INTRODUCTION
Fasciola gigantica is a common liver fluke in the tropics that routinely infects ruminants and accidentally infects human hosts. Its close relative in temperate regions is Fasciola hepatica. Worldwide, both species result in substantial economic loss due to increased morbidity of the infected animals (WHO, 1995; Mas-Coma et al. 1999; Pfukenyi and Mukaratirwa, 2004). Attempts have been made to devise diagnostic methods and vaccines based on specific fluke proteins that could detect and kill the parasites (Mulcahy and Dalton, 2001; Wedrychowicz et al. 2003). Tegument proteins are considered to be more species-specific than other metabolic proteins such as digestive enzymes (Chaithirayanon et al. 2002). Furthermore, during development of the parasite, different tegumental proteins are expressed and may help the parasites to evade the host immune response (Hanna, 1980). The activity, distribution, and expression of these proteins may be controlled by signal transduction processes that use key regulator molecules such as the 14-3-3 protein. The 14-3-3 protein, which has been analysed in many different species, contains highly conserved domains and exists in several isoforms. Functionally, it is involved in many biological processes either as a mediator that brings together different binding partners or chaperone-like causing conformational changes in the bound proteins and thereby, for example, modifying their activity or localization (reviewed by Aitken (1996) and Dougherty and Morrison (2004)).
The 14-3-3 proteins of Schistosoma mansoni and Echinococcus multilocularis have been tested as vaccine candidates in mice and the results indicate that they have the potential to be used as protective tools (Schechtman et al. 2001a; Siles-Lucas et al. 2003). Furthermore, in vitro 14-3-3 protein of E. multilocularis has been shown to reduce nitric oxide production by host cells (Andrade et al. 2004). So far, 14-3-3 protein has not been studied in Fasciola and it is therefore not known in which biological processes it is involved in the tegument of this parasite. In the present study, we have cloned a 14-3-3 protein encoding cDNA by screening F. gigantica cDNA libraries using an antiserum raised against tegumental antigens and have analysed the RNA and protein expression patterns in juvenile and adult F. gigantica.
MATERIALS AND METHODS
Parasite collection
Newly excysted juveniles (NEJ) of F. gigantica were obtained by activating metacercariae in 2% pepsin and 0·5% HCl at 37 °C for 45 min, in 20 mM sodium dithionite, 0·2% taurocholic acid, 1% NaHCO3, 0·8% NaCl, and finally in 0·5% HCl at 37 °C for 45 min. Then the metacercariae were excysted in RPMI-1640 medium (Sigma Chemical Co., St Louis, MO, USA) containing 10% normal fetal calf serum and 10 μg/ml gentamycin at 37 °C for 3 h. The NEJ were collected and kept for further experiments. Four-week-old juveniles were obtained from mice experimentally infected with metacercariae via the oral route. Adult worms were collected from the bile ducts and gall bladders of naturally infected animals killed at local abattoirs.
Schistosoma japonicum and S. mansoni were obtained from mice 8 weeks after infection with cercariae. Eurythrema spp. and Paramphistomum spp. were collected from naturally infected cattle. The parasites were washed 3 times with Hank's balanced salt solution (HBS) to remove all traces of contaminating blood and bile before being processed further for subsequent experiments.
Preparation of crude antigen extracts and a rabbit antiserum against tegumental antigens
Protein extracts from mouse tissue (brain, liver, kidney, heart, spleen, muscles, and testis), whole trematodes, and F. gigantica excretory-secretory antigens (ES) and tegumental antigens were prepared as described previously (Chaithirayanon et al. 2002). New Zealand white rabbits were immunized with 200 μg of the F. gigantica tegumental antigen extract mixed with Freund's complete adjuvant. Two booster injections were administered at 3-week intervals with antigen extract mixed with incomplete Freund's adjuvant, and antisera were obtained from the rabbits 1 week after the third immunization.
Immunoscreening of adult F. gigantica cDNA library
An adult stage cDNA library of F. gigantica constructed in λ TriplEx2 (Meemon et al. 2004) was screened with a rabbit antiserum against F. gigantica tegumental antigens using the picoBlue Immunoscreening Kit (Stratagene). Briefly, the proteins expressed by the bacteria were transfered to a nitrocellulose membrane (Schleicher and Schuell) and probed with the pre-absorbed (E. coli) antiserum (1[ratio ]200). Alkaline phosphatase-conjugated goat anti-rabbit IgG was used as secondary antibody. The enzymatic detection employed 5-bromo-4-chloro-3-indolylphosphate/nitroblue tetrazolium substrates. Clones which remained positive after low-density re-screening were converted to pTriplEx2, and analysed by restriction endonuclease digestion and agarose gel electrophoresis. The selected cDNA insert was subcloned in the Kpn I-Xba I cloning sites of the plasmid pBluescript SK− (Stratagene). The plasmid was propagated in Escherichia coli XL1-blue according to standard protocols (Sambrook and Russell, 2001). DNA sequence analysis was performed by MWG AG Biotech, Germany.
