Introduction
Besnoitia besnoiti is a cyst-forming apicomplexan parasite, belonging to the family Sarcocystidae and sub-family Toxoplasmatinae (Cortes et al., Reference Cortes, Leitao, Gottstein and Hemphill2014). The genus Besnoitia is comprised 10 species. Besnoitia besnoiti is closely related to Neospora caninum, which causes abortion and stillbirth in cattle and neuromuscular disease in dogs, and to Toxoplasma gondii, which causes disease not only in animals, but also in humans. In contrast to these two species, for which canids and felids serve as definitive hosts, the life cycle, and the definitive host of B. besnoiti has not been elucidated (Oryan et al., Reference Oryan, Silver and Sadoughifar2014). Cattle and wild bovids act as intermediate hosts (Alvarez-Garcia et al., Reference Alvarez-Garcia, Frey, Mora and Schares2013). Upon infection, B. besnoiti tachyzoites proliferate in endothelial cells of blood vessels. Subsequently, the formation of tissue cysts in different tissues takes place. During the acute phase of infection, clinical signs such as hyperthermia, weight loss, nasal and ocular discharge, and fibrinoid necrotic vascular lesions can result in death of infected animals (Basso et al., Reference Basso, Schares, Gollnick, Rutten and Deplazes2011; Lesser et al., Reference Lesser, Braun, Deplazes, Gottstein, Hilbe and Basso2012; Alvarez-Garcia et al., Reference Alvarez-Garcia, Frey, Mora and Schares2013; Dubey et al., Reference Dubey, van Wilpe, Blignaut, Schares and Williams2013). In the chronic phase scleroderma, scars, nodules on udders and progressive thickening and wrinkling of the skin occur, and infection of male genital organs may result in sterility (Frey et al., Reference Frey, Gutierrez-Exposito, Ortega-Mora, Benavides, Marcen, Castillo, Casasus, Sanz, Garcia-Lunar, Esteban-Gil and Alvarez-Garcia2013). One form of transmission of bovine besnoitiosis occurs mechanically by haematophagous insects (Bigalke, Reference Bigalke1968; Lienard et al., Reference Lienard, Salem, Grisez, Prevot, Bergeaud, Franc, Gottstein, Alzieu, Lagalisse and Jacquiet2011), through mating or medical devices, without any sexual cycle involved (Cortes et al., Reference Cortes, Leitao, Gottstein and Hemphill2014; Alvarez-Garcia et al., Reference Alvarez-Garcia, Frey, Mora and Schares2013). Besnoitiosis has been noted as an emerging disease in Southern Europe (Waap et al., Reference Waap, Nunes, Cortes, Leitao and Vaz2014) and in tropical and subtropical areas of Africa, Asia including the Middle East and South America (Jacquiet et al., Reference Jacquiet, Lienard and Franc2010; Alvarez-Garcia et al., Reference Alvarez-Garcia, Frey, Mora and Schares2013; Uzeda et al., Reference Uzeda, Andrade, Corbellini, Antonello, Vogel and Gondim2014). More recent cases have shown that bovine besnoitosis has spread from Portugal and France into Italy, Switzerland, Germany and it has also emerged in Central-Eastern Europe, including Hungary (Hornok et al., Reference Hornok, Fedak, Baska, Hofmann-Lehmann and Basso2014), Belgium and Ireland (Vanhoudt et al., Reference Vanhoudt, Pardon, De Schutter, Bosseler, Sarre, Vercruysse and Deprez2015; Ryan et al., Reference Ryan, Lee, Carty, O'Shaughnessy, Kelly, Cassidy, Sheehan, Johnson and de Waal2016).
There is no chemotherapy available (Cortes et al., Reference Cortes, Leitao, Gottstein and Hemphill2014). Besnoitia tachyzoites proliferate and cause disease in experimentally infected gerbils and rabbits, but neither in mice nor rats (Shkap et al., Reference Shkap, Pipano and Greenblatt1987a). Oxytetracyline has prevented the death of infected gerbils, but only when administered simultaneously with the parasites (Shkap et al., Reference Shkap, Pipano and Ungar-Waron1987b). Formalin, pentamidines, sulphonamides, trimethoprim, pyrimethamine and oxytetracycline have been evaluated as potential treatment options in experimentally infected rabbits (Pols, Reference Pols1960; Shkap et al., Reference Shkap, De Waal and Potgieter1985; Shkap et al., Reference Shkap, Pipano and Greenblatt1987a). Only limited numbers of animals were used, and assessments were mainly based on clinical features and histopathology, thus, no definitive conclusions can be drawn from these studies. Formalin, sulphametazine, toltrazuril and oxytetracycline have been assessed as potential treatments in cattle (reviewed in Cortes et al., Reference Cortes, Leitao, Gottstein and Hemphill2014).
