INTRODUCTION
Fasciola hepatica is the causative agent of fasciolosis, a zoonosis that affects livestock production in many countries, thus being responsible for massive economic losses, estimated in 3·2 billion US$ worldwide (Spithill et al. Reference Spithill, Smooker, Copeman and Dalton1999). The infection additionally affects humans. In fact, in the past 10 years, an increase in human cases has been observed in several countries in the five continents (Mas-Coma et al. Reference Mas-Coma, Valero and Bargues2014) including South America (Carmona and Tort, Reference Carmona and Tort2017). For this reason, fasciolosis is considered an emerging human disease by the World Health Organization (WHO, 2007). There are effective drugs for treating fasciolosis, but their availability is limited and reports of resistance are growing (Overend and Bowen, Reference Overend and Bowen1995; Canevari et al. Reference Canevari, Ceballos, Sanabria, Romero, Olaechea, Ortiz, Cabrera, Gayo, Fairweather, Lanusse and Alvarez2013; Kelley et al. Reference Kelley, Elliott, Beddoe, Anderson, Skuce and Spithill2016). Elucidation of molecular mechanisms that allow establishment of the parasite in its host is necessary for the identification of target molecules for the rational development of novel drugs and vaccines.
During infection of mammalian hosts (sheep, cattle, humans), the juvenile forms of F. hepatica penetrate the gut wall and migrate through the liver capsule and parenchyma, finally reaching the bile ducts, where the adult worms mature (Andrews, Reference Andrews and Dalton1999). To assist this process, F. hepatica intramammalian stages express and secrete a wide repertory of cysteine proteinases, mainly cathepsin L-like and cathepsin B-like enzymes (Law et al. Reference Law, Smooker, Irving, Piedrafita, Ponting, Kennedy, Whisstock, Pike and Spithill2003; Cancela et al. Reference Cancela, Acosta, Rinaldi, Silva, Duran, Roche, Zaha, Carmona and Tort2008, Reference Cancela, Ruetalo, Dell'Oca, da Silva, Smircich, Rinaldi, Roche, Carmona, Alvarez-Valin, Zaha and Tort2010; Robinson et al. Reference Robinson, Tort, Lowther, Donnelly, Wong, Xu, Stack, Padula, Herbert and Dalton2008b ; Beckham et al. Reference Beckham, Piedrafita, Phillips, Samarawickrema, Law, Smooker, Quinsey, Irving, Greenwood, Verhelst, Bogyo, Turk, Coetzer, Wijeyewickrema, Spithill and Pike2009; Di Maggio et al. Reference Di Maggio, Tirloni, Pinto, Diedrich, Yates, Benavides, Carmona, da Silva Vaz and Berasain2016). These secreted proteinases play key roles in host invasion, parasite feeding and immune evasion (Robinson et al. Reference Robinson, Dalton and Donnelly2008a ; Corvo et al. Reference Corvo, Cancela, Cappetta, Pi-Denis, Tort and Roche2009) and their activities can be controlled, for example, by synthetizing specific inhibitors also produced by the parasite.
Cystatins (MEROPS Inhibitor Family I25) are natural, small reversible, tight-binding inhibitors of cysteine proteinases. They can regulate the function and activity of cysteine proteinases by blocking its active site through non-covalently binding of a highly conserved QxVxG motif characteristic of the cystatin molecule (Turk et al. Reference Turk, Stoka and Turk2008; Rawlings et al. Reference Rawlings, Barrett and Bateman2012). Based on their amino acid sequences and three dimensional structures, cystatins can be assigned to three major subfamilies (I25A, I25B and I25C). The I25A subfamily (stefins) comprises non-glycosylated inhibitors of about 11 kDa that are devoid of signal peptide and disulfide bonds; mammalian stefins A and B (also called cystatins A and B) are two prominent representatives of this subfamily of intracellular inhibitors. The I25B subfamily (cystatins stricto senso) are extracellular proteins of 13–14 kDa that contain a signal peptide and two nearly C-terminal disulfide bonds. Members of the I25B family, which includes human cystatins C, D, S, SA, SN and egg white cystatin, are found in most animal biological fluids. The subfamily I25C comprises larger, glycosylated, multifunctional proteins with three cystatin-like domains and disulfide bonds. Family members lacking cystatin activity are currently unclassified (Rawlings et al. Reference Rawlings, Barrett and Bateman2014). Cystatins are involved in various physiological and pathological processes, such as protein catabolism, antigen processing, inflammation and tumour metastasis (Barrett, Reference Barrett1986; Henskens et al. Reference Henskens, Veerman and Nieuw Amerongen1996; Kopitar-Jerala, Reference Kopitar-Jerala2015; Stoka et al. Reference Stoka, Turk and Turk2016).