Analysis of 14-3-3 sequences
NCBI BLAST was used to screen for homologous 14-3-3 sequences. The sequences were then retrieved either from the GenBank database (CD137944, CD171110, XP_884002) or the UniProt Knowledgebase (UniProtKB) database through the EMBL sequence retrieval system. The Schistosoma mansoni sequence SM_CD137944 is then combined with the translation product of the 2 overlapping (221 bp/100% identity) GenBank ESTs CD137944 and CD171110 (reverse complementary). All retrieved sequences were aligned using MUSCLE 3.41 (Edgar, 2004). The alignment file is available by anonymous FTP from FTP.EBI.AC.UK in directory/pub/databases/embl/align or via the EMBLALIGN database via SRS at http://srs.ebi.ac.uk; under accession ALIGN_000976. An unrooted phylogenetic tree was constructed with the Phylip 3.65 software (http://evolution.gs.washington.edu/phylip.html) using neighbour-joining with bootstrapping analysis. The Accession numbers of all used 14-3-3 sequences are shown in Fig. 2.
Genomic DNA isolation and Southern blot analysis
A digoxigenin (DIG)-labelled RNA antisense probe of the full length Fg14-3-3 cDNA was produced with the DIG RNA Labeling Kit (Roche) and used in Southern, Northern, and in situ hybridizations. A DIG-labelled Fg14-3-3 RNA sense probe was used as a negative control. Genomic DNA extraction and Southern blot analysis were performed as described previously (Grams et al. 2001). The F. gigantica genomic DNA was digested with BamH I, EcoR I, and Xho I (MBI Fermentas) and size-separated by gel electrophoresis in a 0·7% agarose gel. The Fg14-3-3 cDNA does not contain recognition sites for the selected restriction endonucleases. The DNA was transferred to a nylon membrane (Schleicher and Schuell) and fixed to the membrane at 80 °C. The DNA was hybridized with the antisense RNA probe at 50 °C. Detection of the hybridization products was done according to the manual of the DIG detection kit (Roche).
RNA isolation and Northern blot analysis
Total RNA of adult F. gigantica was extracted using TRIzol reagent (Invitrogen). Electrophoresis of total RNA from adult worms (20 μg per lane) was carried out alongside a RNA size-marker (0·24 to 9·5 kb RNA ladder, Invitrogen) in a 1·2% agarose/2·2 M formaldehyde gel (Grams et al. 2001). After capillary transfer of the RNA to a nylon membrane (Schleicher and Schuell), it was hybridized with the DIG-labelled Fg14-3-3 antisense RNA probe at 75 °C. The membrane was washed at maximum stringency with 0·1×SSC/0·1% SDS at 75 °C. The hybridization products were detected by the same protocol as used for Southern analysis.
RNA in situ hybridization
Four-week-old juveniles and adult worms were fixed in 4% (w/v) paraformaldehyde in DEPC-treated phosphate buffer saline, pH 7·4 for 3 h at room temperature, cut into blocks and embedded in Paraplast. Sections were cut at a thickness of 8 μm, dewaxed, rehydrated, and permeabilized with 5–20 μg/ml RNase-free proteinase K in TE buffer at 37 °C for 30 min, post-fixed with 4% (w/v) paraformaldehyde in PBS at 4 °C for 5 min and pre-hybridized in 4×SSC/50% (v/v) deionized formamide in PBS at 37 °C for at least 10 min. Subsequently, the sections were incubated in hybridization solution (40% deionized formamide, 10% dextran sulfate, 1× Denhardt's solution, 4×SSC, 10 mM dithiothreitol, 1 mg/ml yeast t-RNA, 1 mg/ml denatured and sheared salmon sperm DNA) containing 5 ng of DIG-labelled Fg14-3-3 antisense RNA probe at 42 °C overnight. This experiment was later repeated at a hybridization temperature of 55 °C. The hybridized sections were washed twice with 2×SSC and 1×SSC for 15 min each, and then incubated with 20 μg/ml RNase A in NTE buffer (500 mM NaCl, 10 mM Tris, 1 mM EDTA, pH 8·0) for 30 min at 37 °C. After 2 washes in 0·1×SSC for 30 min each, the detection was done using alkaline phosphatase-conjugated anti-digoxigenin antibodies (Roche) and NBT/BCIP (nitroblue tetrazolium chloride, 5-bromo-4-chloro-3-indolyl phosphate) for colour development. Whole NEJs were fixed with 4% (w/v) paraformaldehyde in DEPC-treated phosphate buffer saline, pH 7·4, overnight and the whole mount in situ hybridization was done as described by Grens et al. (1996).
