Introduction
Ticks (Arachnida: Parasitiformes: Ixodida) are haematophagous ectoparasites found on a wide range of vertebrate hosts. For an overview of their biology and economic significance as disease vectors, see Sonenshine and Roe (Reference Sonenshine and Roe2013). About 900 living species are known (Guglielmone et al. Reference Guglielmone2010), divided across three families and 18 extant genera (Table 1). Molecular data (Mans et al. Reference Mans2012) suggest that the group may have originated during the Carboniferous, but their fossil record is sparse and restricted to deposits dating to the Late Cretaceous or younger. For recent summaries, see Dunlop et al. (Reference Dunlop2016), Chitimia-Dobler et al. (Reference Chitimia-Dobler2017) and Peñalver et al. (Reference Peñalver2017). An extinct family and genus (Deinocrotonidae: Deinocroton Peñalver et al. Reference Peñalver2017) – possibly related to Nuttalliellidae – was described recently. The oldest hard ticks (Ixodidae) are examples of the extant genus Amblyomma CL Koch (1844) and the extinct genera Cornupalpatum Poinar and Brown (Reference Poinar and Brown2003) and Compluriscutula Poinar and Buckley (Reference Poinar and Buckley2008). All of these fossils come from the Late Cretaceous (ca. 99 Ma) Burmese amber outcropping in Myanmar.
Table 1. Summary of the 18 extant (and three extinct) tick genera based on Guglielmone and Nava (Reference Guglielmone and Nava2014) and Guglielmone et al. (Reference Guglielmone2014), indicating the oldest fossil record of each genus, molecular divergence estimates based on Mans et al. (Reference Mans2012), their typical modern hosts, the oldest potential fossil of a host taxon used by living members of this genus, plus suggested origination dates for these hosts based on molecular clock techniques
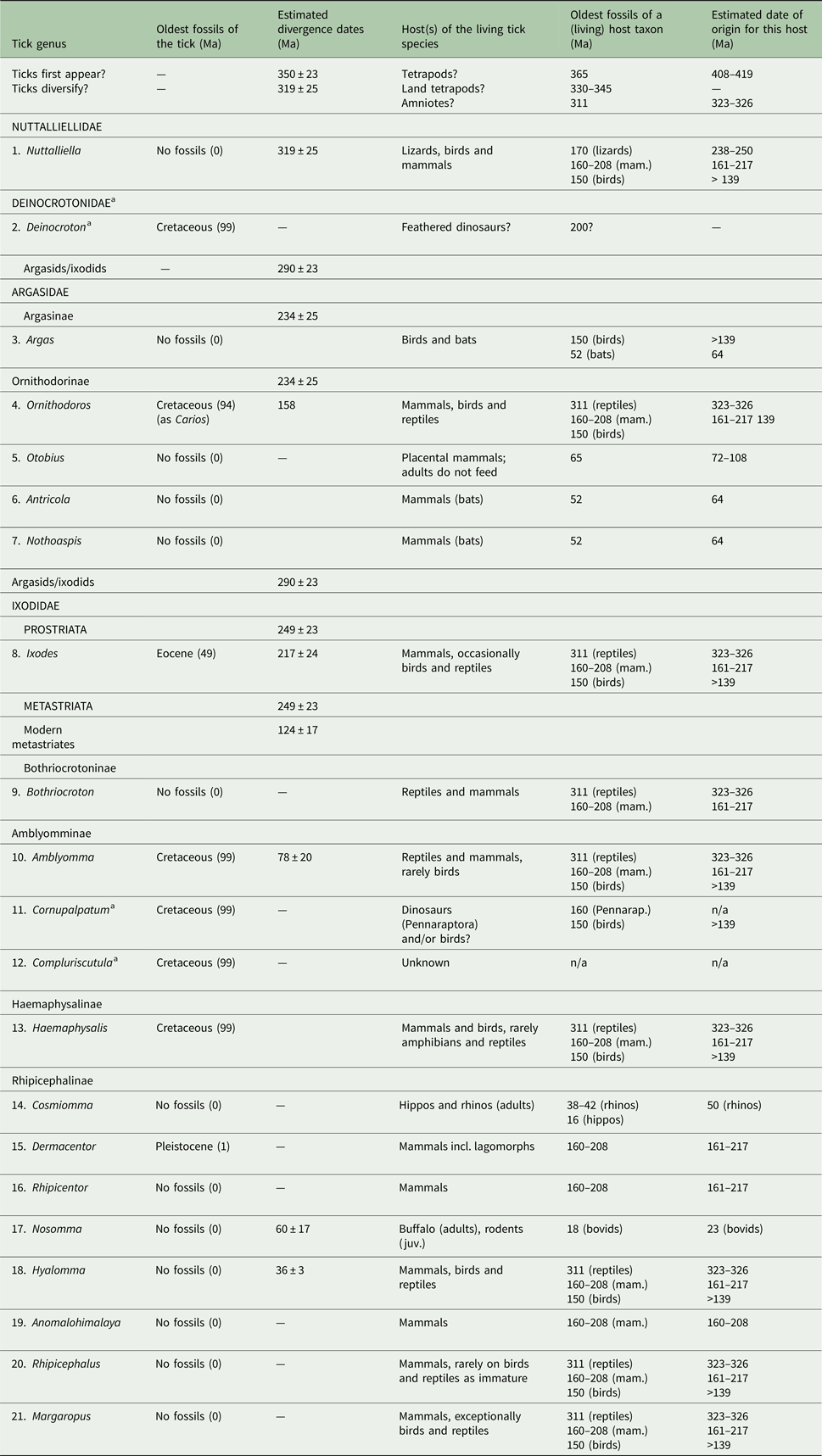
Phylogenetic sequence based on Barker and Murrell (Reference Barker and Murrell2002, Reference Barker and Murrell2004). See text for details.
aAn extinct genus.
Here we describe another Burmese amber tick (Figs 1–3): the first, and so far only, fossil potentially assignable to the extant genus Haemaphysalis CL Koch (1844). The new specimen is a well-preserved nymph that can probably be further placed in the subgenus Alloceraea Schulze (1919) and represents the fourth tick species found in this amber deposit. If this interpretation is correct, it draws another extant tick genus back into the Cretaceous, and is consistent with Mans et al. (Reference Mans2012) hypothesis that the so-called metastriate ticks, i.e. all hard ticks excluding Ixodes Latreille (1795) radiated in the Early Cretaceous. Modern species of Haemaphysalis usually parasitize birds and to a greater extent mammals (see ‘Discussion’ section). A Cretaceous record of this tick genus is thus of particular interest, given that both of their usual host groups would have been present (Brocklehurst et al. Reference Brocklehurst2012; Williamson et al. Reference Williamson, Brusatte and Wilson2014; see also ‘Discussion’ section), and may have been increasing in diversity towards the end of the Mesozoic. In this context, we also take the opportunity to review the tick fossil record compared with the fossil record of their (modern) host groups, together with molecular clock estimates for when both the parasites and their hosts are thought to have originated.
