INTRODUCTION
Gastrointestinal parasites are ubiquitous throughout ruminant species and have been widely documented as having negative subclinical effects on the condition of domestic livestock (Fox, Reference Fox1997). In comparison, the impact of parasites on wild species has been comparatively poorly studied because of the perception that long periods of co-evolution has resulted in innocuous host parasite relationships and the logistical difficulties of research on free-ranging populations (Gulland, Reference Gulland, Grenfell and Dobson1995). However, accumulating empirical evidence suggests that they can affect the condition, fecundity, survival or grazing behaviour of the hosts (Gunn and Irvine, Reference Gunn and Irvine2003; Irvine, Reference Irvine2006), and theory predicts that these effects may be sufficient to regulate population dynamics (Anderson and May, Reference Anderson and May1978; May and Anderson, Reference May and Anderson1978).
One of the most detailed studies of the impact of parasites on a wild ruminant species has been carried out on the Svalbard reindeer (Rangifer tarandus platyrhynchus) and their gastrointestinal parasites. Experimental and observational work showed that two nematode species, Marshallagia marshalli and Ostertagia gruehneri, dominate the gastrointestinal fauna (Dallas et al. Reference Dallas, Irvine and Halvorsen2000), with the latter species affecting host condition (Stien et al. Reference Stien, Irvine, Ropstad, Halvorsen, Langvatn and Albon2002), and having the potential to regulate the host population through its impact on fecundity (Albon et al. Reference Albon, Stien, Irvine, Langvatn, Ropstad and Halvorsen2002). A similar gastrointestinal nematode parasite fauna is present in caribou and other ungulate herbivores across North America (Hoberg et al. Reference Hoberg, Kocan, Rickard, Samuel, Pybus and Kocan2001) suggesting that these impacts may be more widespread. Further research on caribou and reindeer populations has shown there to be strong effects of parasitic oestrid flies on the hosts ecology. Warble flies (Hypoderma tarandi) and nose bot flies (Cephenemyia trompe), can have negative impacts on foraging, herding behaviour and movement during summer (Folstad et al. Reference Folstad, Nilssen, Halvorsen and Andersen1991; Hagemoen and Reimers, Reference Hagemoen and Reimers2002; Fauchald et al. Reference Fauchald, Rodven, Bardsen, Langeland, Tveraa, Yoccoz and Ims2007).
In general, caribou build up body reserves during summer before losing body fat and catabolizing protein (muscle) reserves during winter when food availability is restricted (Miller, Reference Miller, Feldhammer, Thompson and Chapman2003). Parasites may have severe detrimental effects on survival and reproduction in caribou by affecting nutrient assimilation and, if sufficient reserves have not been accumulated, caribou may fail to breed in the autumn (Thomas, Reference Thomas1982) or potentially risk winter starvation (Bergerud, Reference Bergerud1996; Gerhart et al. Reference Gerhart, White, Cameron and Russell1996). However, previous research into parasitism in caribou has been limited, with most data collected from opportunistic sampling of single animals (Kutz et al. Reference Kutz, Hoberg, Nagy, Polley and Elkin2004). Studies on the impacts of parasites on caribou ecology remain sparse.
This study is one of the first to investigate the impact of gastrointestinal parasites, specifically abomasal nematodes, on caribou condition and discuss their potential influence on their ecology. Our study host population, the Dolphin-Union (DU) caribou herd of Victoria Island, Nunavut, Canada, is of particular interest for at least two reasons. Firstly, in comparison to the Svalbard reindeer the DU caribou undergo a long-distance annual migration between the centre of Victoria Island and the Canadian mainland. The seasonal migrations may have significant consequences for the transmission of parasites and their role in caribou ecology.
Secondly the DU caribou underwent an apparent winter range shift around 3 decades ago. During the 19th and early 20th centuries the herd was documented as moving south, crossing the North West Passage from Victoria Island to the mainland to winter, returning again in spring (Manning, Reference Manning1960). By the mid-1920's numbers of observations of caribou on the south of the island, or crossing the ice, had declined, and the herd was thought to have gone extinct (Manning, Reference Manning1960). However, in the 1970's new reports of caribou on the south of the Victoria Island suggested the population had survived but had been remaining for winter on the island (Gunn, Reference Gunn and Harrington1990). Subsequent surveys indicated that caribou numbers were increasing and, additionally, that the herd had resumed the historic winter migration to the mainland (Gunn et al. Reference Gunn, Buchan, Fournier and Nishi1997). This most recent change in migration pattern occurred when both caribou and muskox numbers were thought to be increasing (Jingfors, Reference Jingfors1984; Gunn, Reference Gunn and Harrington1990). Although the change in migration may have been induced by forage limitation (Hughes, unpublished data), parasite avoidance is a possible cause of host migration (Folstad et al. Reference Folstad, Nilssen, Halvorsen and Andersen1991; Alerstam et al. Reference Alerstam, Hedenstrom and Akesson2003) and loss of condition due to increasing parasitism may have been influential.
