INTRODUCTION
Cryptosporidiosis, the most common parasitic gastrointestinal disease in the Scottish population, is caused mainly by 2 protozoan pathogens, Cryptosporidium parvum (C. parvum) and Cryptosporidium hominis (C. hominis) which account for over 90% of human cryptosporidiosis worldwide (Putignani et al. Reference Pönka, Kotilainen, Rimhanen-Finne, Hokkanen, Hänninen, Kaarna, Meri and Kuusi2010). Cryptosporidium species are globally distributed, having a wide host range and transmission of infective oocysts can be either zoonotic or anthroponotic. Various transmission routes of infective fecal material are possible, including direct contact or contact via contaminated food and water sources (Xiao et al. Reference Xiao and Feng2008). Cryptosporidiosis is a self-limiting infection, but can be life-threatening in immunocompromised patients who may suffer from severe, chronic infection resulting in significant losses of fluid (Chalmers and Davies, Reference Chalmers and Davies2010; Bouzid et al. Reference Bouzid, Hunter, Chalmers and Tyler2013).
Microscopy is widely used by diagnostic laboratories to establish the presence or absence of Cryptosporidium oocysts. However, it does not permit speciation as the morphology of oocysts from different Cryptosporidium species, including those which are not clinically significant, can be similar. The advent of molecular diagnostic tools permits further analysis to determine specific Cryptosporidium species and subtypes which is crucial for the management of outbreaks. A variety of molecular methods exist which employ 16s rRNA-based tools, 18S rRNA genotyping, classification according to the outer wall protein gene (COWP), the heat-shock protein 70 gene (HSP 70), the 60 kDa glycoprotein gene (GP60) and various polymorphic loci markers (Feng et al. Reference Feng, Torres, Li, Wanga, Bowman and Xiao2013). The GP60 gene is currently the most widely used genetic marker containing a hyper-variable microsatellite region for investigating Cryptosporidium species whilst also being one of the dominant targets for neutralizing antibody responses in humans (Strong et al. Reference Strong, Gut and Nelson2000).
The GP60 subtyping tool has shown C. hominis to be highly conserved (Chalmers et al. Reference Chalmers, Hadfield, Jackson, Elwin, Xiao and Hunter2008), whilst C. parvum is genetically heterogeneous (Xiao, Reference Xiao2010) resulting in a range of differing subtypes. In Scotland, insufficient information exists on the prevalence, significance and regional molecular epidemiology of Cryptosporidium isolates that cause human disease. This information is essential to facilitate appropriate investigations and to manage both regional and national Cryptosporidium outbreaks impacting on human health. In this report, we describe the prevalence and molecular diversity of C. parvum subtypes from human isolates throughout Scotland. The GP60 subtyping tool was employed during a 2-year period to investigate C. parvum subtypes as part of the remit of the Scottish Parasite Diagnostic and Reference Laboratory (SPDRL) Cryptosporidium Outbreak Service in conjunction with Health Protection Scotland.
MATERIALS AND METHODS
Microscopy detection of Cryptosporidium oocysts
Fecal samples from patients throughout Scotland with gastrointestinal illness were submitted to local diagnostic microbiology laboratories from General Practitioners and Hospital clinicians. Feces were examined for the presence of Cryptosporidium oocysts by staining with either auramine phenol (AP) or modified Ziehl–Neelsen (ZN) based on the UK Standards for Microbiology Investigations (SIM Staining Procedures TP 39). Briefly air dried smears were fixed in methanol before the addition of either AP or ZN. Excess stain was removed with water before decolourizing slides using 3% acid methanol (for AP staining) or 1% acid methanol (for ZN staining). Potassium permanganate (0·1%) or malachite green (0·4%) were used as counterstains. Slides were washed, air-dried and examined for Cryptosporidium oocysts at ×40 objective either using a light microscope to detect ZN staining, or using a fluorescence microscope with FITC filters (690/510 nm wavelength) for AP staining.
