Introduction
Amphibian populations throughout the world are suffering widespread declines and extinctions and diseases are an important driver of these amphibian losses (Stuart et al., Reference Stuart, Chanson, Cox, Young, Rodrigues, Fischman, Debra and Waller2004; Blaustein et al., Reference Blaustein, Gervasi, Johnson, Hoverman, Belden, Bradley and Xie2012). Chytridiomycosis, an emerging infectious disease caused by the fungal pathogen Batrachochytrium dendrobatidis, has received a great deal of scientific consideration, in that it is believed to be the lone culprit of such losses (Berger et al., Reference Berger, Speare, Daszak, Green, Cunningham, Goggin, Slocombe, Ragan, Hyatt, McDonald, Hines, Lips, Marantelli and Parkes1998; Longcore et al., Reference Longcore, Pessier and Nichols1999; Lips et al., Reference Lips, Brem, Brenes, Reeve, Alford, Voyles, Carey, Livo, Pessier and Collins2006; Skerratt et al., Reference Skerratt, Berger, Speare, Cashins, McDonald, Phillott, Hines and Kenyon2007; Fisher et al., Reference Fisher, Garner and Walker2009). However, comprehensive studies of amphibian losses have documented additional infectious agents contributing to such events, some of which caused declines of amphibian populations (Pessier, Reference Pessier2008; Berger et al., Reference Berger, Longcore, Speare, Hyatt, Skerratt, Heatwole and Wilkinson2009; Miller et al., Reference Miller, Gray and Storfer2011; Martel et al., Reference Martel, Spitzen-van der Sluijs, Blooi, Bert, Ducatelle, Fisher, Woeltjes, Bosman, Chiers, Bossuyt and Pasmans2013). There has been a growing interest in fungal-like protists placed at the boundary between animals and fungi, the Mesomycetozoea (Mendoza et al., Reference Mendoza, Taylor and Ajello2002), because their biological characteristics are typical of parasites capable of causing emerging infectious diseases in amphibian populations (Fisher et al., Reference Fisher, Henk, Briggs, Brownstein, Madoff, McCraw and Gurr2012; Rowley et al., Reference Rowley, Gleason, Andreou, Marshall, Lilje and Gozlan2013; Gleason et al., Reference Gleason, Chambouvet, Sullivan, Lilje and Rowley2014). Infections by mesomycetozoean parasites (order Dermocystida, genus Amphibiocystidium) were found in amphibians in the early 1900s and were reported to have mainly low incidence and limited pathogenicity (Pérez, Reference Pérez1907, Reference Pérez1913; Guyénot and Naville, Reference Guyénot and Naville1922; Remy, Reference Remy1931; Poisson, Reference Poisson1937; Carini, Reference Carini1940; Broz and Privora, Reference Broz and Privora1952). The only report of lethal amphibiocystidiosis, pertaining to captive Northern crested newt (Triturus cristatus), occurred in Germany (Moral, Reference Moral1913). Since the late 1990s, new outbreaks of such infections in European and North American amphibians have been causing alarm because of their potential effects on host health. An infectious disease by Amphibiocystidium ranae in frogs of the Pelophylax esculentus complex of Central Italy was associated with the observed frequency decrease of the parental species Pelophylax lessonae compared with the clonal hybrid P. esculentus (Pascolini et al., Reference Pascolini, Daszak, Cunningham, Tei, Vagnetti, Bucci, Fagotti and Di Rosa2003; Pereira et al., Reference Pereira, Di Rosa, Fagotti, Simoncelli, Pascolini and Mendoza2005; Simoncelli et al., Reference Simoncelli, Fagotti, Dall'Olio, Vagnetti, Pascolini and Di Rosa2005; Di Rosa et al., Reference Di Rosa, Simoncelli, Fagotti and Pascolini2007). Dermocystid lesions affecting palmate newts Lissotriton helveticus in France and in Scotland were considered to potentially interfere with newt physiological processes and compromise newt fitness (González-Hernández et al., Reference González-Hernández, Denoël, Duffus, Garner, Cunningham and Acevedo-Whitehouse2010; Fiegna et al., Reference Fiegna, Clarke, Shaw, Baily, Clare, Gray, Garner and Meredith2017). Moreover, a reduced survival rate observed in captive adults of the North American eastern red-spotted newt (Notophthalmus viridescens) was attributed to an infection by Amphibiocystidium viridescens, although the possibility that secondary infections could have been the source of mortality was not ruled out (Raffel et al., Reference Raffel, Bommarito, Barry, Witiak and Shackleton2008).
