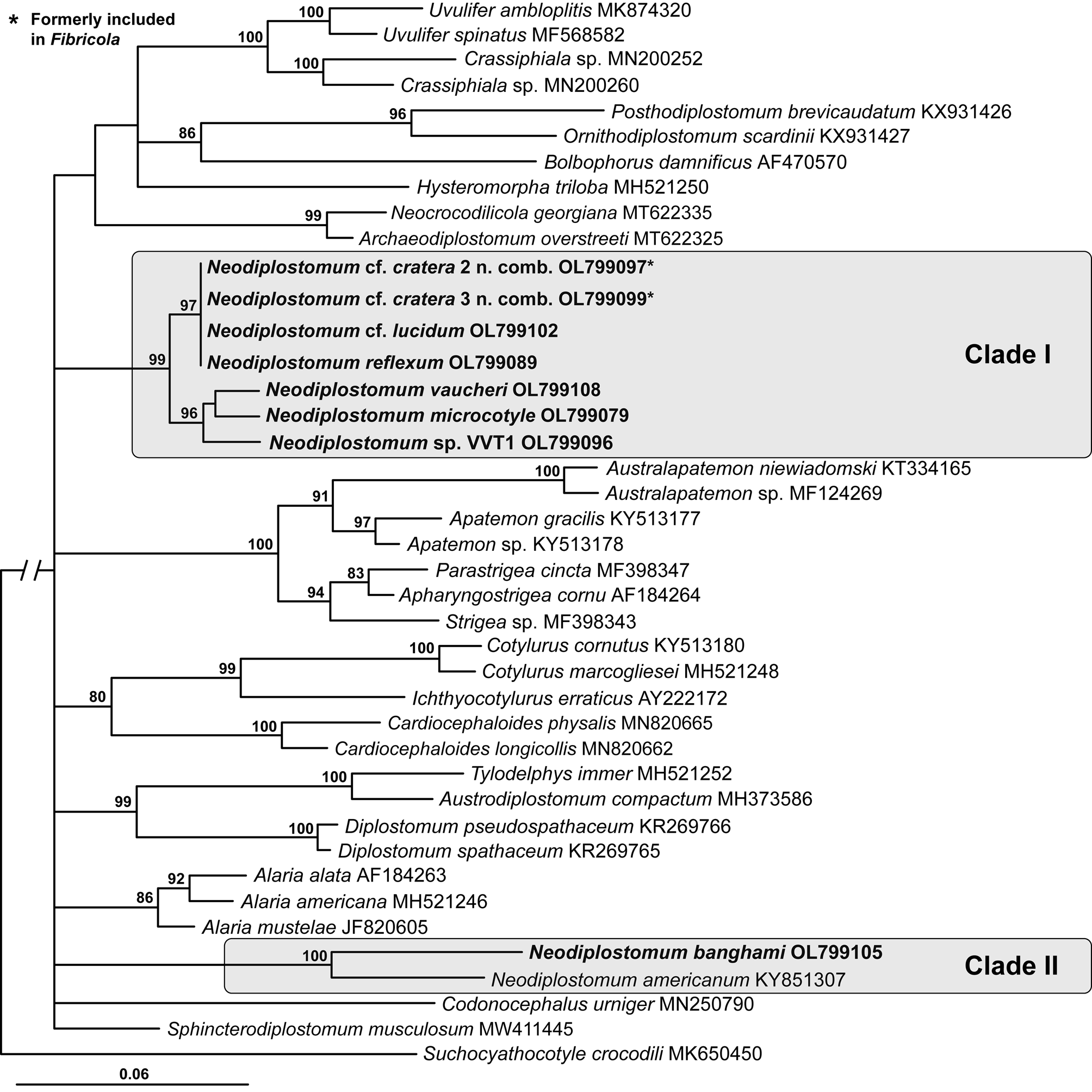
Fig. 2. Phylogenetic interrelationships among the diplostomoidean taxa including Neodiplostomum (syn. Fibricola) based on BI analysis of partial 28S rRNA gene sequences. Members of Neodiplostomum are indicated by the shaded rectangles. BI posterior probability values lower than 80% are not shown. The new sequences generated are indicated in bold. The scale bar indicates the number of substitutions per site. GenBank accession numbers are provided after the names of species.
The suprageneric cox1 alignment was 285 bp long; the alignment length was limited by the short length of sequences published by Heneberg et al. (Reference Heneberg, Sitko and Těšínský2020). Similar to the 18S and 28S analyses, Neodiplostomum taxa were split among the two clades (Fig. 3). Clade I (86% supported) consisted of a large polytomy with a poorly resolved internal topology (Fig. 3). The polytomy consisted of Neodiplostomum spathulaeforme (Brandes, 1888) (type-species of Neodiplostomum) + Neodiplostomum seoulense + a 100% supported clade of [N. reflexum + N. cf. cratera 3 + N. cf. lucidum] + an 88% supported clade of [N. vaucheri + N. microcotyle + Neodiplostomum sp. VVT1] + an 86% supported clade of [N. attenuatum + N. spathula] (Fig. 3).

