Introduction
Leishmaniases are neglected diseases caused by protozoa from the genus Leishmania, affecting individuals from 98 countries, with an estimate of 1·1 to 1·7 million cases of leishmaniasis occuring each year (Alvar et al. Reference Alvar, Velez, Ber, Herrero, Desjeux, Cano, Jannin and Den Boer2012). Leishmaniasis presents a wide-spectrum of clinical manifestations, such as mucocutaneous, cutaneous or visceral disease (Pace, Reference Pace2014).
Currently, drugs used for the treatment of leishmaniasis rely on pentavalent antimonials, amphotericin B (AmpB), miltefosine, pentamidine and paromomycin, which are limited, highly toxic and expensive. Antimonials are still the first line of treatment choice, but in case of therapeutic failure due to resistance cases as in India or French Guiana, AmpB and pentamidine have been used as alternatives, respectively. Miltefosine, the only oral drug available for visceral leishmaniasis treatment in India, is teratogenic and parasite resistance has also been reported (De Menezes et al. Reference De Menezes, Guedes, Petersen, Fraga and Veras2015; Singh et al. Reference Singh, Garg and Ali2016). Therefore, more attention has been given to active extracts and molecules isolated from plant species used in folk medicine as possible sources for developing new drugs to control and prevent leishmaniasis (Sen and Chatterjee, Reference Sen and Chatterjee2011; Ullah et al. Reference Ullah, Nadhman, Siddiq, Mehwish, Islam, Jafri and Hamayun2016).
Essential oils (EOs) are natural and complex mixtures of compounds obtained from different plants parts, such as flowers, leaves, fruits, roots or seeds, commonly used in folk medicine to treat different types of diseases (Costa, Reference Costa1994). In general, EOs are constituted of monoterpenes and sesquiterpenes compounds and some are reported to be active against Leishmania (Kvist et al. Reference Kvist, Christensen, Rasmussen, Mejia and Gonzalez2006; Escobar et al. Reference Escobar, Leal, Herrera, Martinez and Stashenko2010; Medeiros et al. Reference Medeiros, da Silva, Cittó, Borges, Lima, Lopes and Figueiredo2011; Farias-Junior, et al. Reference Farias-Junior, Rios, Moura, Almeida, Alves, Blank, Fernande and Scher2012; Soares et al. Reference Soares, Portella, Ramos, Siani and Saraiva2013; Monzote et al. Reference Monzote, Piñón, Sculli and Setzer2014; Andrade et al. Reference Andrade, Azevedo, Motta, Santos, Silva, Santana and Bastos2016; Ceole et al. Reference Ceole, Cardoso and Soares2017; Moreira et al. Reference Moreira, Martins, Varandas, Cogo, Perego, Roncoli, Sousa, Nakamura, Salgueiro and Cavaleiro2017; Silva et al. Reference Silva, Scher, Santos, Ferreira, Cavalcanti, Correa, Bueno, Alves, Souza, Fujiwara and Dolabella2017).
Many plant species belonging to genus Lippia (Verbenaceae) are popularly used in the folk medicine in Northeast of Brazil for treatment of acne, scabies and skin wounds, and as oral antiseptic or liquid soap preparations to prevent and treat fungal infections (Lacoste et al. Reference Lacoste, Chaumont, Mandin, Plumel and Matos1996). An antiprotozoal effect has been found in an extensive analysis comprising 19 EOs from Lippia species, in which they presented microbicidal effect against Leishmania chagasi and Trypanosoma cruzi (Escobar et al. Reference Escobar, Leal, Herrera, Martinez and Stashenko2010). The anti-leishmanial activity of Lippia sidoides and its two major compounds thymol and carvacol against L eishmania amazonensis and L. chagasi has also been described (Oliveira et al. Reference Oliveira, Moura, Lopes, de Andrade, da Silva and Figueiredo2009; Medeiros et al. Reference Medeiros, da Silva, Cittó, Borges, Lima, Lopes and Figueiredo2011; Farias-Junior et al. Reference Farias-Junior, Rios, Moura, Almeida, Alves, Blank, Fernande and Scher2012).
Aloysia gratissima (Gillies and Hook) (Lippia lycioides Cham. Steudel) species, known as ‘Brazil lavender’, are commonly used in the Brazilian folk medicine for the treatment of digestive and respiratory diseases, and their flowers are employed in perfume compositions. Some reports have characterized the microbicidal effect of A. gratissima against bacteria, yeast and virus (Dellacasa et al. Reference Dellacasa, Bailac and Ponz2003; Garcia et al. Reference Garcia, Talarico, Almeida, Colombres, Duschatzky and Damonte2003; Santos et al. Reference Santos, Pinto, Bertolucci, Alvarenga, Alves, Duarte and Sartoratto2013, Reference Santos, Laemmle, Rebelo, Dalmarco, Cruz, Schmit, Cruz and Zeni2015). Guaiol is a sesquiterpene alcohol found in many medicinal plants, which have been proved to possess antibacterial and antitumor activities (Choudhary et al. Reference Choudhary, Batool, Atif, Hussain and Atta-ur-Rahman2007; Yang et al. Reference Yang, Wu, Luo, Huang, Zhen, Zhou, Sun, Li, Pan and Li2016).