In all these cases, the potential pathogenicity and the population-level effects of parasites were reported as correlative evidence or speculative arguments; the demographic consequences of an Amphibiocystidium infection were not explored. To address the global amphibian decline phenomenon, the need for long-term field data has often been invoked (Blaustein et al., Reference Blaustein, Han, Relyea, Johnson, Buck, Gervasi and Kats2011; Pickett et al., Reference Pickett, Stockwell, Bower, Pollard, Garnham, Clulow and Mahony2014; Quick et al., Reference Quick, Goldingay, Parkyn and Newell2015; Wagner et al., Reference Wagner, Neubeck, Guicking, Finke, Wittich, Weising, Geske and Veith2017), in order to better understand the effects of infections on population dynamics, which is essential for the conservation and the management of susceptible amphibian species (Briggs et al., Reference Briggs, Knapp and Vredenburg2010; Muths et al., Reference Muths, Scherer and Pilliod2011; Newell et al., Reference Newell, Goldingay and Brooks2013). The combination of mark-recapture studies and modelling analyses has been successfully used to assess under field conditions the demographic responses to infection by B. dendrobatidis in natural amphibian populations susceptible to such infection. It has been shown that if some individuals of naïve amphibian populations survive the initial chytridiomycosis epidemic, B. dendrobatidis may remain in the area to cause an endemic infection (McDonald et al., Reference McDonald, Mendez, Muller, Freeman and Speare2005; Murray et al., Reference Murray, Skerratt, Speare and McCallum2009; Briggs et al., Reference Briggs, Knapp and Vredenburg2010). The effects of enzootic B. dendrobatidis have been variable. Some amphibian populations showed stability and disease recovery (Retallick et al., Reference Retallick, McCallum and Speare2004; Puschendorf et al., Reference Puschendorf, Hoskin, Cashins, McDonald, Skerratt, Vanderwal and Alford2011; Tobler et al., Reference Tobler, Borgula and Schmidt2012; Newell et al., Reference Newell, Goldingay and Brooks2013), while others continued to be threatened by endemic chytridiomycosis despite host–pathogen coexistence for multiple host generations (Murray et al., Reference Murray, Skerratt, Speare and McCallum2009; Pilliod et al., Reference Pilliod, Muths, Scherer, Bartelt, Corn, Hossack, Lambert, Mccaffery and Gaughan2010; Phillott et al., Reference Phillott, Grogan, Cashins, McDonald, Berger and Skerratt2013; Scheele et al., Reference Scheele, Hunter, Skerratt, Brannelly and Driscoll2015). Several authors have argued that infection outcome, including chytridiomycosis, depends on physiological responses that must be considered within the context of host-specific factors, including life history, ecology, behaviour and physiology, and environmental conditions in which host and pathogen interact (Råberg et al., Reference Råberg, Graham and Read2009; Blaustein et al., Reference Blaustein, Gervasi, Johnson, Hoverman, Belden, Bradley and Xie2012; Murone et al., Reference Murone, DeMarchi and Venesky2016; Greenberg et al., Reference Greenberg, Palen and Mooers2017).
Here, a monitoring of Amphibiocystidium infection was carried out in a natural population of the Italian stream frog (Rana italica Dubios, 1987) of Central Italy, in which parasitism by Amphibiocystidium sp. was first detected in April–May 2007, during occasional field trips (Rossi et al., Reference Rossi, Barocco, Paracucchi, Di Rosa, Carafa, Di Francesco, Di Tizio and Pellegrini2008). On those survey occasions, the parasite was determined by clinical and histopathological examinations of the cutaneous lesions of infected specimens. The parasite prevalence could not be inferred since frogs had not been marked and multiple captures of the same individuals might have occurred. The site was re-visited over a 7-year period (2008–2014), in order to survey the presence of Amphibiocystidium sp. infection in the R. italica population. To improve the parasite characterization and to explore its affinities with respect to previously described Dermocystida species, light and electron microscope examinations were conducted and partial 18S rDNA sequences were analysed. Moreover, a capture-mark-recapture (CMR) study was carried out in an effort to obtain data on population size and to investigate the effects of amphibiocystidiosis at the population level.
Materials and methods
Study site
The current study was conducted along a single 350 m-long transect located in the upper part of the hydrographical system of the Nestore River (Umbria, Central Italy; 42°56′N, 12°03′E, 450 m a.s.l.). This area is characterized by high-quality environmental conditions and is partially included in the Site of Community Importance IT5210040 (‘Boschi dell'Alta Valle del Nestore’) (SCI; see European Commission Habitats Directive, 92/43/EEC) which is one of the largest and best conserved forests of Quercus cerris in Umbria (Rossi et al., Reference Rossi, Barocco, Paracucchi, Di Rosa, Carafa, Di Francesco, Di Tizio and Pellegrini2008).