Oocyst concentration and DNA extraction
Feces reported by diagnostic microbiology laboratories to be microscopy positive for Cryptosporidium oocysts, and which were deemed to be associated with potential outbreaks, were forwarded to the SPDRL for speciation and subtyping. The definitions used by Health Protection Scotland to define an outbreak are an incident in which 2 or more linked cases experience the same illness or when the observed number of cases unaccountably exceeds the expected number. On arrival at SPDRL, oocysts were concentrated by adding approximately 500 mg of feces to 7 mL of water and 3 mL of diethyl ether. The mixture was inverted for 30 s before being centrifuged at 750 g for 60 s. The sediment was transferred to a sterile tube, and 4 μL of internal control DNA (PrimerDesign, Southampton UK) added before DNA was extracted using the QIAamp DNA stool mini kit (QIAGEN, Germany).
Cryptosporidium speciation
To permit speciation, DNA was amplified using 2 real-time PCR assays to determine if samples were either C. parvum or C. hominis (Hadfield et al. Reference Hadfield, Robinson, Elwin and Chalmers2011). One assay detected C. hominis and an internal control, whilst the second assay detected C. parvum. The C. hominis assay consisted of primers CRULib13F and CRULib13RCh (CRULib13F final concentration 900 nm; CRULib13RCh final concentration 300 nm; Eurofins MWG Operon, Germany), CRULib13TMCh probe (100 nm; Eurofins MWG Operon, Germany) containing 2 μL sample DNA, 1·6 μL internal control primers/probe mix (PrimerDesign, Southampton UK) and 12·5 μL LightCycler 480 probes master mix (Roche Diagnostics, UK) in a total volume of 25 μL. The second assay to detect C. parvum consisted of primers CRULib13F and CRULib13RCp at a final concentration 900 nm, whilst the probe, CRULib13TMCp was at a final concentration of 150 nm. Amplification was performed on a Roche LC480 (Roche Diagnostics, UK) using cycling conditions as described (Hadfield et al. Reference Hadfield, Robinson, Elwin and Chalmers2011). Negative controls (water or extraction buffer) and positive controls (DNA from C. parvum oocysts, IOWA isolate, Idaho, USA and previous positive clinical samples) were included in each experiment. For those isolates that were shown to be negative by the real-time PCR assays, but microscopy positive for Cryptosporidium oocysts, DNA amplification was performed using a previously published nested PCR assay targeting the 18S rRNA gene at Locus 1 using a modified forward primer, WR494F and reverse primer, XR1 (Nichols et al. Reference Nichols, Connelly, Sullivan and Smith2010). The secondary PCR amplicon of approximately 435 bp was subjected to sequencing using an ABI 3500XL sequencer with Big-Dye version 3.1 chemistry.
Cryptosporidium subtyping at the GP60 locus
Samples identified as C. parvum by the real-time PCR assays were subtyped using a nested PCR approach targeting the GP60 gene (Glaberman et al. Reference Glaberman, Moore, Lowery, Chalmers, Sulaiman, Elwin, Rooney, Millar, Dooley, Lal and Xiao2002). To account for variations in DNA concentration and the presence of potential PCR inhibitors, 2 volumes (2 and 3 μL) of DNA template were used for the first PCR amplification (PCR1) in a total volume of 50 μL containing 200 μ m dNTPs (Invitrogen Ltd, UK), 400 μg mL−1 BSA (Sigma-Aldrich, UK), 2% Tween-20 (VWR, UK), 1% PVP pH8 (Sigma-Aldrich, UK), 6 mm MgCl2 (Fisher Scientific, Loughborough, UK), 200 nm of each oligonucleotide GF1 and GR1 (Eurofins MWG Operon, Germany), 5 Units Taq polymerase (Fisher Scientific, Loughborough, UK) and 5 μL Reaction Buffer IV (Fisher Scientific, Loughborough, UK). Amplifications were performed on a 9700 GeneAmp platform, (Applied Biosystems, UK) using cycling conditions as previously described (Glaberman et al. Reference Glaberman, Moore, Lowery, Chalmers, Sulaiman, Elwin, Rooney, Millar, Dooley, Lal and Xiao2002).