In addition, the DU caribou are part of a more complex system than found on Svalbard, as the caribou coexist with a second arctic ruminant, muskox Ovibos moschatus. In comparison to the migrating DU caribou, muskox are sedentary and reside on Victoria Island all year. Muskox occupy the historic DU caribou wintering grounds on the south of the island, utilizing the same vegetation communities as wintering caribou (Hughes, unpublished data). During the summer muskox may potentially increase parasite abundance on these historic winter pastures, where, without muskox, it would normally be expected to be quite low due to the seasonal cycle of parasitic egg production in conjunction with caribou migration. Caribou grazing during winter on tundra that has been contaminated by muskox, may increase their parasite burdens through interspecific transmission of parasites from muskox. This interaction may provide a source of apparent competition between the host species.
This study examines the impact both abomasal parasites and warble flies have on female caribou condition, discussing the results with respect to those found in Svalbard and the implications they may have for the ecology of the caribou. Additional, opportunistic sampling of a small number of muskox abomasums from the same area enables speculation about the possibility of interspecific, parasite-mediated competition and the role parasites may have had in instigating the alteration of migration pattern of the DU caribou herd.
MATERIALS AND METHODS
Study area
In April 2001, 2002 and 2003 DU caribou were sampled from 3 locations between 50 km and 150 km east of Kugluktuk, Nunavut (67°50′N, 115°06′W) on the north coast of mainland Canada. The caribou sampled in the autumn of 2002 and muskox, sampled in summer 2003, were collected from around the Long Point area of Cambridge Bay, Victoria Island, Nunavut (69°06′, 105°27′). Currently DU caribou calving grounds are located in the centre of Victoria Island. Wintering takes place on the Canadian mainland with caribou generally remaining within a couple of hundred kilometres of the north-coast and Bathurst Inlet (Hughes, unpublished data). Winter and summer grounds, on the mainland and Victoria Island respectively, are typically at low elevations (<650 m a.s.l.). The mainland wintering grounds are a patchwork combination of several tundra vegetation communities found within the erect dwarf shrub (east of Bathurst Inlet) and low shrub bioclimatic subzones (Gould et al. Reference Gould, Edlund, Zoltai, Raynolds, Walker and Maier2002). On Victoria Island the vegetation cover is predominantly within the erect dwarf shrub and hemiprostrate dwarf shrub bioclimatic subzones, which constitute a combination of prostrate and erect dwarf-shrub-graminoid tundra (Gould et al. Reference Gould, Edlund, Zoltai, Raynolds, Walker and Maier2002). Annual precipitation is low (annual mean 140 mm) and the region is generally snow covered between late September and early June. Average monthly temperatures, calculated from the years 1971 to 2000, range from −37°C (February) to 8·4°C (July) (Environment Canada 2004 public communication).
Hosts
In total, 82 female caribou from the Dolphin-Union herd were culled on the mainland between 14–16 April, 2001 (n=30), 16–19 April, 2002 (n=22) and 11–12 April, 2003 (n=30) (Table 1). Measurements recorded in the field were (i) body mass as shot; (ii) length from nose to tail; (iii) subcutaneous back fat depth, (measured at the rump by cutting into the carcass), (iv) pregnancy status ascertained by the presence or absence of a foetus and (v) the number of warble larvae present under the skin. Age was later ascertained by analysis of annuli in the cementum of (I1) incisors (Matson's Laboratory, Milltown, MT, USA). Standard weight was recorded as a measure of the whole weight of the carcass without the gastrointestinal tract to reduce variation in mass due to amount of ingested forage. Standard weight and subcutaneous back fat depth were then used as indices of condition. Caribou ranged in age from 1·8 years to 14 years with a mean age of 6·5 years. Standard weight ranged between 38·7 kg and 86·8 kg. Mean standard weight of non-pregnant caribou was 51·9 kg and the mean standard weight of pregnant caribou was 65·6 kg. Only 42% of caribou had any back fat present, with the median depth when present being 6·5 mm. Overall, just over half of the caribou were found to be pregnant with 43%, 55%, and 71% having a foetus in 2001, 2002 and 2003 respectively. In October 2002, 17 caribou abomasums were collected from Victoria Island by working in conjunction with local hunters. In addition, 4 muskox abomasums were collected from routine hunting trips through the local Wildlife Office during the summer 2003 and samples provided nematode species profiles for comparison with caribou. It was not possible to collect any biometric data for these caribou or Muskox apart from hunter-estimated age.
Table 1. Descriptive data for 82 caribou sampled in April 2001, 2002 and 2003

Parasites
Strongylate nematodes were extracted and counted from the abomasum of 76 of the April culled animals. Abomasums were frozen within 1 h of collection until analysis. The abundance of adult males, adult females and larvae in the lumen were estimated using the method described by Halvorsen et al. (Reference Halvorsen, Stien, Irvine, Langvatn and Albon1999), a modification of the standard method from MAFF/ADAS (1986). The abundance of larvae within the mucosal lining of the abomasum was determined from a subset of 38 mucosa from the April samples (2001 n=13; 2002 n=11; 2003 n=14) using an acid-pepsin digest (MAFF/ADAS 1986).