Study species and sampling
Rana italica is an endemic anuran species of the Italian Peninsula found at an elevation ranging between 30 and 1400 m a.s.l. and mainly distributed along the Apennine chain. It is predominantly a diurnal species usually inhabiting fast flowing streams with a rocky substrate, but it may be found in small ponds, mountain peat bogs and in some man-made habitats such as water troughs (Andreone et al., Reference Andreone, Corti, Sindaco and Romano2009). Depending on the altitudinal and microclimatic conditions, both males and females can be active for most of the year, remaining near stream pools, or may interrupt their activity during the colder months (Sindaco, Reference Sindaco2016). Altitude also affects the reproductive activity period that occurs from late January to early May (Buono et al., Reference Buono, Guarino and Vignoli2014). Italian stream frog is listed on Annex IV of the EU Habitats Directive 92/43/EEC, and on Appendix II of the Bern Convention and it is protected by national legislation (Andreone et al., Reference Andreone, Corti, Sindaco and Romano2009).
In this study, a R. italica population in Nestore River was surveyed from March 2008 to June 2014. A first sampling programme included multiple capture sessions along an entire calendar year (March 2008–February 2009), twice a season, in order to observe the presence/absence of macroscopic cutaneous lesions and to assess the frog sighting rates. Based on the results obtained, it was decided to consider only spring and summer seasons for the following surveys, carrying out a monthly sampling from April to July for each year. Each sampling occasion was carried out in daytime by using the standardized spatiotemporal-dependent method according to Heyer et al. (Reference Heyer, Donnelly, McDiarmid, Hayek and Foster1994). In particular, two operators each time walked for 2 h and 15 min along the same 350 m-long transect, searching for adult specimens of R. italica. The frogs were captured by hand using disposable latex gloves, and were placed in plastic containers with stream water to be transported to the laboratory. Instruments were disinfected with bleach before every survey.
In order to verify successful breeding events, presence or absence of tadpoles was recorded in each sampling occasion, using visual survey (Heyer et al., Reference Heyer, Donnelly, McDiarmid, Hayek and Foster1994; Sindaco, Reference Sindaco2016).
Laboratory work
The captured frogs were carefully examined under stereomicroscope for the presence of skin lesions by Amphibiocystidium sp., and uninfected and infected specimens were kept separately in terrarium–aquarium setups and maintained in captivity for about 10 days. All R. italica individuals were sexed and measured from snout-to-vent and for body mass. When caught for the first time, the frogs were marked by toe clipping using the coding system proposed by Hero (Reference Hero1989). This technique apparently does not affect frog survival (Buono et al., Reference Buono, Guarino and Vignoli2014). Only data from adults were considered. Skin biopsies from cystic lesions of the infected frogs were taken for histological diagnostics. All these procedures were carried out under general anaesthesia with a bath of 0.1% buffered tricaine methanesulfonate (MS-222; Sigma-Aldrich, St. Louis, MO, USA). A systematic post-mortem examination was conducted on captivity dead frogs. All the other individuals were released at the capture site after the biopsy procedures.
The infected skin biopsies were processed as follows. For light microscopy, they were fixed in 4% paraformaldehyde at 4 °C, and processed routinely for embedding in paraffin wax. Five μm-thick sections were stained with haematoxylin and eosin and observed for histopathological analysis. For transmission electron microscopy (TEM), skin biopsies were fixed in 2.5% glutaraldehyde in cacodylate buffer, post-fixed in 1% osmium tetroxide, dehydrated in graded ethanol and embedded in Epon-Araldite resin (Sigma-Aldrich, St. Louis, MO, USA). Ultrathin sections were counterstained with uranyl acetate and lead citrate and examined using a Philips 400 TEM (Eindhoven, The Netherlands) at 60 kV.
To study the phylogenetic affinities of the parasite from R. italica, genomic DNA was examined that was extracted from skin biopsies embedded in paraffin for histological analysis. Twenty μm sections were dewaxed with xylene, centrifuged at high speed and the pellet was washed with 95% and 70% ethanol. Tissues were then dried and the genomic DNA was extracted following the protocol of Wizard Genomic DNA Purification Kit (Promega, Madison, WI, USA). To amplify the partial 18s rRNA gene, NS1 and NS8 universal primers were used (Gargas and DePriest, 1996) in a TProfessional Basic Gradient Thermocycler (Whatman Biometra, Göttingen, Germany) with AccuSure DNA polymerase (Bioline, London, UK). The progression was as follows: an activation step at 95 °C for 7 min, 40 cycles of denaturing at 94 °C for 1 min, annealing at 50 °C for 30 s, an extension at 68 °C for 4 min and a final extension at 72 °C for 7 min. With a Concert Rapid Gel Extraction System (Invitrogen, Carlsbad, CA, USA), the resultant products were gel-purified (expected size of 1780 base pairs) and used as templates for another amplification under the same conditions. For this step, the NS8 reverse primer was combined with a forward primer specific to the Mesomycetozoea (Feldman et al., Reference Feldman, Wimsatt and Green2005). The resultant blunt-ended 1800-base-pair amplicons were gel-purified and a 3′-A overhang addition was carried out by incubating them with DyNAzymeTM II DNA Polymerase (Finnzymes, Espoo, Finland). Finally, using a pGEM-T Easy Vector (Promega, Madison, WI, USA), the products were cloned to generate the replicates for sequencing. The clones were submitted to BMR Genomics (Padova, IT) for sequencing in both directions. The obtained sequence was then aligned with the 27 homologous ones currently available in GenBank from genera and species in the order Dermocystida. For the multiple sequence alignment, the MAFFT 6 software with the Q-INS-i option was used (Katoh and Toh, Reference Katoh and Toh2008). Ambiguously aligned positions were removed using GBLOCKS 0.91b with the following parameter settings: minimum number of sequences for conserved and flank positions = 15; maximum number of contiguous non-conserved positions = 8; minimum block length = 8; and allowed gap positions = half (Castresana, Reference Castresana2000). To identify the best model of sequence evolution, the Akaike Information Criterion was used as implemented with Modeltest 3.6 software (Posada and Crandall, Reference Posada and Crandall1998). This analysis indicated TrN + I + Γ as the best-fit model for the data, with Γ = 0.632, I = 0.659 and unequal base frequencies (A = 0.275, C = 0.185, G = 0.256 and T = 0.284). With this model and PAUP* 4.0b10 software (Swofford, Reference Swofford2003), the pairwise divergences and per cent identities were calculated, and the phylogenetic trees were constructed, using maximum likelihood and neighbour-joining algorithms.