Both 2 and 3 μL were used for the second PCR amplification (PCR2) which was performed using the same conditions as PCR1 with the exception of oligonucleotides, GF2 and GR2 and the exclusion of PVP. Negative and positive controls as stated for the real-time PCR assays were included in every experiment. An amplicon of approximately 900 base pairs was visualized by running 10 μL of PCR2 product on a 1·4% agarose gel. Subtyping of PCR2 amplicons was performed by bi-directional sequencing using the oligonucleotides from PCR2. Bi-directional sequencing was performed on-site using an ABI 3500XL sequencer with Big-Dye version 3.1. Searching for similar sequences on the GenBank database was performed using the NCBI BLASTn tool (http://blast.ncbi.nlm.nih.gov/Blast.cgi). Subtypes were confirmed by manually reading through the sequences to search for predominantly trinucleotide repeats and other repeat sequences (Xiao, Reference Xiao2010).
RESULTS
Prevalence and seasonality of Cryptosporidium species
During a 2-year period, 2012 and 2013, a total of 1139 samples in Scotland were reported by diagnostic microbiology laboratories to be microscopy positive for Cryptosporidium oocysts. The seasonal distribution of all Cryptosporidium species is depicted for each year (Fig. 1). Two distinct peaks occurred; 1 during April–May (n = 201; i.e. 28% in 2012; n = 150, 35% in 2013) and the other during October–November (n = 215; 30% of Cryptosporidium species isolates in 2012; n = 93, 22% in 2013).
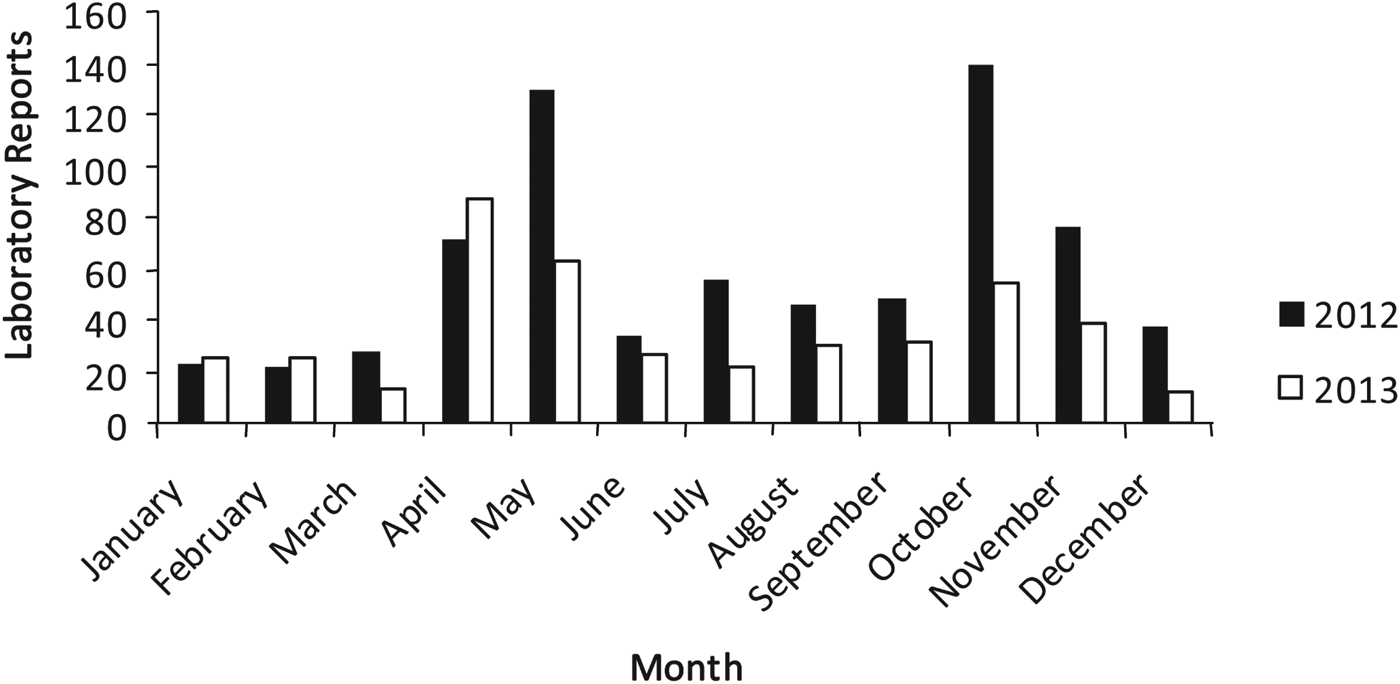
Fig. 1. Number of Cryptosporidium species reported in Scotland during 2012 and 2013.
