Introduction
Despite its profound health implications, chronicity and significant public health burden in developing countries, many aspects of human Necator americanus infection, particularly early events at the interface with the host immune system, are under-researched (Loukas and Prociv, Reference Loukas and Prociv2001; Quinnell et al. Reference Quinnell, Pritchard, Raiko, Brown and Shaw2004; Hotez et al. Reference Hotez, Brindley, Bethony, King, Pearce and Jacobson2008). These insidious parasites infect and re-infect, following which no efficient immunological memory develops in the host, rendering chemotherapeutic treatment as the method of choice, which is also inefficient due to the high prevalence of re-infection.
Efforts in developing more effective therapeutic approaches could be helped by a better understanding of the initial interactions between N. americanus larvae and key components of the innate immune system such as dendritic cells (DCs). DCs are sentinels of the immune system and act as a bridge between the innate and adaptive immune systems (Savina and Amigorena, Reference Savina and Amigorena2007). DCs are abundant in all barrier tissues (e.g. skin and airway epithelium) and equipped with a range of pattern recognition receptors (PRRs) on their surface [e.g. Toll-like and C-type lectin receptors (CLRs)] that can recognize various pathogen-associated molecular patterns (PAMPs) (Salazar et al. Reference Salazar, Sewell, Shakib and Ghaemmaghami2013). Interestingly previous studies have identified a range of lectins isolated from plants capable of binding to sugars present on the N. americanus L3 sheath, including mannose, fucose, heparan sulphate and galactose (Kumar and Pritchard, Reference Kumar and Pritchard1992a) which could potentially act as ligands for CLRs on DCs.
During its life cycle, N. americanus has many opportunities to interact with the host DCs (Quinnell et al. Reference Quinnell, Pritchard, Raiko, Brown and Shaw2004; Geiger et al. Reference Geiger, Caldas, Mc Glone, Campi-Azevedo, De Oliveira, Brooker, Diemert, Correa-Oliveira and Bethony2007). As part of their armoury of PRRs, DCs express a range of CLRs with specificity for the recognition of glycosylated proteins (Thompson et al. Reference Thompson, Kaminski, Kurt-Jones and Fitzgerald2011; Salazar et al. Reference Salazar, Sewell, Shakib and Ghaemmaghami2013). Amongst CLRs expressed by DCs are DC-specific intercellular adhesion molecule-3 grabbing non-integrin (DC-SIGN) and mannose receptor (MR), both of which have been implicated in key DC functions including mediating immune responses to different pathogens as well as immune modulation (Geijtenbeek et al. Reference Geijtenbeek, Engering and Van Kooyk2002; Wollenberg et al. Reference Wollenberg, Mommaas, Oppel, Schottdorf, Gunther and Moderer2002; Royer et al. Reference Royer, Emara, Yang, Al-Ghouleh, Tighe, Jones, Sewell, Shakib, Martinez-Pomares and Ghaemmaghami2010; Emara et al. Reference Emara, Royer, Abbas, Sewell, Mohamed, Singh, Peel, Fox, Shakib, Martinez-Pomares and Ghaemmaghami2011; Emara et al. Reference Emara, Royer, Mahdavi, Shakib and Ghaemmaghami2012; Salazar et al. Reference Salazar, Sewell, Shakib and Ghaemmaghami2013; Garcia-Vallejo and van Kooyk, Reference Garcia-Vallejo and Van Kooyk2013). However, the biological relevance of the glycosylated N. americanus sheath in the context of interaction with CLRs on DCs has not been investigated.
The antigen presenting cell function of DCs directly depends on their ability to migrate to the site of infection (Martin-Fontecha et al. Reference Martin-Fontecha, Lanzavecchia and Sallusto2009). Upon capturing pathogens, DCs migrate to lymph nodes where processed antigens are presented to naïve T cells, in the context of MHC molecules, leading to polarization of T cells towards distinct functional subsets such as Th1, Th2, Th17 and regulatory T cells (Smith-Garvin et al. Reference Smith-Garvin, Koretzky and Jordan2009). Many microorganisms have developed strategies (e.g. masking of PAMPs or inducing changes in PRR expression) to evade efficient recognition by DCs (van Kooyk and Geijtenbeek, Reference Van Kooyk and Geijtenbeek2003). In addition, any physical or chemical barriers that interfere with DC migrating to or from the site of infection could also hamper mounting appropriate immune responses. This function is indispensable for the maintenance of immune surveillance and tissue homeostasis as well as initiating protective tolerogenic and pro-inflammatory responses (Imai et al. Reference Imai, Minamiya, Koyota, Ito, Saito, Sato, Motoyama, Sugiyama and Ogawa2012).