Here we show for the first time the leishmanicidal activity of Aloysia gratissima essential oil (AgEO) and of its major sesquiterpene compound guaiol, on promastigotes and amastigotes of L. amazonensis in vitro. This Leishmania species is found along the Americas and is recognized as the causative agent of a broad spectrum of clinical manifestations that include cutaneous, anergic diffuse cutaneous and even visceral disease (Barral et al. Reference Barral, Pedral-Sampaio, Grimaldi Júnior, Momen, McMahon-Pratt, Ribeiro de Jesus, Almeida, Badaro, Barral-Netto, Carvalho and Johnson1991; Convit et al. Reference Convit, Ulrich, Fernández, Tapia, Cáceres-Dittmar, Castés and Rondón1993; Grimaldi and Tesh, Reference Grimaldi and Tesh1993).
Materials and methods
Ethics statement
All experiments using animals were performed in strict accordance with the Brazilian animal protection law of the National Council for the Control of Animal Experimentation (CONCEA, Brazil). Protocols were approved by the Committee for Animal Use of the Universidade Federal Rural do Rio de Janeiro (CEUA) number 22/14
Plant material
Leaves and branches of Aloysia gratissima (Gillies & Hook.) Tronc. (syn. Lippia lycioides Cham.) were collected in the Manguinhos campus of the Oswaldo Cruz Foundation, Rio de Janeiro located at S 22°52′46″, W 43°14′52″, 9 m above sea level. A voucher of A. gratissima was registered and deposited in the Herbarium of the Rio de Janeiro Botanical Garden Collection under the number RB 327302.
EOs extraction
Fresh aerial parts (leaves and branches, 1020 g) of Aloysia gratissima were hydrodistilled (2 L water) using a 12-L round bottom flask coupled to a modified Clevenger apparatus during 6 h, to afford a yellowish clear fluid (0·52% yield on fresh leaves). After separating an aliquot (10 mg) for gas chromatography (GC) analysis, this volatile oil was stored in sealed amber ampoules at −20°C until the biological tests could be performed.
Gas Chromatography (GC) analysis
Analyses were performed on a gas chromatograph (HP 6890N Network GC System) fitted with a 30 m × 0·32 mm × 0·25 µm film thickness HP-5 capillary column and operating in split mode at a ratio of 1:20 (split/splitless injector). Helium was used as carrier gas (flow: 1 mL min−1, inlet pressure: 5·83 psi). The initial oven temperature was 70 °C (held for 5 min). The temperature was then raised to 250 °C at 3 °C min−1. The injection volume was 1 µL of a 3 mg mL−1 solution of the oil in CH2Cl2. The sample composition was obtained from electronic integration measurements using flame ionization detection (FID, 270 °C) by considering the relative peak. Injections were carried out in triplicate and standard deviations were considered. Samples were also analysed on an HP 6890N GC mass spectrometry (MS) apparatus fitted using the same column and the responses were processed in a Mass Selective Detector (MSD) Productivity ChemStation Software. The chromatographic conditions were the same as above, except for the flow, which was 0·5 mL min−1. The mass analyzer operated at an ion source temperature of 250 °C, electron impact ionization energy of 70 eV and an acquisition mass range from 10 to 400 m/z (3·66 scan s−1). Individual identification of the constituents was performed by comparison of their GC retention indices determined with reference to a homologous series of normal C10-C30 alkanes, and comparison of the fragmentation patterns in the mass spectra with those from the Wiley Library Software 59943B (Adams, Reference Adams2007).
Parasites
Leishmania amazonensis (WHOM/BR/75/Josefa) promastigotes were cultured at 26 °C in Schneider's Insect Medium containing 10% fetal calf serum (FCS – Cripion), 10% human urine and gentamicin (40 µg mL−1; Schering–Plough, Rio de Janeiro, Brazil).
Leishmanicidal activity
Promastigote cultures (106 mL−1) were treated with different concentrations of the AgEO and its constituent (-)-guaiol [3R,6S,10S)-6,10,α,α-tetramethylbicyclo [5·3·0] dec-1(7)-ene-3-methanol, 97% Sigma-Aldrich] and viable parasites were counted daily in a Neubauer chamber.
Anti-amastigote activity was assayed in infected mouse macrophages as previously described (Ferreira et al. Reference Ferreira, Soares, Barreto-Junior, Nascimento, Freire–de-Lima, Delorenzi, Lima, Atella, Folly, Carvalho, Saraiva and Pinto-da-Silva2011; Soares et al. Reference Soares, Pereira, Meireles and Saraiva2007). Briefly, thioglycolate-stimulated macrophages from BALB/c mice cultured in RPMI 1640 (Biochrom KG, Germany) containing 10% FCS at 34 °C and 5% CO2 were infected with promastigotes at a 10:1 ratio for 1 h and cultured for 24 h as described above. Afterwards, the cultures were treated with AgEO or guaiol for 24 h, washed, fixed and Giemsa stained. The infectivity index (% of infected macrophages × average number of amastigotes per macrophage) was determined microscopically by counting at least 200 cells in duplicate cultures.