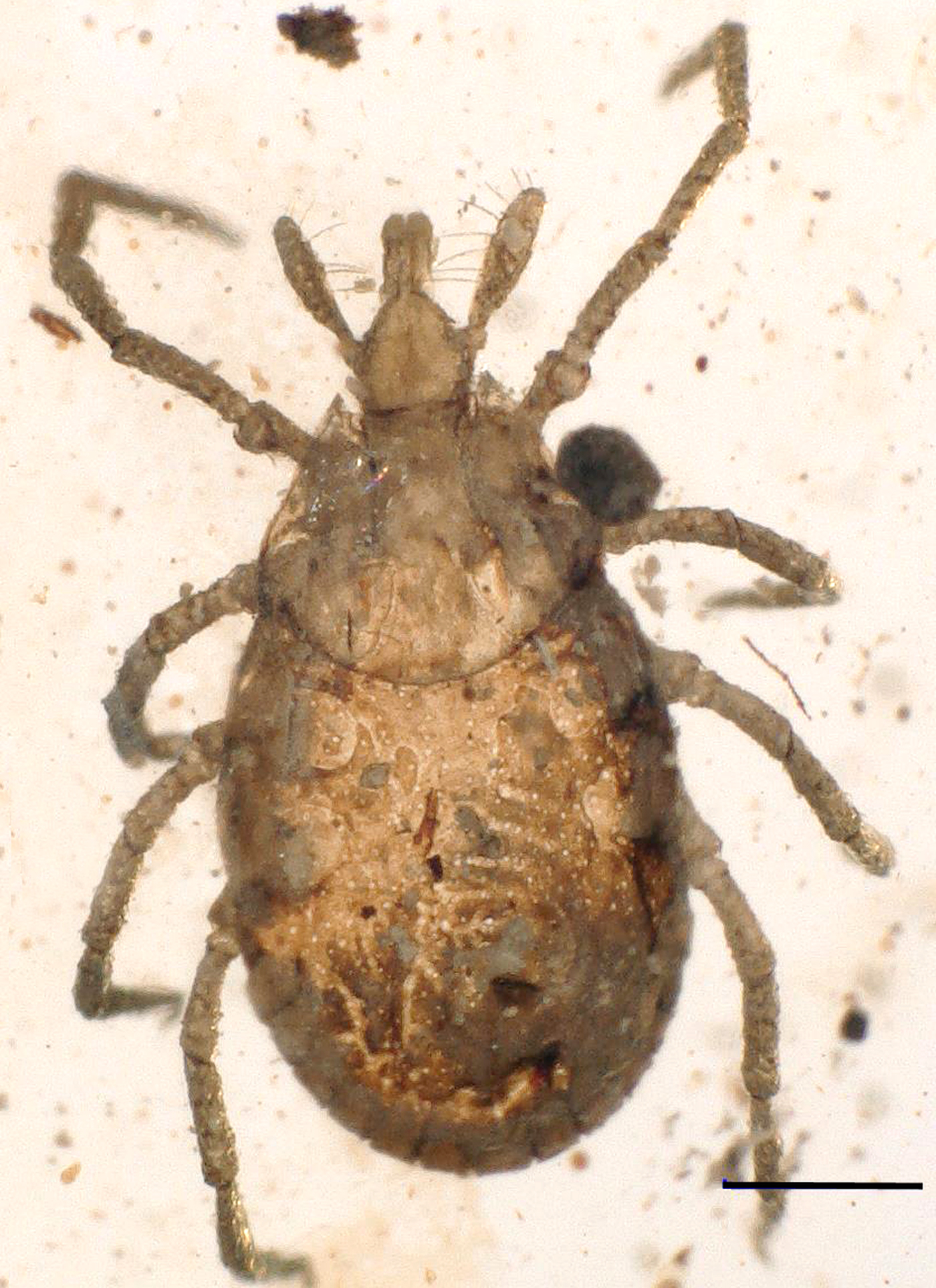
Fig. 1. Holotype of Haemaphysalis (Alloceraea) cretacea sp. nov., P. Müller collection no. BUB990, from Late Cretaceous (ca. 99 Ma) Burmese amber from Myanmar. Overview of dorsal surface. Scale bar equals 200 µ m.

Fig. 2. Camera lucida drawing of the specimen shown in Fig. 1. (A) Dorsal view. (B) Ventral view.
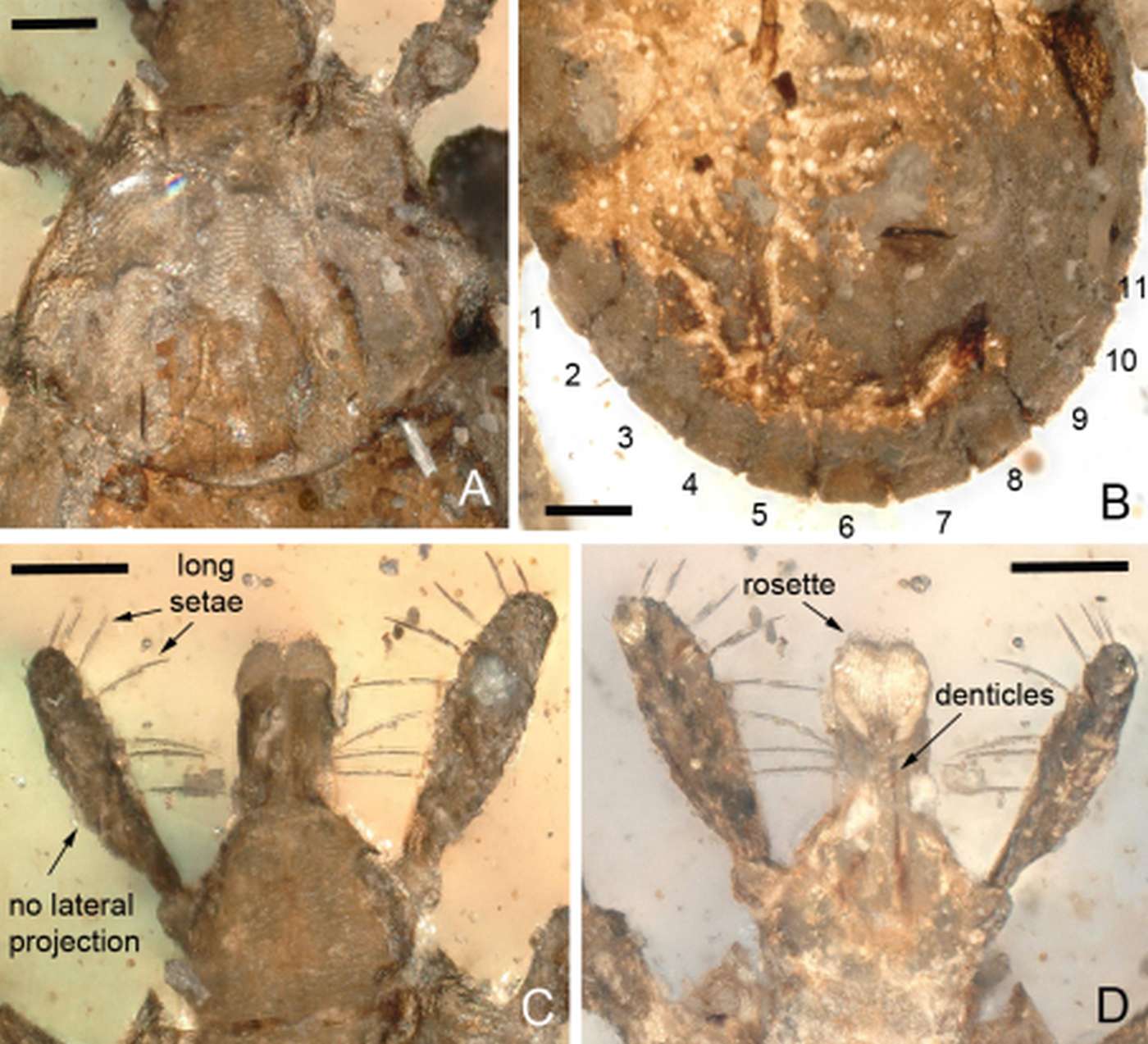
Fig. 3. Morphological details. (A) Dorsal scutum; note the absence of eyes. (B) Ventral idiosoma revealing 11 festoons. (C–D) Details of capitulum including basis capituli, palps and hypostome in dorsal (C) and ventral (D) views. Scale bars equal 100 µ m.
Material and methods
The type and only known specimen comes from the collection of Mr Patrick Müller and bears the inventory number BUB990. Burmese amber mostly comes from deposits in the Hukawng Valley of northern Myanmar and has been dated to the Late Cretaceous (earliest Cenomanian), or about 98.79 ± 0.62 Ma (Shi et al. Reference Shi2012). Further details about the history of discovery and the geological setting can be found in Grimaldi et al. (Reference Grimaldi, Engel and Nascimbene2002) and Ross et al. (Reference Ross and Penney2010); see also Selden and Ren (Reference Selden and Ren2017) for a recent review focused on the arachnids. A complete online list of Burmese amber inclusions can be found in Ross (Reference Ross2017). For photography, stack images were combined using the software Helicon Focus 6.7.1. A Keyence VHX-6000 Digital Microscope with a tiltable stand and a combination of upper light and transmitted light for focus stacking was used (with 100× to 1000× magnification). We partly used polarized light for more details. Drawings were prepared using a M205 C Leica stereomicroscope with a camera lucida attachment.
Systematic palaeontology
Ixodida Leach (1815)
Haemaphysalis CL Koch (1844)
Alloceraea Schulze (1919)
Haemaphysalis (Alloceraea) cretacea sp. nov.
Etymology: From the Cretaceous age of the fossil.
Material: Holotype and only known specimen, BUB990 (coll. P. Müller). Burmese amber, Myanmar, Late Cretaceous (Cenomanian).