Fig. 3. Phylogenetic interrelationships among the Diplostomidae including 16 members of Neodiplostomum (syn. Fibricola) based on BI analysis of partial cox1 mtDNA gene sequences. Members of Neodiplostomum are indicated by the shaded rectangles. BI posterior probability values lower than 80% are not shown. The new sequences generated are indicated in bold. The scale bar indicates the number of substitutions per site. GenBank accession numbers are provided after the names of species. The orders of definitive hosts are provided after GenBank accession numbers for Neodiplostomum spp. in clade I. Abbreviations for orders of definitive host: Acc, Accipitriformes; Car, Carnivora; Chi, Chiroptera; Did, Didelphimorphia; Str, Strigiformes. †We also sequenced additional conspecific isolates collected from additional orders of definitive hosts.
On the basis of their phylogenetic position in the 18S, 28S and suprageneric cox1 analyses, N. microcotyle, N. reflexum, N. vaucheri and Neodiplostomum sp. VVT1 were included in the focused cox1 analysis together with former Fibricola spp. (clade I in Figs 1–3). This alignment was 456 bp long; three bases (one codon) were excluded from the analysis as an indel. The internal branch topology of the resulting tree (Fig. 4) was somewhat different and better resolved than in the 18S and 28S analyses. Neodiplostomum microcotyle + Neodiplostomum sp. VVT1 from B. virginianus + N. vaucheri formed a strongly (100%) supported clade separate from the 100% supported clade of N. reflexum + former Fibricola lineages. Neodiplostomum microcotyle was positioned as a sister group to a weakly supported clade of Neodiplostomum sp. VVT1 + N. vaucheri. All sequences of N. reflexum formed a 100% supported, long-branch clade as a sister clade to a weakly supported clade containing sequences of former Fibricola cf. cratera 3 (Fig. 4). The remaining sequences of former Fibricola formed two clades. One of them was weakly (82%) supported and included N. cf. cratera 1 (formerly F. cf. cratera 1) and specimens that were morphologically identified as N. cf. lucidum (formerly F. cf. lucidum) (Fig. 4). The other was a 100% supported clade of N. cf. cratera 2 (formerly F. cf. lucidum).

Fig. 4. Phylogenetic interrelationships among the 20 members of Neodiplostomum clade I based on BI analysis of partial cox1 mtDNA gene sequences. BI posterior probability values lower than 80% are not shown. The new sequences generated are indicated in bold. The scale bar indicates the number of substitutions per site. GenBank accession numbers are provided after the names of species.