Assessment of parasite load
The infected macrophages incubated or not for 24 h with AgEO were washed and treated with 0·01% sodium dodecyl sulphate (SDS) for 10 min to allow the release of live amastigotes. The cultures were subsequently fed with 1 mL of Schneider's medium supplemented with 10% FCS and cultured at 27 °C for 2 days. The relative intracellular load of amastigotes was measured by the number of differentiated motile promastigotes (Soares et al. Reference Soares, Pereira, Meireles and Saraiva2007, Reference Soares, Andrade, Delorenzi, Silva, Freire-de-Lima, Falcão, Pinto, Rossi-Bergmann and Saraiva2010; Ferreira et al. Reference Ferreira, Soares, Barreto-Junior, Nascimento, Freire–de-Lima, Delorenzi, Lima, Atella, Folly, Carvalho, Saraiva and Pinto-da-Silva2011).
Macrophage cytotoxic assays
2,3-Bis-(2-methoxy-4-nitro-5-sulfophenyl]-2H-tetrazolium-5-carboxyanilide salt) (XTT) test
The thioglycolate-stimulated macrophages (2 × 105 well−1) from BALB/c mice adhered to 24 well plates were treated or not with AgEO or guaiol at the indicated concentrations, followed by incubation at 37 °C and 5% CO2 for 24 h. Afterwards, these cells were treated with XTT for 3 h as previously described (Soares et al. Reference Soares, Pereira, Meireles and Saraiva2007). Sodium azide was used as the positive control. The percentage of viable cells was determined towards the untreated cell cultures (100%).
Phagocytosis assay
The thioglycolate-stimulated macrophages were treated with different concentrations of AgEO at 37 °C and 5% CO2 for 24 h, washed and incubated with promastigotes at a 10:1 ratio at 34 °C and 5% CO2 for 1 h. Afterwards, the cultures were washed, fixed, stained with Giemsa and the percentage of phagocytosed parasites was determined microscopically by counting at least 200 cells in triplicates. The results were expressed as the percentage of phagocytosis determined towards the untreated control as described by Soares et al. (Reference Soares, Pereira, Meireles and Saraiva2007).
Trypan blue exclusion test
The thioglycolate-stimulated macrophages were treated with different concentrations of AgEO or guaiol at 37 °C and 5% CO2 for 24 h, followed by addition of 0·03% Trypan blue. Cell viability was calculated by counting at least 200 cells in triplicates (Ferreira et al. Reference Ferreira, Soares, Barreto-Junior, Nascimento, Freire–de-Lima, Delorenzi, Lima, Atella, Folly, Carvalho, Saraiva and Pinto-da-Silva2011).
Nitric oxide (NO) production
The thioglycolate-stimulated macrophages (106 cells well−1) were activated or not with 100 ng mL−1 of lipopolysaccharide (LPS, Escherichia coli O111:B4, Sigma) and/or murine interferon-γ [100 ng mL−1], and treated or not with 1 µg mL−1 of AgEO or guaiol. After incubation at 37 °C and 5% CO2 for 48 h, the nitrite concentration was determined by Griess method. The results are expressed as the mean ± s.e.m. of 3 independent experiments carried out in triplicates.
Promastigotes ultrastructural analysis
Promastigotes were treated at the IC50 concentration and with 50 µg mL−1 of AgEO, and guaiol was used at 50 µg mL−1, whereas the controls contained 0·2% dimethyl sulfoxide (DMSO). After 48 h, the cells were fixed with 2·5% glutaraldehyde and 4% paraformaldehyde in 0·1 m sodium cacodylate buffer (pH 7·2), 5 mm calcium chloride and 3·7% sucrose. Promastigotes were then washed, post-fixed in 1% OsO4, 0·8% potassium ferricyanide and 5 mm calcium chloride, and further rinsed in the same buffer. After dehydration in acetone, parasites were embedded in PolyBed812 (Polysciences Inc., Warrington, PA, USA). Ultra-thin sections were collected on 300 mesh copper grids, stained with uranyl acetate and lead citrate, then observed in a Field Electron and Ion (FEI) Morgagni F 268 (Eindhoven, the Netherlands) transmission electron microscope operating at 80 kV.
Statistical analysis
Data were analysed using the Student's t-test when comparing two groups or one-way ANOVA for more than two groups, using the GraphPad Prism 5.0 software; P < 0·05 was considered significant.
Results
Aloysia gratissima leaves essential oil (AgEO) was analyzed by GC-FID and its constituents were characterized by GC-MS (Table 1). The alcohol guaiol (Fig. 1) was identified as the major sesquiterpene in the oil (10·5%).