In this study, we have investigated the cross-talk between human DCs and N. americanus larvae, in particular, the biological significance of the glycosylated molecules on the N. americanus L3 sheath in influencing DCs function and their interaction with the N. americanus larvae.
Materials and methods
All materials were purchased from Sigma-Aldrich, UK, unless otherwise stated.
Preparation and identification of N. americanus (L3) larvae
Infective N. americanus larvae were cultured from fecal material derived from infected individuals as described previously (Kumar et al. Reference Kumar, Laouar, Pritchard and Lowe1992). The larvae were deemed to be axenic following microbiological analysis (FDAS, BioCity, Nottingham).
DC generation
Monocyte-derived DCs were generated from peripheral blood monocytes which were obtained from human blood buffy coats after obtaining written informed consent and approval of local Ethics Committee (National Blood Transfusion Service, UK, 2009/D055) as we have previously described (Chau et al. Reference Chau, Johnson, Macneil, Haycock and Ghaemmaghami2013). Briefly, the peripheral blood mononuclear cells (PBMCs) were isolated via histopaque density gradient centrifugation. Monocytes were then isolated out from the PBMC by incubating the suspension with CD14 + magnetic beads (Milteny Biotech, UK) obtaining a purity of >98% as we have described before (Garcia-Nieto et al. Reference Garcia-Nieto, Johal, Shakesheff, Emara, Royer, Chau, Shakib and Ghaemmaghami2010). Subsequently, purified CD14 + monocytes were cultured with complete RPMI medium (10% Fetal Bovine Serum, 2 Mm L-glutamine, 1% penicillin/streptomycin and 1% non-essential amino acid solution) supplemented with 50 ng mL−1 GM-CSF and 250 IU ml−1 IL-4 in a 24-well plate for a period of 6 days to generate immature DCs (Salazar et al. Reference Salazar, Hall, Negm, Awuah, Tighe, Shakib and Ghaemmaghami2016).
N. americanus (L3) incubation with DCs
Immature DCs were cultured in complete RPMI media (10% Fetal Bovine Serum, 2 Mm L-glutamine, 1% Penicillin/Streptomycin and 1% non-essential amino acid solution) and incubated with approximately 50 ensheathed N. americanus L3 larvae for 24-h. During the incubation period, the samples were imaged using the ZOE™ Fluorescent Cell Imager (Biorad).
CLRs blocking experiments
To assess the potential involvement of specific CLRs in DC–hookworm interaction, immature DCs were treated with either 20 µg mL−1 of blocking antibodies for DC-SIGN (clone H-200) and MR (clone 15.2) or mannan (from Saccharomyces cerevisiae) (100 µg mL−1) for 25 min at 37 °C prior to addition of approximately 50 N. americanus larvae. The cells were then incubated for a further 24-h at 37 °C/5% CO2 and the samples were imaged using the ZOE™ Fluorescent Cell Imager. This was compared to DCs treated with the appropriate isotype controls (rabbit IgG and normal mouse IgG1) and DCs which were untreated; both conditions were also incubated with the infective larvae. All antibodies purchased from Santa Cruz Biotechnology.
N. americanus (L3) incubation with conditioned DC media
To assess the effect of DCs secretions on larvae ex-sheathing, DCs were stimulated with either mannan (100 µg mL−1) or lipopolysaccharide (LPS) (100 ng mL−1) from Escherichia coli for 24-h at 37 °C/5% CO2. The cell-free conditioned supernatant was added to approximately 50 N. americanus larvae for 24-h. Following the incubation period, the samples were imaged using an inverted Microscope (Olympus CKX41, Olympus America) and analysed with Lumenera Infinity Capture software. This was compared with the supernatant from untreated DCs incubated with the infective larvae.
Staining for cell surface markers and quantifying DC viability
In order to prepare the DCs for phenotype analysis via flow cytometer, the cells were harvested and washed twice in PBA buffer (5% albumin solution from bovine serum, 0.1% sodium azide in PBS). The desired antibodies (e.g. CD11c, CD14 and CD83) were added to the pellet, vortexed and incubated for 20 min, in dark at 4 °C. Nonreactive isotype-matched antibodies and unstained cells were used to determine non-specific staining. The samples were then washed with PBA and finally fixed with paraformaldehyde solution (0.5% in PBS). This was stored at 4 °C to be analysed within a 7-day period.
The viability of DCs was analysed using the ANNEXIN V– FITC Kit-Apoptosis Detection Kit (Beckman Coulter) according to the manufacturer's protocol. Expression of surface markers and the level of Annexin-V and propidium iodide (PI) expression in DCs were assessed via flow cytometery analysis (Cytomics FC 500, Beckman Coulter) with a minimum of 20 000 events collected for each sample. The data obtained were analysed using the Weasel V.2.7.4 software. Median fluorescence intensity and percentage of positive cells for each marker were determined and further evaluated using GraphPad Prism 6 analysis software.