Statistical analysis
The prevalence of Amphibiocystidium infection was calculated as the proportion of individuals found to be positive. Exact version of χ 2 test was used to analyse if the Amphibiocystidium prevalence was associated with the year of sampling. At the same time, a χ 2 test was used to compare the differences in the proportions between the captive dead frogs and the captive survived ones. Differences in weight (dependent variable) between uninfected and infected frogs were investigated via a two-way ANOVA, including sex as an independent variable. The significant level of analyses was set at 0.05. Finally, the simplest model in program MARK (White and Burnham, Reference White and Burnham1999) with POPAN parametrization was used to estimate apparent survival (φ), capture probability (p) and population size (N) by setting constant values (.) for these parameters in order to analyse the effect of Amphibiocystidium infection on Italian stream frog population demography. Analyses were performed in the statistical framework R (R Core Team, 2018) with the support of the package RMark (Laake, Reference Laake2013).
Results
Clinical features and histopathology of Amphibiocystidium infection
Over a 7-year period, 126 adult Italian stream frogs (R. italica) were captured at the Nestore River during visits between March 2008 and June 2014. Reproduction occurred each year, since tadpoles were observed every year. Of the sampled individuals, 67 had massive numbers of externally visible skin lesions usually with whitish hemispherical swellings, suggestive of Amphibiocystidium infection. The lesions occurred mostly on the dorsal and ventral surfaces of the host's limbs, torso and head, including the eyelids and palpebral conjunctivae, as well as in the oral cavity and on the pharyngeal ends of the eustachian tubes. They often formed large clusters, mainly near the vent (Fig. 1A–C). On histopathological examination, the swellings revealed parasitic cysts shaped like a short bent cylinder (<100 µm in diameter) and/or an elongated U-shaped cylinder (100–500 µm in diameter), containing hundreds of encapsulated endospores. The cysts developed in the host dermis and were embedded in a host-produced fibrous granuloma-like connective tissue with high cellular density, including fibroblasts, macrophages and a considerable amount of inflammatory eosinophil infiltrate (Fig. 1D). Electron microscopic examination of the U-shaped cysts showed a cyst wall (1–3 µm thick) composed of an electron-dense layer of homogeneous material to which host connective tissue cells and host phagocytes were attached. These cells aligned themselves with their long axis parallel to the cyst wall (Fig. 2A). The organization of the internal mature endospore (6–10 µm in diameter) showed a nucleus that was displaced to a peripheral position by a central, globular osmiophilic inclusion body and mitochondria-like structures (Fig. 2B).