The cox1 alignment of the second Neodiplostomum clade (clade II in Figs 1–3) was 366 bp long. The sequences of N. americanum and N. banghami formed separate 100% supported clades (Fig. 5).
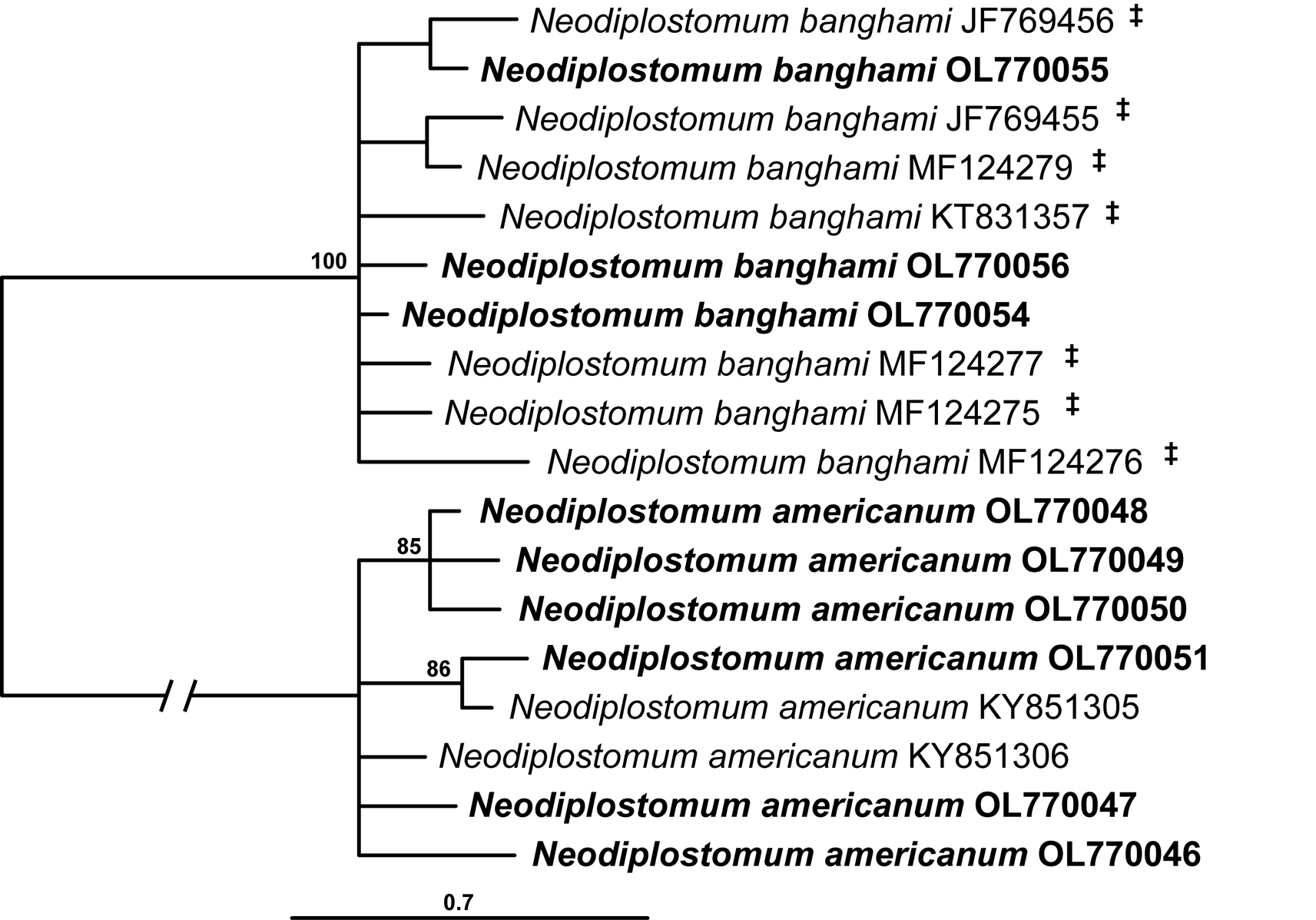
Fig. 5. Phylogenetic interrelationships among the two species of Neodiplostomum clade II based on BI analysis of partial cox1 mtDNA gene sequences. BI posterior probability values lower than 80% are not shown. The new sequences generated are indicated in bold. The scale bar indicates the number of substitutions per site. GenBank accession numbers are provided after the names of species. ‡Isolates previously identified as Neodiplostomum americanum in GenBank.
Genetic variation
Taxa included in clade I demonstrated a low interspecific divergence in 18S sequences (0–1.1%). No differences were detected among the 18S sequences of N. cf. cratera 3 (multiple sequences), N. cf. lucidum and N. reflexum; Neodiplostomum cf. cratera 3 vs N. vaucheri, N. cf. lucidum vs N. vaucheri and N. reflexum vs N. vaucheri had the greatest level of interspecific divergence in 18S among Neodiplostomum spp. in clade I. Neodiplostomum americanum and N. banghami, members of clade II, differed by 1.6% between their 18S sequences. No intraspecific variation was detected among the 18S sequences of N. cf. cratera 3 n. comb., N. reflexum and N. americanum. Complete pairwise comparisons of 18S sequences are provided in Table 2.
Table 2. Pairwise comparisons of partial sequences of the 18S rDNA among Neodiplostomum (syn. Fibricola) species included in this study based on a 1602 bp long alignment

Percentage differences are given above the diagonal and the number of variable nucleotide positions is given below the diagonal. Taxa previously included in Fibricola are denoted by *.
The interspecific divergence in 28S sequences of Neodiplostomum spp. (clade I) was similar to differences among the 18S sequences (0–1.2%). No differences were detected among the 28S sequences of N. cf. cratera 2 and 3 n. comb., N. cf. lucidum and N. reflexum. The unidentified Neodiplostomum sp. VVT1 from B. virginianus vs N. cf. cratera 2 and 3 n. comb., Neodiplostomum sp. VVT1 vs N. cf. lucidum and Neodiplostomum sp. VVT1 vs N. reflexum had the greatest level of interspecific divergence in 28S (1.2%) among Neodiplostomum spp. in clade I. In contrast, the overall interspecific variability among the members of Neodiplostomum spp. in clade II was greater; N. americanum and N. banghami were 3.7% divergent in the sequenced 28S fragment. Notably, no intraspecific variation in sequences of 28S was detected in any of the Neodiplostomum taxa with multiple isolates included in the analysis. Complete pairwise comparisons of 28S sequences are provided in Table 3.