Expression of recombinant F. gigantica 14-3-3 protein in E. coli and production of a 14-3-3 antiserum in rabbit
The coding sequence of Fg14-3-3 was amplified using specific forward and reverse primers that contained recognition sites for Nhe I and Hind III endonucleases (5′-cat atg TCG CCG TGC TGG TTG AC-3′ and 5′-aag ctt GTC ACC AGC ATC AAC CTC-3′). The PCR product was digested with Nhe I and Hind III (MBI Fermentas), gel purified and subcloned into the bacterial expression vector pET21a (Novagen). After transformation of E. coli BL21 (DE3), expression was induced with isopropyl-β-D-thiogalactopyranoside (IPTG) and the recombinant Fg14-3-3 protein was purified under denaturing conditions in 6 M urea by Ni-NTA column chromatography (Novagen). The purified rFg14-3-3 protein was analysed by SDS-PAGE. A polyclonal antiserum against the purified rFg14-3-3 was produced in a rabbit. The rabbit was subcutaneously immunized with 125 μg of rFg14-3-3-6 as described above.
Western blot analyses
The extracted proteins from parasite and mouse and rFg14-3-3 protein were size-separated by 12·5% SDS-PAGE and transferred to nitrocellulose membranes. Non-specific binding sites were blocked with 5% non-fat dry milk in TBST (TBS, 0·05% Tween 20) before the nitrocellulose membranes were incubated with the rabbit anti-rFg14-3-3 antiserum (1[ratio ]2000). Alkaline phosphatase (AP)-conjugated goat anti-rabbit IgG (1[ratio ]2000 in TBST) was used as secondary antibody and NBT/BCIP substrates were used for enzymatic detection. Pre-infected rabbit sera (NRS) were used as negative controls.
Immunolocalization of Fg14-3-3 protein
Paraffin sections of adult F. gigantica were deparaffinized in xylene for 10 min and rehydrated in decreasing concentrations of ethanol (100%, 90%, 80%, 70%) for 5 min each. The sections were rinsed with water and were then incubated in citrate buffer, pH 6·0 for 5 min twice and rinsed in tap water for 5 min. Then the treated sections were overlaid with 3% hydrogen peroxide in methanol for 30 min. Non-specific binding was blocked with normal horse serum for 10 min before incubation with rabbit anti-rFg14-3-3 antiserum (1[ratio ]500) in buffer containing 1·5% blocking serum for 1 h. After washing with PBS for 5 min, the sections were incubated with biotinylated horse anti-rabbit IgG (Vector Laboratories, Inc., Burlingame, CA) for 10 min, washed with PBS, and incubated with streptavidin/peroxidase complex solution for 10 min. Colorimetric detection was performed using aminoethyl carbazole as substrate (AEC, Zymed Laboratories Inc.) and the reaction was terminated with several washes with distilled H2O.
RESULTS
Isolation and identification of the cDNA encoding Fg14-3-3 protein 1
A single positive clone was selected after high- and low-density immunoscreening of 2×105 phages of an adult F. gigantica cDNA library with rabbit antiserum against F. gigantica tegumental antigens. The obtained Fg14-3-3 cDNA has a size of 869 bp and contains a single 759 bp open reading frame. This open reading frame encodes a protein containing 252 amino acids with a calculated molecular mass of 28·7 kDa and an isoelectric point of 4·96. A search in the PROSITE database (Bairoch and Bucher, 1994), showed that this sequence contains 2 signature motifs of the 14-3-3 protein family, PS00796, [RA]-N-L-[LIV]-S-[VG]-[GA]-Y-[KN]-N-[IVA] and PS00797, Y-K-[DE]-[SG]-T-L-I-[IML]-Q-L-[LF]-[RHC]-D-N-[LF]-T-[LS]-W-[TANS]-[SAD] which are located between amino acids 53–63 and 221–240, respectively (Fig. 1). A multiple alignment of Fg14-3-3 with known and deduced amino acid sequences of 14-3-3 proteins from H. sapiens, S. japonicum, and S. mansoni created in the MUSCLE program (Edgar, 2004) revealed a third conserved sequence motif between residues 171–213 (Fig. 1). It is the TPH binding site that associates with phosphorylated tryptophan hydroxylase and is also used for binding of the Raf kinase (Ichimura et al. 1995). In amino acid sequence comparisons, Fg14-3-3 protein 1 showed the highest identity values with the 14-3-3 beta, zeta, theta, and eta isoforms of vertebrate species and with the 14-3-3 protein 2 (56·5%) and CD137944 (60·3%) of S. mansoni (Table 1). The 14-3-3 epsilon class isoforms found in many species including Schistosoma seem to be more distantly related proteins with identity values between 45·4 and 50·6% (Table 1). To classify Fg14-3-3 protein 1 within the 14-3-3 isoforms found in other species, an unrooted phylogenetic tree was constructed using neighbour joining (Saitou and Nei, 1987) (Fig. 2). Fg14-3-3 protein 1 was found to be closely related with S. mansoni and S. japonicum 14-3-3 protein 1. These 3 proteins and 2 further, not described, 14-3-3 sequences of S. japonicum (Q5DA69) and S. mansoni (deduced from ESTs CD137944/CD171110), form a cluster and are more related to the non-epsilon 14-3-3 classes of the compared species. The nucleotide sequence data of Fg14-3-3 are available in the GenBank™, EMBL and DDBJ databases under the Accession number AY878648.