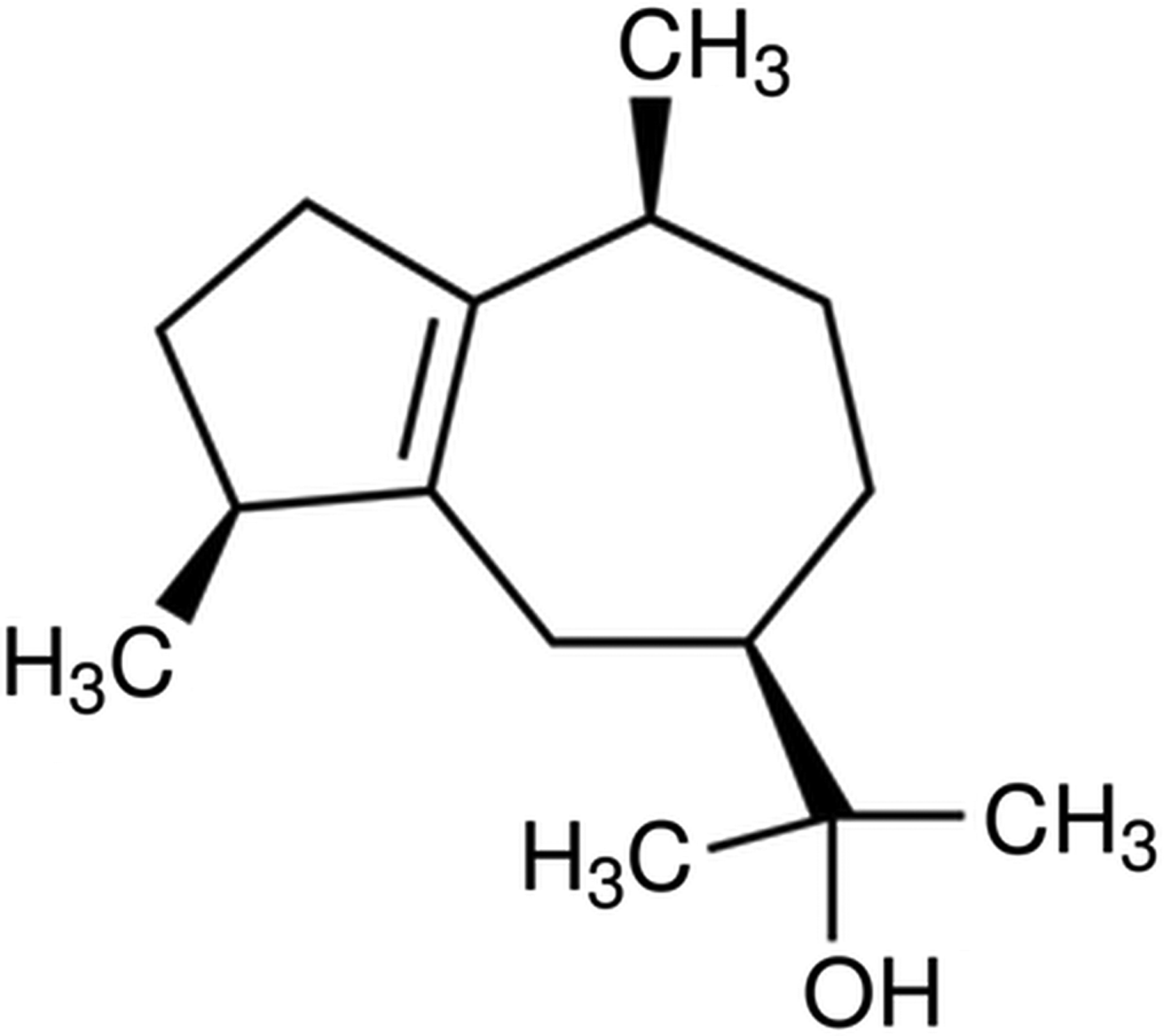
Fig. 1. Chemical structure of guaiol.
Table 1. Chemical composition of Aloysia gratissima essential oil

RI, retention index. See Adams (Reference Adams2007).
aValues from the relative peak area in the chromatograms.
Initially, we evaluated the AgEO toxicity for L. amazonensis assessing promastigotes growth. Our results demonstrated that AgEO affected promastigote growth in a dose-dependent manner. Inhibitions of 31%, 44% and 85% of parasite growth was obtained after 72 h post-treatment with 1, 10 and 100 µg mL−1 of AgEO, respectively. The IC50 at 48 h and 72 h to compound exposure were 25 and 14 µg mL−1, respectively (Fig. 2).

Fig. 2. Inhibition of Leishmania amazonensis promastigote proliferation by Aloysia gratissima essential oil (AgEO). Parasites (106 mL−1) were treated or not with the indicated concentrations of AgEO or 1% DMSO (vehicle control). Anti-promastigote activity was determined by counting viable parasites in a Neubauer chamber at 48 and 72 h post-treatment. Results are expressed as a percentage of growth inhibition + standard error of the mean (s.e.m.) of 3 independent experiments. *P < 0·05 in relation to untreated control.
To evaluate the toxicity of AgEO to host macrophages, these cells were treated with different concentrations of AgEO and the dehydrogenase activity was assessed by the XTT method. AgEO was nontoxic to peritoneal macrophages at the tested concentrations (Fig. 3A). In the same way, AgEO did not affect the membrane integrity, as assayed by the Trypan blue exclusion test (Fig. 3B). Moreover, AgEOs did not inhibit the macrophage phagocytic ability when compared with 1% DMSO vehicle. Interestingly, around 50% increase in the macrophage phagocytic capacity was observed after treatment with AgEO (Fig. 3C).