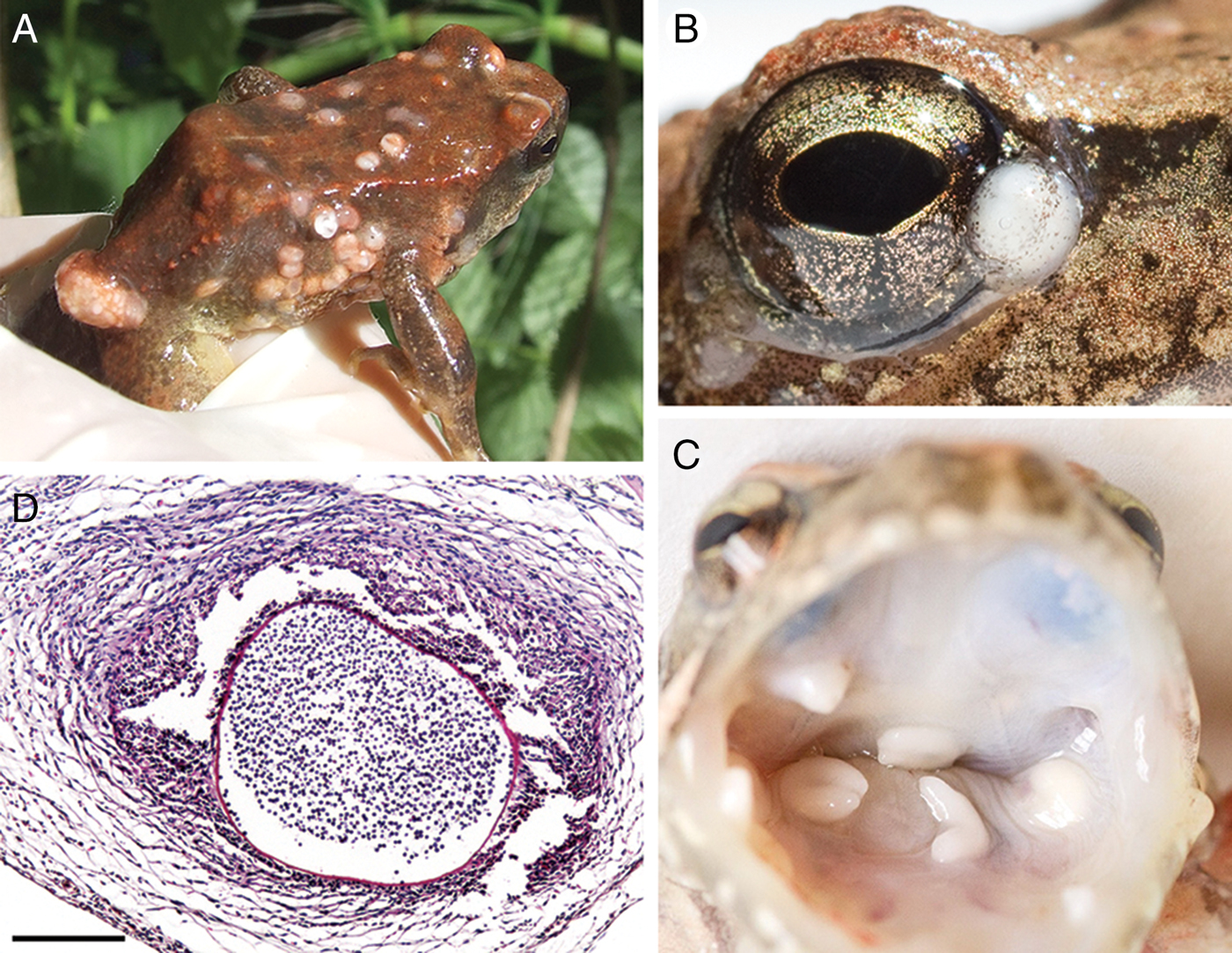
Fig. 1. Amphibiocystidium skin lesions in the Italian stream frog Rana italica. Whitish hemispherical elevations are present on the dorsal and ventral surfaces of frog limbs, torso and head (A), in the eyelids and palpebral conjunctivae (B) and in the oral cavity (C). When dissected, the lesions appear as parasitic cysts containing numerous spherical spores that are embedded in a host-produced fibrous connective tissue with high cellular density (D). Scale bar = 200 µm.

Fig. 2. Amphibiocystidium parasitic cyst. Fibroblasts (f) and an eosinophil (e) with their long axes parallel to the cyst wall (arrow) are evident around the cyst (A). The mature spore displays a peripheral nucleus (n), an inclusion body (b) and a mitochondrion (m) (B). Scale bar = 5 µm (A); 2 µm (B).
During the captivity period, events of mortality were recorded among both uninfected and infected frogs. No evidence of parasite cysts was found in any of the internal organs of the dead animals.
Genetics
The 18S small-subunit rRNA sequence isolated from R. italica parasite was 1720 bp in length (GenBank accession number EU650666). A BLAST search (http://www.ncbi.nlm.nih.gov/blast/Blast.cgi) confirmed that the parasite was closely related to all Dermocystida members in the Mesomycetozoea. After the removal of ambiguously aligned positions with GBLOCKS, the final sequence alignment with all the 18S rRNA sequences from representatives of the order Dermocystida included 1667 of the 1777 positions resulting from the alignment with MAFFT 6 (i.e. 93% of positions retained) (EMBL-Align database accession number: ALIGN_001240). The 1720-base-pair sequences matched a 778-base-pair fragment from A. viridescens (EF493030), and differed in 1.0–8.6% of aligned positions from the other sequences corresponding to Dermocystida members, including the amphibian parasites A. ranae (AY550245 and AY692319) and Amphibiothecum penneri (AY772000) (Fig. 3). Phylogenetic analyses were also carried out (using maximum-likelihood and maximum-parsimony approaches) to further characterize the genetic affinities of this pathogen. Since the phylogenetic trees were congruent with those previously reported by Raffel et al. (Reference Raffel, Bommarito, Barry, Witiak and Shackleton2008) for A. viridescens, they will not be discussed here.