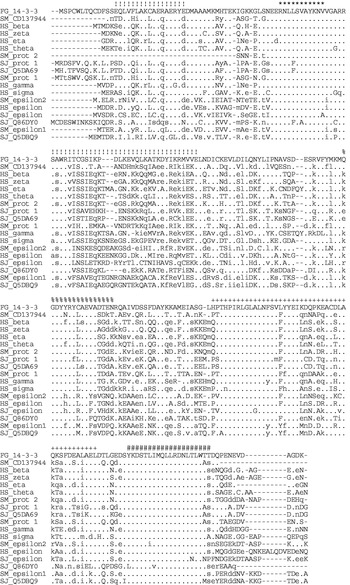
Fig. 1. Multiple alignment of the deduced amino acid sequence of Fg14-3-3 protein 1 with the amino acid sequences of 14-3-3 proteins from Homo sapiens, Schistosoma japonicum, and S. mansoni. The sequence of Fg14-3-3 protein 1 is shown in full, the remaining sequences are sorted by their identity values (from highest to lowest, see Table 1) as compared with Fg14-3-3 protein 1. A dot (.) indicates identical residues, lower case letters indicate similar residues and upper case letters indicate different residues. Star (*) and hatch characters (#) indicate the two PROSITE signature motifs PS00796 and PS00797 of the 14-3-3 protein family. The plus signs (+) indicate the TPH binding site. The exclamation marks (!) indicate the residues involved in the dimerization of 14-3-3 proteins. The percentage signs (%) indicate the exocytosis-stimulating motif found in annexin and 14-3-3 proteins. The sequences database Accession numbers are given in Fig. 2.

Fig. 2. Phylogenetic analysis of 14-3-3 proteins. A multiple sequence alignment of the listed 14-3-3 sequences was done in MUSCLE 3.41. Phylip 3.65 was used to create an unrooted phylogenetic tree using the neighbour-joining method and bootstrapping; the bootstrap values are shown. Fg14-3-3 is clustered with Schistosoma japonicum and S. mansoni 14-3-3 protein 1 isoforms.

Southern and Northern hybridization analyses
To validate that the isolated cDNA represents a DNA sequence from F. gigantica and to assess the 14-3-3 gene copy number, a Southern blot analysis was performed. Under stringent hybridization conditions, a DIG-labelled Fg14-3-3 antisense RNA probe hybridized with varying intensities with up to 4 fragments of genomic DNA, BamH I (3 fragments), EcoR I (4 fragments) and Xho I (1 fragment) (Fig. 3A). The results suggest that the isolated Fg14-3-3 gene is a single copy gene.

Fig. 3. Nucleic acid hybridization analyses with a DIG-labelled Fg14-3-3 antisense RNA probe. (A) Southern hybridization of Fasciola gigantica genomic DNA (15 μg/well) digested with BamH I, EcoR I, and Xho I (lanes 1, 2 and 3, respectively). The positions of standards for fragment sizes (lambda DNA digested with EcoR I/Hind III) are indicated on the left. (B) Northern hybridization of 25 μg total RNA from adult F. gigantica. The detected Fg14-3-3 transcripts have a size of 1250 nucleotides. Positions of the RNA size standard fragments are indicated on the left.