Species profile for the nematodes from a subset of the abomasum samples was based on identification of only adult males (O. Halvorsen and C. Vollelve, Zoologisk Museum, University of Oslo). Samples identified were from the 23 animals culled in April 2001, the 17 October samples and 4 muskox abomasums. This gave an indication of the nematode fauna present in muskox and caribou in late (April) and early (October) winter. Data on the species composition of adult males is presented; however, as male:female ratios may differ between species, this may not reflect the species composition of the total parasite burden.
For comparison with caribou, data on the abundances and species composition of abomasal parasite fauna in Svalbard reindeer were gathered from research carried out by Irvine et al. (Reference Irvine, Stien, Halvorsen, Langvatn and Albon2000) between 1994 and 1998. Abomasal samples were collected in either late summer (between August and October) or late winter (between February and April) in each year. Only data on species profiles in female reindeer were used (October n=112, Feb–April n=72). The Svalbard data were also based on the identification of males and the proportional composition is comparable as they assumed a 1:1 ratio of males:females.
Warble abundance was determined in the field and only for the 3 April samples. The number of encysted warble larvae found under the skin of each caribou was counted by the hunter after skinning the carcass.
Faecal egg counts
In order to look at the potential for pasture loading of parasites by muskox, parasite egg counts from muskox faecal matter were carried out over the summers of 2002 and 2003. None of the faecal samples came from caribou or muskox sampled for abomasal parasites. Fresh muskox faecal samples were obtained by locating muskox, observing defecation and then collecting freshly deposited material. Sampling occurred fortnightly from animals grazing in the study area on southeast Victoria Island. In 2002 faecal samples were collected between 28 July and 19 October. In 2003 samples were collected from 13 June to 28 August 2003. Faecal samples from caribou were also collected in October 2002 on their arrival on the south coast of Victoria Island, and June 2003 as they crossed on to Victoria Island from the mainland. Eggs per gram (epg) faeces was estimated using the modified McMaster flotation technique (MAFF/ADAS, 1986). Each faecal sample was homogenized and 10×3 g samples removed. Each 3 g sample was ground up in 42 ml of saturated salt solution using a sieve and pestle. The solution was then pipetted into a 2 chamber McMaster slide and eggs within both chambers were counted.
Statistical analyses
All analyses were carried out using SAS (SAS v9.1 SAS Institute Inc., Cary, USA). As parasite prevalence was 100% we analysed the intensity of infection and aggregation of parasites (see below). Data on indices of caribou condition were modelled with relation to abomasal parasite burden, in addition to the categorical variables year and pregnancy status (see below). To remove the effect of age on body weight variation all animals under 2 years (n=4) were removed from the data. Among caribou over 2 years old there was no detectable effect of age on weight, back fat depth or pregnancy when fitted as a continuous predictor variable, and also no effect of significant length and weight relationship that may have been necessary to standardize caribou sizes. Therefore there was no need to correct for age or body size in the models. The predictive parasite variables used in models were: lumen parasites (the sum of the estimated abundance of adult and larval stages of parasites found in the abomasal lumen); mucosal larvae (the abundance of parasitic larvae in the abomasal mucosal), and warble abundance. Only individual caribou with a complete data set for all terms being used in analysis were included in models. Separate analyses were therefore carried out when investigating the effect of warble and mucosal larvae abundance as these data were available for fewer samples than those with lumen parasite abundance data.
Parasite distribution
The distribution of warbles and abomasal parasites at each stage, i.e. mucosal larvae, lumen larvae and adult, and for the groupings, i.e. total larvae, total lumen parasites and total abomasal parasites, was investigated. Parasite distribution was modelled with a generalized linear model using a negative binomial distribution (Shaw et al. Reference Shaw, Grenfell and Dobson1998) and log link function. Year, as a categorical variable and age, as a continuous variable, were examined in the model. The aggregation index, k, the dispersion parameter from the negative binomial distribution, was estimated by maximum likelihood methods.
Indices of caribou condition
Standard weight was modelled with a general linear model (SAS v9.1 SAS Institute Inc., Cary, USA), as data were normally distributed, with the significance of terms assessed by a Type 1 test of the F-values. Probability of being pregnant was modelled with a generalised linear model using a binomial distribution with logit link function. Model fit was assessed by deviance values and Chi-square statistic.
Back fat depth was considered censored data bounded at 0·5 mm as the data were being used as an index of condition and, although condition may continue to decrease, negative back fat values are impossible. All zero values were adjusted to 0·5, the upper limit of the 0 mm class, data were then log transformed and modelled using the QLIM (Qualitative and LImited dependent variable Model) procedure (SAS, 2002). When the endogenous variable, in this case back fat depth, is specified as censored the QLIM procedure estimates a standard tobit model to calculate the log-likelihood. To reduce disparity between warble and lumen parasite abundances in this model, the lumen parasite abundance values were log transformed prior to comparison. The difference in log-likelihood from the models was used to compare model fit as each term was added. For the analysis of back fat data in the subset where mucosal larvae data were available, there were insufficient data to estimate parameters in the model with both mucosal larvae and warble abundance included.