Table 3. Pairwise comparisons of partial sequences of the 28S rDNA among Neodiplostomum (syn. Fibricola) species included in this study based on a 1176 bp long alignment

Percentage differences are given above the diagonal and the number of variable nucleotide positions is given below the diagonal. Taxa previously included in Fibricola are denoted by *.
The interspecific divergence in ITS1 + 5.8S + ITS2 sequences of Neodiplostomum spp. (clade I) was greater than among the 18S and 28S sequences (0.2–6.6%). Up to 0.1% variation was detected among the ITS region sequences of N. cf. lucidum and N. reflexum. Neodiplostomum reflexum and N. vaucheri were the most divergent pairs of sequences among Neodiplostomum spp. in clade I. Up to 0.2% variation was detected among the N. cf. lucidum/cratera lineages. At the same time, the interspecific variability among the ITS region sequences from the two members of Neodiplostomum spp. in clade II was even greater (9.3–9.4%). Intraspecific variation in sequences of the ITS region was detected in N. americanum (up to 0.6%). The divergence between the members of clades I and II was much greater (13–16.7%). Complete pairwise comparisons of ITS region (ITS1 + 5.8S + ITS2) sequences are provided in Table 4.
Table 4. Pairwise comparisons of ITS1 + 5.8S + ITS2 rDNA region among Neodiplostomum (syn. Fibricola) species included in this study based on a 1073 bp long alignment

Percentage differences are given above the diagonal and the number of variable nucleotide positions is given below the diagonal. Taxa previously included in Fibricola are denoted by *.
Interspecific differences of cox1 sequences among Neodiplostomum spp. of clade I, excluding the N. cf. cratera/N. cf. lucidum cluster, were in the range of 8.6–13.4%. Neodiplostomum vaucheri vs Neodiplostomum sp. VVT1 showed the lowest divergence (8.6–8.8%), whereas N. reflexum and N. microcotyle had the greatest divergence (12.7–13.4%). The N. cf. cratera/N. cf. lucidum cluster demonstrated up to 6.2% divergence among its members. The cox1 sequences of N. americanum and N. banghami (clade II) differed by 12.3–14.2%. With the exception of N. cf. cratera lineages, all Neodiplostomum spp. in clades I and II with more than a single sequence available showed no more than 1.6% intraspecific variation in cox1 sequences (Supplementary Tables S1 and S2). Complete pairwise comparisons of cox1 sequences of clade I Neodiplostomum taxa are provided in Supplementary Table S1 and clade II Neodiplostomum taxa in Supplementary Table S2.
Discussion
The systematic histories of Fibricola and Neodiplostomum are complex and tightly interwoven. Dubois (Reference Dubois1932) originally established genus Fibricola for F. cratera described from muskrat Ondatra zibethicus (Linnaeus) by Barker (Reference Barker1915). Subsequently, Dubois (Reference Dubois1937) added Fibricola minor Dubois, 1936 to the genus and noted an error in the topography of reproductive system organs in the original description of F. cratera. Dubois (Reference Dubois1937) used parasitism in mammals along with confinement of the vitellarium to the prosoma as the justification for separation between Fibricola and Neodiplostomum which typically parasitizes birds and has vitellarium in both parts of the body. However, Dubois (Reference Dubois1938) noted that the vitellarium of F. cratera may extend into the opisthosoma to the level of the anterior testis. Miller (Reference Miller1940) later described a second North American species of the genus, Fibricola laruei Miller, Reference Miller1940, from raccoon Procyon lotor (Linnaeus) collected in Quebec.
A third member of the genus from North America, Fibricola texensis Chandler, 1942, was described based on specimens collected from P. lotor in Texas. The original description of the species reported its vitellarium extending to variable levels in the opisthosoma (Chandler, Reference Chandler1942). Additionally, Chandler (Reference Chandler1942) noted that the vitellarium of F. laruei also extended into the opisthosoma, but only to the level of the vitelline reservoir situated between the testes. Zerecero (Reference Zerecero1943) subsequently described the fourth species of Fibricola from North America, Fibricola caballeroi Zerecero, 1943, collected from the brown, or Norway, rat Rattus norvegicus (Berkenhout) in Mexico.
Later, Dubois (Reference Dubois1944) erected Theriodiplostomum Dubois, 1944 for F. texensis and N. lucidum from Virginia opossum Didelphis virginiana (Kerr) collected in Texas, based on vitellarium distributed in both the prosoma and opisthosoma and parasitism in mammals. Theriodiplostomum spp. were considered morphologically intermediate forms between Fibricola and Neodiplostomum (Dubois, Reference Dubois1944).