In a Northern blot analysis of total RNA from adult F. gigantica hybridized with a Fg14-3-3 antisense RNA probe under highly stringent hybridization conditions a 1·25 kb RNA product of the Fg14-3-3 gene was detected (Fig. 3B).
Expression of Fg14-3-3 in developmental stages of F. gigantica
To examine the distribution of Fg14-3-3 RNA in various tissues of different developmental stages, tissue sections of 4-week-old and adult F. gigantica were analysed by in situ hybridization with a Fg14-3-3 antisense RNA probe. In the adult stage, positive staining was detected in the cytoplasm of various cell types including caecal epithelial cells, parenchymal cells, and tegumental cells (Fig. 4B). Hybridization signals in the muscle tissue were generally weak or absent (Fig. 4B). The concentration of Fg14-3-3 transcripts was high in the reproductive organs, including the prostrate gland and cells underlying the cirrus epithelium, while the epithelium itself was unstained (Fig. 4C). The Mehlis gland cells were intensely stained (Fig. 4E), while the testicular cells, ovarian cells, vitelline cells and ovum within the eggs were moderately stained (Fig. 4D, F, G). The control, hybridized with Fg14-3-3 sense RNA, showed no staining (Fig. 4A). In 4-week-old juveniles, a similar hybridization pattern with the Fg14-3-3 antisense RNA probe was observed (Fig. 5B). In a whole mount hybridization of NEJ, the Fg14-3-3 antisense probe showed particularly intense staining along the digestive tract, including pharynx and the caecal branches (Fig. 5D), whereas no staining was observed in the negative control section (Fig 5C).

Fig. 4. RNA in situ hybridization with 14-3-3 probes in tissue sections of adult Fasciola gigantica. (A) Negative control hybridized with a Fg14-3-3 sense RNA probe. Ca caeca, Te tegument, Vi vitellaria. (B) Strong hybridization signals with the Fg14-3-3 antisense RNA probe are observed in the tegumental cells (Tc), caecal epithelial cells (Ca), parenchymal cells (Pc), and vitellaria (Vi), but not in muscle (Mu), nuclei (Nu), spines (Sp), syncytial tegument (Te). (C, D) In male reproductive organs, the Fg14-3-3 transcripts are abundant in prostrate gland (Pg, arrow), cells underlining the cirrus (Ci, arrow) and the germ cells in the testis (Ti). (E–G) Female reproductive organs exhibit intense staining of cells in Mehlis gland (Mg), vitelline cells within eggs (Eg) in the uterine lumen (F) and the germ cells in the ovary (Ov).

Fig. 5. RNA in situ hybridization with 14-3-3 probes in tissue sections of 4-week-old juvenile and NEJ Fasciola gigantica. (A, C) Control cross-sections of 4-week-old juvenile and NEJ hybridized with a Fg14-3-3 sense RNA probe. (B) In 4-week-old juveniles, the Fg14-3-3 antisense RNA probe shows strong staining in tegumental cells (Tc, arrows), caecal epithelial cells (Ca), and parenchymal cells (Pc). (D) In the whole mount hybridization of NEJ strong staining was found in the pharynx (Ph) and caeca (Ca), and moderate staining in parenchyma.
Expression and immunoreactivity of recombinant Fg14-3-3 protein
After induction by IPTG, rFg14-3-3 protein 1 was expressed in E. coli at the calculated size of 30 kDa (Fig. 6). This recombinant protein was used for the production of a polyclonal antiserum in rabbit. The antiserum detected the 14-3-3 antigen as a 29 kDa product in immunoblots of F. gigantica protein extracts from newly excysted juveniles, 4-week-old juveniles, adult worms, and the tegument (Fig. 7A). In the ES antigens the antiserum reacted with a 28 kDa product (Fig. 7A, lane ES). The antiserum showed slight variations in its reactivity against immunoblotted antigen extracts from S. japonicum, S. mansoni, Eurytrema spp., and Paramphistomum spp. Two products of slightly different size were detected in S. japonicum, 1 light immunoreactive band was found in S. mansoni, in Eurytrema spp., and in Paramphistomum spp. (Fig. 7B).

Fig. 6. SDS-PAGE (12·5%) showing expression and purification of recombinant Fg14-3-3 protein. Lane 1: non-induced bacterial lysate; lane 2: IPTG induced bacterial lysate; lane 3: recombinant Fg14-3-3 protein after purification by Ni-NTA (arrow). Positions of the molecular weight standards are indicated on the left.