RESULTS
Parasites of the Dolphin-Union caribou
There was a large variation in abundance of both warbles and abomasal nematodes in the caribou (Table 2). Although 97% of warble counts were below 250, there were 2 large counts of 428 and 457 in 2001. When modelling different measures of abomasal parasite abundance there was no relationship with caribou age. There was no significant difference between years when modelling the total abomasal parasite abundance, however, abundances of the other stage categories of the abomasal parasites varied between years and between seasons (Fig. 1). Overall, caribou generally carried more larval stage than adult parasites, except in April 2003 when there were a high proportion of adults. During April the majority of larvae were in the lumen, contrasting with the high proportion of larvae found in the mucosa in October. Within season no correlation was found between abundances of any of the nematode stages. As the negative binomial dispersion index, k, is small it shows that the distribution is skewed and that there is a high level of aggregation of both stages of parasites in the population (Table 2).
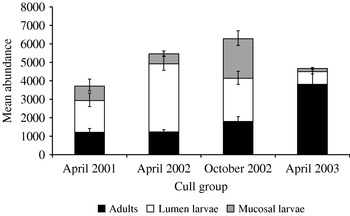
Fig. 1. Abomasal nematodes in the Dolphin-Union Caribou herd. The bars show the mean abundance of nematodes per caribou from the negative binomial distribution model of parasite abundance at each stage (Adults, Lumen Larvae and Mucosal larvae) where the model included a single parameter for sample year and sample sizes varied between groups.
Table 2. Parasite abundance in caribou sampled in April
(Median and ranges are given for counts of warbles and abomasal nematodes per caribou. The abundances of larvae and adults found in the lumen for (a) all samples and (b) the subset with additional mucosal larvae counts recorded. The negative binomial dispersion index, k, was estimated by maximum likelihood.)

Analysis of caribou condition: body weight
There was a significant negative relationship between body mass and worm burden. After correcting for the negative effect of pregnancy status and year, the model fit was significantly improved by adding a term that included lumen parasite abundance and the interaction of lumen parasites with pregnancy status (Table 3a). The negative relationship between weight and parasite abundance was largely in non-pregnant caribou (Fig. 2A; slope, Pregnant=−0·0005 s.e±0·0006, Non-pregnant=−0·0034 s.e.±0·0024). The removal of the 2 outliers (>10 000), decreased the strength of the overall pregnancy relationship, but there was still a significant relationship between parasite abundance which varied between pregnant and non-pregnant caribou (Lumen parasite∗Pregnant, F1,56=4·10, P=0·048).
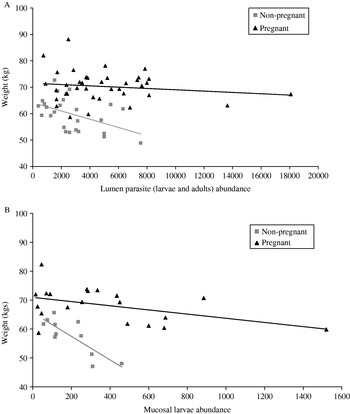
Fig. 2. (A and B). Correlation between caribou standard weight and abundance of parasites in (A) the lumen (N=64) and (B) the mucosa (N=31). Lines show the predicted weight of pregnant and non-pregnant caribou as estimated by the general linear model and points show caribou weights corrected for differences between years.
Table 3. The relationship of body weight and backfat depth to: (a) lumen parasite abundance and (b) mucosal larvae abundance
(The Type 1 test results for sum of squares (SS) or 2∗log likelihood, degrees of freedom (d.f.) and P values are given for significant terms used in the best-fit model (figures in bold). Other terms were added individually to the model, with interactions (denoted by *) added after both first order terms had been included. N indicates the total number of samples available for analysis.)
(a)

(b)

A similar, but stronger, negative relationship was observed between standard weight and the abundance of mucosal larvae. The model fit was improved with the addition of the interaction between larval abundance and pregnancy status (Table 3b). The relationship was again more pronounced in non-pregnant caribou (Fig. 2B; slope, Pregnant=−0·016 s.e.±0·008 Non-Pregnant=0·090 s.e.±0·032) and remained significant even with the removal of the most extreme outlier at (1520, 59·8) (Mucosal larvae+Mucosal larvae∗Pregnant F2,24=4·16, P=0·03).
Analysis of caribou condition: back fat
The depth of back fat was not related to the abundance of abomasal parasites (Table 3a, b). However, there was an overall significant negative relationship between back fat depth and warble abundance after removal of the two outlying warble counts, of 427 and 458 (Table 4). The relationship differed between years: significantly negative in 2001 (Slope –0·037 P=0·013); non-significantly negative in 2003 and positive in 2002. In 2002 caribou were in better condition than other years, only 19% of caribou were recorded as having no back fat present, whereas 64% and 78% had no measurable back fat in 2001 in 2003, respectively.
