INTRODUCTION
Parasites may negatively affect host fitness through increased mortality and reduced reproductive output. Fecundity, one measure of reproductive output, may be reduced through mechanisms decreasing the recruitment of oocytes into vitellogenesis, decreasing the number of vitellogenic oocytes through atresia, or, in batch-spawning species, reducing the number of serial spawnings during a reproductive season by increasing the interspawning interval. We would expect to observe some or all of these effects in hosts experiencing the exploitation by parasites of energy reserves needed for host oocyte growth and maturation. These effects should be especially significant in hosts facing the dual energetic demands of repeated spawning and rapidly growing parasites that attain a large size, because both processes should impose substantial costs to host energy reserves. Threespine stickleback fish Gasterosteus aculeatus infected with the pseudophyllidean cestode Schistocephalus solidus appears to provide an ideal host-parasite system in which to study these effects of parasitism on host reproduction (Heins and Baker, Reference Heins and Baker2008).
Infection by S. solidus imposes a high energetic demand on sticklebacks (Arme and Owen, Reference Arme and Owen1967; Walkey and Meakins, Reference Walkey and Meakins1970; Lester, Reference Lester1971; Meakins and Walkey, Reference Meakins and Walkey1975; Pascoe and Mattey, Reference Pascoe and Mattey1977). Female hosts of S. solidus showed a decrease in body condition with an increase in parasite index (Bagamian et al. Reference Bagamian, Heins and Baker2004), and Schultz et al. (Reference Schultz, Topper and Heins2006) found that infected stickleback females had lower somatic energy reserves than uninfected females. Notwithstanding the energetic demand of S. solidus infections, many infected threespine stickleback females in Alaska are capable of producing multiple batches (clutches) of eggs in serial spawnings, some in the face of substantial infections (Heins et al. Reference Heins, Singer and Baker1999; Heins and Baker, Reference Heins and Baker2008). Female stickleback in Alaska produce clutches of eggs at intervals of 2–8 days during a reproductive season lasting approximately 6 weeks (Baker et al. Reference Baker, Foster, Heins, Bell and King1998; Heins et al. Reference Heins, Singer and Baker1999; Brown-Peterson and Heins, Reference Brown-Peterson and Heins2009), requiring a large investment of energy. Bagamian et al. (Reference Bagamian, Heins and Baker2004) found that uninfected stickleback females showed higher body condition early in the breeding season than late in the season. Thus, the co-occurrence of energetic demands for both reproduction and infection appear to reduce body condition and, in turn, to affect reserve energy required for reproductive function (Bagamian et al. Reference Bagamian, Heins and Baker2004).
Here, we used histological analyses to investigate the influence of S. solidus infections on mechanisms of oocyte development. The use of histological analyses to estimate the interspawning interval of threespine sticklebacks in the wild has been demonstrated using the post-ovulatory follicle (POF) method (Brown-Peterson and Heins, Reference Brown-Peterson and Heins2009). Analyses of ovarian histology may also be used to study oocyte recruitment from primary growth into secondary growth (vitellogenesis) as well as atresia of vitellogenic oocytes. Annual fecundity of female stickleback is determined by the number of eggs in each spawn as well as by the number of spawnings each reproductive season. Any deleterious effect of S. solidus infection on the oocyte development processes resulting in fewer spawnings (longer interbrood interval) or reduced fecundity of each spawn (reduced recruitment or greater atresia) should, therefore, result in a decrease in annual reproductive output. Many female stickleback reproduce in only 1 spawning season before dying (Baker et al. Reference Baker, Heins, Foster and King2008); therefore, these processes are especially important because annual fecundity often represents lifetime fecundity.