Cytokine expression
The levels of cytokines were measured with ELISA kits and were analysed according to the manufacturer's protocol. All samples were analysed in duplicates. Absorbance was measured at 450 nm with SpectraMax Paradigm. IL-1β (200-01B), TNFα (900-K25), IL-10 (900-K21), IL-12 (900-K96) and IL-6 (900-K16) were purchased from PeproTech and IL-8 (DY208) from R&D Systems.
Statistical analysis
The means ± s.e.m. are shown. The statistical significance of the data was analysed and evaluated using Student's t-test with GraphPad Prism 6 analysis software. Statistical significance was determined using the Holm–Sidak method with a P value of ⩽0.05.
Results
Ex-sheathing of N. americanus (L3) larvae upon co-culture with immature DCs
To assess the consequence of a physical interaction between DCs and N. americanus, immature DCs were incubated with 50 N. americanus L3 larvae. The interactions were imaged and monitored using the ZOE™ Fluorescent Cell Imager (BioRad) for up to 24-h. Microscopy data showed that upon contact with the larval sheath, DCs are sequestered on the surface of the larval sheath which in turn triggered ex-sheathing, whereby the larvae discarded their outer cuticle. Ex-sheathing in this study is defined by the initial breach and emergence of the larva from its sheath. This phenomenon is observed at variable intervals after a minimum of 1 h incubation with DCs and complete ex-sheathing (i.e. full-length larva leaving the cuticle) could take up to 4 h. Notably, DCs in direct contact with the sheath seems to attract a majority of bystander cells leading to the formation of large DC aggregates and sequestration of DCs around the sheath, alluding to an adhesion cascade. Upon full ex-sheathing the larvae migrate away from the sheath with the sequestered DCs, as well as free DCs, exhibiting negligible interaction with the ex-sheathed larvae. The ex-sheathing of a single hookworm was examined, documenting this novel interaction (Fig. 1) (Supplementary Video 1).
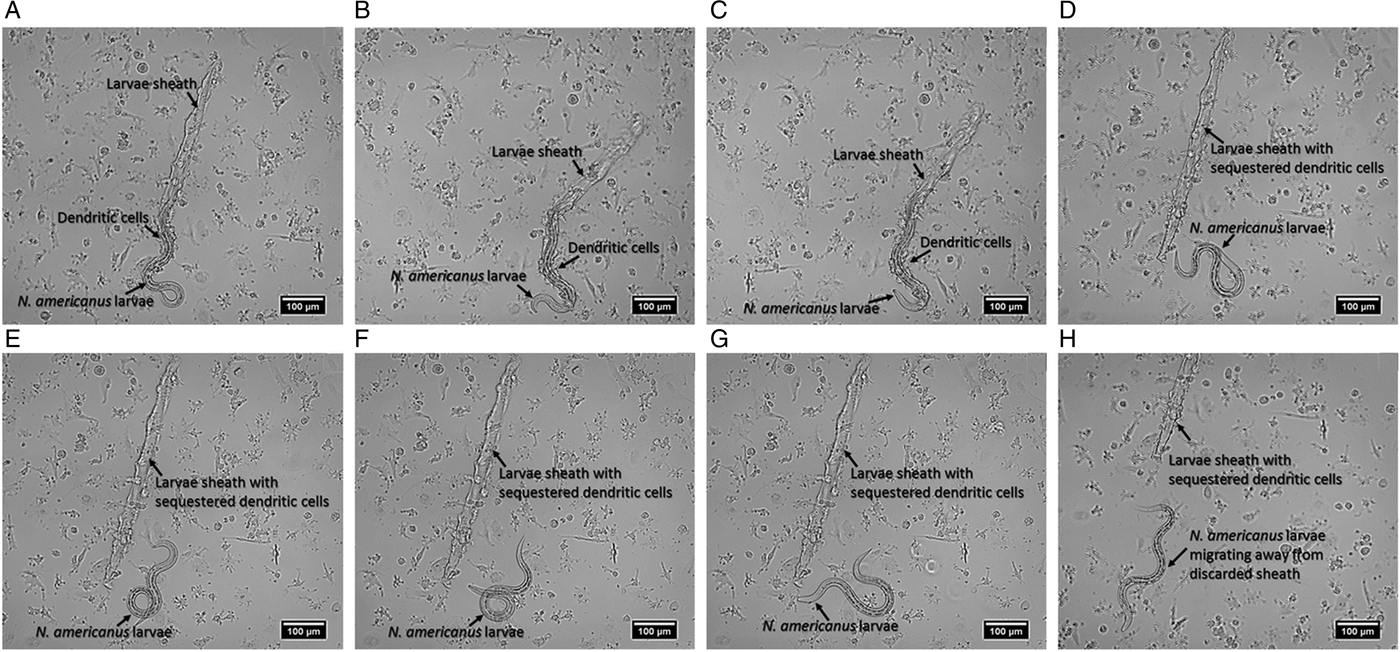
Fig. 1. The mechanical interaction of immature DCs with Necator americanus. The sheathed larva remains dormant during the initial incubation period where DCs are sequestered onto its sheath (up to ~1 h). Subsequently, the ensheathed larva begins to move dynamically until it finally breaches its sheath (A). Once the larvae begin ex-sheathing, the process occurs within seconds as the sheathed hookworm exits the cuticle sheath swiftly. For this individual larva ex-sheathing was initiated at the 4-h time point. At the first sight of ex-sheathing, images were captured sequentially with 10 s intervals (B: 10 s; C: 20 s). The ex-sheathed hookworm progressively migrates away from its discarded cuticle sheath with sequestered DCs (H). Images are representative of three independent experiments using DCs from three different donors and three larvae batches.