Diagnosis: Body oval-elongate, scutum broader than long with margins broadly rounded, palpi elongate and clavate, 11 festoons, eyes absent, spiracle plates oval–elongate, coxae I–IV with small spurs, trochanter spurs lacking.
Description: Unengorged nymph (Fig. 1). Idiosoma: Ornamentation indistinct; body integument leathery; body oval–elongate; length (excluding capitulum) 1489 µ m, greatest width 884 µ m; scutum width 684.1 µ m (measured in the middle of the scutum) and 539.6 µ m (from the scapula to the edge); margins broadly rounded, no evidence for punctuations; scapulae acute-angled, resembling elongate, sharp prongs, cervical grooves deep, linear anteriorly and diverging posteriorly, can be traced along whole length of the scutum (Fig. 2); eyes absent (Figs 2A and 3A); 11 festoons ranging from 87 to 110 µ m in basal width and 73 to 87 µ m in length (Figs 2 and 3B); stigma oval–elongate with a narrower part dorsally and rounded macula located on the antero-inferior side (Fig. 2B); anus and anal groove not visible (Fig. 3B).
Capitulum: (Figs 3C, D): Length from apices to posterior margin of basis 406.6 µ m; basis capituli slightly wider (240 µ m) than long (216 µ m), posterior margin straight, lateral margins straight, cornua absent, ventrally posterior margin straight, length from palpal insertion to posterior margin of basis 83.7 µ m, width 239.6 µ m; palpi elongated and clavate, with length of four articles as follows: article 1, 49.8 µ m; article 2, 170 µ m – with external proximal side concave, while distal end of article 2 is noticeably wider, 1.6 times longer as the third article (but appears shorter in the figures due to the angle of view) – article 3, 106 µ m, without external spur; article 4 is in apical pit, visible ventrally; hypostome almost as long as palpi, length 182 µ m, width at base 97.7 µ m; dental formula equivocal, but with numerous denticles (almost 8–10 in a single file), distally a rosette is visible (Fig. 3B); porose areas absent (Fig. 3A). Legs: Short and robust (Figs 1 and 2). Coxae I with very small, short, widely triangular spur, located in the middle (Fig. 2B); coxae II with sub-basal triangular spur, located in the middle; coxae III and IV with small basal ridge spur, located externally; tarsus I tapering distally, length 378 µ m, clear, oval area on dorsum of tarsi I is Haller's organ; claws paired, slender, simple, slightly curved; with distinct pulvillus on all legs (Fig. 2).
Chaetotaxy: Eight long setae on palpi (Fig. 3C), four on the article 2 and four on the article 3 (the left article 2 has only three setae, probably one was broken). Small and tiny hairs can be observed on the legs.
Discussion
The presence in the new fossil of marginal festoons (Figs 1 and 2) rules out affinities with the recently described Burmese amber tick Deinocroton draculi Peñalver et al. (Reference Peñalver2017). The absence of eyes in our fossil (Fig. 3A) also excludes the Burmese amber species Amblyomma birmitum Chitimia-Dobler et al. (Reference Chitimia-Dobler2017). The absence of eyes matches the condition in the extinct Burmese amber species Compluriscutula vetulum Poinar and Buckley (Reference Poinar and Buckley2008). However, the pedipalps of C. vetulum are different from those in our new fossil; in C. vetulum, the fourth segment of the pedipalp is long and ends with elongate terminal setae and there are also 13 festoons on the body (not 11, cf. our Fig. 3B) (Poinar and Buckley, Reference Poinar and Buckley2008: Fig. 3). The other extinct Burmese amber tick, Cornupalpatum burmanicum Poinar and Brown (Reference Poinar and Brown2003), differs from our new fossil in that it has a unique extra claw on the penultimate (third) pedipalp article (Poinar and Brown, Reference Poinar and Brown2003: Figs 3 and 4).
Excluding Amblyomma (including Aponomma) and Bothricroton
The absence of eyes is also characteristic for species that used to be placed in the genus Aponomma Neumann (1899) and that are now included either in Amblyomma or Bothriocroton Keirans, King and Sharrad (1994) (e.g. Klompen et al. Reference Klompen, Dobson and Barker2002; Guglielmone et al. Reference Guglielmone2014). There are currently 14 extant Amblyomma species without eyes (Madder et al. Reference Madder, Horak and Stoltz2010; Schachat et al. Reference Schachat, Robbins and Goddard2018). However, unlike our fossil, species belonging to Amblyomma (either with or without eyes) invariably have a more circular body, a striated integument texture, straight lateral margins on the basis capitulum and unequal paired spurs on coxae 1. Kaufman (Reference Kaufman1972) described what used to be called the ‘indigenous Australian Aponomma’ ticks with the following character combination: a single subterminal spur on the trochanter (absent in all other Aponomma) and lateral grooves on the scutum of the male being partial or complete. These grooves are absent in ‘typical Aponomma’, A. elaphense Price (1959) and A. glebopalma, but present in A mblyomma sphenodonti Dumbleton (1943). These seem to be reliable phylogenetic markers. Other characters that may support ‘indigenous Australian Aponomma’ are a hypostomal dentition of 3/3 or 2/2; it is 3/3 or more in ‘typical Aponomma’, 2/2 in A. transversale Lucas (1845), 2/2 in A. elaphense and 3/3 but with the internal row almost gone in A. sphenodonti. Large wax glands laterally near setae s6 [Md3 of Clifford et al. (Reference Clifford, Anastos and Elbl1961)] and anterior to the first festoons (Klompen et al. Reference Klompen1996) found in the larvae are probably the most promising diagnostic characters for the ‘indigenous Australian Aponomma’. Overall, although the new fossil shares a similar palpal morphology with modern members of the subgenus Aponomma, it cannot be easily matched to any of these living ticks in which the body of all life stages is almost circular and never elongated like our amber example.
The absence of eyes and elongate palps are also characteristic for living Bothriocroton species. On the other hand, a subpentagonal basis capituli – it is subtriangular in Bothriocroton oudemansi nymphs (Beati et al. Reference Beati, Keirans and Durden2008) – coxae with two spurs in all instars and trochanters with a single subterminal ventral spur (absent in Bothriocroton glebopalma, cf. Klompen et al. Reference Klompen, Dobson and Barker2002) exclude the possibility that the new fossil belongs to Bothriocroton. It can be further excluded from Bothriocroton (in other words the Australian species placed in Aponomma; see above) as these ticks are generally large animals with a subcircular body and long mouthparts. Iridescent ornamentation on the scutum can be absent or present and the hypostomal dentition in Bothriocroton adults is 2/2 or 3/3. The coxae generally have two spurs in all instars and the trochanters have a single subterminal ventral spur. However, we should note that none of these characters is unique for ‘indigenous Australian Aponomma’ (Keirans et al. Reference Keirans, King and Sharrad1994; Klompen et al. Reference Klompen, Dobson and Barker2002; Beati et al. Reference Beati, Keirans and Durden2008).
Excluding Anomalohimalaya
Like our new fossil, living members of the genus Anomalohimalaya Hoogstraal, Kaiser and Mitchell (1970) have an elongate body, but the basis capituli is peculiar, the palps are shorter and there are many punctations on the scutum (Filippova, Reference Filippova1997), which make them quite different from our amber specimen. Filippova and Bardzimashvily (Reference Filippova and Bardzimashvily1992) discussed morphological differences between the nymphs of Anomalohimalaya. The Anomalohimalaya cricetuli nymph has a smooth, nitidous scutum with closely positioned lateral and cervical grooves, forming a narrow deep and short furrow, while in the other two species, the scutum is dull, the furrow between the lateral and cervical grooves is short and nearly reaches the posterolateral margins of the scutum. The shape and location of the lateral projections of the gnathosoma basis correspond to those of females. Filippova and Panova (Reference Filippova and Panova1978) concluded that Anomalohimalaya combines characters of Haemaphysalis and Rhipicephalus Koch (1844), and is thus potentially significant for a more precise definition of the phylogeny of the subfamily Amblyomminae; however, it does not appear to be relevant of the affinities of the new fossil.