Fig. 3. Toxicity of Aloysia gratissima essential oil (AgEO) for murine peritoneal macrophages. (A) Adhered peritoneal macrophages were treated for 24 h with 1% DMSO (vehicle), or AgEO at the indicated concentrations and cell viability was evaluated by the XTT assay. Sodium azide (1%, Azide) was used as positive control. Data are expressed as mean +s.e.m. from three independent experiments in triplicate. *P < 0·05. (B) Macrophages treated as above and the cell viability was evaluated by Trypan Blue exclusion assay. Data are expressed as mean +s.e.m. from three independent experiments in triplicate. (C) Macrophages treated as above were incubated with promastigotes at 10 parasites: 1 macrophage ratio for 1 h, washed, fixed and the phagocytic index calculated in relation to untreated controls (100%). Data are expressed as mean +s.e.m. from three independent experiments in triplicate. **P < 0·01
Amastigotes are responsible for the maintenance of infection in vertebrate hosts. Hence, the effect of AgEO on intramacrophage amastigotes was investigated. Our data showed that AgEO induced a significant decrease in the parasite survival index when compared with untreated control, with inhibition rates of 35·5%, 50% and 61% at 0·01, 0·1 and 1 µg mL−1 of AgEO, respectively. The IC50 found for amastigotes was 0·16 µg mL−1 (Fig. 4A). To further confirm the anti-amastigote effect, cultures were assayed in conditions to allow the AgEO-surviving amastigote to differentiate into promastigotes. We found an 85% reduction in the amastigote intracellular load at 2 µg mL−1 of AgEO, similar to the reduction promoted by 1 µg mL−1 of amphotericin B (Fig. 4B).

Fig. 4. Anti-Leishmania amazonensis effect of Aloysia gratissima essential oil (AgEO) on amastigote-infected macrophages. (A) Infected peritoneal macrophages were treated with 1% DMSO (vehicle) or with AgEO at the indicated concentrations and amastigote survival assessed 24 h later. Results from at least four independent experiments done in triplicate are shown as percentage of inhibition of amastigotes survival in relation to vehicle-treated cells ± s.e.m.. *P < 0·05; ***P < 0·001. (B) Amastigote-infected mouse peritoneal macrophages treated with 2 µg mL−1 AgEO and after 24 h Schneider medium was added to the cultures. Parasite survival was assessed after 48 h by counting motile promastigotes. Amphotericin B (AMB 1 µg mL−1), was used as a positive control. Results from three independent experiments done in triplicate are shown as percentage of amastigotes killing in relation to vehicle (DMSO 1%) treated cells +s.e.m.. *** P < 0·001. (C) Effect of Aloysia gratissima essential oil (AgEO) on nitric oxide (NO) production. Murine peritoneal macrophages were activated or not with LPS [100 ng mL−1] and IFN-ɣ [100 ng mL−1] in the presence or not of AgEO at 1 µg mL−1. NO production was evaluated 48 h later by the Griess method. The results are expressed as nitrite [μM] and represent the mean of 4 experiments.*P < 0·05.
We also evaluated the ability of AgEO to modulate NO production, since this cellular signaling molecule is known to be highly effective against Leishmania. Our results demonstrated that 1 µg mL−1 AgEO did not affect NO production in resting macrophages and in lipopolisacaride (LPS)/Interferon-gama (IFN-γ) activated-macrophages as well (Fig. 4C).
The cytotoxicity of guaiol was likewise evaluated, and our results demonstrated its non-toxicity to macrophages up to 100 µg mL−1, as determined by Trypan blue exclusion (Fig. 5A) and XTT (Fig. 5B) assays.

Fig. 5. Toxicity of Guaiol for peritoneal macrophages. (A) Adhered macrophages treated or not with 1, 10 and 100 µg mL−1 guaiol, DMSO 1% (vehicle) for 24 h and cell viability was evaluated by the Trypan Blue exclusion assay. Data are expressed as the mean +s.e.m. of three independent experiments in triplicate. (B) Adhered peritoneal macrophages were treated with 100 µg mL−1 guaiol, DMSO 1% (vehicle) or 1% sodium azide (Azide) and cell viability was evaluated by XTT assay. Data are expressed as mean +s.e.m. from three independent experiments in triplicate. **P < 0·01.
Thereafter, we tested the guaiol activity against amastigotes, finding an inhibition of 39·65, 51·75 and 75·35% of amastigotes survival inside macrophages compared with untreated control at 0·001, 0·01 and 1 µg mL−1 of guaiol, respectively (Fig. 6A). Guaiol showed IC50 of 0·01 µg mL−1 against amastigotes. In addition, the antiamastigote activity observed was independent of NO, since the guaiol treatment of stimulated or unstimulated macrophages, at 1 µg mL−1, did not modulate NO production (Fig. 6B).