Following 24 h, DCs form dense aggregates around the sheath and remain un-attracted to the exposed larvae. Despite highly dense cell aggregates surrounding discarded sheaths, they can still be visualized within some aggregates (Fig. 2).
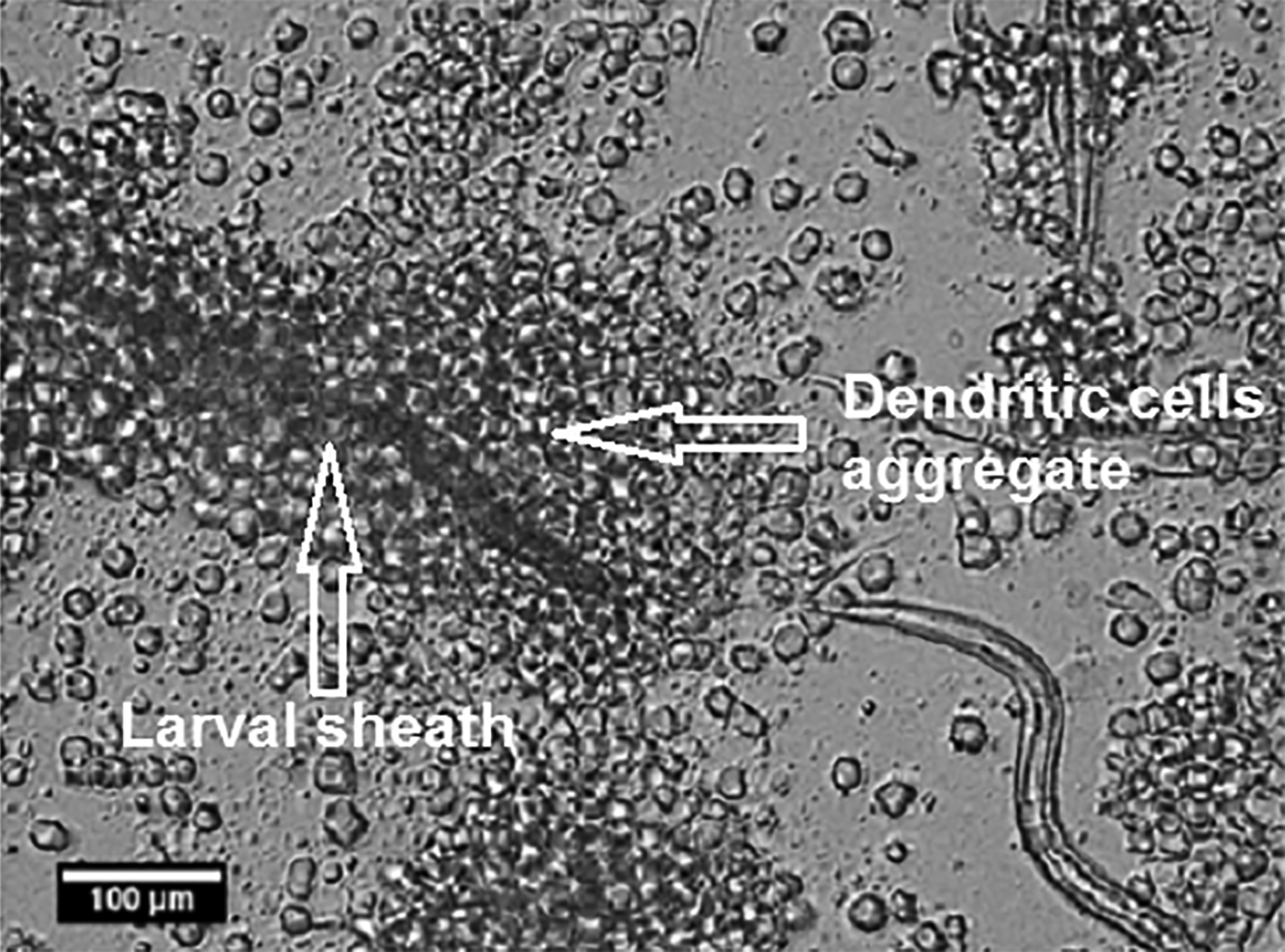
Fig. 2. Dendritic cell sequestration around Necator americanus larvae sheath. Following 24-h majority of DCs form dense aggregates around the discarded larval sheath. Data show representative images of six independent experiments.