Uninfected, field-caught stickleback females show a greater interspawning interval, hence fewer numbers of spawnings, late in the reproductive period than early in the spawning season (Brown-Peterson and Heins, Reference Brown-Peterson and Heins2009). The increase in interbrood interval appears to be the result of decreased energy reserves reflected in lower body condition late in the reproductive season (Bagamian et al. Reference Bagamian, Heins and Baker2004). Thus, we hypothesize that the nutrient exploitation by S. solidus and its attendant reduction of host energy reserves would lengthen the interspawning interval by slowing oocyte development in host females as compared to uninfected individuals. We also hypothesize that there may be lowered oocyte recruitment into vitellogenic oocytes and greater atresia of vitellogenic oocytes among infected females when compared to uninfected females.
MATERIALS AND METHODS
Study area
Samples were obtained from Walby Lake (61°37.230′N 149°12.798′W) in the Matanuska-Susitna (Mat-Su) Valley north of the Cook Inlet, Alaska. Walby Lake has a surface area of 22 hectares and lies among many lakes and ponds distributed across the glacial moraine of the Mat-Su Valley. Lakes in the Mat-Su Valley usually are covered with ice from approximately October into May (Woods, Reference Woods1985). The average July air temperature from 2 distant stations in the Mat-Su Valley is about 14–15°C (Woods, Reference Woods1985), and the average warmest summer temperatures throughout the area are about 18–20°C (Reger and Updike, Reference Reger, Updike, Pewe and Reger1983).
Fish collections
Samples of threespine sticklebacks were obtained from Walby Lake between late May and early June in the years 2000–2001. This time of the year is about the first half of a 6-week spawning season that occurs after ice-out in Alaska (Heins et al. Reference Heins, Singer and Baker1999). Collections were made using 6 mm un-baited wire-mesh minnow traps which were set near shore. Water temperatures were recorded to the nearest 1°C.
Female sticklebacks were anaesthetized until quiescent in MS222. Each specimen was prepared for histological examination in the field by removing the tail with an oblique cut through the caudal peduncle and cutting open the abdomen before placing it in a histological megacassette to be fixed and stored in 10% buffered formalin. The presence or absence of S. solidus was recorded for each specimen. The necessity to preserve specimens quickly with ovaries intact in the field for histological examination precluded counting or weighing the parasites.
Histological methods
Ovaries were removed from each specimen in the laboratory and staged macroscopically following the protocols of Baker et al. (Reference Baker, Foster, Heins, Bell and King1998) and Heins et al. (Reference Heins, Singer and Baker1999). Whole ovaries were placed into labelled cassettes, rinsed overnight in running tap water, dehydrated in a series of graded ethanols and embedded in paraffin following standard histological procedures. Ovarian tissue was sectioned at 4 μm and stained with Haematoxylin 2 and Eosin Y (Richard Allen Scientific, Kalamazoo, MI, USA) for histological examination.
Oocyte developmental stages (Grier et al. Reference Grier, Uribe-Aranzábel, Patiño and Jamieson2009) and ovarian developmental phases (Brown-Peterson et al. Reference Brown-Peterson, Lowerre-Barbieri, Macewicz, Saborido-Rey, Tomkiewicz and Wyanski2007) used here are briefly described, and the references may be consulted for more details. All oocytes to be spawned during the reproductive season are recruited from the primary growth stage oocytes into non-vitellogenic cortical alveolar (CA) oocytes and then into vitellogenic early secondary growth stage oocytes. Each group of oocytes that will undergo further synchronous growth, maturation, ovulation, and then oviposition is drawn from the pool of early secondary growth oocytes and forms a clutch or batch of distinctly larger late secondary growth oocytes. Females in the Spawning Capable reproductive phase have late secondary growth oocytes that are physiologically and developmentally capable of progressing through oocyte maturation and ovulation, which occur in the Actively Spawning reproductive phase.