Table 4. The relationship of body weight and back fat with the abundance of warbles and lumen parasites
(The Type 1 test results for sum of squares (SS) or 2∗log likelihood, degrees of freedom (d.f.) and P value are given for significant terms used (figures in bold) in the best-fit model. Other terms were added individually to the model, with interactions (denoted by *) added after both first order terms had been included. N indicates the total number of samples available for analysis with the 2 highest, outlying warble counts removed.)

Analysis of caribou condition: pregnancy
The probability of being pregnant was significantly related to both lumen parasites and warbles, after controlling for the positive effects of weight and year (Table 5). However, whilst the probability of pregnancy declined with increasing warble abundance (Fig. 3A) it, unexpectedly, increased with increasing lumen parasite abundance (Fig. 3B). Removal of the two samples with the highest parasite burdens did not change either relationship and they were retained in the analysis. In the smaller subset of samples where mucosal larvae counts were available, there was no relationship between the probability of being pregnant with parasites from either the mucosa or lumen, or with warble abundance.
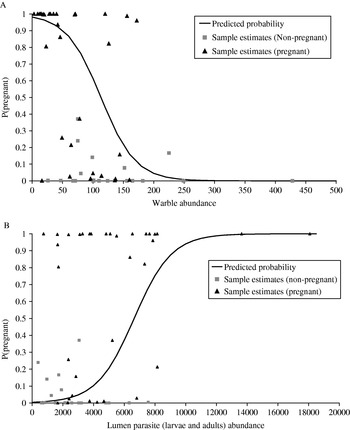
Fig. 3. (A and B) Relationship between parasites and the probability of being pregnant. The ‘Predicted’ lines show model estimates for the probability of caribou being pregnant at increasing (A) warble abundance and (B) lumen parasite abundance. Predicted values were calculated by centring the model around a mean weight of 62·02 kg, mean back fat depth of 0 mm and either, for (A) a mean lumen parasite count of 4161·4 or (B) a mean warble count of 90·5. Sample estimate probabilities were calculated from model parameters.
Table 5. The probability of being pregnant in relation to the condition, expressed as weight or back fat, and parasite burden of caribou
(Significant terms in the model are shown in bold.)

Faecal egg counts
Egg counts sampled from caribou arriving in the area in October 2002 showed very low egg production with only 1 egg found, equivalent to 50 epg faecal matter, in 1 of 14 samples collected over 2 weeks. Parasitic egg output from muskox increased from late June to mid-summer with the peak in density of epg of faeces occurring in mid-August (Fig. 4A). Thereafter egg counts declined towards late September. Prevalence of eggs in samples also fluctuated over this time-period. In late June prevalence of eggs in faecal matter was low at only 20%, but the number of samples containing eggs increased to 80% towards the end of July and into August. During late August and September egg output declined and by mid-October samples showed a prevalence of only 10%.
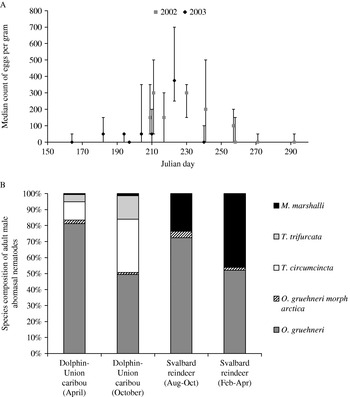
Fig. 4. (A) Counts of strongyle eggs from muskox faeces during 2002 and 2003. Median (geometric mean) eggs per gram of faeces are shown with their ranges. Data come from 3 g samples taken between 28 July and 19 October 2002, and 13 June and 28 August 2003. Days are given in Julian days where July 1=182 Julian days. (B) Species profile of adult male strongylate nematodes found in the abomasums of the caribou (culled in: April, N=22; and October, N=17), and Svalbard reindeer culled in late summer (August–October, N=112) and Late Winter (February–May, N=72).
Identification of abomasal nematodes in DU caribou
Morphometric examination of male nematodes resulted in a number of different species of nematode identified in the Dolphin-Union caribou, Marshallagia marshalli, Ostertagia gruehneri/O. arctica, (Dallas et al. Reference Dallas, Irvine and Halvorsen2000), and Teladorsagia circumcincta, with the associated morph T. trifurcata (Suarez and Cabaret, Reference Suarez and Cabaret1991; Lichtenfels and Hoberg, Reference Lichtenfels and Hoberg1993).
Samples were dominated by the major morph of O. gruehneri (>50% of parasites in 80% of abomasal samples from both seasons) with the T. circumcincta and T. trifurcata the second and third most common in both seasons. There was some evidence for seasonal variation in species profile suggested by the data as the April samples showed a high dominance by O. gruehneri. Comparatively, in the October samples there was a three-fold increase in proportion of T. circumcincta and T. trifurcata (Fig. 4B).