Blocking CLRs on DC surface and N. americanus (L3) treatment with conditioned media
Previous research has distinguished N. americanus from other hookworm species based on its distinct glycosylated surface chemistry, which binds to a range of lectins derived from plants (Kumar and Pritchard, Reference Kumar and Pritchard1992a). It was, therefore, reasonable to assume that sugars on the worm sheath could mediate the interaction with DCs. To investigate this possibility, we blocked DC-SIGN and MR, two major C-type lectins expressed by human DCs, using specific blocking antibodies or mannan (to partially saturate MR and DC-SIGN) before incubation with N. americanus larvae for 24 h as described earlier. Our data clearly show almost complete abrogation of DC aggregation around N. americanus larvae in the presence of either α-DC-SIGN, α-MR or mannan compared with untreated DCs (Fig. 3a). In parallel, DCs treated with the respective isotype control antibodies were also examined which illustrated no changes in DC aggregation compared with untreated DCs (data not shown).

Fig. 3. The impact of blocking CLRs on DCs interaction with Necator americanus. (A) Microscopy data illustrate that DCs treated with either α-DC-SIGN, α-MR or mannan prior to incubation with N. americanus exhibit a significant decrease in aggregation following 24-h incubation with the larvae compared with untreated DCs where DCs form aggregates around larvae (also shown in Fig. 2). (B) Necator americanus larvae do not ex-sheath in culture with ‘cell-free’ conditioned media from DCs stimulated with mannan. Data show representative images of three independent experiments.
To elucidate whether soluble factors produced by DCs play a role in the observed ex-sheathing, DCs were stimulated with mannan (100 µg mL−1) (to simulate CLR ligation) and the conditioned media was collected after 24 h. The N. americanus larvae were then incubated with the conditioned media for 24 h as described. In parallel, media from unstimulated DCs were collected and incubated with the larvae as a control. The hookworms did not ex-sheath in culture with neither the conditioned media nor media collected from un-stimulated DCs, suggesting that a cell-mediated interaction is required for the induction of ex-sheathing (Fig. 3b).
DC surface phenotype in response to N. americanus (L3)
To better understand the effect of N. americanus larvae on DCs function we assessed DCs phenotype after 24 h incubation with N. americanus larvae. In control cultures, DCs were stimulated with 100 ng mL−1 LPS to induce maturation. In this study, we report that DCs retrieved from co-culture with viable axenic larvae maintained an immature phenotype as evidenced by a lack of up-regulation in maturation markers CD80, CD83, CD86, CD40 and HLA-DR. In addition, there was a significant downregulation in CD206 expression (Fig. 4). Subsequently, the ability of DCs to acquire a mature phenotype in response to co stimulation with LPS in the presence of N. americanus larvae was assessed. These data showed DCs treated with N. americanus larvae will remain responsive to LPS stimulation (Fig. 5). In all these experiments we monitored DC viability using Annexin-V and PI staining and did not observe any significant changes in DCs viability upon co-culture with N. americanus larvae (Fig. 6).

Fig. 4. Dendritic cells (DCs) maintain an immature phenotype upon interaction with Necator americanus. DCs were cultured in the presence of N. americanus larvae for 24 h followed by assessing the expression of co-stimulatory receptors/maturation markers CD40, CD80, CD83, CD86, CD206 (mannose receptor), CD209 (DC-SIGN) and HLA-DR using flow cytometry. Data show no changes in the expression of CD40, CD80, CD83, CD86 and HLA-DR compared with un-stimulated cells which are in line with an immature phenotype. While there are no changes in CD209 expression levels in response to N. americanus larvae, there is a significant downregulation in CD206 expression. Cells stimulated with LPS show an increase in the expression of maturation markers as expected. Data shown are mean values ± s.d. of three independent experiments using blood samples from three different donors.

Fig. 5. Necator americanus larvae do not modulate the dendritic cells (DCs) response to LPS stimulation. DCs were simultaneously stimulated with LPS and N. americanus larvae for 24 h followed by assessing the expression of co-stimulatory receptors/maturation markers CD40, CD80, CD83, CD86, CD206 (mannose receptor), CD209 (DC-SIGN) and HLA-DR using flow cytometry. Data indicate that DCs remain responsive to LPS stimulation when co-stimulated with N. americanus larvae. Data shown are mean values ± s.d. of three independent experiments using blood samples from three different donors.