Fig. 3. Affinities of Amphibiocystidium sp. from the Italian stream frog Rana italica. The 1720-base-pair segment of the protist's 18s rRNA gene (EU650666) matches a 778-base-pair fragment from A. viridescens (EF493030), and differs in 1.0–8.6% of the aligned positions from A. ranae (AY550245 and AY692319) and Amphibiothecum penneri (AY772000). Positions of the 778-base-pair fragment that vary among the 28 sequences are shown. Dots indicate nucleotides that match those of the isolate from R. italica. Hyphens indicate gaps. For the full alignment, see the EMBL-Align database (ALIGN_001240).
Effects of Amphibiocystidium infection
The prevalence of Amphibiocystidium infection was found to be significantly associated with year of sampling (χ 2 = 20, P = 0.02), with higher prevalence in 2011 and 2013 (75 and 69%, respectively) (Fig. 4) and an average value in the period of 49.2 ± 17.7%. It was found that the ratio between captive dead frogs and survived frogs was not dependent on the infection (χ 2 = 0.1, P = 0.7). In both males and females, data on body mass were similar between uninfected and infected frogs (ANOVA F = 1.06, P > 0.05) and the analysis revealed that there was no interaction effect between sex and infection (F = 2.13, P = 0.147).

Fig. 4. Correlation between prevalence of Amphibiocystidium infection and year of sampling. Disease prevalence varies significantly with the years, peaking at 75 and 69% in 2011 and 2013, respectively. Dotted line represents the mean value.
Analysis with the POPAN design showed that the estimated population sizes (N ± s.e.) were 495 ± 169 for uninfected frogs and 559 ± 193 for infected frogs; apparent daily survival probabilities (φ ± s.e.) were 0.957 ± 0.012 for uninfected frogs and 0.963 ± 0.011 for infected frogs; capture probabilities (P ± s.e.) were 0.463 ± 0.212 for uninfected frogs and 0.385 ± 0.169 for infected frogs.
Discussion
To date, most reports of dermocystid mesomycetozoean infections in Europe have been primarily found in caudates (González-Hernández et al., Reference González-Hernández, Denoël, Duffus, Garner, Cunningham and Acevedo-Whitehouse2010; Garner et al., Reference Garner, Martel, Bielby, Bosch, Anderson, Meredith, Cunningham, Fisher, Henk, Pasmans, Heatwole and Wilkinson2013; Fiegna et al., Reference Fiegna, Clarke, Shaw, Baily, Clare, Gray, Garner and Meredith2017), while similar data from amphibian anurans were obtained only from Pool frog (P. lessonae) of Central Italy (Pascolini et al., Reference Pascolini, Daszak, Cunningham, Tei, Vagnetti, Bucci, Fagotti and Di Rosa2003). In the present study, evidence of outbreaks of Amphibiocystidium sp. infection in a population of the Italian stream frog (R. italica) of Central Italy is reported.
The histological and histopathological features of the parasite resemble those of other members of Dermocystida. In particular, the curved cylindrical and U-shaped cysts, and the presence of endospores with a single, large inclusion body, resemble the characteristics of A. ranae found on Rana temporaria in Switzerland and Czechoslovakia (Guyenot and Naville, 1922; Broz and Privora, Reference Broz and Privora1952) and on Rana esculenta in France (Remy, Reference Remy1931). At the ultrastructural level, the relationship between intact U-shaped cysts and host phagocytes is consistent with that described for A. ranae infecting R. temporaria (Broz and Privora, Reference Broz and Privora1952). Interestingly, the Amphibiocystidium sp. cysts were found for the first time on the palpebral conjunctiva and on the inner end of the eustachian tubes, which are typical locations of lesions associated with the related parasite Rhinosporidium spp. found in humans, other mammals and birds (Arseculeratne, Reference Arseculeratne2002; Mendoza et al., Reference Mendoza, Taylor and Ajello2002).
The parasite from R. italica also showed genetic affinities with dermocystid mesomycetozoeans, revealing 100% sequence identity with A. viridescens from Eastern red-spotted newt (N. viridescens) (Raffel et al., Reference Raffel, Bommarito, Barry, Witiak and Shackleton2008). The most parsimonious explanation of this last finding is that the described pathogen from North America is the same as the one found in R. italica from Central Italy. This genetic match across continents is common in other microbial species, such as bacteria, protists, fungi and microscopic animals, supporting the ‘moderate-endemicity’ model of microbial biogeography (Bass et al., Reference Bass, Richards, Matthai, Marsh and Cavalier-Smith2007; Foissner, Reference Foissner2008; Fontaneto et al., Reference Fontaneto, Barraclough, Chen, Ricci and Herniou2008). This model proposes that microbial species often inhabit widely separated regions, such as different continents, because of unassisted widespread dispersal. Since strong genetic similarity of microbial species on different continents does not necessarily imply human transport, it remains unclear whether A. viridescens was endemic to a particular region before human intervention began or is naturally widespread. However, the fact that Amphibiocystidium infection has been found in two different host orders (Anura and Caudata) confirms the generalist nature of the parasite. This acknowledged characteristic of mesomycetozoeans, along with other biological features, contributes to the important role they may play in being responsible for emerging infectious diseases in amphibian (Glocking et al., Reference Glocking, Marshall and Gleason2013; Rowley et al., Reference Rowley, Gleason, Andreou, Marshall, Lilje and Gozlan2013).