Cryptosporidium genotypes and C. parvum subtypes
Of the 1139 isolates, 445 were identified to the species level in response to potential outbreak investigations; C. parvum (n = 256); C. hominis (n = 187); C. felis (n = 1) and C. meleagridis (n = 1). Over the 2-year period, the majority of C. parvum isolates were sent from Grampian, Fife and Ayrshire/Arran (n = 67, 45 and 41, respectively), whereas the majority of C. hominis isolates were from Grampian, Lothian and Glasgow (n = 53, 26 and 26, respectively) (Fig. 2). Of the 256 C. parvum isolates, 158 were identified in 2012 compared to 98 in 2013. Only 2 were stated to be imported and the sex ratio was similar with 54% (n = 140) isolated from females and 46% (n = 116) from males. The median age of infected individuals was 20 years (2012 = 19 years, 2013 = 21 years). Cryptosporidium parvum demonstrated marked seasonality with numbers peaking during April–May (n = 80, in 2012; n = 66, in 2013) (Fig. 3).

Fig. 2. Distribution of the total number of Cryptosporidium species per Scottish Health Board during 2012 and 2013.

Fig. 3. Seasonal distribution of C. parvum in humans within Scotland during 2012 and 2013.
Of the 256 C. parvum isolates, a total of 87 were subjected to further analysis for GP60 subtyping to assist with 5 potential outbreak investigations. Putative associations included animal contact at a visitor farm (also referred to as a petting farm), farms and consumption of contaminated food. These associations were based on limited patient information provided to local health protection teams and outbreak investigation teams, rather than being based on laboratory evidence, largely due to the challenges in obtaining samples from environmental sources. The most prevalent subtype in the Scottish population was IIaA15G2R1 which comprised of 47% (n = 41) of those samples subtyped (26 females: 15 males, age range 1–50) (Table 1). The second and third most common were IIaA17G1R1 (n = 14, 16%) and IIaA19G1R1 (n = 11, 9%), respectively (Table 1). Further 16 isolates were identified as belonging to the IIa family, whilst unusual non-IIa strains included; IIcA5G3 (n = 2), IIdA19G1 (n = 1), IIdA16G1 (n = 1) and IIgA9R1 (n = 1) (Table 1). Age ranges and sex ratios were similar between the different subtypes, with ages ranged from 1 to 50 years with a greater number of females than males affected in 4 of the 5 outbreaks.
Table 1. Summary of Scottish C. parvum outbreaks during 2012 and 2013

The IIaA15G2R1 subtype was the most common in Fife, Grampian and Tayside (n = 18, 8 and 5, respectively) (Table 2), whereas one of the largest health boards in Scotland, namely Lothian was predominantly non-IIaA15G2R1 subtypes (n = 13), as was Ayrshire and Arran (n = 12). All other Health Boards had similar numbers of isolates of IIaA15G2R1 vs non-IIaA15G2R1 subtypes.
Table 2. Distribution of C. parvum subtypes from human isolates across Scotland

DISCUSSION
This is the first report to describe the molecular epidemiology of C. parvum subtypes which infect humans in Scotland. In addition, it highlights cryptosporidiosis as being the most frequently reported intestinal parasitic disease with more than 3 times the number of cases than the second most frequently reported intestinal parasite, Giardia species (n = 191, in 2012; n = 167, in 2013) (communications with Health Protection Scotland).