Chandler and Rausch (Reference Chandler and Rausch1946) described a fifth member of Fibricola in North America, Fibricola nana Chandler and Rausch, 1946, from American red squirrel Tamiasciurus hudsonicus (Erxleben) (syn. Sciurus hudsonicus) in Michigan. Importantly, Chandler and Rausch (Reference Chandler and Rausch1946) deemed the use of the distribution of vitellarium and parasitism in either mammals or birds not tenable for differentiation among the genera and rejected Theriodiplostomum. Read (Reference Read1948) agreed with this decision and considered F. nana and F. laruei synonyms of F. cratera. Read (Reference Read1948) proposed the tendency for greater concentration of vitelline follicles in the prosoma in members of Fibricola species as the main distinguishing character from Neodiplostomum spp.
Dubois and Rausch (Reference Dubois and Rausch1950) transferred the former Theriodiplostomum lucidum (La Rue and Bosma, 1927) to Fibricola. In contrast to the previous authors, Pearson (Reference Pearson1959) viewed Fibricola as a subgenus of Neodiplostomum. Odening (Reference Odening1965) maintained Fibricola as a subgenus of Neodiplostomum based on similarities of larval morphology (i.e. the identical flame cell formula, 2[(1 + 1 + 1) + (1 + 1 + [1])] = 12).
Several Fibricola spp. were previously described from mammalian hosts outside of North America and later transferred to Neodiplostomum. For example, N. seoulensis, described from R. norvegicus collected in Korea, was originally included in Fibricola based, in part, on parasitism in mammals. Noteworthily, this species has been reported from humans in Korea (Huh et al., Reference Huh, Lee and Huh1994). Hong and Shoop (Reference Hong and Shoop1994) transferred this species into Neodiplostomum based on the morphology of adults and metacercariae. Similarly, Cribb and Pearson (Reference Cribb and Pearson1993) transferred three Fibricola spp. from Australian mammals into Neodiplostomum based on adult morphology.
Despite similarities in larval and adult morphology, Dubois (Reference Dubois1970) rejected the placement of Fibricola as a subgenus of Neodiplostomum and insisted that the distribution of vitellarium and specificity to mammals were sufficient for separation between the two genera. In spite of his own statement, Dubois (Reference Dubois1983) placed N. vaucheri collected from a chiropteran host into Neodiplostomum.
Although specificity to either mammalian or avian hosts has often been used as a distinguishing characteristic of Fibricola and Neodiplostomum species, some studies (e.g. Ulmer, Reference Ulmer1955; Seo, Reference Seo1989) demonstrated that Fibricola spp. can develop in avian hosts. Nevertheless, the most recent revision of the Diplostomoidea by Niewiadomska (Reference Niewiadomska, Gibson, Jones and Bray2002) maintained Fibricola and Neodiplostomum as separate genera belonging to different subfamilies (the Alariinae and the Diplostominae Poirier, 1886, correspondingly) based on parasitism in either mammals or birds. Shoop (Reference Shoop1989) provided an alternative hypothesis for the subfamily structure of the Diplostomidae; in his system, Fibricola was placed together with Neodiplostomum within the Neodiplostominae Shoop, 1989 based on morphology. Recently, Achatz et al. (Reference Achatz, Chermak, Martens, Pulis, Fecchio, Bell, Greiman, Cromwell, Brant, Kent and Tkach2021c) rejected the use of subfamilies of the Diplostomidae based on morphological and molecular data. The molecular phylogeny presented by Achatz et al. (Reference Achatz, Chermak, Martens, Pulis, Fecchio, Bell, Greiman, Cromwell, Brant, Kent and Tkach2021c) and other recent molecular phylogenetic studies (e.g. Hernández-Mena et al., Reference Hernández-Mena, García-Varela and Pérez-Ponce de León2017; Achatz et al., Reference Achatz, Pulis, Fecchio, Schlosser and Tkach2019c, Reference Achatz, Martens, Kostadinova, Pulis, Orlofske, Bell, Fecchio, Oyarzún-Ruiz, Syrota and Tkach2022, Reference Achatz, Chermak, Martens, Woodyard, Rosser, Pulis, Weinstein, McAllister, Kinsella and Tkachin press; Queiroz et al., Reference Queiroz, López-Hernández, Locke, Pinto and Anjos2020) clearly do not support the system provided by Shoop (Reference Shoop1989).