The results of this study provide preliminary information regarding the possible effects of amphibiocystidiosis long occurrence on the dynamics of the surveyed R. italica population. Since no population demographic data were gathered before the observed emergence of Amphibiocystidium infection, it is not known if the population previously experienced range contractions following epizootic amphibiocystidiosis. However, the estimated population size over the 7-year survey period did not significantly differ between uninfected and infected frogs, and none of uninfected individuals was later recaptured as infected. The daily survival probability values were high and comparable in both uninfected and infected frogs, and were not influenced by capture estimates, even though these values were slightly higher in uninfected frogs. Males and females became ill in like manner, and the infection did not result in changes in body mass of either gender. During the captivity period, the ratio between survived frogs and dead frogs did not seem to depend on the infection. All these data highlight the absence of negative effects of amphibiocystidiosis on the population trend of R. italica throughout the sampling period, despite the fact that high estimates of disease prevalence were obtained.
In general, disease prevalence is known to depend mainly on two variables: the incidence, i.e. the number of new infections, and the duration of the disease, involving events of recovery and/or mortality. These epidemiological parameters can be altered in opposite ways by two defence mechanisms, resistance and tolerance. Resistance strategies prevent the spread of infection by reducing the number of newly infected hosts and the duration of infection, thereby limiting growth and reproduction of the parasite within the host. Tolerance traits prolong the survival of infected hosts without effects on parasite burden by reducing the negative fitness consequences of infectious diseases. This increases infection prevalence while having a neutral or even positive effect on parasite virulence (Roy and Kirchner, Reference Roy and Kirchner2000; Read et al., Reference Read, Graham and Råberg2008; Schneider and Ayres, Reference Schneider and Ayres2008; Råberg et al., Reference Råberg, Graham and Read2009; Horns and Hood, Reference Horns and Hood2012; Medzhitov et al., Reference Medzhitov, Schneider and Soares2012).
Similar to what has been suggested for the amphibian B. dendrobatidis coexistence (Lips, Reference Lips2016), the long persistence of Amphibiocystidium infection in the Nestore system, at least since 2007, may have allowed Italian stream frogs to develop resistance and/or tolerance against amphibiocystidiosis. Resistance strategies could also be evoked with regard to cutaneous microbiota, thought to play a role in preventing/protecting R. italica against the Amphibiocystidium infection (Federici et al., Reference Federici, Rossi, Fidati, Paracucchi, Scargetta, Montalbani, Franzetti, La Porta, Fagotti, Simoncelli, Cenci and Di Rosa2015). Nevertheless, the prevalence estimates of infection observed during the 7-year study period exclude a protection mechanism similar to herd immunity that allowed the frog coexistence with the parasite. This was probably due to the lack of a sufficient proportion of individual frogs with protective anti-Amphibiocystidium bacteria (Lam et al., Reference Lam, Walke, Vredenburg and Harris2010). A tolerance strategy, on the other hand, would have reduced frog vulnerability to harm caused by parasite infection, prolonging their survival but not inhibiting the parasite transmission process. Some signs of how tolerance could have mitigated the impact of infection on population fitness were observed, such as the constant occurrence of reproductive activity, revealed by the presence of tadpoles every year during the study period (Sindaco, Reference Sindaco2016). Moreover, the absence of changes in body mass in both infected male and female individuals was used as a proxy for frog tolerance to infection, since in anurans mass is considered a good predictor of health (Reading, Reference Reading2007; Earl and Whiteman, Reference Earl and Whiteman2015; Murone et al., Reference Murone, DeMarchi and Venesky2016). Other signs of tolerance traits in the frogs could be seen in an increased prevalence of infection, reaching almost epidemic levels in some sampling years. In those cases, the disease incidence increased and the infected frogs eliminated the infection after a longer time than previously. A disease triangle (host–pathogen–environment interactions) has often been considered the key for explaining disease outcome (Scholthof, Reference Scholthof2007; James et al., Reference James, Toledo, Rödder, da Silva Leite, Belasen, Betancourt-Román, Jenkinson, Soto-Azat, Lambertini, Longo, Ruggeri, Collins, Burrowes, Lips, Zamudio and Longcore2015; Lips, Reference Lips2016; Greenberg et al., Reference Greenberg, Palen and Mooers2017; Scheele et al., Reference Scheele, Skerratt, Grogan, Hunter, Clemann, McFadden, Newell, Hoskin, Gillespie, Heard, Brannelly, Roberts and Berger2017). The trend observed in this study could have been promoted by changes in the environmental context in which host–parasite interaction favoured both the rate of infection transmission and the spread of the parasite, increasing its ability to reproduce, but without causing the loss of individuals. Pathogen virulence is also a function of host tolerance and it decreases when host tolerance to damage caused by the pathogen increases (Medzhitov et al., Reference Medzhitov, Schneider and Soares2012). However, signs of a combination of resistance and tolerance traits were also detected, namely immune-driven resistance promoted the activation of tissue-damage control mechanisms that established tolerance to infection (Soares et al., Reference Soares, Teixeira and Moita2017). The formation of the granuloma-like structures around Amphibiocystidium cysts could have been an adaptive immunity mechanism that, by ‘walling off’ the parasite, the host tissues were protected and tolerant to the presence of the persistent parasite (Allen and Wynn, Reference Allen and Wynn2011). A combined mechanism of resistance and tolerance traits that limited tissue damage has also been reported in Pseudacris regilla infected by B. dendrobatidis and yet persistent (Reeder et al., Reference Reeder, Pessier and Vredenburg2012).