Cryptosporidiosis cases show seasonal fluctuations particularly during 2012 with sharp increases from April to June then later in October and November. During May 2012, the large rise in the number of C. parvum cases across the UK prompted enhanced surveillance and a case controlled study which identified contaminated ‘ready to eat’ pre-cut mixed salad leaves being implicated as the most likely cause of illness (manuscript submitted). Cryptosporidium parvum outbreaks have previously been associated with food sources, including salad (Pönka et al. Reference Pönka, Kotilainen, Rimhanen-Finne, Hokkanen, Hänninen, Kaarna, Meri and Kuusi2009), contaminated fruit/juice and beef (Yoshida et al. Reference Yoshida, Matsuo, Miyoshi, Uchino, Nakaguchi, Fukumoto, Teranaka and Tanaka2007; Vojdani et al. Reference Vojdani, Beuchat and Tauxe2008). Springtime peaks of C. parvum have been noted in various geographical regions, including New Zealand, Netherlands, Ireland, China and England (McLauchlin et al. Reference McLauchlin, Amar, Pedraza-Diaz and Nichols2000; Learmonth et al. Reference Learmonth, Ionas, Ebbett and Kwan2004; Wielinga et al. Reference Wielinga, de Vries, van der Goot, Mank, Mars, Kortbeek and van der Giessen2008; Zintl et al. Reference Zintl, Proctor, Read, Dewaal, Shanaghy, Fanning and Mulcahy2009; Wang et al. Reference Wang, Wang, Sun, Zhang, Jian, Qi, Ning and Xiao2011). The springtime peak usually corresponds to an increase of infectious fecal material during the calving/lambing season. Isolates from human cases within and out-with the UK have the same GP60 subtypes as animal sources supporting the hypothesis that ruminants are the sources of infection (Trotz et al. Reference Trotz-Williams, Martin, Gatei, Cama, Peregrine, Martin, Nydam, Jamieson and Xiao2006; Thompson et al. Reference Thompson, Dooley, Kenny, McCoy, Lowery, Moore and Xiao2007; Ng et al. Reference Ng, Eastwood, Durrheim, Massey, Walker, Armson and Ryan2008; Wielinga et al. Reference Wielinga, de Vries, van der Goot, Mank, Mars, Kortbeek and van der Giessen2008; Zintl et al. Reference Zintl, Proctor, Read, Dewaal, Shanaghy, Fanning and Mulcahy2009; Smith et al. Reference Smith, Chalmers, Mueller-Doblies, Clifton-Hadley, Elwin, Watkins, Paiba, Hadfield and Giles2010). A further sharp rise in cases occurred during late summer of 2012, but in this instance, C. hominis rather than C. parvum was implicated. A similar rise in C. hominis cases was also noted in the Netherlands and Germany with no common source identified (Fournet et al. Reference Fournet, Deege, Urbanus, Nichols, Rosner, Chalmers, Gorton, Pollock, van der Giessen, Wever, Dorigo-Zetsma, Mulder, Mank, Overdevest, Kusters, van Pelt and Kortbeek2013).
Previous studies demonstrate the differences in prevalence rates between C. hominis and C. parvum depending on the geographical location where, in the Netherlands, a greater percentage of C. hominis has been described (Wielinga et al. Reference Wielinga, de Vries, van der Goot, Mank, Mars, Kortbeek and van der Giessen2008). In contrast, our study demonstrates that, since 2012, there were almost twice as many cases of C. parvum than C. hominis, a finding similar to previous reports from Ireland and the USA (Feltus et al. Reference Feltus, Giddings, Schneck, Monson, Warshauer and McEvoy2006; Zintl et al. Reference Zintl, Proctor, Read, Dewaal, Shanaghy, Fanning and Mulcahy2009). Only around one-third of the isolates in Scotland are fully speciated in response to potential outbreak investigations; therefore to gain accurate data pertaining to the numbers of specific Cryptosporidium species in both sporadic and outbreak cases would require speciation of all isolates. However, the data achieved during 2012 and 2013 highlight a possible change in the trend within Scotland where previously, the total numbers of C. parvum and C. hominis isolates from sporadic and outbreak cases were similar (Chalmers and Pollock, Reference Chalmers and Pollock2012).