The repurposing of established drugs may be a suitable strategy to discover novel compounds against besnoitiosis (Sateriale et al., Reference Sateriale, Bessoff, Sarkar and Huston2014). In vitro, Besnoitia tachyzoites are easily cultured, as they proliferate in a variety of host cells of different mammalian origin, including the two monkey kidney cell lines Vero and MARC-145, and in human foreskin fibroblasts (HFF) among others (Cortes et al., Reference Cortes, Reis, Waap, Vidal, Soares, Marques, Pereira da Fonseca, Fazendeiro, Ferreira, Caeiro, Shkap, Hemphill and Leitao2006; Frey et al., Reference Frey, Regidor-Cerrillo, Marreros, Garcia-Lunar, Gutierrez-Exposito, Schares, Dubey, Gentile, Jacquiet, Shkap, Cortes, Ortega-Mora and Alvarez-Garcia2016). More detailed investigations employing in vitro cultures have shown that thiazolides (Cortes et al., Reference Cortes, Müller, Esposito, Leitao, Naguleswaran and Hemphill2007a), new-generation pentamidine derivates and arylimidamides (Cortes et al., Reference Cortes, Müller, Boykin, Stephens and Hemphill2011), and calcium-dependent protein kinase class 1 (CDPK1) inhibitors such as BKI-1294 and related compounds (Jimenez-Melendez et al., Reference Jimenez-Melendez, Ojo, Wallace, Smith, Hemphill, Balmer, Regidor-Cerrillo, Ortega-Mora, Hehl, Fan, Maly, Van Voorhis and Alvarez-Garcia2017) inhibited proliferation of Besnoitia tachyzoites in vitro.
Buparvaquone (BPQ) is another interesting candidate for drug repurposing (McHardy and Morgan, Reference McHardy and Morgan1985). The drug is commercially available in different areas of the world with different names and formulations (McDougall et al., Reference McDougall, Hillerton and Pegram2016). However, none of these commercial products are available in the EU.
In Theileria-endemic regions BPQ is the drug of choice to be used against bovine theileriosis. Against Theileria parva-infected cells, BPQ displays an EC50 of 0.0003 mg L−1, it has a plasma half-life of at least 7 days, and a low toxicity (LD50 in rats >8000 mg kg−1). Besides an outstanding activity against T. parva and T. annulata (Hashemi-Fesharki, Reference Hashemi-Fesharki1991; Hostettler et al., Reference Hostettler, Müller, Stephens, Haynes and Hemphill2014), BPQ is also active against other protozoan parasites including Leishmania spp. (Croft et al., Reference Croft, Hogg, Gutteridge, Hudson and Randall1992; da Costa-Silva et al., Reference da Costa-Silva, Galisteo, Lindoso, Barbosa and Tempone2017), Theileria equi (formerly named Babesia equi) (Zaugg and Lane, Reference Zaugg and Lane1992), and against N. caninum and T. gondii, not only in vitro but also in both non-pregnant and pregnant mouse models (Müller et al., Reference Müller, Aguado-Martinez, Manser, Balmer, Winzer, Ritler, Hostettler, Solís, Ortega-Mora and Hemphill2015, Reference Müller, Aguado-Martinez, Manser, Wong, Haynes and Hemphill2016, Reference Müller, Aguado-Martinez, Ortega-Mora, Moreno-Gonzalo, Ferre, Hulverson, Choi, McCloskey, Barrett, Maly, Ojo, Van Voorhis and Hemphill2017b). Experiments in pregnant mice did not only demonstrate the outstanding efficacy of BPQ treatment in preventing vertical transmission by these parasites but also documented the safety of the compound upon application during pregnancy. As BPQ is already licensed for use in cattle, although not in the EU, we studied the effects of the drug against B. besnoiti tachyzoites, and demonstrate that, despite a low IC50 in short-term treatment assays, these parasites adapted to increased drug concentrations within few days, and resistant parasites exhibited distinct ultrastructural characteristics, including a lack of discernible mitochondria.
Material and methods
Tissue culture media, biochemicals and drugs
If not stated otherwise, all tissue culture media were purchased from Gibco-BRL (Zürich, Switzerland), and biochemical reagents were from Sigma (St. Louis, MO). Kits for molecular biology were purchased from Qiagen (Hilden, Germany). BPQ was provided by Cross Vetpharm Group Limited (Dublin, Ireland), and was kept as a 10 mm stock solution in dimethyl sulphoxide (DMSO) at −20 °C.
Host cell cultivation and maintenance of B. besnoiti tachyzoites
HFF were maintained in DMEM (Dulbecco's modified Eagle medium), and Vero cells were cultured in the RPMI-1640 medium, both with phenol red supplemented with 10% heat-inactivated and sterile filtrated fetal calf serum, 50 U of penicillin mL−1, and 50 µg streptomycin mL−1 (=culture medium). Cultures were maintained at 37 °C and 5% CO2 in tissue culture flasks (Sarstedt, Nürnbrecht, Germany), and were passaged at least once a week. Tachyzoites of B. besnoiti (Bb1Evora, kindly provided by Dr Helder Cortes, the University of Evora, Portugal) were maintained by serial passages in Vero cells as previously described (Cortes et al., Reference Cortes, Müller, Esposito, Leitao, Naguleswaran and Hemphill2007a). Tachyzoites were harvested by removing infected cell layers with a rubber cell scraper, followed by repeated passages through a 25-gauge needle at 4 °C to liberate parasites. Tachyzoites were separated from cell debris by Sephadex-G25 chromatography and were immediately used for infection experiments (Hemphill et al., Reference Hemphill, Gottstein and Kaufmann1996).