Assignment to Haemaphysalis
We are confident that our new fossil belongs to a previously unrecognized species in Burmese amber, and that at least five distinct species of tick (four hard ticks, plus the extinct Deinocroton) were present in the original amber forest. Three apparently belonged to the extinct genera and, as we will argue here, the other two belonged to the living genera (Amblyomma and Haemaphysalis). A re-evaluation of relationships in the Metastriata by Klompen et al. (Reference Klompen1997) clearly showed that morphological analysis of the genus Haemaphysalis is complicated by the presence of plesiomorphic, so-called ‘structurally primitive’ species (see below). Their final conclusion was that A. sphenodonti and A. elaphense lack any of the characters unique to the derived Haemaphysalis species: e.g. the ‘blade-like dorsal retrograde process’ on trochanter I, palps projecting laterally over the basis capitulum or the presence of large wax glands mid-dorsal on segment XI in the larvae (Klompen et al. Reference Klompen1997).
We entertained the possibility of creating a new (extinct) genus for our fossil, but on balance we feel that it is better to assign the inclusion to the extant genus Haemaphysalis based primarily on a combination of: (1) an elongated body, (2) the absence of eyes, (3) the presence of festoons, (4) a dental formula with 8–10 denticles in a file, (5) lack of cornua, (6) the absence of trochanter spurs and (7) segment III of palps lacking a ventral spur and segment IV being an apical pit. Haemaphysalis ticks are generally small, inornate animals with short mouthparts: the brevirostra condition. The genus Haemaphysalis is further defined by specific morphological features, which we cannot resolve in the fossil like the presence of a prominent ‘blade-like’ dorsal retrograde process on trochanter I (Nuttall and Warburton, Reference Nuttall and Warburton1915).
Assignment to the subgenus Alloceraea
Most members of Haemaphysalis can also be recognized by the second palpal article being expanded and projecting laterally beyond the basis capitulum (Geevarghese and Mishra, Reference Geevarghese and Mishra2011). Hoogstraal and Kim (Reference Hoogstraal, Kim and Kim1985) studied Haemaphysalis and proposed a trend in the evolution of morphology within and among the subgenera from atypical and ‘primitive’ Amblyomma-like forms, such as the subgenus Alloceraea Schulze (1919) (Geevarghese and Mishra, Reference Geevarghese and Mishra2011), to the more typical Haemaphysalis-like forms. Hoogstraal and Kim (Reference Hoogstraal, Kim and Kim1985) thus graded the subgenera of Haemaphysalis into three groups: (1) the structurally primitive, (2) structurally intermediate and (3) structurally advanced species. In several species belonging to the ‘structurally primitive’ group, the expanded and laterally projecting second palpal article is not seen. This includes Haemaphysalis (Alloceraea) inermis Birula (1895) [also known as Allocerea inermis Birula (1895), A. inermis inermis Birula (1895), A. inermis aponomoides Warburton (1913)], H. ambigua Neumann (1901) and H. ibrikliensis Ozkan (1978) (Feider, Reference Feider1965; Guglielmone and Nava, Reference Guglielmone and Nava2014).
Other structurally primitive species are H. (A.) aponommoides Warburton (1913), H. (Allophysalis) garhwalensis Dhanda and Bhat (1968), H. (A.) kitaokai Hoogstraal (Reference Hoogstraal1969), H. (A.) vietnamensis Hoogstraal and Wilson (Reference Hoogstraal and Wilson1966) – currently known as H. colasbelcouri Santos Dias (1958) (Guglielmone and Nava, Reference Guglielmone and Nava2014) – H. verticalis Itagaki, Noda and Yamaguchi (1944) and H. (A.) primitiva Teng (1982). Further details about these taxa can be found in, e.g. Feider (Reference Feider1965), Hoogstraal and Kim (Reference Hoogstraal, Kim and Kim1985), Filippova (Reference Filippova1997), Geevarghese and Mishra (Reference Geevarghese and Mishra2011) and Guglielmone et al. (Reference Guglielmone2014). Our new fossil tick also lacks an expanded and laterally projecting second palpal article (Fig. 3C and D). Its palps are elongate and clavate and the external proximal side of the second article is concave, while the distal end is noticeably wider. This is similar to the condition seen in the structurally primitive taxa mentioned above and implies that the fossil could be a structurally primitive Haemaphysalis sensu Hoogstraal and Kim (Reference Hoogstraal, Kim and Kim1985), and may be further related to this particular Alloceraea group of living species.
Haemaphysalis biology and systematics
Haemaphysalis is the second largest tick genus, currently including 167 species. It occurs in all six zoogeographic regions, with the greatest diversity in south-eastern Asia and poorly represented in the Nearctic and the Neotropics (Kolonin, Reference Kolonin2009; Guglielmone et al. Reference Guglielmone2014). The best represented area for Haemaphysalis is the Oriental region (with 64 species) – which is of course geographically consistent with Burmese amber – followed by the Afrotropical region with 38 species (Guglielmone et al. Reference Guglielmone2014). One hundred and forty-six species are distributed exclusively (118 species) and non-exclusively on continents derived from Gondwana, and 20 species are established in areas derived from Laurasia (Guglielmone et al. Reference Guglielmone2014).
Haemaphysalis is currently divided into 11 subgenera (Hoogstraal and Kim, Reference Hoogstraal, Kim and Kim1985). The structurally primitive Haemaphysalis (see above) include four subgenera: Alloceraea, Allophysalis, Aboimisalis and Sharifiella (Hoogstraal and Kim, Reference Hoogstraal, Kim and Kim1985; Geevarghese and Mishra, Reference Geevarghese and Mishra2011).
In a review of Paul Schulze's contributions to tick systematics, Guglielmone et al. (Reference Guglielmone2017) compiled all the information about Alloceraea, which Schulze originally proposed as a genus for the Palearctic species H. inermis Birula (1895) – the type species for the subgenus Alloceraea – which possesses some morphological characteristics suggestive of Aponomma. Thereafter, Alloceraea was generally considered a subgenus of Haemaphysalis, but he later (Schulze, Reference Schulze1942) returned it to full genus rank and it was also treated as a valid genus by Zumpt (Reference Zumpt1951). Currently, Alloceraea is considered again a subgenus of Haemaphysalis (e.g. Camicas and Morel, Reference Camicas and Morel1977; Hoogstraal and Kim, Reference Hoogstraal, Kim and Kim1985; Filippova, Reference Filippova1997; Camicas et al. Reference Camicas1998). The subgenus Alloceraea contains the species H. (A.) aponommoides from India; H. (A.) inermis from Europe and Asia; H. (A.) kitaokai, H. (A.) verticalis and H. (A.) primitiva from Asia; and H. (A.) colasbelcouri from the Orient (Feider, Reference Feider1965; Filippova, Reference Filippova1997; Geevarghese and Mishra, Reference Geevarghese and Mishra2011; Guglielmone et al. Reference Guglielmone2014). As noted above, our new fossil tick most resembles these Alloceraea species in being small ticks which, like our fossil, lack eyes (Nicholson et al. Reference Nicholson, Mullen and Durden2009).
The 11 well-defined festoons in the new amber specimen (Fig. 3B) are all more or less the same size. This is the third tick with 11 festoons found in Burmese amber; the others being C. burmanicum (Poinar and Buckley, Reference Poinar and Buckley2008) and A. birmitum (Chitimia-Dobler et al. Reference Chitimia-Dobler2017). Most extant Haemaphysalis species also have 11 festoons (e.g. Feider, Reference Feider1965; Filippova, Reference Filippova1997; Geevarghese and Mishra, Reference Geevarghese and Mishra2011), but there are also species with nine, e.g. Haemaphysalis (Herpetobia) sulcata Canestrini and Fanzago (1877), Haemaphysalis (Segalia) parva Neumann (1897) – previously also known as Haemaphysalis otophila Schulze (1919) – and Haemaphysalis (Ornithophysalis) pavlovskyi Pospelova-Shtrom (1935) (Feider, Reference Feider1965; Filippova, Reference Filippova1997).
In the mouthparts, the dental formula of the hypostome in the new specimen is not entirely clear, but numerous denticles can be observed (almost 8–10 in a single file) and a rosette is visible distally (Fig. 3D). The fossil larva described as C. vetulum has a 2/2 dental formula (Poinar and Buckley, Reference Poinar and Buckley2008), as does A. birmitum (Chitimia-Dobler et al. Reference Chitimia-Dobler2017) which is an adult female. Structurally advanced Haemaphysalis species usually have a 2/2 dental formula when immature, although in some species it can be 3/3 or 4/4. As adults they usually have a 4/4 arrangement, but exceptionally they can have a 3/3 or 5/5 to 7/7 dental formulae. In this respect, our new fossil is again closest to structurally primitive Haemaphysalis, and in particular to nymphs which have a 2/2 dental formula with only 8–12 denticles in each file. Compared with modern Alloceraea species, H. aponommoides has a 3/3 dental formula with eight denticles in a file as a nymph, H. (A.) kitaokai has a 2/2 dental formula with 4–5 denticles in a file as a nymph and H. (A.) inermis has a 2/2 dental formula with 6–8 denticles in a file as a nymph (Feider, Reference Feider1965; Hoogstraal, Reference Hoogstraal1969; Geevarghese and Mishra, Reference Geevarghese and Mishra2011).