Fig. 6. Anti-amastigote effect of Guaiol. (A). Leishmania-infected BALB/c mouse peritoneal macrophages were treated with 1% DMSO (vehicle) and guaiol at the indicated concentrations and amastigote survival assessed 24 h later. Results from three independent experiments done in triplicate are shown as percentage of inhibition of amastigote survival in relation to vehicle-treated cells +s.e.m.. **P < 0·05. Amphotericin B (AMB 1 µg mL−1), was used as a positive control. (B) Effect of Guaiol on nitric oxide (NO) production. Murine peritoneal macrophages were activated or not with lipopolysaccharide (LPS) [100 ng mL−1] in the presence or not of guaiol at 1 µg mL−1. NO production was evaluated 48 h later by the Griess method. The results are expressed as nitrite [μM] and represent the mean of 4 experiments.***P < 0·001
The morphological alterations on AgEO and guaiol treated-promastigotes were analyzed by transmission electron microscopy (Fig. 7A–G). Control promastigotes exhibited a dense cytoplasm, where the lipid bodies, the nucleus with the characteristic heterochromatin (Fig. 7A) and the compact array of kDNA filaments and the flagellar pocket containing multiple vesicles can be observed (Fig. 7B). Parasites treated with 25 µg mL−1 AgEO showed an increase in the size of lipid bodies causing the rupture of the parasite membrane (Fig. 7C). Promastigotes treated with 50 µg mL−1 AgEO and guaiol presented mitochondrial swelling and a remarkable alteration in the kDNA network, with disorganization of the DNA filaments (Fig. 7D). Moreover, lipid bodies presenting irregular edges and electronic densities and budding of small vesicles from the cell body membrane could be observed (Fig. 7E). This treatment induced also an intense swelling of the mitochondrion and cytoplasmic compartments (Fig. 7F) and the appearance of long cell body membrane projections in the edge of the flagellar pocket (Fig. 7G).
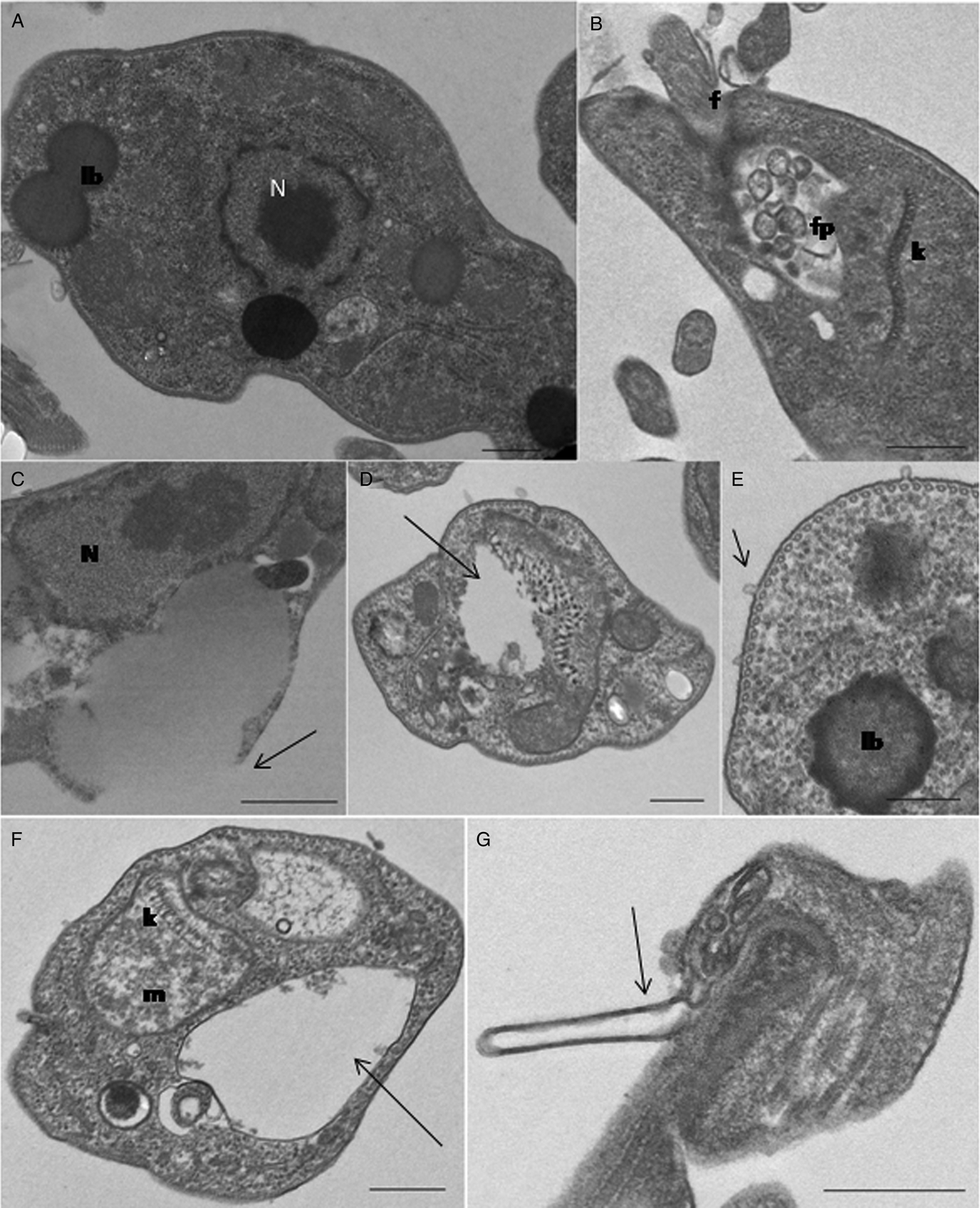
Fig. 7. Ultrastructural effects of AgEO and Guaiol on Leishmania amazonensis promastigotes. (A, B) Untreated parasites displayed the normal nuclear morphology (N), kinetoplast (k), lipid bodies (lb) and flagelar pocket (fp); (C-E) AgEO treated parasites with 25 µg mL−1 (C) and 50 µg mL−1 (D-E) swollen of the lipid bodies with the rupture of the plasma membrane (arrow in C), mitochondrial sewlling, altered kDNA network (arrow in D), the presence of lipid bodies showing irregular edges and the budding of small vesicles from the cell body membrane (small arrow in E); (F-G) Guaiol treated parasites showed the swelling of mitochondria and cytoplasmic compartments (arrow in F) and the presence of large projections of the cell body membrane (arrow in G). f, flagellum. Bars = 0·5 nm.