Oocytes in all developmental stages (primary growth, CA, early secondary growth, late secondary growth, ovulated, atretic) were counted in 2 randomly chosen 100× fields of view, representing 1 mm2/view. For most sections, this represented about 80% of the entire ovary. The mean percentage of each oocyte type was calculated for each individual fish, and the ratio of primary growth to late secondary growth oocytes for each individual was used to assess recruitment from primary growth into vitellogenesis. The frequency distribution of percentage occurrence of late secondary growth oocytes was plotted for uninfected fish early- and mid-season in 2001 to determine fecundity type (i.e., determinate or indeterminate; Murua and Saborido-Rey, Reference Murua and Saborido-Rey2003). For oocyte analyses, n=99 for 2000 (55 infected, 44 uninfected), n=77 for early 2001 (35 infected, 42 uninfected) and n=74 for mid-2001 (54 infected, 20 uninfected).
POFs were used to assess interspawning interval based on Brown-Peterson and Heins (Reference Brown-Peterson and Heins2009). The time between successive spawns was inferred from POF age in females in the Spawning Capable and Actively Spawning phases with ovulated or non-ovulated oocytes contained in the ovary. Fish with POFs aged 36 h or younger are considered to have spawned less than 2 days previously, a 2-day interspawning interval corresponds to fish with 48 h POF, a 3-day interval corresponds to fish with 60–72 h POF, a 4-day interval corresponds to fish with 4-day-old POF, and fish classified as spawning more than 4 days previously had no POFs in the ovary. The POF percentages of females with ovulated oocytes in the ovary and POF <24 h was determined from the age of the oldest POF >24 h in the ovary. In cases where there were no POF >24 h present, these fish were assigned an interspawning interval of >4 days. This technique assured that POF percentages were not influenced by fish ovulating during capture. Female sticklebacks in Alaskan lakes show as much as a 48 h delay between ovulation and ovoposition (Brown-Peterson and Heins, Reference Brown-Peterson and Heins2009). For the purposes of our estimates of spawning periodicity, we assumed that ovulation frequency is closely related to spawning frequency although they do not occur simultaneously. Ovulated fish with POF ⩽24 h of age were included in the calculations of estimates of interspawning intervals for infected and uninfected fish. Calculations of interspawning intervals were based on fish with recent POF (⩽36 h), and thus give a more accurate estimate of recent spawning history than POFs of all ages. Older POFs should not be included in spawning frequency calculations (Hunter and Macewicz, Reference Hunter, Macewicz and Lasker1985). For POF and interspawning interval analyses, n=198 for 2000 (109 infected, 89 uninfected), n=75 for early 2001 (33 infected, 42 uninfected) and n=76 for mid 2001 (54 infected, 22 uninfected).
Statistical analysis
The ratio of oocyte stages (primary growth to late secondary growth) between infected and uninfected fish for each collection was compared using a Student's t-test. The percentages of atretic oocytes in infected and uninfected fish were compared among samples using a two-way ANOVA. All percentage values were arcsine-square-root transformed prior to analysis (Sokal and Rohlf, Reference Sokal and Rohlf1995). Data distributions were tested using a Kolmogorov-Smirnov one-sample test (normality) and Levene's Test (equality of variance). The interspawning intervals of infected and uninfected fish were compared using the Pearson Chi-Square and the strength of the association was evaluated using Phi and the Mantel-Haenszel common odds ratio. All tests were performed with SPSS version 11.5 (SPSS, Inc), and differences were considered significant if P⩽0·05.
RESULTS
Water temperatures
In 2000, temperatures ranged from 12 to 16°C during the May 31–June 1 sampling period. Temperatures ranged from 14 to 17°C during the early (May 29–30) collecting dates and 17 to 18·5°C during the mid-season (June 7–8) sampling period in 2001.
Determination of fecundity type
Frequency distributions of the percentage of late secondary growth oocytes in the ovaries of uninfected fish in 2001 showed that more sticklebacks had a higher percentage of late secondary growth oocytes early in the spawning season than in mid-season (Fig. 1). Furthermore, there was a significant difference (t60=2·185, P=0·033) in the mean percentage of secondary growth oocytes in the early season (40·5%) compared to mid season (29·9%). This decrease in the stock of late vitellogenic oocytes as the spawning season progressed provides evidence that threespine stickleback have determinate fecundity.