Determination of BPQ efficacy
HFF were grown in six-well plates until a confluent monolayer was formed. Just prior to infection, BPQ was added at concentrations of 30, 60, 120 and 240 nm. The bumped kinase inhibitor BKI 1294 (Jimenez-Melendez et al., Reference Jimenez-Melendez, Ojo, Wallace, Smith, Hemphill, Balmer, Regidor-Cerrillo, Ortega-Mora, Hehl, Fan, Maly, Van Voorhis and Alvarez-Garcia2017; Van Voorhis et al., Reference Van Voorhis, Doggett, Parsons, Hulverson, Choi, Arnold, Riggs, Hemphill, Howe, Mealey, Lau, Merritt, Maly, Fan and Ojo2017) was added as an inhibition control at 5 µ m. Controls received the corresponding amounts of DMSO. HFF were then infected with 5 × 104 freshly purified B. besnoitia tachyzoites, in a total volume of 5 mL. After 4 days, cells were collected with a cell scraper, centrifuged, washed once in PBS and the pellet was stored at −20 °C prior to quantification of parasite proliferation. Assays were carried out in quadruplicates and each experiment was repeated at least twice. DNA purification was performed employing the DNeasy Blood & Tissue Kit (Qiagen, Basel, Switzerland) according to the standard protocol suitable for animal cells. Besnoitia besnoiti parasite load was determined by real-time PCR as previously described (Cortes et al., Reference Cortes, Gottstein, Hemphill, Leitao and Müller2007b). The parasite counts were calculated by interpolation from a standard curve with DNA equivalents from 1000, 100 and 10 culture-derived tachyzoites included in each run.
Visualization of BPQ-induced changes by transmission electron microscopy (TEM)
HFF were grown to confluence in T-25-flasks and were infected with B. besnoiti (106 tachyzoites per flask). After 48 h, cultures were supplemented with 1 µ m BPQ, or the corresponding amount of DMSO as a control. At 24, 48 and 96 h of drug treatments monolayers were washed with 100 mm sodium cacodylate buffer (pH 7.3) and fixed with cacodylate buffer containing 2.5% glutaraldehyde for 10 min (Alaeddine et al., Reference Alaeddine, Hemphill, Debache and Guionaud2013). Cells were collected using a rubber cell scraper and centrifuged for 10 min at 1200 rpm at 20 °C. The supernatant was removed, and infected cells were fixed further in glutaraldehyde/cacodylate at 4 °C overnight. Post-fixation in 2% OsO4, dehydration, embedding in Epon 820 epoxy resin and cutting of ultrathin sections was done as previously described (Guionaud et al., Reference Guionaud, Hemphill, Mevissen and Alaeddine2010; Alaeddine et al., Reference Alaeddine, Hemphill, Debache and Guionaud2013). Specimens were viewed on a CM12 TEM operating at 80 kV.
Adaptation of B. besnoiti tachyzoites to increased BPQ concentrations
HFF were grown to confluence in T25 tissue culture flasks and were infected with 106 B. besnoitii tachyzoites. After 24 h, the medium of the flasks was substituted by fresh culture medium, or medium containing 100 nm, 500 nm or 1 µ m of BPQ as indicated in Table 1. Flasks were daily checked microscopically for the occurrence of parasite growth. Patches of HFF monolayers destroyed as a consequence of tachyzoite egress were considered as proof of growth. After 9 days, parasites cultured in 500 nm or 1 µ m of BPQ were exposed to higher drug concentrations (5, 7.5 and 10 µ m, respectively) for another 9 days. During these time periods, the flasks were again microscopically checked daily for signs of re-emerging parasite replication and parasitophorous vacuole formation. Stabilates of B. besnoiti cultures adapted to 5 and 10 µ m (named R_5 or R_10, respectively) were prepared. As controls, stabilates of parasites that had not been exposed to BPQ were also prepared. All samples were stored at −196 °C for at least 1 month prior to further assessments.
Table 1. Treatment of Besnoitia besnoiti infected HFF with BPQ does not act parasiticial and tachyzoites adapt readily to increased BPQ concentrations

Confluent HFF grown in T-25 tissue culture flasks were infected with 106 B. besnoiti tachyzoites. After 2 h non-invaded parasites were removed and medium with or without 100, 500 and 1000 nm BPQ was added. Cultures were maintained during a maximal timespan of 9 days in the presence of the drug, during which parasites resumed proliferation at all concentrations. Those parasites treated with 500 and 1000 nm BPQ were exposed to increased BPQ concentration for another 9 days, but proliferation in those cultures was also observed at the time points indicated.
dpi, days post-infection.
a Indicates cultures of which stabilates were made that were re-introduced into the culture.