Heneberg et al. (Reference Heneberg, Sitko and Těšínský2020) demonstrated the non-monophyly of Neodiplostomum and proposed Conodiplostomum to be a junior synonym of Neodiplostomum based on molecular phylogenies. Unfortunately, this solution did not remove the problem of the non-monophyly of Neodiplostomum. Members of Neodiplostomum consistently formed two distinct clades in our analyses (Figs 1–3). Currently, 18S and 28S sequences of N. spathulaeforme (type-species) are not available. The suprageneric analysis of shorter fragment of cox1 (Fig. 3) revealed a fairly well supported clade of Neodiplostomum (including N. spathulaeforme) + former Fibricola + the former type-species of Conodiplostomum (N. spathula). At the same time, the second well-supported clade of Neodiplostomum was positioned separately within this phylogeny (Fig. 3) and contained only N. americanum + N. banghami. Similar patterns related to the constituents of the two Neodiplostomum clades (e.g. the position of Fibricola within clade I) were strongly supported in 18S and 28S analyses (Figs 1 and 2). The position of the type-species of Neodiplostomum (N. spathulaeforme) in the suprageneric analysis of cox1 (Fig. 3) clearly indicates that taxa within clade I should be considered true Neodiplostomum.
On the basis of our examination of adult morphology (e.g. variable distribution of vitellarium in the prosoma and opisthosoma among and within Fibricola species) and previous studies of larval morphology (e.g. Odening, Reference Odening1965), no morphological characters reliably support the status of Fibricola as an independent genus. Neodiplostomum reflexum from avian hosts and F. cratera lineages from mammals lack any differences among the sequences of 18S and 28S, which demonstrates the taxa to be congeneric. Molecular data demonstrate the lack of specificity to mammalian or avian definitive hosts within the Neodiplostomum + Fibricola clade. Therefore, we consider Fibricola to be a junior synonym of Neodiplostomum and transfer the constituent species of Fibricola into Neodiplostomum. Fibricola cratera and F. caballeroi are being transferred into Neodiplostomum as N. cratera n. comb. and Neodiplostomum caballeroi Zerecero, 1943, respectively. Notably, F. lucidum was originally described as N. lucidum; thus, this species is returned to its original genus. Below, we provide an amended diagnosis of Neodiplostomum based on the diagnosis by Niewiadomska (Reference Niewiadomska, Gibson, Jones and Bray2002). Due to the lack of distinct morphological features differentiating Neodiplostomum spp. clade II from true Neodiplostomum (clade I), we temporarily retain the species from clade II within Neodiplostomum. We anticipate that future detailed studies of their morphology and/or life cycles will provide differentiating characters and may allow placement of the clade II members into a currently undescribed genus.
Neodiplostomum Railliet, 1919 (after Niewiadomska, Reference Niewiadomska, Gibson, Jones and Bray2002 with changes)
Diagnosis: Body distinctly bipartite; prosoma spatulate or oval; opisthosoma cylindrical or oval. Pseudosuckers absent. Oral and ventral suckers and pharynx present. Holdfast organ round or oval, with median slit. Testes of similar size, tandem; anterior usually asymmetrical; posterior symmetrical, often bilobed. Ovary reniform or ellipsoidal, pretesticular, median or submedian, situated close to borderline between prosoma and opisthosoma, rarely near middle of opisthosoma. Vitellarium may extend almost to intestinal bifurcation. Copulatory bursa small or large; genital cone absent; hermaphroditic duct opens directly into bursa. In avian and mammalian definitive hosts. Cosmopolitan. Metacercariae in amphibians; paratenic hosts reptilians and mammals. Cercariae with two pairs of pre- and paracetabular penetration glands; flame-cell formula 2[(1 + 1 + 1) + (1 + 1 + [1])] = 12. Type-species N. spathulaeforme (Brandes, 1888).
After the re-evaluation of the validity of Fibricola and its constituents in North America, 11 valid named species of Neodiplostomum are currently known from North America: N. cratera n. comb., N. lucidum and N. caballeroi n. comb. from mammals as well as Neodiplostomum accipitris Dubois and Rausch, 1948, N. attenuatum, Neodiplostomum centuri Dubois and Macko, 1972, Neodiplostomum isomegalocotyle Dubois and Macko, 1972, Neodiplostomum pearsoni Dubois, 1962, N. reflexum, N. americanum, N. banghami from birds (e.g. Dubois, Reference Dubois1968, Reference Dubois1982; Dubois and Macko, Reference Dubois and Macko1972; current data). As mentioned above, N. americanum and N. banghami are kept in Neodiplostomum only provisionally due to the lack of suitable differentiating morphological characters. The same may potentially apply to N. accipitris, N. centuri, N. isomegalocotyle and N. pearsoni for which sequence data are currently lacking.