Fig. 1. Frequency distribution of the percentage of secondary growth oocytes in the ovaries of uninfected threespine stickleback from Walby Lake, Alaska, from early- (29–30 May) and mid-season (7–8 June) collections in 2001. Percentages of secondary growth oocytes are shown in groups of 10% each (n=42 early season, and n=20 mid-season).
Female reproductive condition
All uninfected and infected threespine stickleback females captured in 2000 and uninfected females captured in 2001 were in the Spawning Capable or Actively Spawning reproductive phases. Among infected females in 2001, however, 9% of those captured during the early part of the season were in the Developing phase and not yet capable of spawning. During the later portion of the 2001 season, 6% of the infected fish were in the Regressing phase and had ceased spawning. The remainder of infected females caught in 2001 was in the Spawning Capable or Actively Spawning phases.
Oocyte recruitment into vitellogenesis
The ratio of primary growth to late secondary growth oocytes was greater in infected than uninfected threespine sticklebacks for each of the 3 samples (Fig. 2). There was a significant difference in this ratio in 2000 (t90=−3·247, P=0·002) and early in the 2001 season (t68=−2·731, P=0·009). The ratio was marginally significant in the later portion of the 2001 season (t55=−1·745, P=0·087). These results show that fewer oocytes may be recruited into clutches from the stock of primary growth oocytes in fish infected with S. solidus.
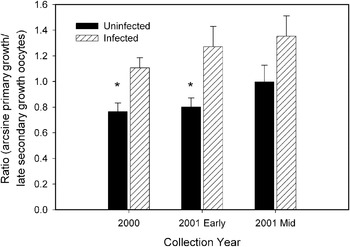
Fig. 2. Arcsine square root transformed primary growth to late secondary growth oocyte ratios (mean±s.e.) in threespine stickleback females that were uninfected or infected by Schistocephalus solidus in Walby Lake, Alaska (31 May–1 June, 2000, n=99; 29–30 May, 2001 [early], n=77; 7–8 June, 2001 [mid] n=74). *Significant difference (t-test, P<0·05).
Atresia of secondary growth oocytes
Atretic vitellogenic oocytes were uncommon in all collections regardless of parasitism, and ranged from 0% to 3·4% of total oocytes in a histological section among all females examined. There was no interaction between parasitism and collection date (F3, 324=0·556, P=0·644) and no significant difference in atresia between infected and uninfected fish (F1, 324=0·356, P=0·551). There was a marginally significant difference in atresia among collection dates (F3, 324=2·596, P=0·052), with fish collected in mid-season 2001 having a marginally higher percentage of atretic oocytes than those from 2000 (P=0·086, Games-Howell post-hoc test). These results show that infection by S. solidus does not reduce threespine stickleback fecundity nor the entire pool of vitellogenic ooctyes through increased atresia resulting in a greater resorption of vitellogenic oocytes.
Interspawning interval
Threespine stickleback showed a range of POF ages in the ovaries, from ⩽12 h through 4 days. Among both infected and uninfected fish, females without any POFs made up the greatest percentage of each sample, indicating a spawning frequency >4 days (Fig. 3) among the largest percentage of females. A greater percentage of infected fish had no POFs than uninfected females in each sample. Additionally, few infected fish had POFs <36 h (Fig. 3), suggesting less frequent spawns by fish infected with S. solidus. Although the interspawning interval of threespine stickleback infected with S. solidus was greater than that of uninfected fish in each sample (Table 1), none of the differences were significant. Furthermore, the low, and non-significant, values of Phi and the Mantel-Haenszel Odds Ratio (Table 1) confirm that there was a very weak association between interspawning interval and incidence of parasitism in all samples. This shows that infection by S. solidus did not affect ovulation and number of spawnings of threespine stickleback.