Assessments of BPQ-adapted tachyzoites
Drug adapted as well as non-adapted stabilates were thawed and cultured on Vero cells without drug pressure for up to 2 weeks (3–4 passages). Subsequently, they were harvested and 104 tachyzoites were seeded onto HFF monolayer grown in six-well plates. Just prior to infection, BPQ was added at concentrations, of either 0, 100, 625 or 5000 nm, and specimens were cultured at 37 °C and 5% CO2 for 4 days. Subsequently, the parasite load was determined by real-time PCR as described above. To visualize the ultrastructure of drug-adapted B. besnoiti tachyzoites, stabilates grown in Vero cells for 2 weeks were fixed and processed for TEM as described above, and specimens were viewed on a Phillips 400 TEM operating at 80 kV.
Statistics
IC50 values were calculated after the logit-log-transformation of the relative growth (RG; control = 1) according to the formula ln[(RG/(1−RG)] = a×ln(drug concentration) + b and subsequent regression analysis by the corresponding software tool contained in the Excel software package (Microsoft, Seattle, WA, USA). Student's t-test was used to assess the growth of BPQ-adapted and treated B. besnoiti strains with respect to the non-adapted wild-type strain exposed to 100 nm BPQ.
Results
In short-term treatment assays, BPQ severely interferes in B. besnoiti tachyzoite proliferation
To establish the in vitro efficacy assays, HFF monolayers were infected with B. besnoiti tachyzoites and the proliferation of parasites was monitored by quantitative PCR over time. As a control for inhibition of infection, we added the CDPK1-inhibitor BKI-1294 (5 µ m) to some wells prior to infection. The other wells received only DMSO as a solvent control. In the solvent control samples, a proliferation of tachyzoites could be measured after 2 days post-infection (p.i.), reaching the exponential phase after 4 days. In the cells treated with BKI-1294, no proliferation was visible (Fig. 1A). Since after 4 days, the infected monolayers showed first lesions and the numbers of extracellular parasites started to increase, the endpoint of our efficacy tests was set at 4 days p.i. In these tests, BKI-1294 inhibited the proliferation of Besnoitia tachyzoites with an IC50 of 0.12 ± 0.03 µ m, and nearly complete inhibition was achieved at 5 µ m (Fig. 1B). The IC50 of BPQ was much lower, namely 10 ± 3 nm, virtually complete inhibition was obtained with 125 nm BPQ, and the IC99 of BPQ was 250 nm (Fig. 1C).

Fig. 1. Effects of BKI-1294 (1294) and BPQ on the intracellular proliferation of Besnoitia besnoitii tachyzoites in human HFF. HFF were grown to confluence in six-well tissue culture plates, supplemented with BKI-1294, BPQ or with DMSO as a solvent control and were immediately infected with B. besnoitii tachyzoites (2 × 104 per well). (A) Cultures treated with 5 µ m BKI-1294 and harvested after 1–4 days. (B) Cultures were treated with a concentration series of BKI-1294 and harvested after 4 days. (C) Cultures were exposed to a concentration series of BPQ and harvested after 4 days. Besnoitia besnoitii tachyzoites were quantified by qPCR. Mean ± s.e. values correspond to four replicates.
BPQ-treatment induces severe ultrastructural changes in B. besnoiti tachyzoites
Besnoitia besnotia-infected HFF were exposed to 1 µ m BPQ for 24, 48 and 96 h, or were maintained in the absence of BPQ, but in the presence of the corresponding amount of DMSO, and processed for TEM. Tachyzoites, cultured in the absence of BPQ (Fig. 2A) exhibited the typical features of apicomplexan parasites, including the apical conoid and a set of secretory organelles named micronemes and rhoptries at the anterior end, and dense granules localized apically as well as at the posterior part. A prominent feature of these parasites is the mitochondrion, parts of which are seen on a single section, with a relatively electron-dense matrix and cristae-like structures clearly visible in untreated parasites (Fig. 2A). Exposure to 1 µ m BPQ for 24 h (Fig. 2B, C) did not lead to severe changes in parasite ultrastructure, but some alterations, most notably in some of the mitochondria, became already evident, including a less electron dense matrix and slight disturbances in cristae structure (Fig. 2C). Changes became more profound after 48 h of BPQ treatment (Fig. 3A, B), where discernible mitochondria were largely absent, and the mitochondrial matrix was basically replaced by an amorphous substance. At this stage, rhoptries, dense granules and micronemes were still visible, and parasites kept residing within a parasitophorous vacuole. Upon treatment for 96 h (Fig. 3C, D), tachyzoites were still trapped within the parasitophorous vacuole but appeared largely distorted, the cytoplasm became continuously filled with amylopectin granules and other vacuoles containing components of the unknown composition, and nuclei appeared fragmented. However, the conoid remained still intact, with adjacent micronemes and dense granules in place, but rhoptries were not discernible anymore. Overall, BPQ treatment induced considerable damage, initially targeting the mitochondria, and subsequently exposure to this compound led to severe alterations in tachyzoites. In contrast, host cell mitochondria did not appear to be notably affected by the drug.