Parasitic helminths make use of cystatins to control their endogenous cysteine proteinases and also to manipulate host responses in their favour (Klotz et al. Reference Klotz, Ziegler, Danilowicz-Luebert and Hartmann2011a ). Immunoregulation by cystatins has been intensively studied for nematode cystatins (Hartmann and Lucius, Reference Hartmann and Lucius2003; Klotz et al. Reference Klotz, Ziegler, Figueiredo, Rausch, Hepworth, Obsivac, Sers, Lang, Hammerstein, Lucius and Hartmann2011b ; Sun et al. Reference Sun, Liu, Li, Chen, Liu, Liu and Su2013; Schuijs et al. Reference Schuijs, Hartmann, Selkirk, Roberts, Openshaw and Schnoeller2016). Indeed, nematode cystatins hold promise for treating inflammatory diseases (Schnoeller et al. Reference Schnoeller, Rausch, Pillai, Avagyan, Wittig, Loddenkemper, Hamann, Hamelmann, Lucius and Hartmann2008; Danilowicz-Luebert et al. Reference Danilowicz-Luebert, Steinfelder, Kuhl, Drozdenko, Lucius, Worm, Hamelmann and Hartmann2013; Whelan et al. Reference Whelan, Rausch, Ebner, Gunzel, Richter, Hering, Schulzke, Kuhl, Keles, Janczyk, Nockler, Wieler and Hartmann2014; Coronado et al. Reference Coronado, Barrios, Zakzuk, Regino, Ahumada, Franco, Ocampo and Caraballo2017). In platyhelminths, cystatins are present in all groups/species that have been examined (Cuesta-Astroz et al. Reference Cuesta-Astroz, Scholte, Pais, Oliveira and Nahum2014; Kang et al. Reference Kang, Ju, Lee, Kim, Pak, Sohn and Na2014; Guo, Reference Guo2015). Partial biochemical and/or functional characterizations have been reported for cystatins from the genus Schistosoma (Morales et al. Reference Morales, Furtado and Rumjanek2004; He et al. Reference He, Cai, Ni, Li, Zong and He2011; Cuesta-Astroz et al. Reference Cuesta-Astroz, Scholte, Pais, Oliveira and Nahum2014; Yang et al. Reference Yang, Liu, Yue, Chen, Song, Zhan and Wu2014; Chen et al. Reference Chen, He, Hou and He2017). Of these, an I25B-group cystatin from Schistosoma japonicum has demonstrated immune-regulatory properties, being capable to regulate macrophage activation (Yang et al. Reference Yang, Liu, Yue, Chen, Song, Zhan and Wu2014) and to attenuate arthritis (Liu et al. Reference Liu, Cheng, Pappoe, Hu, Wen, Luo, Wang, Deng, Xie, Xu and Shen2016) and colitis in mouse models (Wang et al. Reference Wang, Xie, Yang, Wang, Yan, Zhong, Xu, Zhang, Liu and Shen2016). In addition to Schistosoma, cystatins belonging to the I25A group were reported in the carcinogenic trematode Clonorchis sinensis (Kang et al. Reference Kang, Lee, Sohn and Na2011, Reference Kang, Ju, Lee, Kim, Pak, Sohn and Na2014), and in the liver fluke Fasciola gigantica (Tarasuk et al. Reference Tarasuk, Vichasri Grams, Viyanant and Grams2009; Siricoon et al. Reference Siricoon, Grams and Grams2012). In F. hepatica, only a multidomain cystatin, expressed in newly excysted juvenile (NEJ) and adult, has been described; two of its five domains present potent inhibitory activity on fluke cysteine proteinase Ls (Khaznadji et al. Reference Khaznadji, Collins, Dalton, Bigot and Moire2005). In addition, a F. hepatica sequence encoding a putative single-domain cystatin was deposited in GenBank (accession AAV68752.1), but with no further characterization.