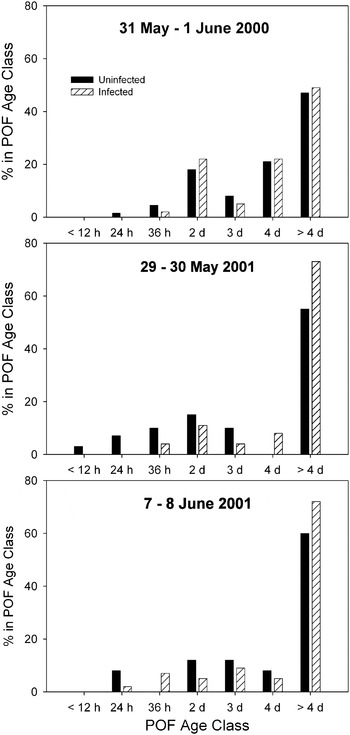
Fig. 3. Age distribution of post-ovulatory follicles (POF) in threespine sticklebacks that were uninfected or infected by Schistocephalus solidus from Walby Lake, Alaska, during 2000–2001. Age classes are <12 h, 12 h<x⩽24 h, 24 h<x⩽36 h, 36 h<x⩽2 d, 2 d<x⩽3 d, 3 d<x⩽4 d, and >4 d. Total sample sizes are n=198 for 2000, n=75 for early 2001, n=76 for mid 2001.
Table 1. Percentage of threespine stickleback uninfected and infected by Schistocephalus solidus containing POF ⩽36 h, and their estimated interspawning interval (days)
(Fish with ovulated oocytes in the ovaries are included in these calculations. n, number of fish examined.)

DISCUSSION
The results of this investigation demonstrate reduced recruitment of primary growth oocytes through intermediate stages of oocyte development into vitellogenic late secondary growth oocytes in threespine stickleback females infected with Schistocephalus solidus. This decreased recruitment reduces seasonal fecundity through smaller numbers of eggs in each spawn. Infection apparently does not influence female fecundity through atresia of vitellogenic oocytes, nor changes in the length of the interspawning interval. Thus, the distinct mechanisms operating within female stickleback at different stages in the oocyte development process are differentially affected by the presence of S. solidus.
Data presented here, as well as information from previous histological studies on stickleback (Wallace and Selman, Reference Wallace and Selman1979; Sokolowska and Kulzczkowska, Reference Sokolowska and Kulczykowska2006) indicate that G. aculeatus is a species with determinate fecundity. A decrease in the stock of secondary growth oocytes in the ovary during the spawning season (Fig. 1) is a diagnostic characteristic of species with determinate fecundity (Murua and Saborido-Rey, Reference Murua and Saborido-Rey2003). Determinate fecundity is a common reproductive strategy of fishes in northern latitudes that produce large demersal eggs (Murua and Saborido-Rey, Reference Murua and Saborido-Rey2003), such as stickleback. In fish exhibiting this strategy, the entire pool of vitellogenic oocytes (early and late secondary growth) from which oocytes will be drawn to develop multiple clutches of ripe eggs for ovoposition during the reproductive season is determined just before the spawning season begins, and the stock of vitellogenic oocytes is not replenished during the spawning season (Hunter et al. Reference Hunter, Macewicz, Lo and Kimbrell1992). The reduction of vitellogenic oocytes in the ovary as the spawning season progresses in fish with determinate fecundity helps explain the lack of difference in the ratio of primary growth to late secondary growth oocytes between infected and uninfected fish during the mid-season collection. Since all fish, regardless of infection status, would have fewer late secondary growth oocytes in the ovaries (hence a higher primary growth:secondary growth ratio) later in the season, the difference in ratio between infected and uninfected fish would be confounded by the naturally higher ratio occurring in uninfected fish.