Fig. 7. Western blot analyses of recombinant Fg14-3-3 (2·5 μg per lane) and protein extracts (12·5 μg per lane) from trematodes and mouse using a rabbit anti-rFg14-3-3 antiserum (A, B, C) and sera of Fasciola gigantica-infected rabbits (D). (A) Protein extracts from F. gigantica: newly excysted juveniles (NEJ), 4-week old juveniles (4 wk), whole body of adult worm (WB), excretory-secretory fraction (ES, adult), tegument fraction (TA, adult) and rFg14-3-3 protein. (B) Whole body extracts from trematodes: Schistosoma japonicum (Sj), S. mansoni (Sm), Eurytrema spp. (Eu), Paramphistomum spp. (Pa), and F. gigantica (Fg). (C) Protein extracts from mouse tissues: brain (Br), heart (He), kidney (Ki), liver (Li), spleen (Sp), muscle (Mu), and testis (Ti). (D) Detection of recombinant Fg14-3-3 protein by sera of rabbits orally infected with metacercariae 2, 5, 7, and 10 weeks post-infection. Position and size (kDa) of protein molecular weight markers are indicated on the left. NS, rabbit pre-immune serum.
The cross-reactivity of the antiserum against mammalian 14-3-3 protein was analysed in mice. Protein extracts from mouse brain, heart, liver, kidney, spleen, muscle, and testis exhibited differences in 14-3-3 abundance and isoforms. Two immunoreactive bands, with the major band at 30 kDa and a minor band at 31 kDa, were found in brain and testis (Fig. 7C). The tissue extracts from kidney, spleen, and liver showed only 1 moderately immunoreactive band at 30 kDa (Fig. 7C). The remaining tissues, i.e., heart and muscle, showed only a weak immunoreactive band at 30 kDa (Fig. 7C).
The humoral immune response against the 14-3-3 protein was analysed in a rabbit after oral infection with F. gigantica metacercariae. Antiserum collected from the rabbit 2 weeks after infection detected immunoblotted rFg14-3-3 and the intensity of the staining increased markedly along the course of infection up to 10 weeks, while the normal rabbit serum did not show any reactivity against the recombinant 14-3-3 protein (Fig. 7D).
Immunolocalization of 14-3-3 protein in adult F. gigantica by a rabbit anti-rFg14-3-3 antiserum
Paraffin sections of adult F. gigantica were probed with pre-immune rabbit serum (negative control) and rabbit anti-rFg14-3-3 antiserum. Rabbit pre-immune serum did not result in positive staining (Fig. 8A) whereas the anti-rFg14-3-3 antiserum detected the native protein throughout the body of the worms with the exception of muscle layers underlying the tegument and surrounding the caecum (Fig. 8B–E). The characteristic red staining of the AEC substrate was intense in the tegumental cells, their processes and the tegument syncytium, especially in its outer zone, while the spines were not stained (Fig. 8B–C). Light to moderate staining was found in the parenchymal cells and caecal epithelial cells, respectively. Also stained were the male reproductive organs, including the spermatogenetic cells of the testis (Fig. 8D), prostrate gland and its duct, seminal vesicle and cirrus (data not shown). In the female reproductive organs, staining was more intense in mature oocytes than in young oocytes located at the periphery of the ovary (Fig. 8E). Mehlis gland, vitelline cells, and ova inside the uterus were also stained (data not shown).
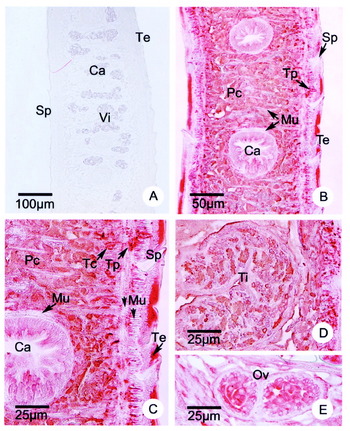
Fig. 8. Immunolocalization of Fg14-3-3 in tissue sections of adult Fasciola gigantica using a rabbit anti-rFg14-3-3 antiserum. (A) Control section probed with rabbit pre-immune serum. (B, C) Intense staining is visible in the tegument (Te), tegumental cells (Tc) and their processes (arrow), while no staining is visible in spines (Sp) and muscle tissue (Mu, arrowheads) underlying the tegument. Moderate and light staining was found in parenchymal cells (Pc) and caecal epithelial cells (Ca), respectively. (D, E) High magnification of male and female reproductive organs that show intense staining in the spermatogenetic cells of the testis (Ti) and strong to moderate staining in the ovum and surrounding vitelline cells.