Fig. 2. Ultrastructural changes in Besnoitia besnoiti tachyzoites induced by BPQ treatment. Infected HFF were fixed and processed for TEM on day 3 post-infection and treatment with DMSO but no drug (A), or after 24 h of 1 µ m BPQ treatment (B, C). Panel (A) shows a parasitophorous vacuole containing several tachyzoites located within the HFF cytoplasm. Note that mitochondria (mito) contain an electron-dense matrix and clearly discernible cristae. Panels (B, C) show infected HFF exposed to 1 µ m BPQ for 24 h. The boxed area in (B) is enlarged in (C). Note the changes in the mitochondrial matrix (mito) occurring with drug exposure (B, C), while the host cell mitochondria (h_mito) appear unaffected; nu, parasite nuclei; mic, micronemes; rop, rhoptries; dg, dense granules; con, conoid. Bars in (A) and (B) = 0.6 µ m, in (C) = 0.32 µ m.

Fig. 3. Ultrastructural changes in Besnoitia besnoiti tachyzoites visualized after 2 and 4 days of BPQ treatment. Panels (A, B) show alterations induced after 48 h, (C, D) after 96 h. The boxed areas in (A) and (C) are enlarged in (B) and (D), respectively. Note the profound BPQ-induced changes in tachyzoite mitochondria in (B), and parasites still located within a parasitophorous vacuole (pv) in (C), including large numbers of amylopectin granules (apg); nu, parasite nuclei; mic, micronemes; rop, rhoptries; con, conoid. Bars in (A) = 1.8 µm; in (B) = 0.35 µ m; (C) = 2.2 µ m; in (D) = 0.3 µ m.
Upon long-term treatment, BPQ does not act parasiticidal and B. besnoiti tachyzoites readily adapt to increased BPQ concentrations
To investigate whether BPQ would also affect an established infection and whether this drug acted parasiticidal or parasitostatic, infected HFF monolayers were treated with 0.1, 0.5 or 1 µ m BPQ at 24 h p.i., and cultures were inspected daily by light microscopy. Initially, BPQ stopped intracellular proliferation when added 24 h p.i. However, when treatments were continued for a period of 9 days, the initial inhibitory effects got lost, and parasites started to resume growth in the presence of BPQ. The concentrations of BPQ were subsequently increased to 5, 7.5 and 10 µ m (see Table 1). At these concentrations, the proliferation of parasites was still noted after 2 weeks of drug exposure. Higher concentrations of BPQ were not sustained by the host cells. Thus, upon prolonged exposure, B. besnoiti tachyzoites exhibit a remarkable adaptive potential, rendering them largely tolerant to massively increased concentrations of BPQ.
To see whether this adaptation was permanent or occurred only transiently, tachyzoites adapted to 5 and 10 µ m of BPQ were transformed into stabilates, stored at −196 °C for 1 month, and were regrown in Vero cells without drug pressure for up to 2 weeks. Subsequently, they were harvested and subjected to drug efficacy tests on HFF as described. Non-resistant tachyzoites were processed identically, and these control Besnoitia were strongly susceptible to treatment with 100 nm BPQ (Fig. 4). However, Besnoitia that were adapted to 5 µ m or 10 µ m BPQ (R_5 or R_10; i.e. adapted to 5 or 10 µ m) had maintained their strongly decreased susceptibility and resumed proliferation even at 5 µ m BPQ, namely to 28% of the control value for R_5 and to 58% of the control value for R_10 (Fig. 5). These effects were statistically significant (Student's t-test, P < 0.01).