It is interesting to note the comparatively large number of C. parvum isolates from health boards comprising predominantly rural settings, including Grampian, Fife and Ayrshire/Arran compared with Glasgow and Lothian, which are the 2 largest urban settings in Scotland. A previous study highlighted that C. parvum was more prevalent in rural areas with lower population density and in those with a higher density of livestock reflecting the zoonotic nature of the infection (Pollock et al. Reference Pollock, Ternent, Mellor, Chalmers, Smith, Ramsay and Innocent2010). Within the rural areas of Scotland, there are over 11 000 private water supplies compared to less than 10 in urban areas. Areas with a greater number of private water supplies to human inhabitants have reported a greater number of C. parvum cases (Pollock et al. Reference Pollock, Ternent, Mellor, Chalmers, Smith, Ramsay and Innocent2010). Similarly, in New Zealand and Ireland, an increased prevalence of C. parvum in rural, lowly populated areas has been described (Learmonth et al. Reference Learmonth, Ionas, Ebbett and Kwan2004; Zintl et al. Reference Zintl, Proctor, Read, Dewaal, Shanaghy, Fanning and Mulcahy2009).
In this study, 3 of the Scottish outbreaks were suspected to be linked to contact with animals on farms based on the limited data available to the local Health Protection Team and Outbreak Control teams. There is strong evidence to highlight that, for C. parvum, contact with farm animals or their feces either through visiting or residing on a farm is associated with illness (Hunter et al. Reference Hunter, Hughes, Woodhouse, Syed, Verlander, Chalmers, Morgan, Nichols, Beeching and Osborn2004, Reference Hunter, Hadfield, Wilkinson, Lake, Harrison and Chalmers2007). Worldwide evidence for potential zoonotic transmission between cattle and humans on farms also exists (Feltus et al. Reference Feltus, Giddings, Schneck, Monson, Warshauer and McEvoy2006; Khan et al. Reference Khan, Debnath, Pramanik, Xiao, Nozaki and Ganguly2010).
The geographical distribution and prevalence of common Cryptosporidium subtypes vary and predominant subtypes identified in our analysis belonged to the IIa family which is the only one to be identified in cattle in Scotland. We report that IIaA15G2R1 is highly prevalent in the Scottish population, accounting for almost half of those isolates subtyped and this subtype is also commonly found in cattle within Scotland (personal communications, Hotchkiss, E., Moredun Institute, Midlothian); therefore it is likely that zoonotic transmission occurs. This same subtype is common in cattle in the Netherlands, Spain and the USA, (Xiao et al. Reference Xiao, Zhou, Santin, Yang and Fayer2007; Quilez et al. Reference Quilez, Torres, Chalmers, Robinson, Del Cacho and Sanchez-Acedo2008a ; Wielinga et al. Reference Wielinga, de Vries, van der Goot, Mank, Mars, Kortbeek and van der Giessen2008) and also in humans (Wielinga et al. Reference Wielinga, de Vries, van der Goot, Mank, Mars, Kortbeek and van der Giessen2008). It is also prevalent throughout farms in England with evidence for local transmission between farms (Brook et al. Reference Brook, Anthony Hart, French and Christley2009). Chalmers et al. (Reference Chalmers, Smith, Hadfield, Elwin and Giles2011) demonstrated that in England, IIaA15G2R1 and IIaA17G1R1 subtypes predominated with subtype IIaA17G1R1 being associated with human cases who had visited farms or had previous animal contact prior to the onset of any illness. IIaA17G1R1 was also commonly identified in our study but without enhanced surveillance, it was not possible to determine the degree of animal contact for each case to clearly establish an association. In contrast, in Ireland, the most prevalent subtype in human and cattle cases of cryptosporidiosis is IIaA18G3R1 (Thompson et al. Reference Thompson, Dooley, Kenny, McCoy, Lowery, Moore and Xiao2007; Zintl et al. Reference Zintl, Proctor, Read, Dewaal, Shanaghy, Fanning and Mulcahy2009, Reference Zintl, Ezzaty-Mirashemi, Chalmers, Elwin, Mulcahy, Lucy and De Waal2011). This subtype is also one of the most commonly identified in human cases in Australia (Ng et al. Reference Ng, MacKenzie and Ryan2010) and has been identified in foals (Grinberg et al. Reference Grinberg, Learmonth, Kwan, Pomroy, Lopez Villalobos, Gibson and Widmer2008). Interestingly, no Scottish cases were identified with this subtype.