Globally, the results of our study seem to lean towards the hypothesis that in a single apparently stable population of R. italica amphibiocystidiosis is an ongoing endemic/epidemic infection that has not exerted negative effects on individual survival and population fitness. However, since information about size and dynamics of the examined population prior to this study is not available, it cannot be excluded that in other natural R. italica populations an outbreak of Amphibiocystidium infection could exert negative effects that could lead to severe frog declines. Resistance and tolerance strategies to diseases must be considered within a context that includes multiple ecological stressors and host-specific factors that interact in a synergistic fashion (Blaustein et al., Reference Blaustein, Gervasi, Johnson, Hoverman, Belden, Bradley and Xie2012). Factors such as habitat destruction, invasive species, climate change and environmental contaminants can interact in complex ways which affect amphibian susceptibility to diseases (Blaustein et al., Reference Blaustein, Gervasi, Johnson, Hoverman, Belden, Bradley and Xie2012). Moreover, variations in susceptibility, resistance and tolerance to B. dendrobatidis infection among amphibian species have also been attributed to species-specific immunogenomic architecture (Ellison et al., Reference Ellison, Tunstall, Direnzo, Hughey, Rebollar, Belden, Harris, Ibáñez, Lips and Zamudio2014). These laboratory data have been confirmed by analysing population genetic composition under natural environmental conditions. Environment and genetics predicted infection prevalence, and genetic diversity alone predicted mortality following chytridiomycosis outbreaks (Savage et al., Reference Savage, Becker and Zamudio2015). It is possible that both environmental and genetic factors may drive variations in susceptibility to Amphibiocystidium infection in conspecific populations of R. italica. It would therefore be of interest to extend the analyses to other contexts in order to test the generality or the specificity of these results, considering that the intraspecific genetic diversity of R. italica populations of Italian peninsula has been shown (Canestrelli et al., Reference Canestrelli, Cimmaruta and Nascetti2008). Ecological and host traits could also account for interaction variability between Amphibiocystidium and other amphibian species, as observed in the negative correlation between amphibiocystidiosis and R. italica mortality and the positive correlation reported for both captive T. cristatus (Moral, Reference Moral1913) and N. viridescens (Raffel et al., Reference Raffel, Bommarito, Barry, Witiak and Shackleton2008). Finally, the possible spread of frog tolerance could permanently increase the incidence of infection, since tolerance prolongs the survival of infected hosts but also of parasites (Roy and Kirchner, Reference Roy and Kirchner2000). Considering the generalist nature of the parasite, tolerant Italian stream frogs could be a reservoir species for highly susceptible, non-tolerant amphibian species (Fisher et al., Reference Fisher, Henk, Briggs, Brownstein, Madoff, McCraw and Gurr2012).
Author ORCIDs
Daniele Canestrelli, 0000-0001-9351-4972; Gianandrea La Porta, 0000-0002-6465-5423; Ines Di Rosa, 0000-0002-8881-4361
Supplementary material
The supplementary material for this article can be found at https://doi.org/10.1017/S0031182019000076.
Acknowledgements
We thank R. Barocco for supplying Fig. 1A, and Sister Nancy Hutchinson for critical reading of the manuscript. The study was authorized by the Italian Ministry for Environment, Land and Sea Protection (0011282/DPN of 25/05/2009 and 0014170 of 13/07/2012) and by the Italian Ministry of Health (34/2003-A and 46/2003-B of 20/03/2003 and 28/03/2003).
Financial support
This work was supported by the Fondazione Cassa di Risparmio di Perugia (grant number 2009.010.0476).
Conflict of interest
None.
Ethical standards
The frog capture and manipulation were carried out following the recommendations provided by the Italian Ministry for Environment, Land and Sea Protection and by the Italian Ministry of Health.