DISCUSSION
Various isoforms of protein 14-3-3 with highly conserved sequence motifs have been isolated and characterized in eukaryotic organisms ranging from protozoa, fungi, and plants to mammals. They are reported to be involved in fundamental cellular processes, including signal transduction, cell-cycle control, apoptosis, and protein trafficking (reviewed by Aitken et al. 1992, 1995; Aitken, 1995, 1996, 2002). In Platyhelminthes, genes encoding various isoforms of protein 14-3-3 have been isolated and characterized in the blood fluke Schistosoma and the tapeworm Echinococcus (reviewed by Siles-Lucas and Gottstein, 2003). The 14-3-3 protein 1 of S. japonicum has been localized in the tegument, parenchyma, and muscles of adult parasites and 15-day-old schistosomula (Zhang et al. 1999). Consistent with these results, the homologous 14-3-3 protein 1 of S. mansoni has been localized in the tegument, parenchyma, muscle, and reproductive organs in both sexes of the adult parasite (Schechtman et al. 2001b). In E. multilocularis, 14-3-3 protein was abundant in the germinal layer of the metacestode stage (Siles-Lucas et al. 1998). Interestingly, in adult stage E. granulosus, 14-3-3 protein was not only detected as a cellular protein in the periphery of the testes but also externally at the apical rostellum and adjacent tegument (Siles-Lucas et al. 2000). In the present study, the 14-3-3 protein was detected for the first time in Fasciola. The abundant expression of Fg14-3-3 protein in the tegument of F. gigantica facilitated the cloning of the encoding cDNA by immunoscreening of an adult stage cDNA library with an antiserum against the parasite's tegumental antigens. The sequence analysis showed that the cloned Fasciola 14-3-3 cDNA encodes a homologue of the Schistosoma 14-3-3 protein 1 and is related to the 14-3-3 beta, zeta, theta, and eta isoforms described in other organisms ranging from Arthropoda to Mammalia. The epsilon isoforms of 14-3-3 protein in Schistosoma and other species possess more distantly related sequences. All 14-3-3 isoforms share highly conserved regions, which make it very likely that polyclonal antisera raised against any full-length 14-3-3 isoform will cross-react with other 14-3-3 isoforms. In structure models of 14-3-3 proteins, these sequences form part of a negatively charged tunnel, which facilitates the binding of target proteins with common features (Liu et al. 1995; Xiao et al. 1995).
The Southern hybridization of genomic DNA with a Fg14-3-3 probe exhibited a small number of hybridizing DNA fragments which indicates that only 1 or 2 Fg14-3-3 protein 1 encoding genes exist in F. gigantica. Other 14-3-3 encoding sequences, which are likely to be present, should possess divergent sequences that do not permit cross-hybridization. A comparison of the nucleic acid sequences of the schistosome 14-3-3 genes shows that only the clustered 14-3-3 protein 1 sequences have an ability to cross-hybridize. In the remaining schistosome 14-3-3 genes, the motifs that are highly conserved at the protein level are frequently changed in the third codon position, thereby preventing hybridization under stringent conditions. The obtained 869 bp Fg14-3-3 cDNA contains the full-length coding sequence but does not contain the full 5′ UTR sequence as indicated by the 1·25 kb hybridization signal obtained by Northern analysis. RNA in situ hybridization using an Fg14-3-3 RNA antisense probe and the immunohistochemical analysis using anti-rFg14-3-3 antiserum show corresponding results and demonstrate that Fg14-3-3 gene products can be found in many tissues of the parasite. In both techniques muscle tissue shows a low concentration of Fg14-3-3. The antiserum, raised against the full-length recombinant Fg14-3-3 protein 1, could detect other 14-3-3 isoforms due to the conserved motifs. This is attested by its cross-reaction to 14-3-3 proteins in various mouse tissues and antigen extracts of other parasites. Presently, it is not known whether 14-3-3 isoforms with different tissue-specific distribution exist in F. gigantica as Fg14-3-3 protein 1 reported here is the first isolated isoform from Fasciola. Interestingly, a 14-3-3 protein of slightly smaller size was detected in the excretion/secretion material of the parasite, while this smaller form is not present in whole body or tegument antigen extracts from the adult parasite. Fg14-3-3 protein 1, like all other described 14-3-3 proteins, does not contain a signal peptide. This suggests that proteolytic processing into the smaller form takes place after 14-3-3 protein is released into the ES material. Whether the protein has a function outside the parasite or whether its presence here is only the result of a putative involvement in exocytotic processes remains to be analysed. When compared to the published structure models of mammalian 14-3-3 proteins, the removal of up to 10 amino acids on either end of the Fg14-3-3 protein should not impair its dimerization and/or target protein binding properties as the involved motifs are not truncated.