Hoberg et al. (Reference Hoberg, Monsen, Kutz and Blouin1999) previously described the nematode Teladorsagia boreoarcticus from muskox abomasal samples collected in the same region as this study and shed doubt on previous identifications of T. circumcincta morphs from the arctic. Morphometric analysis of our samples was, in most cases, clear and it was not possible to verify the presence of T. boreoarcticus in the current muskox or caribou samples. However, some individuals approached T. boreoarcticus and sequencing may be the only way to ascertain identification definitely. This was not possible in the remit of this study.
Abomasal parasites of Svalbard reindeer
Data from Irvine et al. (Reference Irvine, Stien, Halvorsen, Langvatn and Albon2000) show the mean abundance of adult worms found in female reindeer in late summer (9852±4562 s.d.) and late winter (12 943±5016 s.d.). Three different species of parasite were observed in the Svalbard reindeer: Marshallagia marshalli (both morph marshalli and morph occidentalis); Ostertagia gruehneri (both the major morph gruehneri and minor morph arctica); and Teladorsagia circumcincta (both the major morph circumcincta and minor morph trifurcata). O. gruehneri proportionally dominates the faunal composition in both seasons with M. marshalli being the second major component. Both T. circumcincta and T. trifurcata amount to less than 0·1% of the parasite population (Fig. 4B).
Abomasal parasites of muskox
The 4 muskox abomasums were around 3 times heavier than those sampled from caribou, (mean mucosa weight: caribou 113·6 g s.e.±27·8; muskox, 381·0 g s.e.±127·0), yet the abundance of parasites in the lumen was well within the range found in caribou in April (muskox, median 1764·9, range 863–8391; caribou, Table 2). Two species of nematode were found in the muskox abomasum samples, Marshallagia marshalli and both morphs of T. circumcincta. M. marshalli abundance was low, being found in just 1 sample where it only accounted for 7% of parasites, and Teladorsagia species dominated with T. circumcincta slightly more common (53%) than the minor morph T. trifurcata (45·2%).
DISCUSSION
Our results demonstrate that increasing abomasal nematode and warble burdens correlate with negative subclinical impacts on caribou, suggesting they have the potential to affect their condition and reproduction. These findings are consistent with the observation that parasites may impact the efficiency of nutrient assimilation and consequently may escalate the effects of forage limitation, further reducing host condition and reproduction (Hagemoen and Reimers, Reference Hagemoen and Reimers2002; Stien et al. Reference Stien, Irvine, Ropstad, Halvorsen, Langvatn and Albon2002). Intriguingly, the negative relationships between abomasal parasities and condition in the Dolphin-Union herd were detected at burdens of around one tenth of those observed in the conspecific Svalbard reindeer (Irvine et al. Reference Irvine, Stien, Halvorsen, Langvatn and Albon2000). Experimental work on the Svalbard population established that reducing Ostertagia gruehneri burdens increased reindeer body mass, fat depth and fecundity (Stien et al. Reference Stien, Irvine, Ropstad, Halvorsen, Langvatn and Albon2002). Furthermore, the parasite burden, which is related to host population size 2 years previous, was sufficient to regulate reindeer population growth (Albon et al. Reference Albon, Stien, Irvine, Langvatn, Ropstad and Halvorsen2002). Whether caribou populations may be subject to similar regulatory mechanisms, and whether the caribou-parasite relationship is ecologically similar in the Canadian arctic, requires specific experimental research similar to that undertaken on Svalbard.
Abomasal nematodes may affect ruminant host body condition both directly, through physical damage (Fox, Reference Fox1997), and may indirectly affect condition by depressing food intake (Arneberg et al. Reference Arneberg, Folstad and Karter1996). Mucosal larvae directly affect body weight by damaging the abomasum which reduces appetite and digestive efficiency (Holmes, Reference Holmes1987; Fox, Reference Fox1997). Anthelmintic treatment of reindeer reduces parasite burden and increases body weight (Stien et al. Reference Stien, Irvine, Ropstad, Halvorsen, Langvatn and Albon2002). Our results show a negative correlation between the body mass of non-pregnant caribou, and both mucosal larvae and lumen nematodes, consistent with an impact on nutrient assimilation. The slightly weaker relationship with lumen parasite burden, in comparison to the mucosal larvae, may be a signal of the damage these nematodes inflict on the abomasal mucosa when they emerge to develop into adults. It is possible that the subsequent reduction in efficiency of nutritional uptake may have severe consequences for the survival of individuals during the forage limited winter period, which, for the DU caribou, can last for around 8 months.
In contrast to the non-pregnant caribou, the pregnant caribou showed less weight reduction with increasing parasite burden. This may indicate that they were the higher quality individuals. A similar relationship has been seen in red deer, Cervus elaphus, in Scotland, where the effect of abomasal parasites on the body mass of red deer interacted with pregnancy status (Irvine et al. Reference Irvine, Corbishley, Pilkington and Albon2006). Irvine et al. (Reference Irvine, Corbishley, Pilkington and Albon2006) also found a significant relationship between lactational status, parasite burden and body condition. Lactating hinds had less kidney fat – relative to body size – but it exhibited no relationship with parasite burden, whereas non-lactating hinds showed a significant decline in kidney fat as parasite burden increased. Although we were unable to examine the effects of lactation in our analysis, this is further indication that reproductively successful females may be the ones less prone to the negative effects of parasitism.