Fig. 6. The viability of dendritic cells (DCs) post-treatment with Necator americanus larvae. DCs viability shows no significant changes after 24 h treatment with the N. americanus larvae with >80% viability quantified by measuring the expression levels of Annexi-V and propidium iodide (PI). Cells in the lower left quadrant are negative for both Annexin-V and PI which indicates viability. Data shown are representative of three independent experiments using blood samples from three different donors.
DC cytokine expression in response to N. americanus (L3) and LPS stimulation
Following the stimulation of DCs with either the infective L3 N. americanus larvae, LPS or both, supernatant samples were collected at 24 h and were analysed for IL6, 8, 10 and 12 using ELISA. Our data show that while axenic N. americanus larvae on its own do not induce any cytokine production by DCs, they seem to suppress LPS-induced cytokine production; however, these changes were not statistically significant (P value ⩽0.3) (Fig. 7).

Fig. 7. The cytokine profile of dendritic cells (DCs) in response to Necator americanus in the presence and absence of LPS stimulation. N. americanus infective larvae on their own did not induce production of selected pro (IL-6, IL-8, IL-12) or anti-inflammatory (IL-10) cytokines. However, cytokine production in response to LPS seems to be ameliorated when DCs are stimulated with LPS and N. americanus simultaneously. Such suppression was consistently observed in all cytokines tested but did not reach statistical significance expression (P value ⩽0.3 Data shown are mean values ± s.d. of three independent experiments using blood samples from three different donors.
Discussion
Infection with N. americanus has remained a major health problem with significant health implications. The high prevalence of reinfection, due to inefficient protective immunity, makes disease eradication a challenge. Therefore a better understanding of how the immune system interacts with infective larvae could pave the ways for the rational design of novel treatment strategies. This study provides new insights into early immunological events at the interface of human DCs and N. americanus larvae and could explain the lack of efficient immune response during the early stages of infection.
The ex-sheathing of N. americanus larvae has been observed during the initial stages of infection as well as in the presence of human sweat (Matthews, Reference Matthews1982; Hawdon et al. Reference Hawdon, Volk, Rose, Pritchard, Behnke and Schad1993; Pasuralertsakul and Ngrenngarmlert, Reference Pasuralertsakul and Ngrenngarmlert2006), however the factors initiating ex-sheathing and the benefit of this to the parasite are yet to be fully understood (Loukas and Prociv, Reference Loukas and Prociv2001). From the previous literature, it is known that the larval sheath does not accompany the hookworm post the skin stages of infection and progression into the blood circulation (Kumar and Pritchard, Reference Kumar and Pritchard1992b); implying the ensheathed larvae encounter immune cells in the skin in vivo.
Our data show for the first time that immature DCs bind to the ensheathed larvae, initiating the N. americanus to ex-sheath and mechanically migrate away from this site leaving behind its sheath. DCs are sequestered onto the discarded sheath and continue to form aggregates, exhibiting no interest in binding to the exposed larval cuticle allowing its unchallenged movement away from DCs. Migration of immature DCs from the site of infection to draining lymph nodes, where they interact with naïve T cells, is a key step in initiating an efficient immune response (Heuze et al. Reference Heuze, Vargas, Chabaud, Le Berre, Liu, Collin, Solanes, Voituriez, Piel and Lennon-Dumenil2013). Therefore, it is reasonable to suggest that DC sequestration on the larval sheath and no interaction between DCs and ex-sheathed larvae could play a major role in the lack of immune recognition/activation at early stages of infection.
The differential interaction between DCs and the larval sheath vs the larvae allude to a disparity between the surface chemistry of the sheath and the larvae. While the exact nature of differences in the surface properties of the larvae and sheath are yet to be fully characterized, these data clearly indicate the presence of distinct chemical signatures on the N. americanus sheath that attract DCs. Interestingly earlier work by authors has identified a range of sugars on the L3 sheath, including mannose, fucose, heparan sulphate and galactose (Kumar and Pritchard, Reference Kumar and Pritchard1992a). These sugars could be clear targets for a range of CLRs expressed on the surface of immature DCs that are part of DCs armoury of pattern recognition receptors (PRRs) (Thompson et al. Reference Thompson, Kaminski, Kurt-Jones and Fitzgerald2011).