The appearance of antibodies against Fg14-3-3 in a rabbit as early as 2 weeks after infection and the continuously increasing immunoreactivity until the end of the experiment suggest that the presence of this protein in the collected ES material is not an artifact. Furthermore, in the syncytial tegument and the gut epithelial cells, Fg14-3-3 protein is concentrated in the apical areas towards either tegument surface or gut lumen, the same area where secretory vesicles are concentrated and where exocytosis occurs. RNA and protein are also found in other secretory active tissues, namely Mehlis gland, prostrate gland, and vitelline cells. Secretion of 14-3-3 has been reported in adult E. granulosus (Siles-Lucas et al. 2000). Two forms of 14-3-3 protein were detected, a cytoplasmic form and a slightly smaller ‘secreted’ form. The external 14-3-3 protein was associated with the apical rostellum and adjacent worm tegument. In vitro, recombinant E. multilocularis 14-3-3 protein was shown to cause a reduction of nitric oxide as well as the mRNA of inducible nitric oxide synthetase (iNOS) in alveolar macrophages obtained from mouse (Andrade et al. 2004). This suggests that in vivo 14-3-3 protein may help the parasite to escape nitric oxide-mediated killing by cells of the host immune system. In F. hepatica it has been shown that rat peritoneal cells mediate in vitro killing of NEJ, that the severity of damage is correlated with the production of NO, and that the parasite's ES products have a role in the suppression of NO production in these cells during the first 14 days post-infection (Spithill et al. 1997; Cervi et al. 1998). Previous studies of 14-3-3 protein in Schistosoma have not included the ES material and may, therefore, have missed the presence of a secreted form. Likewise sera of schistosome-infected animals and human have not been analysed for their antibody titres against circulating 14-3-3 protein, although recombinant 14-3-3 protein 1 of S. japonicum was strongly recognized by sera from mice, rats and bovines vaccinated with irradiated S. japonicum cercariae (Zhang et al. 1999).
In several studies 14-3-3 proteins have been implicated in exocytosis, vesicle transport and membrane phospholipid binding. A conserved amino acid motif present in annexins and 14-3-3 proteins has been reported to facilitate calcium-dependent exocytosis in adrenal chromaffin cells (see feature annotation in Fig. 1) (Roth et al. 1993). Later, the direct binding of the epsilon and gamma 14-3-3 isoforms to phospholipids (Roth et al. 1994), a role of 14-3-3 in the priming of exocytosis (Chamberlain et al. 1995), and 14-3-3 regulated reorganization of the cortical actin network (Roth and Burgoyne, 1995) were reported. In Drosophila melanogaster, the 14-3-3 protein Leonardo was shown to play a role in the regulation of synaptic vesicle dynamics (Broadie et al. 1997). In rats, Rim, a component of the pre-synaptic active zone and modulator of exocytosis, was shown to bind 14-3-3 through its N terminus (Sun et al. 2003). In human embryonic kidney cells, 14-3-3 epsilon was reported to influence the secretion of endopeptidase 24.15 (Carreno et al. 2005). The observed Fg14-3-3 RNA/protein in Mehlis gland, prostrate gland, and vitelline cells also suggest a function in exocytosis within these tissues. The intensity of anti-Fg14-3-3 immunoreactivity is especially high in the tegument. Tegument and overlying glycocalyx shield the parasite from the host immune responses and the continuous turnover of tegument membrane and glycocalyx, mediated by the exocytotic fusion of tegumental granules (G1, G2 types) with the surface membrane, provides the protective mechanism necessary for the survival of the parasite (Sobhon et al. 2000). It is tempting to speculate that fusion of tegumental granules with the surface membrane through their exocytosis at the tegument surface, and the secretory processes in other tissues of Fasciola, are in some way regulated by 14-3-3 proteins. In further studies we would like to analyse the subcellular localization of Fg14-3-3 and determine the mechanism that leads to the secretion of Fg14-3-3. We are also interested in isolating additional 14-3-3 encoding sequences from F. gigantica and in analysing their tissue-specific expression. Finally, the use of Fg14-3-3 protein as a vaccine against fasciolosis will be analysed in animal models. The cross-reactivity of the anti-r14-3-3 antiserum to mouse 14-3-3 isoforms indicates that it is not possible to use the full-sized Fg14-3-3 protein 1 as a vaccine. Antisera must be raised against parasite-specific 14-3-3 peptide sequences.
This research was supported by the Thailand Research Fund (Senior Research Fellowship to Prasert Sobhon, and the Royal Golden Jubilee Ph.D. Scholarship to Kulathida Chaithirayanon).