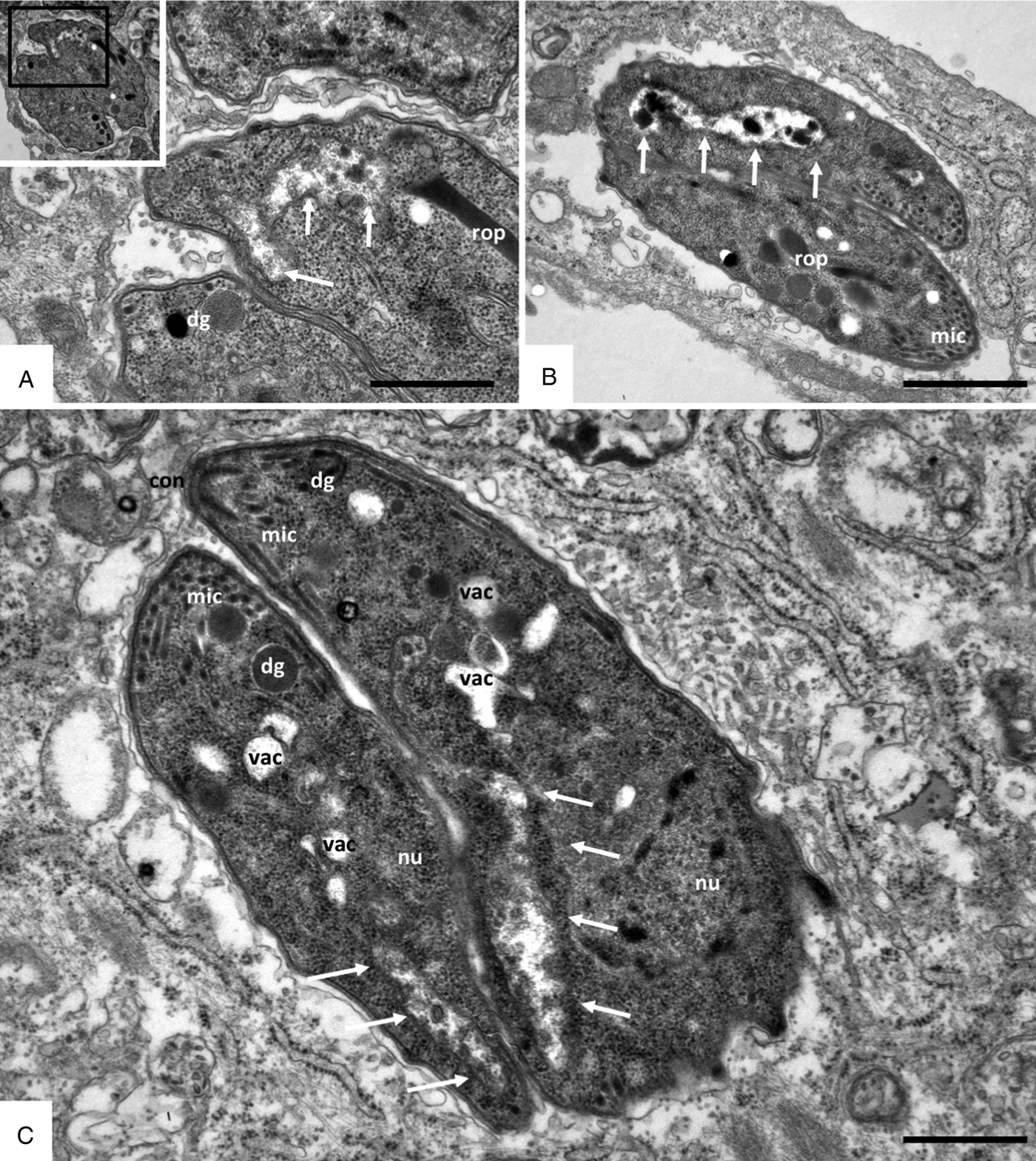
Fig. 4. Ultrastructure of BPQ-adapted Besnoitia besnoiti strains grown in HFF after stabilate preparation and re-introduction into culture for 2 weeks. Panels (A, B) show R_5 and (C) shows the R_10 strain. The insert in (A) is a low magnification view, and the respective boxed area is shown enlarged. Parasites appear structurally normal, except for the absence of a visible mitochondrial matrix [indicated by arrows in (A), (B) and (C)]. nu, parasite nucleus; dg, dense granules; mic, micronemes; rop, rhoptries; con, conoid; vac indicates cytoplasmic vacuoles. Bars in (A) = 0.66 µ m; (B) = 1 µ m; (C) = 0.6 µ m.

Fig. 5. Effects of BPQ on the intracellular proliferation of normal and BPQ-adapted Besnoitia besnoiti tachyzoites in HFF. HFF monolayer were infected with either normal (wt = B. besnoitii never exposed to BPQ) or BPQ-adapted tachyzoites [R_5 and R_10 are two strains that still underwent proliferation in the presence of 5 and 10 µ m BPQ (see Table 1)]. BPQ was added at 0, 625 or 5000 nm, and proliferation tachyzoites were quantitatively assessed by qPCR after 4 days. Mean ± s.e. values correspond to four replicates. Asterisks indicate highly significant growth (P < 0.01) in relation to the control treated with 100 nm BPQ.
Visualization of these adapted Besnoitia tachyzoites by TEM (Fig. 4) showed that they, similar to non-adapted tachyzoites, were localized within a parasitophorous vacuole, underwent endodyogeny and contained micronemes, rhoptries and dense granules similar to non-adapted tachyzoites (Fig. 2A). However, a prominent feature of these BPQ adapted parasites was the apparent lack of an organized mitochondrial matrix. In some cases, the matrix was completely lacking, and mitochondria were filled with amorphous electron-dense material of unknown composition (Fig. 4A, B). In other instances, the matrix was partially present, most notably in the periphery of the mitochondria, but no matrix was discernible in the central part of the mitochondrium (Fig. 4C). Tachyzoites that had adapted to increased BPQ concentrations also exhibited frequent vacuolization.