The recruitment of primary growth oocytes into vitellogenesis is controlled by pituitary gonadotropins; they also regulate the production of ovarian oestrogens necessary for the regulation of vitellogenesis which influences the progression of oocytes from early to late secondary growth stages (Patiño and Sullivan, Reference Patiño and Sullivan2002). The process of oocyte maturation and eventual ovulation is controlled by a ‘maturation-inducing hormone’ (MIH), which is produced in the ovarian follicle (Patiño and Sullivan, Reference Patiño and Sullivan2002). Follicular competence to produce MIH depends on gonadotropin (Patiño and Thomas, Reference Patiño and Thomas1990). Thus, the basic hormonal regulation triggering the recruitment of a new batch of oocytes from early to late secondary growth stages is also important in oocyte maturation and ovulation. In the presence of S. solidus, threespine stickleback females recruit fewer primary growth oocytes into vitellogenesis, but do not change the inter-spawning interval although both functions have been thought to be dependent upon gonadotropin (Wallace and Selman, Reference Wallace and Selman1979). The counting (number of oocytes in each spawn) and the timing (occurrence of ovulation) functions of spawning, therefore, appear to be independent of one another despite their reliance on similar hormonal signals.
Infected threespine stickleback females in Alaska show a reduced clutch size (Heins and Baker, personal observation), apparently as a result of a reduction in the process of recruiting oocytes from early to late secondary growth oocytes as shown in this study. The late secondary growth oocytes undergo further growth, followed by maturation, ovulation and oviposition synchronously as a clutch (Heins and Rabito, Reference Heins and Rabito1986; Baker et al. Reference Baker, Foster, Heins, Bell and King1998). Food levels available to uninfected stickleback females can influence clutch sizes, with those females fed higher rations producing larger clutches than those on lower rations (Fletcher and Wootton, Reference Fletcher and Wootton1995; Ali and Wootton, Reference Ali and Wootton1999). That infected female stickleback recruit fewer oocytes into late secondary growth appears consistent with these observations because nutrient theft by S. solidus essentially starves the host fish (Schultz et al. Reference Schultz, Topper and Heins2006; Hall et al. Reference Hall, Becker and Cáceres2007).
After an uninfected female stickleback makes a commitment of oocytes for the next spawning, the number of oocytes in the clutch is not adjusted to the amount of energy income (Wootton, Reference Wootton, Bell and Foster1994). This earlier conclusion is consistent with our histological data for uninfected fish, which show that atresia of vitellogenic oocytes was low in uninfected fish. That atresia was also low in infected females and did not differ significantly from uninfected females shows that S. solidus infection does not have a differential effect at this stage of oocyte development. Thus, both uninfected and infected females largely complete maturation of all the oocytes recruited into late secondary growth.
Infected stickleback females produce ovulated, ripe eggs which are smaller in mass than uninfected females (Heins and Baker, Reference Heins and Baker2003), suggesting that nutrient theft during oocyte development depletes the female of the energy necessary to produce eggs as large as those of uninfected females. Resources for vitellogenic oocyte growth in threespine stickleback females are derived from ingested food (Wootton, Reference Wootton1977; Fletcher, Reference Fletcher1984), as is typical of an income breeder (Jönsson, Reference Jönsson1997; Poizat et al. Reference Poizat, Rosecchi and Crivelli1999). When insufficient food is consumed during interspawning intervals, however, somatic tissues may be depleted in uninfected fish to meet the energetic demands of growing oocytes (Wootton, Reference Wootton1977; Fletcher, Reference Fletcher1984). Thus, we would expect the size of the oocytes to be reduced because the number of oocytes in each clutch is not reduced by atresia. Moreover, host females with greater parasite:host mass ratios show a greater reduction in oocyte mass (Heins and Baker, Reference Heins and Baker2003), apparently as a result of greater energy loss.
Apparently there is no absolute ‘target’ mass for oocyte size that must be reached before the oocytes are ovulated, because infected fish ovulate eggs of significantly smaller mass than uninfected fish (Heins and Baker, Reference Heins and Baker2003). Perhaps the amount of time between recruitment of late secondary growth oocytes and ovulation is ‘fixed’ in this species, thus allowing smaller, nutrient-starved oocytes to achieve maturational competence within the same development time as larger oocytes from uninfected fish. This supposition is supported by the lack of difference in interspawning interval between infected and uninfected fish.