In addition to the IIa family, a small number of non-IIa strains were also identified, namely IIc, IId and IIg. The IIc subtype appears to be uncommon in Scottish human cases and has not been identified in cattle in this region providing supporting evidence for anthroponotic transmission. In contrast, the IIc family is found relatively frequently in other regions including Portugal and Slovenia (Alves et al. Reference Alves, Xiao, Sulaiman, Lal, Matos and Antunes2003; Soba and Logar, Reference Soba and Logar2008). Although in low numbers, cases of the IId family have been reported to cause human cryptosporidiosis in Portugal, the Netherlands, Ireland, Jordan, Japan, Spain, Kuwait and Australia (Alves et al. Reference Alves, Xiao, Sulaiman, Lal, Matos and Antunes2003; Sulaiman et al. Reference Sulaiman, Hira, Zhou, Al-Ali, Al-Shelahi, Shweiki, Iqbal, Khalid and Xiao2005; Abe et al. Reference Abe, Matsubayashi, Kimata and Iseki2006; Quilez et al. Reference Quilez, Torres, Chalmers, Hadfield, Del Cacho and Sanchez-Acedo2008b ; Wielinga et al. Reference Wielinga, de Vries, van der Goot, Mank, Mars, Kortbeek and van der Giessen2008; Zintl et al. Reference Zintl, Proctor, Read, Dewaal, Shanaghy, Fanning and Mulcahy2009; Hijjawi et al. Reference Hijjawi, Ng, Yang, Atoum and Ryan2010). Human subtypes, namely IIdA17G1 and IIdA18G1 have been isolated in a small number of cases in England (Chalmers et al. Reference Chalmers, Smith, Hadfield, Elwin and Giles2011), which differ from the 2 IId subtypes in this report. The IId family has been isolated from lambs, goats and cattle in Spain, China and Malaysia suggesting these animals as potential sources (Quilez et al. Reference Quilez, Torres, Chalmers, Robinson, Del Cacho and Sanchez-Acedo2008a , Reference Quilez, Torres, Chalmers, Hadfield, Del Cacho and Sanchez-Acedo b ; Muhid et al. Reference Muhid, Robertson, Ng and Ryan2011; Wang et al. Reference Wang, Wang, Sun, Zhang, Jian, Qi, Ning and Xiao2011). A third non-IIa strain, namely IIg identified in this study was first reported in Uganda (Akiyoshi et al. Reference Akiyoshi, Tumwine, Bakeera-Kitaka and Tzipori2006) and has rarely been reported elsewhere. As neither the IIg nor the IId family have been isolated in Scottish animals, it is likely that the cases in this report have been acquired abroad however the travel history in each case was not stated.
Establishing enhanced surveillance of Cryptosporidium cases in Scotland and typing of both sporadic and outbreak isolates would provide valuable information to increase our understanding of potential transmission routes, clinical symptoms and the biological impact of specific subtypes. In addition, future studies are required to validate methodologies for typing of human Cryptosporidium isolates to ensure robust, standardized procedures are in place to assist with the management of future local and national outbreaks.