Discussion
The present study shows that in 4-day treatment assays BPQ is highly efficacious against B. besnoiti tachyzoites with IC50 values in the nanomolar range. Thus, in vitro, BPQ is more effective in terms of proliferation inhibition than thiazolides (Cortes et al., Reference Cortes, Müller, Esposito, Leitao, Naguleswaran and Hemphill2007a) and arylimidamides (Cortes et al., Reference Cortes, Müller, Boykin, Stephens and Hemphill2011), two classes of compounds investigated in previous screens. BPQ was also more effective than a range of BKIs recently studied (Jimenez-Melendez et al., Reference Jimenez-Melendez, Ojo, Wallace, Smith, Hemphill, Balmer, Regidor-Cerrillo, Ortega-Mora, Hehl, Fan, Maly, Van Voorhis and Alvarez-Garcia2017), including the previously characterized BKI-1294. The IC50 for BKI-1294 against B. besnoitia obtained in this study (0.12 ± 0.03 µ m) was in the same range but slightly higher than the one (0.045 µ m) reported by Jimenez-Melendez et al. (Reference Jimenez-Melendez, Ojo, Wallace, Smith, Hemphill, Balmer, Regidor-Cerrillo, Ortega-Mora, Hehl, Fan, Maly, Van Voorhis and Alvarez-Garcia2017). Such differences can be attributed to the different isolates, and also different host cells, that were used, and most likely also culture conditions that vary between the laboratories. BKI-1294 impairs the activity of CDPK1, which is an essential kinase involved in host cell invasion and egress of apicomplexan parasites (Lourido et al., Reference Lourido, Shuman, Zhang, Shokat, Hui and Sibley2010). In T. gondii, N. caninum and B. besnoiti, BKI-1294 treatment of already intracellular parasites leads to the formation of multinucleated complexes (Ojo et al., Reference Ojo, Reid, Kallur Siddaramaiah, Müller, Winzer, Zhang, Keyloun, Vidadala, Merritt, Hol, Maly, Fan, Van Voorhis and Hemphill2014; Winzer et al., Reference Winzer, Müller, Aguado-Martinez, Rahman, Balmer, Manser, Ortega-Mora, Ojo, Fan, Maly, Van Voorhis and Hemphill2015; Jimenez-Melendez et al., Reference Jimenez-Melendez, Ojo, Wallace, Smith, Hemphill, Balmer, Regidor-Cerrillo, Ortega-Mora, Hehl, Fan, Maly, Van Voorhis and Alvarez-Garcia2017). Thus, besides interference in invasion and egress, BKI-1294 also impairs processes involved in the completion of the cell cycle and formation of daughter zoites. In contrast, the major processes involved in the mechanism of action of BPQ are not those associated with host cell invasion, but BPQ rapidly affects the structural integrity of intracellular parasites and thus interferes in intracellular proliferation. After 24 h of treatment, the primary indications of interference that are noted are rather subtle changes in the matrix of the mitochondrium of B. besnoitia tachyzoites. Other alterations that were visualized at later time points, such as increased cytoplasmic vacuolization, fragmentation of the nucleus and a general build-up of amylopectin granules in the cytoplasm of these parasites, have also been described after treatments with nitazoxanide and pentamidine derivatives (Cortes et al., Reference Cortes, Müller, Esposito, Leitao, Naguleswaran and Hemphill2007a; Cortes et al., Reference Cortes, Müller, Boykin, Stephens and Hemphill2011), and also during treatment with bumped kinase inhibitors recently reported by Jimenez-Melendez et al. (Reference Jimenez-Melendez, Ojo, Wallace, Smith, Hemphill, Balmer, Regidor-Cerrillo, Ortega-Mora, Hehl, Fan, Maly, Van Voorhis and Alvarez-Garcia2017). Thus, these features are most likely associated with general physiological stress and upcoming cell death rather than being specific indicators of the mechanism of action of BPQ. The rapid action of BPQ in good agreement with previous findings on Theileria where gene expression of the intracellular parasite decreases already after 2 h of BPQ treatment and increased vacuolization of the parasite cytoplasm is seen already after 6 h (Hostettler et al., Reference Hostettler, Müller, Stephens, Haynes and Hemphill2014). There is, however, a marked difference in N. caninum, which is closely related to B. besnoiti, where ultrastructural changes upon treatment with 1 µ m BPQ were reported to be clearly visible only after 3 days of treatment (Müller et al., Reference Müller, Aguado-Martinez, Manser, Balmer, Winzer, Ritler, Hostettler, Solís, Ortega-Mora and Hemphill2015). In 4-day proliferation assays, BPQ inhibited N. caninum tachyzoite replication in a similar manner as B. besnoiti (IC50 = 4.9 nm; MIC = 100 nm). In the long term, tachyzoites also adapted and resumed proliferation in the presence of 100 nm BPQ after 20 days. However, for N. caninum parasiticidal activity was noted after 9 days of culture with 0.5 µ m or 6 days with 1 µ m BPQ.