Notably, our data revealed the presence of three genetically distinct lineages of digeneans morphologically corresponding to N. cratera in North America (Fig. 4). One of these lineages appeared in the clade with specimens morphologically corresponding to N. lucidum. Our adult specimens of N. cf. cratera collected from several mammalian hosts throughout the USA, morphologically conform to the original description of F. cratera by Barker (Reference Barker1915) from O. zibethicus collected in Nebraska and redescribed by Dubois (Reference Dubois1937). Because this situation does not affect the main conclusions from the present phylogenetic study, we cautiously designate these forms as N. cf. cratera 1–3 and N. cf. lucidum. Although the cox1 sequences of N. cf. cratera 1 (GenBank: OL770020) were clearly conspecific to sequences of samples that morphologically correspond to N. cf. lucidum, the cox1 sequences of N. cf. cratera 1 and 2 differ from N. cf. cratera 3 by 4.6–6.2% of nucleotide positions (Supplementary Table S1). Currently, N. cratera and N. lucidum are differentiated based on the distribution of vitellarium (primarily in prosoma in N. cratera vs extending far into opisthosoma in N. lucidum) (e.g. Dubois, Reference Dubois1968). However, based on our data, it is clear that distribution of vitellarium cannot be used to distinguish between these species.
Interestingly, the morphology of samples in the cluster of N. cf. cratera 3 somewhat varied. Specimens collected in the northern USA (HWML-216766, 216767) were distinctly smaller than the sequenced specimen from Mississippi (HWML-216755). These morphologically distinct forms from geographically distant regions differed by 2.6–2.9% of nucleotide positions in cox1. It should be mentioned that the vertebrate hosts of these species have broad, overlapping distributions. Notably, F. laruei, a species synonymized with F. cratera (=N. cratera) by Read (Reference Read1948), was originally described from Canada, relatively close to the area where we collected our specimens. The main characters differentiating F. laruei from F. cratera were the smaller body size and elliptical shape of the holdfast organ in the former species. The somewhat significant level of genetic divergence between the larger form from the south and smaller form from the north suggests that the validity of F. laruei may need to be re-visited. A definitive answer can be obtained only when DNA sequence data from the type territory of F. laruei (Quebec) become available and the question of morphological identity of N. cratera is resolved.
Our results clearly demonstrate that the sequences of N. americanum available in GenBank represent two distinct species (Tables 2 and 3, Fig. 3; Supplementary Table S2). Our specimens of N. americanum are conspecific with specimens previously published by Woodyard et al. (Reference Woodyard, Rosser and Griffin2017) based on partial sequences of 28S, the ITS region and cox1. Furthermore, our specimens and the material of Woodyard et al. (Reference Woodyard, Rosser and Griffin2017) conform to the original morphological description of N. americanum by Chandler and Rausch (Reference Chandler and Rausch1947). Our morphological examination of voucher specimens of adult N. americanum sequenced by Blasco-Costa and Locke (Reference Blasco-Costa and Locke2017) revealed that the taxon was misidentified. The morphological characteristics of N. americanum sequenced and deposited by Blasco-Costa and Locke (Reference Blasco-Costa and Locke2017) closely conform to those of N. banghami (Supplementary Table S3). Additionally, cox1 sequences of N. americanum published by Blasco-Costa and Locke (Reference Blasco-Costa and Locke2017) are clearly conspecific with our sequences of N. banghami (Supplementary Table S2; Fig. 5).
Our cox1 phylogeny (Fig. 3) demonstrated at least two independent host-switching events between avian and mammalian hosts in the evolutionary history of Neodiplostomum. The clade of N. reflexum + a cluster of [N. cf. lucidum + N. cf. cratera] suggest a transition from avian definitive hosts (orders Accipitriformes Vieillot and Strigiformes Wagler) to a diversity of mammalian definitive hosts (orders Carnivora Bowdich and Didelphimorphia Gill). The position of N. vaucheri in a clade with N. microcotyle and Neodiplostomum sp. VVT1 confirmed the initial generic placement of this species by Dubois (Reference Dubois1983) and revealed a transition to bats (order Chiroptera Blumenbach); additional data are needed to determine the directionality of the secondary host-switching event due to the lack of internal support within this clade. The bat in which N. vaucheri was found is known to feed on amphibians. This dietary overlap with more traditional hosts of Neodiplostomum spp. (birds of prey, carnivorous mammals) created conditions for host switching. It remains to be observed how DNA sequences from other former Fibricola species that parasitize mammals as adults (e.g. N. caballeroi n. comb.) and species from southeast Asia and Australia (e.g. N. australiense) will impact the current picture of the interrelationships of Neodiplostomum.