Structurally primitive Haemaphysalis show some distinctive morphological characters: each developmental stage – or only the larvae and nymphs – presents a lateral convexity of the basis capituli or a projection from each side of the basis capituli; the palps are elongate and compact, but not basolaterally salient, and capitular and leg-spur development is (with a few notable exceptions) exceedingly slight (Hoogstraal and Kim, Reference Hoogstraal, Kim and Kim1985; Geevarghese and Mishra, Reference Geevarghese and Mishra2011). Structurally primitive Haemaphysalis, and the other non-rhipicephaline ixodids, differ distinctly from other haemaphysaline species in other subgenera. For example, life stages from the subgenus Alloceraea have a laterally convex or otherwise laterally projecting basis capituli, lacking cornua, and elongate (clavate) pedipalpi lacking a ventral spur (Geevarghese and Mishra, Reference Geevarghese and Mishra2011). Our new fossil has some of these Alloceraea characteristics, such as elongate pedipalpi, absence of a ventral spur on the pedipalps and lacking cornua, but can be differentiated from the living species in that it does not show the convex or laterally projecting basis capituli (Fig. 3C and D). The new fossil is perhaps morphologically closest to H. (A.) inermis, which also lacks a lateral projection (Feider, Reference Feider1965) and both this species and the fossil present six setae on the palps; the setae being longer in our fossil. The various spur or spur-like angles of the body appendages are obsolete or only very slightly developed in each Alloceraea stage. Extant Alloceraea species uniquely have a leathery cuticle, which is probably adapted for water conservation (Geevarghese and Mishra, Reference Geevarghese and Mishra2011). The identification of species within the subgenus Alloceraea is rather difficult. Different authors sometimes consider conspecific ticks collected in the same region as different species. Therefore, the data on the precise distribution of H. (A.) aponommoides, H. (A.) inermis, H. (A.) kitaokai and H. (A.) colasbelcouri in the territory of China is rather inconsistent (Kolonin, Reference Kolonin2009).
In conclusion, we concede that our new fossil is not a typical Haemaphysalis, but we propose that it most closely resembles members of the extant Haemaphysalis subgenus Alloceraea; albeit differing from living taxa in some features. The basis capitulum of our fossil is dorsally 2.6 times as broad as long (length from palpal insertion to posterior margin of basis 83.7 µ m, width 239.6 µ m) (Fig. 3C). It is four times as broad as long in H. (A.) aponommoides, 2.9 times as broad as long in H. (A.) kitaokai, five times as broad as long in H. (A.) garhwalensis and broader than long in H. (A.) inermis (Feider, Reference Feider1965; Hoogstraal, Reference Hoogstraal1969; Geevarghese and Mishra, Reference Geevarghese and Mishra2011). The scutum of our new fossil is 1.2 times as broad as long (Fig. 3A), but with margins broadly rounded, no evidence of punctuations, the scapulae are acute-angled and resemble elongate sharp prongs; the cervical grooves are deep and linear anteriorly (Fig. 2A) and diverge posteriorly where they can be traced along the whole length of the scutum. In H. (A.) aponommoides, the scutum is approximately 1.4 times as broad as long, few punctuations are seen and the cervical grooves form deep arcs. In H. (A.) kitaokai, the scutum is 1.25 times as broad as long, cordiform, punctations are few, shallow, small and of moderate size; cervical grooves are slightly converging anteriorly, slightly diverging posteriorly and extend to the posterior margin of scutum. In H. (A.) garhwalensis, few punctuations are present, numbering eight to ten and the cervical grooves are linear, extending to almost to the posterior margin of the scutum (Hoogstraal, Reference Hoogstraal1969; Geevarghese and Mishra, Reference Geevarghese and Mishra2011). The scutum of H. (A.) inermis is 1.4 times as broad as long, cordiform with the posterior part converging, deep and uniform spread punctuations are seen, and the cervical grooves are deep, almost reaching the margin of the scutum (Feider, Reference Feider1965). These morphological differences justify treating the Cretaceous fossil tick is an extinct Haemaphysalis species.
Haemaphysalis host taxa
Haemaphysalis ticks typically parasitize mammals, and to a lesser extent birds, in most regions of the world (Nicholson et al. Reference Nicholson, Mullen and Durden2009). Frogs, etc. (Anura), and crocodiles have not been recorded as hosts for any life stage of Haemaphsysalis species, while lizards and snakes (Squamata) and tortoises (Testudines) make only a relatively minor contribution (Guglielmone et al. Reference Guglielmone2014). Of the closest living matches for our fossil, adult H. (A.) inermis ticks feed on a variety of mammals, while the immature stages feed on small mammals and reptiles (Feider, Reference Feider1965; Manilla, Reference Manilla1998; Perez-Eid, Reference Perez-Eid2007). Haemaphysalis (A.) aponommoides adults feed on domestic and wild mammals (including the flying squirrel), while the immature stages feed on lizards, shrews, rodents and birds (Geevarghese and Mishra, Reference Geevarghese and Mishra2011). Haemaphysalis (A.) kitaokai adults have been collected from cattle, horse, Japanese serow and sika deer in the Honshu and Kyushu islands (Hoogstraal, Reference Hoogstraal1969). Haemaphysalis (A.) colasbelcouri adults have been collected from cattle and deer, for H. (A.) verticalis two females were collected from the domestic dog in Shansi, but mostly occur on ground squirrels and sometimes from another rodent, the long-clawed jird (Emel'yanova and Hoogstral, Reference Emel'yanovat and Hoogstraal1973). The normal hosts are squirrels (Sciuridae) for all life stages, and exceptionally birds (Guglielmone et al. Reference Guglielmone2014).
Our new fossil is a nymph, and so could potentially have used lizards, birds, (small) dinosaurs or mammals as hosts. However, we might predict that the adult of H. (A.) cretacea sp. nov. was primarily a parasite of Cretaceous mammals; similar to the modern members of this subgenus. This makes it potentially one of the oldest pieces of evidence for an arthropod parasitizing a mammal. We should add that other data suggest that parasitic insect groups such as lice (Smith et al. Reference Smith2011) and fleas (Huang et al. Reference Huang2012) may have been present on Late Cretaceous mammals too.
Structurally primitive Haemaphysalis species represent approximately 10% of the total number of known species in the genus. Ecologically and geographically, all except one species of this group are confined to temperate zones of North America – H. (Aboimisalis) chordeilis Packard (1869) – and Eurasia. Haemaphysalis (Sharifiella) theilerae Hoogstraal (1953) is the only exception, being present in the tropical climate of Madagascar. Low temperatures and slow reproductive rates appear to be partially responsible for the preservation of the primitive morphotype and perhaps also for the survival of certain species that have changed little, if at all, since the genus first originated; see Hoogstraal and Wilson (Reference Hoogstraal and Wilson1966) for further discussion. In terms of biogeography, the species in the Alloceraea subgenus have different distributions. Haemaphysalis (A.) aponommoides is closer to Myanmar, having been partially found in Southeast Asia: namely Iran, India, Nepal, Taiwan and Japan (Geevarghese and Mishra, Reference Geevarghese and Mishra2011). Haemaphysalis (A.) inermis has been recorded from the former Soviet Union, Turkey, Iran, Romania, Albania, Greece, Bulgaria, Czech Republic, Germany and France (Feider, Reference Feider1965; Manilla, Reference Manilla1998). Haemaphysalis (A.) colasbelcouri (vietnamensis) has been recorded only in the Vietnam highlands (Hoogstraal and Wilson, Reference Hoogstraal and Wilson1966). Haemaphysalis (A.) kitaokai has been reported from Japan and Taiwan (Hoogstraal, Reference Hoogstraal1969). Haemaphysalis (A.) verticalis has been recorded from China (Emel'yanova and Hoogstral, Reference Emel'yanovat and Hoogstraal1973).