Amongst the CLRs expressed by DCs are DC-SIGN and MR (or CD206) both of which have been implicated in mediating immune responses to different pathogens as well as immune modulation (Geijtenbeek et al. Reference Geijtenbeek, Engering and Van Kooyk2002; Wollenberg et al. Reference Wollenberg, Mommaas, Oppel, Schottdorf, Gunther and Moderer2002; Salazar et al. Reference Salazar, Sewell, Shakib and Ghaemmaghami2013; Garcia-Vallejo and van Kooyk, Reference Garcia-Vallejo and Van Kooyk2013). Given the high expression of DC-SIGN and MR on myeloid DCs and their prominent role in recognition of different pathogens, we investigated their potential role in DCs interaction with N. americanus live larvae by blocking MR and DC-SIGN. Our data illustrate that DCs treated with blocking antibodies against DC-SIGN and MR or mannan (a ligand for both DC-SIGN and MR) (Salazar et al. Reference Salazar, Sewell, Shakib and Ghaemmaghami2013) exhibit a significant decrease in induction of larvae ex-sheathing and forming DC aggregates around the larval sheath, compared with untreated DC. These data clearly indicate a role for MR and DC-SIGN in mediating interactions between DC and the larval sheath. Additionally, the treatment of N. americanus larvae with conditioned media from DCs stimulated with mannan, a ligand for both MR and DC-SIGN, showed that the hookworms do not ex-sheath in response to cell-free conditioned media, proposing a cell-mediated interaction and that binding to CLRs on DC are necessary for induction of hookworms ex-sheathing.
Previous studies have shown the ability of some pathogens to subvert DC-SIGN function in order to evade immune detection and surveillance; these include viral pathogens, such as HIV-1 via gp120 protein, and non-viral pathogens including Mycobacterium tuberculosis (van Kooyk and Geijtenbeek, Reference Van Kooyk and Geijtenbeek2003; Ludwig et al. Reference Ludwig, Lekkerkerker, Depla, Bosman, Musters, Depraetere, Van Kooyk and Geijtenbeek2004). However, in the context of this study, the N. americanus uses interaction with both DC-SIGN and MR to sequester DCs onto its sheath. This could provide a plausible explanation for the poor T cell responses observed in vivo, as the sequestration of DCs on larval sheaths could prevent necessary DC migration to lymph nodes that is a prerequisite for developing an effective adaptive immune response (Martin-Fontecha et al. Reference Martin-Fontecha, Lanzavecchia and Sallusto2009). These data provide strong rationale for further experiments (e.g. targeting-specific lectins on larvae surface and/or silencing MR and DC-SIGN expression on DCs) (Royer et al. Reference Royer, Emara, Yang, Al-Ghouleh, Tighe, Jones, Sewell, Shakib, Martinez-Pomares and Ghaemmaghami2010; Emara et al. Reference Emara, Royer, Mahdavi, Shakib and Ghaemmaghami2012) in order to fully elucidate the cross-talk between the sugar moieties on larvae sheath and the CLRs on DCs.
Another interesting observation arising from these experiments was a significant reduction in MR expression upon co-culture with N. americanus larvae, while all the other tested surface markers did not change and DCs maintained an immature phenotype. The immature phenotype of DCs is perhaps a reflection of the axenic nature of larvae that are used in these experiments which is unlikely to be the case in vivo. Nevertheless, the downregulation of MR expression on DCs in co-culture with N. americanus is of interest and further highlights the presence of MR ligands on the larval sheath. This observation is in line with other studies in Schistosoma mansoni infection, revealing a novel pathway involving the internalization (and decrease in MR expression) of helminth-derived glycoproteins through the MR. This interaction has been shown to interfere with DC protein synthesis, conditioning DCs to support a Th2 phenotype differentiation (Everts et al. Reference Everts, Hussaarts, Driessen, Meevissen, Schramm, Van Der Ham, Van Der Hoeven, Scholzen, Burgdorf, Mohrs, Pearce, Hokke, Haas, Smits and Yazdanbakhsh2012).
To further investigate whether the larvae are able to modulate DC responses to other stimuli we also studied DC cytokine profile after co-stimulation with LPS from E. coli, which is likely to be present during any in vivo exposure. Interestingly, DCs co-cultured with N. americanus larvae maintained their ability to respond to LPS stimulation as evidenced by upregulation of maturation markers. Furthermore, our data clearly show a general suppression of LPS-induced cytokine (IL-6, IL-8, IL-10 and IL-12) production in the presence of larvae which was not due to any changes in DC viability. Although such suppression in cytokine production was not statistically significant (P value ⩽0.3), most likely due to a small number of donors, it is in line with the generation of non-immunogenic or poorly immunogenic DCs.
In summary, our data clearly suggest that the N. americanus larvae actively target DC-SIGN and MR on DCs that leads to DC sequestration on the surface of the larval sheath and unchallenged migration of unsheathed larvae enabling larvae to escape immune surveillance and potentially promote pathogen survival (Fig. 8). These data provide new insights into the early events at the interface of DCs and N. americanus larvae which could pave the way for the rational design of new and more efficient intervention strategies against hookworm infection.