Similar to other previous molecular phylogenetic studies, in our analyses Neodiplostomum did not form a clade with other members of the formerly accepted Diplostominae (Figs 1–3) (e.g. Achatz et al., Reference Achatz, Dmytrieva, Kuzmin and Tkach2019b, Reference Achatz, Bell, Melo, Fecchio and Tkach2021a, Reference Achatz, Chermak, Martens, Pulis, Fecchio, Bell, Greiman, Cromwell, Brant, Kent and Tkach2021c; Queiroz et al., Reference Queiroz, López-Hernández, Locke, Pinto and Anjos2020). Our results, along with other recent molecular phylogenetic studies (e.g. Blasco-Costa and Locke, Reference Blasco-Costa and Locke2017; Hernández-Mena et al., Reference Hernández-Mena, García-Varela and Pérez-Ponce de León2017; Locke et al., Reference Locke, Van Dam, Caffara, Pinto, López-Hernández and Blanar2018; Sereno-Uribe et al., Reference Sereno-Uribe, Andrade-Gómez, Ostrowski de Núñez, Pérez-Ponce de León and García-Varela2019; Achatz et al., Reference Achatz, Pulis, González-Acuña and Tkach2020, Reference Achatz, Bell, Melo, Fecchio and Tkach2021a, Reference Achatz, Brito, Fecchio and Tkach2021b, Reference Achatz, Chermak, Martens, Pulis, Fecchio, Bell, Greiman, Cromwell, Brant, Kent and Tkach2021c, Reference Achatz, Martens, Kostadinova, Pulis, Orlofske, Bell, Fecchio, Oyarzún-Ruiz, Syrota and Tkach2022, Reference Achatz, Chermak, Martens, Woodyard, Rosser, Pulis, Weinstein, McAllister, Kinsella and Tkachin press; Queiroz et al., Reference Queiroz, López-Hernández, Locke, Pinto and Anjos2020; Locke et al., Reference Locke, Drago, López-Hernández, Chibwana, Núñez, Van Dam, Achinelly, Johnson, Assis, Melo and Pinto2021), strongly suggest that the most recently accepted subfamilies of the Diplostomidae cannot be considered valid. Achatz et al. (Reference Achatz, Chermak, Martens, Pulis, Fecchio, Bell, Greiman, Cromwell, Brant, Kent and Tkach2021c) rejected the subfamily system of the Diplostomidae. The data presented in the current study and Reference Achatz, Chermak, Martens, Woodyard, Rosser, Pulis, Weinstein, McAllister, Kinsella and TkachAchatz et al. (in press) further corroborate the decision by Achatz et al. (Reference Achatz, Chermak, Martens, Pulis, Fecchio, Bell, Greiman, Cromwell, Brant, Kent and Tkach2021c). Re-evaluation of the systematics of the superfamily Diplostomoidea remains necessary, but is beyond the scope of the current study.
Supplementary material
The supplementary material for this article can be found at https://doi.org/10.1017/S003118202100216X.
Data availability
The data supporting the findings of this study are available within the article and in the Supplementary materials. All newly generated sequences were deposited in the GenBank database under the following accession numbers: OL764381, OL770020–OL770068, OL770124–OL770126 and OL799069–OL799108.
Acknowledgements
The authors are grateful to Tim Driscoll for providing carcases of hawks and owls. The authors are grateful to Dr João B. Pinho (Universidade Federal de Mato Grosso, Cuiabá, Brazil) for his help in organizing the collection of the specimens used in this study and in obtaining collecting permits for avian hosts in Brazil. The authors also extend their gratitude to Dr James Flowers for providing some of the specimens used in this study. The authors also acknowledge Dr Isabel Blasco-Costa of the Natural History Museum of Geneva and Georgia Tschen, Katie Ahlfeld and Dr Anna Phillips of the Smithsonian Institution Museum of Natural History for sending voucher specimens.
Author contributions
TJA, EEP, ETW, TGR and VVT conceived and supervised the study. All authors involved in the collection of digeneans or their hosts. TJA, EEP, ETW, TGR, JRM and VVT conducted the morphological and molecular studies of the digeneans. TJA, JRM and VVT were responsible for writing and revising the manuscript. All authors read and approved the final manuscript.
Financial support
This study was supported by the National Science Foundation, USA (VVT, grant DEB-1120734) The University of North Dakota (TJA, Esther Wadsworth Hall Wheeler Award, Student Research Stipend and Summer Doctoral Fellowship; JRM, Student Research Stipend) and the American Society of Parasitologists (ETW, Willis A Reid, Jr. Student Research Grant). AF was supported by a postdoctoral fellowship (PNPD scholarship) from the Coordenação de Aperfeiçoamento de Pessoal de Nível Superior (CAPES). JRM was supported by the National Science Foundation (REU Site award number 1852459) and the National Institute of General Medical Sciences of the National Institutes of Health (Institutional Development Award (IDeA) grant number P20GM103442) and the University of North Dakota School of Medicine & Health Sciences. Examination of specimens deposited at the NHM was supported (VVT) by the SYNTHESYS+ (project: http://www.synthesys.info/) financed by European Community Research Infrastructure Action under the H2020 Integrating Activities Programme, project number 823827.
Conflict of interest
The authors declare there are no conflicts of interest.
Ethical standards
All applicable institutional, national and international guidelines for the collecting, care and use of animals were followed.