Discussion
Our data presented for the first time the leishmanicidal activity of Aloysia gratissima (Gillies and Hook) on L. amazonensis, a New World species that is the causative agent of cutaneous, diffuse cutaneous and visceral diseases. We demonstrated that AgEO is active against promastigotes by inhibiting their growth in a dose-dependent manner. Moreover, the AgEO effectiveness attained higher levels along the exposition time, given that, after a unique treatment, the IC5O value at 72 h was 1·8 times lower compared with the IC50 at 48 h. Importantly, the AgEO was also able to reduce amastigote survival inside infected macrophages in vitro. The amastigotes were unable to differentiate into promastigotes after AgEO treatment, as verified by the parasite load assay, thus confirming parasite damage by the EO usage. The IC50 value of AgEO for amastigotes was lower than that found for promastigotes, indicating that amastigotes are somehow more sensitive to the oil leishmanicidal effects. This finding is also relevant since amastigotes are responsible for the disease maintenance in the vertebrate hosts.
Microbicidal effects of AgEO have been demonstrated for bacteria, fungi and viruses (Dellacasa et al. Reference Dellacasa, Bailac and Ponz2003; Garcia et al. Reference Garcia, Talarico, Almeida, Colombres, Duschatzky and Damonte2003; Santos et al. Reference Santos, Pinto, Bertolucci, Alvarenga, Alves, Duarte and Sartoratto2013). AgEO obtained from leaves and flowers was bactericidal and fungicidal, although EO obtained from flowers was more effective against the Gram-negative P. aeruginosa, the Gram-positive S. pneumonia and the yeast C. albicans. These differences may be attributed to the higher amount of sesquiterpenes present in the flower EO (Santos et al. Reference Santos, Pinto, Bertolucci, Alvarenga, Alves, Duarte and Sartoratto2013). It has also been reported that AgEO is able to inhibit the growth of Junin virus and inactivate herpes simplex virus type 1 (Garcia et al. Reference Garcia, Talarico, Almeida, Colombres, Duschatzky and Damonte2003).
The composition of AgEO obtained by hydrodistillation was characterized by GC-MS. The main constituents are the monoterpene 1,8-cineole (17·6%) and the sesquiterpene alcohol guaiol (10·5%), along with other guaiol isomers (azulene-types structures, 7·3%) and hydrocarbons of the germacrene-types sesquiterpenes (total up to 17%). Additionally, trans-caryophyllene and its oxide (7% total) were also identified in the oil composition. A similar sesquiterpene constitution in the oil of A. gratissima leaves and flowers was recently described (Santos et al. Reference Santos, Pinto, Bertolucci, Alvarenga, Alves, Duarte and Sartoratto2013), although a distinct monoterpene composition was characterized. Other studies identified similar compounds as well as different proportions of guaiol in A. gratissima leaf oil such as 2·6% (Santos et al. Reference Santos, Pinto, Bertolucci, Alvarenga, Alves, Duarte and Sartoratto2013), 11·5% (Santos et al. Reference Santos, Laemmle, Rebelo, Dalmarco, Cruz, Schmit, Cruz and Zeni2015) and 12·5% of guaiol (Trovati et al. Reference Trovati, Chierice, Sanches and Galhiane2009). These differences can be attributed to diverse environmental conditions, including the type of soil, climate, plant age and the part of the plant used to obtain the oil (Velasco-Negueruela and Pérez-Alonso, Reference Velasco-Negueruela and Pérez-Alonso1993).