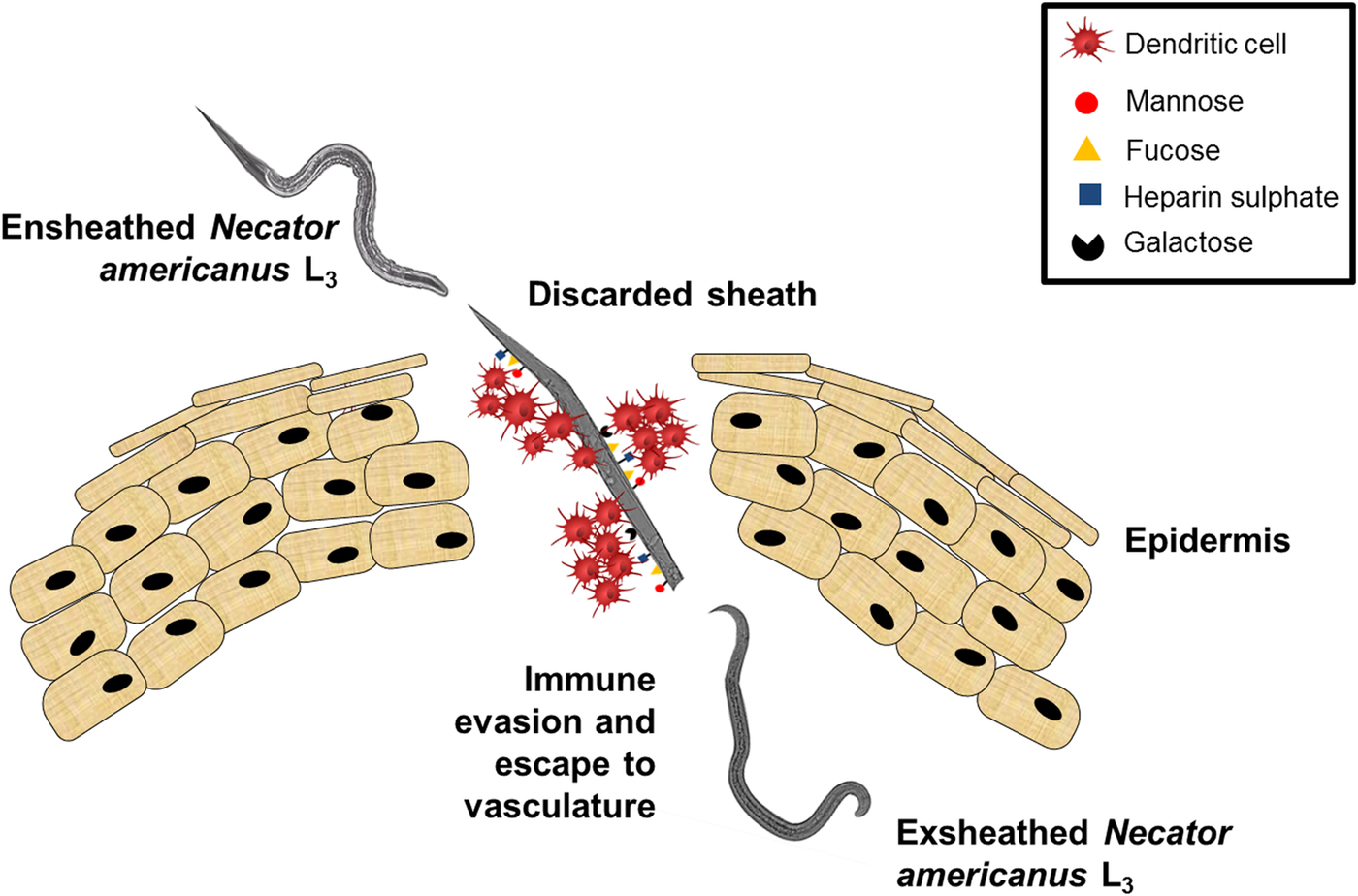
Fig. 8. The proposed mechanism of Necator americanus immune evasion strategy. Dendritic cells (DCs) bind the N. americanus sheath via CLRs which triggers the ex-sheathing of the larvae. DCs are then sequestered onto the discarded sheath and the larvae escape to the vasculature unchallenged.
Supplementary material
The supplementary material for this article can be found at https://doi.org/10.1017/S0031182018000136
Acknowledgements
Authors would like to thank Dr Gary Telford (School of Pharmacy, University of Nottingham) for preparing the infective L3 Necator americanus larvae.
Financial support
The production of the axenic larvae used in this work was supported by an MS Society grant.