The negative relationship between the probability of being pregnant and warble abundance intimates support for the idea that there is a cost of high warble infestations. Previous research has suggested the cost of warbles reflects the harassment during the summer, which reduces time spent feeding (Hagemoen and Reimers, Reference Hagemoen and Reimers2002). With the commensurate reduction in weight gain, this harassment has the potential to affect future reproductive performance (Weladji et al. Reference Weladji, Holand and Almoy2003). However, social status may also influence warble infestation through influencing their position in the herd and their susceptibility to attack (Fauchald et al. Reference Fauchald, Rodven, Bardsen, Langeland, Tveraa, Yoccoz and Ims2007). Poorer individuals, less likely to be reproductively successful anyway, may be more likely to be parasitized by warbles. Further research is required to establish the causal relationship between these factors.
Furthermore, the cause and effect of the positive relationship between the lumen parasite abundance and the probability of being pregnant also requires investigation. That a higher abundance of lumen parasites is more common in pregnant animals may reflect a change in physiological state, such as reduced immune function, enabling greater proliferation of parasites within the abomasum (Wilson et al. Reference Wilson, Bjornstad, Dobson, Merler, Poglayen, Randolph, Read, Skorping, Hudson, Rizzoli, Grenfell, Heesterbeek and Dobson2002). In Svalbard reindeer the majority of O. gruehneri transmission occurs in summer (Irvine et al. Reference Irvine, Stien, Dallas, Halvorsen, Langvatn and Albon2001) before the rut. If the assumption is made that the same seasonality in transmission occurs in the Canadian arctic, the total parasite burdens found in the April samples would have been accumulated the previous summer, prior to the rut. The differences in lumen parasite abundances in pregnant and non-pregnant caribou may therefore be a result of arrested larvae from the mucosa emerging earlier in pregnant caribou. This raises questions about the host physiological changes that would stimulate the larval parasites in pregnant caribou to continue development, and presents the possibility that pregnant and non-pregnant hosts may have interestingly different roles in parasite cycling and population dynamics. Alternatively, if parasite transmission continues to occur throughout winter, as has been seen with M. marshalli (Irvine et al. Reference Irvine, Stien, Dallas, Halvorsen, Langvatn and Albon2001), the relationship could be an indication of differing foraging behaviours that result in pregnant caribou ingesting more larvae than their non-pregnant counterparts after the rut.
Abomasal parasite burdens in the caribou were more similar to those found in reindeer on the Norwegian mainland (Bye, Reference Bye1987) and red deer Cervus elaphus in Scotland (Irvine et al. Reference Irvine, Corbishley, Pilkington and Albon2006) than those found in Svalbard reindeer. The high burdens in Svalbard reindeer may be due to a variety of factors. Svalbard reindeer do not suffer from warble fly harassment and spend a higher percentage of their time feeding than their Norwegian counterparts (Reimers, 1980 in Halvorsen and Bye, Reference Halvorsen and Bye1999) potentially increasing their intake rate of infective larvae from the pasture. Additionally the longer Svalbard summers and lack of long distance seasonal migration (Halvorsen and Bye, Reference Halvorsen and Bye1999) means Svalbard reindeer may be exposed to parasites for longer periods than the caribou, and that greater parasite densities accumulate on the pastures used all year round on Svalbard. Long-distance migration by the caribou would reduce the time spent in any one area and reduce the rate of parasite transmission; moreover flexibility in the migration pattern would enable them to avoid areas of high parasite density (Folstad et al. Reference Folstad, Nilssen, Halvorsen and Andersen1991; Alerstam et al. Reference Alerstam, Hedenstrom and Akesson2003).
At low population levels caribou have been observed to reduce migration ranges, reducing exposure to carnivore predation (Bergerud, Reference Bergerud1996), which potentially explains the proposed reduction in range of the DU caribou in the early 20th century (Gunn, Reference Gunn and Harrington1990). The subsequent resumption of the winter migration to the mainland may have been induced by forage limitation (Hughes, unpublished data) as there are indications that the population was rising again (Gunn, Reference Gunn and Harrington1990) but it is possible that a complex combination of factors may have been influential. For example, other processes that may have played a role in stimulating a change in foraging range include climate change, which may have resulted in a change in vegetation community distribution (Walker et al. Reference Walker, Wahren, Hollister, Henry, Ahlquist, Alatalo, Bret-Harte, Calef, Callaghan, Carroll, Epstein, Jonsdottir, Klein, Magnusson, Molau, Oberbauer, Rewa, Robinson, Shaver, Suding, Thompson, Tolvanen, Totland, Turner, Tweedie, Webber and Wookey2006), or the perceived increase in muskox may have meant there was increasing interspecific foraging competition (Jingfors, Reference Jingfors1984). It is also possible that a factor influencing shifts in migration pattern include a behavioural avoidance response to increased risk of parasitism. Many ruminants, including reindeer, reduce parasitism through faecal avoidance behaviour, with trade-offs made between forage quality and parasitism as faecal density increases in the preferred, and presumably better quality, pasture (van der Wal et al. Reference Van Der Wal, Irvine, Stien, Shepherd and Albon2000; Hutchings et al. Reference Hutchings, Gordon, Kyriazakis and Jackson2001a; Ezenwa, Reference Ezenwa2004). Research on sheep has shown that parasitized animals will avoid contaminated pasture (Hutchings et al. 2001 a); however, this is dependent on the individual's feeding motivation, as having higher feeding motivation leads to less avoidance (Hutchings et al. Reference Hutchings, Kyriazakis, Gordon and Jackson1999, 2001 b). The increasing ungulate population on the south of Victoria Island may have led to accumulation of parasites on the tundra. Avoiding the high parasite load may cause the caribou to alter foraging ranges.