The fossil record of ticks compared to their hosts
The Mesozoic is popularly referred to as the ‘Age of Reptiles’. This is somewhat misleading, as there were also increasing numbers of both birds and mammals from the Jurassic onwards (see below) but, as noted above, living species of Amblyomma, the extant tick genus found previously in Burmese amber (Grimaldi et al. Reference Grimaldi, Engel and Nascimbene2002; Chitimia-Dobler et al. Reference Chitimia-Dobler2017), do largely use reptiles as their hosts today. By contrast, H. (A.) cretacea sp. nov. appears to be closest to ticks, which in modern ecosystems feed on (placental) mammals as adults, and mammals, lizards and birds when immature. Hoogstraal (Reference Hoogstraal1965) postulated that Haemaphysalis ticks arose alongside reptiles in the Late Paleozoic or Early Mesozoic in warm, humid forests of tropical Southeast Asia. In this hypothesis, severe competition from numerous more advanced forms led to most structurally primitive haemaphysalids becoming extinct. This scenario touches on the wider question of to what extent there has been coevolution between these parasites and their hosts. A caveat here is that lineages may have originated on groups that are now extinct or they may have changed hosts over deep geological time. Thus, Klompen et al. (Reference Klompen1996) cautioned against the assumption that host phylogeny should determine tick phylogeny. Bearing this in mind, it may still be instructive to compare the fossil record of ticks with records of their vertebrate hosts, as well as molecular clock estimates for when the parasite and/or host lineages may have originated.
Tick origins
For a recent review of tick origins, and their probable relationships to other mites, see Mans et al. (Reference Mans2016). Modern ticks are ectoparasites on terrestrial and semi-aquatic vertebrates (Guglielmone et al. Reference Guglielmone2014), thus an obvious question is who their initial hosts were? Dobson and Barker (Reference Dobson and Barker1999), Barker and Murrell (Reference Barker and Murrell2002) and Barker et al. (Reference Barker, Walker and Campelo2014) argued for Devonian labyrinthodont amphibians (i.e. tetrapods), specifically those living in Australia, as the original host species. The lungfish–tetrapod split has been estimated to the Early Devonian (408–419 Ma, Müller and Reisz, Reference Müller and Reisz2005), although both groups were presumably still (primarily) aquatic at this time. Although not Australian, fossils such as Tiktalik from the Late Devonian (ca. 375 Ma) of Canada (Daeschler et al. Reference Daeschler, Shubin and Jenkins2006) are essentially fish with several transitional characters towards the tetrapod condition. Slightly younger fossils from the Late Devonian (ca. 365 Ma) of Greenland, such as Acanthostega or Ichthyostega, can be considered true tetrapods and may offer an upper constraint on the appearance of potential hosts for ancestral ticks (Table 1). However, these early tetrapods are also thought to have been essentially aquatic (reviewed by Clack, Reference Clack2012), and are sometimes referred to as ‘fish with legs’. In other words, could a Devonian tick have lived on a primarily aquatic host? Some modern ticks have been documented surviving for weeks or even months underwater (e.g. Smith, Reference Smith1973), thus the labyrinthodont hypothesis should not be dismissed out of hand. Nevertheless, tetrapods may have only been habitually terrestrial from the Early Carboniferous (330–345 Ma, Smithson et al. Reference Smithson2012) onwards. This partly overlaps with Mans et al.’s (Reference Mans2012) molecular estimate of 350 ± 23 Ma for when the ticks diverged from mesostigmatid mites (Table 1); although it should be noted that these authors regarded Holothyrida – for which molecular data were not available – as the sister group of Ixodida. Mans et al. (Reference Mans2016) also favoured tick origins in the Carboniferous or Permian as opposed to an older Devonian date.
An alternative scenario would constrain ticks to hosts within the Amniota, i.e. vertebrates who did not need to return to water to breed, and presumably spent most (if not all) of their time on land. Some authors have indeed suggested the initial tick hosts were Palaeozoic reptiles (e.g. Hoogstraal and Aeschlimann, Reference Hoogstraal and Aeschlimann1982). The oldest unequivocal amniote fossils – which also provide a maximum age for reptiles in general – date to the Late Carboniferous (ca. 311 Ma, e.g. Modesto et al. Reference Modesto2015). Amniota is thought to have diverged a little earlier (e.g. Reisz and Fröbisch, Reference Reisz and Fröbisch2014) to form two main lineages, both of which regularly host ticks today. Of these, Sauropsida (which includes all modern reptiles) would also later lead to birds, while Synapsida would eventually produce the mammals. However, molecular estimates for what is essentially the bird–mammal split are not well constrained (see comments in Müller and Reisz, Reference Müller and Reisz2005), partly because the Late Carboniferous amniote fossil record is too sparse to bracket this divergence with any confidence. Published estimates include, e.g. 323–326 Ma for the diapsid–synapsid split (Pereira and Baker, Reference Pereira and Baker2006) and younger dates of ca. 285 Ma (Hugall et al. Reference Hugall, Foster and Lee2007) for the bird–lizard split, reflecting the origins of crown-group reptiles. In summary, amniotes probably evolved and diversified at some point in the Late Carboniferous (298–323 Ma), and this approximate date is consistent with Mans et al.’s (Reference Mans2012) 319 ± 25 Ma molecular estimate for when the ticks began to diverge from one another.
Nuttalliellidae
Nuttalliella namaqua Bedford (Reference Bedford1931) is the only representative of the remarkable family Nuttalliellidae and shares several characteristics with both hard ticks (Ixodidae) and soft ticks (Argasidae). It has thus been envisaged as a ‘missing link’ between the other two tick families (e.g. Bedford, Reference Bedford1931; El Shoura, Reference El Shoura1990; Latif et al. Reference Latif2012; Mans et al. Reference Mans2016). Recent studies have recovered N. namaqua as the sister group of all other ticks (Mans et al. Reference Mans2012), or sister group to Ixodidae only (Burger et al. Reference Burger2014) to the exclusion of the soft ticks. Early studies suggested that the preferred hosts of N. namaqua could be rock hyraxes (Procavia capensis), swallows, rodents and meerkats (Bedford, Reference Bedford1931; Keirans et al. Reference Keirans1976), while Agama or other lizards were also considered (Hoogstraal, Reference Hoogstraal1985). Efforts to feed females and nymphs on chickens, pigeons, rabbits, rats or mice were unsuccessful (Hoogstraal, Reference Hoogstraal1985). Later, Mans et al. (Reference Mans2011) and Horak et al. (Reference Horak2012) collected N. namaqua larvae from different murid rodent species; while a gut meal analysis from field-collected female ticks showed that it had fed on lizards. In conclusion, it seems likely that N. namaqua is a multi-host tick.
Nuttalliella namaqua has been called a ‘living fossil’ (Mans et al. Reference Mans2012), although it lacks a fossil record itself. Several authors consider the nuttalliellid lineage to have split off first from the argasid/ixodid ticks, and as noted above, Mans et al. (Reference Mans2012) dated this to the Late Carboniferous (319 ± 25 Ma). The alternative (Nuttalliella + Ixodidae) hypothesis is not yet associated with a date estimate. Of the known nuttalliellid hosts, lizards are the oldest potential group. The origins of Lepidosauria (i.e. lizards, snakes and the tuatara) are probably to be found in the Triassic. The oldest unequivocal lizards come from the mid-Jurassic (e.g. Evans, Reference Evans1998). Lepidosaur origins were reviewed in some detail by Jones et al. (Reference Jones2013) who noted that published molecular estimates ranged from the Early Jurassic (ca. 179 Ma) to the Early Permian (ca. 294 Ma). They themselves favoured a mid-Triassic estimate of about 238–250 Ma. In this scenario, there is a discrepancy of some 80 million years between the time when the lineage, which includes N. namaqua, may have originated and the expected appearance of at least some of the hosts they are known to use today. The implication is that nuttalliellids initially had to use other (extinct) reptile groups – perhaps for tens of millions of years – before adopting at least some of the hosts they use today.
Deinocrotonidae
Although nuttalliellids lack a fossil record, a recent and remarkable discovery (Peñalver et al. Reference Peñalver2017) was the extinct family Deinocrotonidae described from Burmese amber with a single species D. draculi. The authors documented several characteristic features of deinocrotonids – including the structure of the integument, the palp, the shape of the preanal groove and a uniquely discontinuous genital groove – but suggested that Deinocrotonidae and Nuttalliellidae may be sister taxa, sharing synapomorphes such as a subterminal hypostome and the presence of a pseudoscutum. If this hypothesis is correct, the (Deinocrotonidae + Nuttalliellidae) clade must be at least 99 million years old, although a possible deinocrotonid was mentioned by Peñalver et al. (Reference Peñalver2017) from the slightly older (ca. 105 Ma) Spanish amber, which awaits formal description. As noted above, molecular data suggest a much older (Carboniferous) date for when nuttalliellids split from the clade encompassing both the hard and soft ticks.