Treatment of B. besnoiti-infected host cells with increasing concentrations of BPQ starting at 100 nm and going up to 1000 nm leads to a rapid adaptation of these parasites with unique features, showing that BPQ, while being highly effective in the short term (i.e. within the first few days of treatment), does not act parasiticidal, even after several days of continuous treatment at concentrations of up to 10 µ m. In two independent experiments, we have selected B. besnoiti strains proliferating in the presence of 5 µ m BPQ, thus at a concentration nearly three orders of magnitude above the IC50. Tachyzoites of these strains maintained their decreased susceptibility to BPQ even after freeze–thawing of stabilates and re-introduction into culture for 2 weeks. When inspected by TEM, we found that these parasites exhibited the overall normal features of apicomplexan parasites, with the exception of distinct structural alterations in the mitochondrium, which was lacking an intact matrix, partially devoid of content or filled with electron dense inclusions of unknown nature. At this point, it is not clear whether the mitochondrium in these parasites is functional or not. Clearly, these structural alterations do not automatically imply that all mitochondrial activities are impaired, thus the question of how these parasites can overcome this obvious structural defect needs to be investigated in the future.
Previous studies in Theileria showed that point mutations of the cytochrome b-gene are associated with resistance to BPQ (Sharifiyazdi et al., Reference Sharifiyazdi, Namazi, Oryan, Shahriari and Razavi2012; Mhadhbi et al., Reference Mhadhbi, Chaouch, Ajroud, Darghouth and BenAbderrazak2015). The cytochrome-bc1-complex (respiratory Complex III) transfers electrons from coenzyme Q to cytochrome c (Mitchell, Reference Mitchell1975). BPQ could block this transfer, thereby causing the relocation of electrons to other biomolecules generating free radicals and reactive oxygen species (ROS) detrimental to the parasite. By point mutations on the target or – like in this case – by the disappearance of structurally intact mitochondria, the parasite could overcome this effect of the drug. A plethora of studies with yeast has revealed the role of mitochondria in ROS-dependent cell death [see Pereira et al. (Reference Pereira, Silva, Saraiva, Johansson, Sousa and Corte-Real2008) and references therein]. In one case, the deleterious effects of ROS production are counteracted by the induction of petite mutants lacking mitochondria (Granot et al., Reference Granot, Levine and Dor-Hefetz2003).
The cytochrome-bc1-complex may not be the only target for BPQ, as shown more recently by the identification of a secreted prolyl-isomerase of T. annulata as another potential target (Marsolier et al., Reference Marsolier, Perichon, DeBarry, Villoutreix, Chluba, Lopez, Garrido, Zhou, Lu, Fritsch, Ait-Si-Ali, Mhadhbi, Medjkane and Weitzman2015). Inhibition of this enzyme would, however, cause rather delayed effects, as seen previously for N. caninum, due to the accumulation of misfolded effector proteins whereas inhibition of the respiratory chain is in good agreement with the more rapid effects observed in Besnoitia and with the high adaptation potential by the absence of functional mitochondria. Since Besnoitia tachyzoites exhibiting strongly altered mitochondria are fully viable (albeit with a slower proliferation rate), their energy metabolism must be entirely depending on glycolysis. Moreover, the intermediate metabolites provided by intact mitochondria must be scavenged from the host cell. Certainly, more research is needed to elucidate this mechanism that leads to this marked tolerance to increased drug concentrations.
Our observations could potentially discourage the repurposing of BPQ for the treatment of besnoitiosis in cattle. However, more recently other compounds such as several BKIs were shown to exhibit excellent efficacy in vivo in both pregnant and non-pregnant mice infected with N. caninum (Ojo et al., Reference Ojo, Reid, Kallur Siddaramaiah, Müller, Winzer, Zhang, Keyloun, Vidadala, Merritt, Hol, Maly, Fan, Van Voorhis and Hemphill2014; Winzer et al., Reference Winzer, Müller, Aguado-Martinez, Rahman, Balmer, Manser, Ortega-Mora, Ojo, Fan, Maly, Van Voorhis and Hemphill2015; Müller et al., Reference Müller, Aguado-Martinez, Balmer, Maly, Fan, Ortega-Mora, Ojo, Van Voorhis and Hemphill2017a), and BPQ was also shown to be highly effective in a pregnant mouse model for congenital toxoplasmosis, clearly limiting cerebral infection and disease in dams and in offspring mice (Müller et al., Reference Müller, Aguado-Martinez, Ortega-Mora, Moreno-Gonzalo, Ferre, Hulverson, Choi, McCloskey, Barrett, Maly, Ojo, Van Voorhis and Hemphill2017b). Thus, BPQ could be potentially useful for the treatment of besnoitiosis when applied in combination with another highly effective compound that exerts another mechanism of action.
Acknowledgements
We thank Helder Cortes (the University of Evora, Portugal), for providing the B. besnoitia isolate Bb1Evora, and Vreni Balmer and Rita Cardoso for excellent technical support.
Financial support
This study was financed by the Swiss National Science Foundation (AH, grant No. 310030_165782).
Conflicts of interest
None.
Ethical standards
Not applicable.