Another issue for consideration with regards to the caribou-parasite relationship is the species of parasite present in the abomasal fauna. Despite the order of magnitude difference in absolute burdens, identification of the adult males from a subsample of the abomasums showed that the same species of nematodes are present in both the Svalbard reindeer and DU caribou, with O. gruehneri dominating the species composition of adult males at similar proportions. However, T. circumcincta and T. trifurcata are much more abundant amongst the caribou in contrast to the Svalbard population where they constitute less than 1%. Conversely there is a much lower proportion of M. marshalli in caribou, the second most abundant species on Svalbard. The difference in parasite species, their different annual cycles and parasite community interactions could have consequences for the role they play in their host's ecology and invites much further analysis.
The difference in proportional composition is potentially due to the presence of the sympatric host, muskox, in Canada. Muskox and caribou in North America have been recorded as sharing some similar abomasal parasites (Hoberg et al. Reference Hoberg, Kocan, Rickard, Samuel, Pybus and Kocan2001), although the previous identifications of T. circumcincta have been questioned after the description of T. boreoarcticus (Hoberg et al. Reference Hoberg, Monsen, Kutz and Blouin1999). In this study, T. circumcincta and T. trifurcata were 2 of only 3 types found in the muskox and were the only nematode species present in 3 of the 4 samples. T. boreoarcticus has previously been identified in muskox from south Victoria Island (Hoberg et al. Reference Hoberg, Monsen, Kutz and Blouin1999) but none could be verified as present in our samples.
The high proportion of Teladorsagia spp. amongst the caribou, in comparison to the Svalbard population, suggests that these nematodes benefit from the presence of the second host. If the presence of muskox enables the Teladorsagia nematodes to maintain higher population levels in co-habiting caribou compared to when caribou are the only host, it is possible that parasite-mediated apparent competition may occur. However, even if muskox and caribou are capable of sharing the same parasites, the complex question still remains about whether interspecific transmission occurs. On the south of Victoria Island parasite faecal egg counts from muskox on the south of Victoria Island showed the classic summer transmission pattern, with increasing egg output from spring towards mid-August before a decline through to autumn. This demonstrates that the muskox are loading the tundra with parasites in this region. Whether the parasites are transmitting to caribou depends on many factors of the host and parasite ecology such as the species of parasite laying eggs in summer, larval development rates and overlap in host foraging range, that still remain to be determined.
Further research is required to determine the potential role parasites play in both the behavioural and population ecology of caribou. In light of our results from abomasal parasite populations further attention should be paid to other parasites found in the caribou gastrointestinal tract (Hoberg et al. Reference Hoberg, Kocan, Rickard, Samuel, Pybus and Kocan2001). Furthermore, there is a need to elucidate and factor in the effects of parasite-mediated apparent competition from muskox. As more studies begin to focus on natural pastoral systems, it is becoming increasingly clear that although the logistics of such work may prove challenging, experimental research on the role of parasites is integral to a mechanistic understanding of the population dynamics of wild host species (Stien et al. Reference Stien, Irvine, Ropstad, Halvorsen, Langvatn and Albon2002) and has consequences for our understanding of plant herbivore interactions.
The authors gratefully thank Brent Patterson, Nathalie Griller, Mathieu Dumond, Monica Angohiatok and the Wildlife Division at the Government of Nunavut for carrying out all the April sampling. The Wildlife Division and the Centre for Ecology and Hydrology, UK, funded much of the field and laboratory work. The research on Victoria Island would not have been possible without the support of many people in the Cambridge Bay community including Naikak Hakongak, Shane Sather and Attima Hadlari, Joanne Rozitis, and staff at the Nunavut Arctic College for logistical support. Field assistants included Imogen Cust, Macneill Ferguson and Pete Bates. Lab assistants included Amy Clarke and Adam Boulton. Nematode taxonomy was carried out by Odd Halvorsen and Catherine Vollelve Zoologisk Museum, University of Oslo. Statistical advice was provided by David Elston, BioStatistics Scotland. Joelene Hughes was supported by a studentship from the University of Aberdeen, UK, with additional support from the Centre of Hydrology and Ecology, and the Macaulay Institute, Scotland.