With respect to their ecology, the Deinocroton fossils were found associated with specialized hairs (hastisetae) typical for larvae of the beetle family Dermestidae, which today are often found in bird nests. This discovery led Peñalver et al. (Reference Peñalver2017) to infer that Deinocroton may also have been also associated with nest ecosystems and may thus have parasitized feathered dinosaurs. The age of the oldest feathered dinosaur is not so easy to resolve as there was almost certainly a gradation from downy filaments though to the fully developed flight feathers of birds (cf. Prum, Reference Prum1999); see also discussion of the oldest birds below. Several Jurassic fossils show evidence of feather-like structures and a similar date, approaching 200 Ma, was adopted by Peñalver et al. (Reference Peñalver2017: Fig. 10).
Argasidae
Argasids are sometimes referred to as ‘bird ticks’, but this is misleading because, as a group, they are multi-host parasites with several genera utilizing mammals. Five argasid genera are currently recognized traditionally divided into the subfamilies Argasinae and Ornithodorinae. The argasines are restricted to the genus Argas, which includes parasites of both birds and bats (Nicholson et al. Reference Nicholson, Mullen and Durden2009). Of the four ornithodorine genera, Ornithodoros feeds on a range of both marsupial and placental mammals, and occasionally on birds and reptiles, Otobius has two species which feed, respectively, on rabbits and several domestic animals, while Antricola and Nothoaspis specifically parasitize bats. Note that most of the Carios species (currently an invalid genus) are now considered to belong to Ornithodoros or Argas (11 species) or to Antricola and Nothoaspis (see Estrada-Peña et al. Reference Estrada-Peña2010; Guglielmone et al. Reference Guglielmone2010).
Mans et al. (Reference Mans2012) estimated an Early Permian (290 ± 23 Ma) split between Argasidae and Ixodidae, and a Late Triassic (234 ± 25 Ma) split between Argasinae and Ornithodorinae. Argas currently lacks a fossil record (Table 1). Of the groups it parasitizes today (birds and bats), the oldest fossil traditionally recognized as a bird is still Archaeopteryx from the Late Jurassic (ca. 150 Ma) of Germany, although there is debate in the literature about precisely where to draw the line between birds and the non-avian (feathered) dinosaurs from which they evolved. A corollary of this is that argasids may have been hosted previously by other groups (e.g. dinosaurs), which would be consistent with Mans et al.’s Triassic origination date. At some stage, Argas shifted to bird hosts, and while several Jurassic fossils are evidently close to bird origins, the fragility of bird skeletons means that their subsequent Cretaceous record is poor (Brocklehurst et al. Reference Brocklehurst2012). This ambiguity in the fossil record makes it hard to calibrate molecular estimates for when birds first appeared. For example, Pereira and Baker (Reference Pereira and Baker2006) could only confidently bracket the split at the base of the archosaurs (i.e. crocodiles, dinosaurs and birds) to ca. 258 Ma and the split between the palaeognath and neognath bird lineages (both crown-group clades with living species) to at least 139 Ma; which is obviously younger than the current fossil record for the Aves total group. A similar point about the lack of robust calibrations for bird origins was made by Prum et al. (Reference Prum2015) who also stressed that, on their data, most modern birds radiated after the end Cretaceous mass extinction. For bats, the oldest unequivocal fossils are Eocene in age (ca. 52 Ma); see, e.g. Simmons et al. (Reference Simmons2008). Molecular data (Teeling et al. Reference Teeling2005) support the hypothesis that bats originated shortly after the K–T extinction event (ca. 64 Ma).
Among the ornithodorines, Ornithodoros (as Carios) is known from Late Cretaceous (ca. 94 Ma) New Jersey amber (Klompen and Grimaldi, Reference Klompen and Grimaldi2001) and the ca. 16 Ma Miocene Dominican Republic amber (Poinar, Reference Poinar1995). Otobius, Antricola and Nothoaspis lack a fossil record. As noted above, ornithodorine ticks usually parasitize mammals. Identifying the oldest unequivocal fossil mammal is also difficult, as there is an obvious gradation from synapsid reptiles through to modern mammal groups. Mammals evolved from a broader group, usually referred to as the Mammaliaformes, whose origins are to be found in the mid- to Late Triassic. Thus depending on the author, and the definition of Mammalia they use, the oldest mammals may be cited at Triassic or Jurassic in age; see Williamson et al. (Reference Williamson, Brusatte and Wilson2014) for a review. An estimate of ca. 160–208 Ma would encompass the oldest putative mammal fossils through to the oldest unequivocal members of the group (e.g. Luo et al. Reference Luo2011). As with birds, debate about the exact position of certain fossils hinders effective calibration of molecular trees, but Phillips et al. (Reference Phillips, Bennett and Lee2009), e.g. estimated the split between monotremes and all other mammals to 161–217 Ma; with a caveat that other dates can be found in the literature. Otobius is only found on placental mammals. The origins of crown placental mammals is also controversial (e.g. dos Reis et al. Reference dos Reis, Donoghue and Yang2014 and references therein), with dates both before and after the K–T extinction being proposed. The oldest unequivocal placental fossil dates to the Paleogene (ca. 65 Ma), while dos Reis et al. (Reference dos Reis, Donoghue and Yang2014) proposed Cretaceous origins for placental mammals based on the molecular data between 72 and 108 Ma; depending on the calibration method used.
Translating this into an evolutionary scenario for Argasidae, early members of this family could have been hosted by one of several groups of Late Palaeozoic or Early Mesozoic reptiles, and there is a gap of at least 50 million years between the inferred origins of argasids and the appearance of at least extinct relatives of their modern host groups. The inferred Late Triassic split into the subfamilies Argasinae (with bird/bat hosts) and Ornithodorine (mostly with mammal hosts) is particularly interesting. It corresponds well with the time at which dinosaurs, which eventually gave rise to birds, were thought to have been evolving from the other archosaurs, and is also the time when the mammaliaform/mammal lineage emerged from the synapsids. A deep (Triassic) split in host preferences between the argasid subfamilies is thus plausible; see also comments in Mans et al. (Reference Mans2012). Bats are geologically much younger (Paleogene/Eocene) and the shift to bat hosts by members of both the argasines and the ornithodorines must have occurred more than once. In general, we should not forget that hosts almost certainly changed over time and that modern associations of a given tick species with particular living bird or (placental) mammal group must also be geologically quite young. Both the fossil record and molecular data strongly imply that modern mammal and bird lineages radiated after the Cretaceous (dos Reis et al. Reference dos Reis, Donoghue and Yang2014; Prum et al. Reference Prum2015).
Ixodidae: Prostriata
Hard ticks (Ixodidae) can be divided into two main lineages: Prostriata restricted to Ixodes, and Metastriata containing the remaining genera. There seems to be a consensus that Ixodes can be divided into an Australian and a non-Australian lineage, and some authors (Klompen et al. Reference Klompen2000) have even questioned whether the genus is monophyletic. Hosts for Australian Ixodes species were reviewed by Barker et al. (Reference Barker, Walker and Campelo2014) and include monotreme, marsupial and placental mammals, as well as sea birds. A similar pattern is seen in the remaining Ixodes ticks, which are usually found on mammals, but also includes species which parasitize birds and occasionally reptiles (Hoogstraal and Aeschlimann, Reference Hoogstraal and Aeschlimann1982).
The oldest fossil Ixodes is I. (Partipalpiger) succineus from Eocene (ca. 44–49 Ma) Baltic amber (Weidner, Reference Weidner1964; Dunlop et al. Reference Dunlop2016), plus a subfossil record from an owl pellet. As noted above, the Ixodidae–Argasidae split has been estimated to the Early Permian (290 ± 23 Ma). Within ixodids, Mans et al. (Reference Mans2012) placed the Prostriata–Metastriata split in the earliest part of the Triassic (249 ± 23 Ma) and the split into Australian and non-Australian Ixodes lineages in the Late Triassic (217 ± 24 Ma) (Table 1). The amber fossil is thus much too young to offer any insights into the origins of the genus, but the preference for mammalian hosts among several living Ixodes species could imply a long association, potentially beginning with the mammal-like reptiles, which belong to the broader synapsid clade. To reiterate (see above), Synapsida originated in the Late Carboniferous (Reisz and Fröbisch, Reference Reisz and Fröbisch2014) when they split off from the Sauropsida, which includes extinct groups (e.g. Parareptilia) as well as the ancestors of modern reptiles and birds. The Late Carboniferous is also the time when argasids are estimated to have split from ixodids. During the Permian early members of both tick families would have had mammal-like synapsid reptiles and sauropsids as potentially available hosts, although any host preferences at this stage remain speculation.