Egg size in uninfected stickleback females in the laboratory is little influenced by reduced energy income until the daily food ration is reduced to low levels (2% body weight) for an extended period of time (Fletcher and Wootton, Reference Fletcher and Wootton1995; Ali and Wootton, Reference Ali and Wootton1999). Studies of energy intake of uninfected stickleback may not be applicable to nutrient theft in infected females, as reduced energy input due to low food rations and reduced energy reserves due to nutrient theft may act through different underlying mechanisms. Alternatively, the results of Wootton and co-workers (Fletcher and Wootton, Reference Fletcher and Wootton1995; Ali and Wootton, Reference Ali and Wootton1999) may not extend to natural conditions under which wild, infected females are operating. If parasite growth resulting in increasing parasite:host mass ratio causes declining energy reserves in female stickleback below experimental levels, female stickleback may show a consistent reduction in oocyte size with increasing parasite mass, which would not be expected from the experiments of Wootton and others (Fletcher and Wootton, Reference Fletcher and Wootton1995; Ali and Wootton, Reference Ali and Wootton1999). Another hypothesis for the reduced oocyte size in infected females is that the counting function is inaccurate when early secondary growth oocytes are recruited into a clutch, resulting in a larger number of oocytes than can be supported for normal oocyte growth and development.
Although the data on interbrood interval suggest there may be a longer interspawning interval among infected females than among uninfected fish, the differences were not significant. Furthermore, the Phi statistic shows there is a weak, negative relationship between interspawning interval and incidence of parasitism, confirming the non-significant Chi-square value. The Odds Ratio, a measure of the effect size (Field, Reference Field2005), also confirms that there is no relationship between interspawing interval and parasitism, despite robust sample sizes. Thus, the time to ovulation is the same in both uninfected and infected females once the production of a clutch begins. Nonetheless, there may be ecological conditions under which the interbrood interval is greater among infected females. Interbrood interval significantly increases in uninfected sticklebacks as the spawning season progresses and as energy reserves reflected in body condition decrease (Bagamian et al. Reference Bagamian, Heins and Baker2004; Brown-Peterson and Heins, Reference Brown-Peterson and Heins2009). Thus, we expect there may be circumstances under which infection may result in a longer interbrood interval.
Schistocephalus solidus is a trophically transmitted parasitic castrator (Lafferty and Kuris, Reference Lafferty and Kuris2002; Kuris, Reference Kuris2003), and all data to date (Heins and Baker, Reference Heins and Baker2003; Bagamian et al. Reference Bagamian, Heins and Baker2004; Schultz et al. Reference Schultz, Topper and Heins2006) support the hypothesis that castration in Alaska occurs as a side effect of simple nutrient theft (Heins and Baker, Reference Heins and Baker2008). Host female sticklebacks are effectively operating like starved fish (Hall et al. Reference Hall, Becker and Cáceres2007), with metrics of reproduction declining until complete castration occurs. Thus, the number of oocytes recruited into late secondary growth in infected females is reduced in comparison to uninfected females, likely in response to lower energy reserves. Few vitellogenic oocytes are lost due to atresia, suggesting infected stickleback do not attempt to ‘recover’ energy through resorption of vitellogenic oocytes. Moreover the interbrood interval is unchanged in comparison to uninfected females. As a result, oocyte mass in spawns of infected fish appears to be reduced because nutrient theft by the parasite reduces the energy available for production of a previously set number of eggs in an unchanged interbrood interval.
ACKNOWLEDGEMENTS
We thank H. Martin and E. Birden (Tulane University) for assistance with field sampling in Alaska. Emily Birden (Tulane) also helped with laboratory processing of the 2001 samples. Gretchen Waggy-Grammer (The University of Southern Mississippi) was responsible for much of the histological processing, sectioning and staining. Mark S. Peterson and R.J. Griffitt (USM) provided valuable statistical analyses and discussions. The field sampling in Alaska was supported by research grants to D.C.H. from the Newcomb College Foundation of Tulane University.