EOs from diverse plant species as well as isolated mono- and sesquiterpenes have been investigated for their antiprotozoal activity (Piątkowska and Rusiecka-Ziółkowska, Reference Piątkowska and Rusiecka-Ziółkowska2016; Sharifi-Rad et al. Reference Sharifi-Rad, Sureda, Tenore, Daglia, Sharifi-Rad, Valussi, Tundis, Sharifi-Rad, Loizzo, Ademiluyi, Sharifi-Rad, Ayatollahi and Iriti2017). Concerning the AgEO constituents, there are reports on the leishmanicidal activity of trans-caryophyllene (Soares et al. Reference Soares, Portella, Ramos, Siani and Saraiva2013), whilst the monoterpenes 1,8-cineole and sabinene showed to be biologically barely effective or ineffective at all (Mikus et al. Reference Mikus, Harkenthal, Steverding and Reichling2000; Leal et al. Reference Leal, Pino, Stashenko, Jairo, Martínez and Escobar2013; Camargos et al. Reference Camargos, Moreira, Mendanha, Fernandes, Dorta and Alonso2014; Machado et al. Reference Machado, Dinis, Santos-Rosa, Alves, Salgueiro, Cavaleiro and Sousa2014). EOs obtained from other Lippia species, having different compositions, have also been demonstrated to be toxic against L. amazonensis and L. chagasi promastigotes and amastigote forms (Oliveira et al. Reference Oliveira, Moura, Lopes, de Andrade, da Silva and Figueiredo2009; Medeiros et al. Reference Medeiros, da Silva, Cittó, Borges, Lima, Lopes and Figueiredo2011; Farias-Junior et al. Reference Farias-Junior, Rios, Moura, Almeida, Alves, Blank, Fernande and Scher2012). Thus, the L. sidoides EO resulted in an IC50/48 h of 44·38 µg mL−1 on inhibiting L. amazonensis promastigotes and an IC50/72 h of 89 µg mL−1 on L. chagasi promastigotes (Oliveira et al. Reference Oliveira, Moura, Lopes, de Andrade, da Silva and Figueiredo2009; Medeiros et al. Reference Medeiros, da Silva, Cittó, Borges, Lima, Lopes and Figueiredo2011). In our study, we found the IC50/48 h around 1·77 times lower for A. gratisima on L. amazonensis promastigotes, compared with L. sidoides.
Recently, a study with EOs from several plants demonstrated their toxicity for L amazonensis promastigotes, and guaiol (9·35%; 48·3%) was identified in Matricaria chamomilla and Bulnesia sarmientoi, respectively, although pure guaiol was not tested against the parasites (Andrade et al. Reference Andrade, Azevedo, Motta, Santos, Silva, Santana and Bastos2016). Once guaiol was the major sesquiterpene constituent identified in our leaf oil extract, we tested the capacity of this component to inhibit amastigotes survival inside infected macrophages, using a commercially available guaiol. The use of a compound already commercially available is cheaper, faster and interesting for future in vivo tests to find promising leishmanicidal compound. This is one of the recommendation of Drugs for Neglected Diseases initiative (DNDi), in order to facilitate the discovery of novel compounds for future treatment for neglected diseases. Our data established for the first time that guaiol inhibits amastigotes survival with IC50 of 0·01 µg mL−1 against amastigotes, suggesting that AgEO effects could be assigned to the major presence of guaiol in the oil constitution, although the effect of other similar sesquiterpenes (e.g. bulnesol and germacrenes) cannot be definitely excluded.
Since the AgEO was toxic to the promastigotes, we then evaluated its toxicity for macrophages and we found that the cells viability was not affected upon the higher concentration tested (100 µg mL−1) when evaluated by the dehydrogenases assay. Moreover, the macrophage membrane integrity was not affected by AgEO treatment at the higher concentrations tested, and the macrophage phagocytic capacity was even increased; together, these results pointed out to the selectivity of AgEO. Similar toxicity properties for host cells were found for guaiol
NO is a mediator involved in the leishmanicidal activity of macrophages and, thus, we analyzed whether AgEO or guaiol could modulate this mechanism. Our results indicated a NO-independent effect of both AgEO and guaiol suggesting a direct effect on the parasite. This possibility is strengthened by the ultrastructural alterations observed in parasites treated by both samples, as altered kinetoplast, loss of mitochondrial matrix, membrane rupture and altered lipid bodies. Chemical studies about microbial guaiol transformation using fermentation techniques with different species of fungi showed that guaiol can be converted in metabolites, which are toxic for several bacterium species (Choudhary et al. Reference Choudhary, Batool, Atif, Hussain and Atta-ur-Rahman2007). Thus, we can speculate that guaiol can be either directly metabolized by the parasites, generating toxic products or by host cells, hence generating leishmanicidal metabolites.
In conclusion, we report for the first time the leishmanicidal effect of EO from Aloysia gratissima and of its main sesquiterpene constituent, guaiol, against Leishmania amazonensis. Together our results could contribute to the establishment of new antiprotozoal compounds, aiming at treating leishmaniasis.
Acknowledgements
We thank Dr D. Chequer Bou-Habib for the critical review of the manuscript
Author contributions
MCG, DCS, TSP and RCS performed the experiments; LHP, DCS conceived and designed the experiments; MCG, DCS, EMS, ACS, MFSR and LHP analysed the data; MGMD, ACS, MFSR and TSP contributed with reagents/materials/analysis tools; MCG, DCS, EMS and LHP wrote the paper.
Financial support
This work was supported by Fundação de Amparo a Pesquisa do Rio de Janeiro Carlos Chagas Filho (FAPERJ) and Conselho Nacional de Pesquisa (CNPq). We are grateful to the Coordenação de Aperfeiçoamento de Pessoal de Nível Superior (CAPES) for the M. C. Freitas Garcia fellowship.
Conflict of interest
The authors declare no conflict of interest.