What could be significant is the estimated split of Ixodidae into Prostriata and Metastriata at about the time of the End-Permian mass extinction. This major event fundamentally changed the tetrapod landscape. Within Synapsida, groups such as the, probably paraphyletic, pelycosaurs were replaced by the more mammal-like cynodonts; see, e.g. Rubidge and Sidor (Reference Rubidge and Sidor2001) or Abadala and Ribeiro (Reference Abadala and Ribeiro2010) for reviews. One hypothesis would be that prostriate ticks adopted cynodonts as hosts during the Triassic, and essentially remained with them through their subsequent development into mammals in the Late Triassic or Early Jurassic. However, we should note that in several studies (e.g. Dobson and Barker, Reference Dobson and Barker1999; Fukunaga et al. Reference Fukunaga2000) species such as the Australian paralysis tick Ixodes holocyclus Neumann 1899 resolved in a basal position within Ixodes. This is a multi-host parasite found both on mammals and birds, and it is unclear to what extent this reflects the original host preferences, or a general adaptability in host choice within the genus. For example, other Ixodes ticks use birds when immature and switch to mammals as adults (Barker et al. Reference Barker, Walker and Campelo2014) and several Ixodes ticks prefer birds in general.
Ixodidae: Metastriata
Metastriata includes 11 extant and two extinct genera. Following authors such as Hoogstraal and Aeschlimann (Reference Hoogstraal and Aeschlimann1982) and Barker and Murrell (Reference Barker and Murrell2002, Reference Barker and Murrell2004), the sequence of genera, from basal to derived, is probably along the lines of: Bothriocroton (the subfamily Bothriocrotoninae), Amblyomma (Amblyomminae), Haemaphysalis (Haemaphysalinae), followed by Cosmiomma Schulze (1919); Dermacentor CL Koch (1844); Rhipicentor Nuttall and Warburton (1908); Nosomma Schulze (1919); Hyalomma CL Koch (1844); Anomalohimalaya, Rhipicephalus and Margaropus Karsch (1879) (all Rhipicephalinae). For an alternative phylogeny, see Burger et al. (Reference Burger, Shao and Barker2013) who recognized two major metastriate lineages: (1) Amblyomma s.s. + rhipicephalines and (2) Haemaphysalis + Bothriocroton + A. sphenodonti. Host preferences for individual genera are given in Table 1. The important message here is that Bothriocroton and Amblyomma – representing the more basal subfamilies sensu Barker and Murrell (Reference Barker and Murrell2002) – use a mixture of reptilian and mammalian hosts. For example, species previously assigned to the genus Aponomma invariably parasitize reptiles, but following the revision of Klompen et al. (Reference Klompen, Dobson and Barker2002), they were transferred to Bothriocroton and Amblyomma, respectively. As noted above, Haemaphysalis is usually found on mammals, but also sometimes on birds. The rhipicephalines are strongly, or exclusively (Cosmiomma, Dermacentor, Rhipicentor, Nosomma and Anomalohimalaya), associated with mammals throughout their life cycle.
The oldest fossil metastriate ticks include the two (99 Ma) Burmese amber records of Amblyomma (Chitimia-Dobler et al. Reference Chitimia-Dobler2017) and Haemaphysalis (this study). The extinct genera Cornupalpatum and Compluriscutula from Burmese amber are probably metastriates too and close to Amblyomma. There is evidence that Cornupalpatum may have fed on feathered dinosaurs and/or birds, with Peñalver et al. (Reference Peñalver2017) figuring a tick belonging to this extinct genus entangled in a pennaceous feather. In detail, the feather was considered as belonging to stage V of Prum's (Reference Prum1999) model of feather evolution and implies a host belonging to the Pennaraptora sensu Foth et al. (Reference Foth, Tischlinger and Rauhut2014); a clade encompassing several derived genera of feathered dinosaurs as well as crown-group birds. The only other metastriate fossils are Amblyomma records from Miocene (ca. 16 Ma) Dominican Republic amber and a subfossil record of Dermacentor. As noted by Chitimia-Dobler et al. (Reference Chitimia-Dobler2017), a published record of Hyalomma in Eocene Baltic amber (de la Fuente, Reference de la Fuente2003) is a misidentification. Metastriata is estimated to have split off from Prostriata in the Early Triassic (ca. 249 ± 23 Ma) with a further putative divergence (Mans et al. Reference Mans2012) into the modern genera in the Early Cretaceous at ca. 124 ± 17 Ma. In other words, the Burmese amber fossils come from a time not long after the suggested radiation into living genera. The fossils also now encompass two of the four subfamily groups recognized by Barker and Murrell (Reference Barker and Murrell2002, Reference Barker and Murrell2004): namely Amblyomminae and Haemaphysalinae.
In the Mans et al. (Reference Mans2012) scenario, there is a ca. 125 Ma hiatus from the Triassic through to the Early Cretaceous during which metastriate ticks should have been present, but had not yet diversified into their modern genera. Several reptilian and mammal-like reptile groups, as well as eventually mammals and birds, would have been available as potential hosts during this time. What may be significant is that the basal metastriate ticks (Bothriocroton and Amblyomma) retain a stronger association to reptiles. While prostriates (i.e. Ixodes) may have adopted mammal-like reptiles as hosts back in the Triassic (see above), the first members of the metastriate lineage may have taken up other reptiles such as squamates or archosaurs as their original hosts instead.
A subsequent shift in host preferences may have taken place in the more derived haemaphysaline and rhipicephaline metastriate genera, for which H. (A.) cretacea sp. nov. is the oldest known putative example. In the two most derived tick subfamilies, mammals appear to play a more significant role. Unfortunately, most of the rhipicephaline genera lack a fossil record (Table 1). Molecular data from Sands et al. (Reference Sands2017) suggested that the modern Hyalomma species diverged towards the end of the Eocene at around 34.8–39.8 Ma – although we should caution that they were still using the erroneous Baltic amber record of this genus for calibration – with Fig. 2 of the same paper suggesting a Palaeocene Nosomma/Hyalomma split at ca. 60 ± 17 Ma and a deeper (Cretaceous) split from Amblyomma at 78 ± 20 Ma. We might predict that rhipicephaline evolution in general is linked to the Late Cretaceous origins of modern mammal lineages (e.g. dos Reis et al. Reference dos Reis, Donoghue and Yang2014) and their subsequent diversification after the K–T extinction event.
Indeed some (monotypic) genera here have quite specific host preferences: Cosmiomma is found as adults on hippos and rhinos (Aspanaskevich et al. Reference Aspanaskevich2013) and Nosomma occurs on buffalo as adults and rodents when immature (Kahn et al. Reference Kahn, Naithani and Singh1982). These taxa may be constrained by the appearance of their hosts. Fossil rhinos are known since the Eocene (38–42 Ma) (Cerdeño, Reference Cerdeño1998), while hippos and bovids are known from the Miocene (16 and 18 Ma, respectively), but are predicted to have originated earlier depending on their sister group (Theodor, Reference Theodor2004). For example, molecular origination dates of ca. 50 Ma for perissodactyls (including rhinos) and 23 Ma for bovids have been proposed (Norman and Ashley, Reference Norman and Ahsley2000; Matthee and Davis, Reference Matthee and Davis2001). A final thought is that Burmese, and other Cretaceous, amber deposits may yield further ticks belonging to other (rhipicephaline?) genera, which may help to confirm and refine the hypotheses presented above.
Acknowledgements
We thank Patrick Müller for making this material available for study and Professor Horst Aspöck for discussion regarding the name of our fossil tick. We thank also Dr Santiago Nava and Professor Richard Robbins for the comments, suggestions and provision of the literature. Two reviewers offered valuable comments on an earlier draft which greatly improved the manuscript.
Financial support
This research received no specific grant from any funding agency, commercial or not-for-profit sectors.