In the present work, we surveyed the F. hepatica cystatin complement in the available sequence databases and found sequences related to at least three proteins belonging to I25 family (FhStf-1, -2 and -3). We cloned and expressed these three proteins in Escherichia coli, demonstrating their functionality as inhibitors and analysing their oligomeric states and expression in adult F. hepatica excretion/secretion (E/S) products and tissues. The recombinant F. hepatica cystatins had a strong inhibitory activity against F. hepatica cysteine proteinases and showed distinct inhibitory profiles against mammalian lysosomal cathepsin L, B and C proteinases.
MATERIALS AND METHODS
Sequence data and phylogenetic analyses
In order to identify cystatin-like proteins in flatworms, we surveyed the available sequence databases, namely WormBase parasite (http://www.parasite.wormbase.org/) and GenBank (http://www.ncbi.nlm.nih,gov/) for eight different flatworms (F. hepatica, F. gigantica, Schistosoma mansoni, S. japonicum, Echinostoma caproni, C. sinensis, Opisthorchis viverini, Echinococcus granulosus, Schmidtea mediterranea). We used two different approaches to identify cystatin-encoding genes: (1) by Pfam ID (Pfam00031) or (2) by tblastN using the previously identified F. hepatica cystatin (accession number: AAV68752.1) as a query. Human I25 family sequences were retrieved from Universal Protein resource, UniProt (http://www.uniprot.org).
Phylogenetic analyses were performed following a pipeline similar to that used previously for Schistosoma cystatins (Cuesta-Astroz et al. Reference Cuesta-Astroz, Scholte, Pais, Oliveira and Nahum2014). Briefly, we used the Decrease Redundancy tool (http://www.expasy.org) to remove sequences too distantly related. Decrease redundancy parameters were set as 98% for maximum similarity and 30% for minimum similarity. Sequence alignment was performed using MAFFT 7 (Katoh and Standley, Reference Katoh and Standley2013) and MEGA 7.0 (Kumar et al. Reference Kumar, Stecher and Tamura2016) and using conserved pfam00031 domain and protein signatures (G, QxVxG and PW or LP motifs) from each sequence (Cuesta-Astroz et al. Reference Cuesta-Astroz, Scholte, Pais, Oliveira and Nahum2014). To reconstruct the phylogenetic tree, we performed the maximum likelihood method using PhyML v3.0 (Guindon et al. Reference Guindon, Dufayard, Lefort, Anisimova, Hordijk and Gascuel2010) and bootstrap (1000 replicates) methods for branch support, and the tree was visualized and edited using Evolview (http://www.evolgenius.info).
Signal sequence and trans-membrane domain predictions in FhStefin sequences were performed by using the SignalP 4.0 (Petersen et al. Reference Petersen, Brunak, von Heijne and Nielsen2011) and TMHMM 2.0 (Krogh et al. Reference Krogh, Larsson, von Heijne and Sonnhammer2001) software tools, respectively.
Parasites
Mature F. hepatica flukes were obtained from the bile ducts of infected cattle from an abattoir in the metropolitan region of Porto Alegre, Brazil. Liver flukes were extensively washed in 10 mm phosphate-buffered saline (PBS) pH 7·3 at 37 °C. Live adult flukes were used immediately for culture or stored at −80 °C until use. Eggs were collected from bovine bile by sequential filtering through 300 and 50 µm sieves (Costar, Corning Co., NY, USA). The 50 µm retained fraction, highly enriched in eggs, were collected, thoroughly washed in distilled water and kept at 4 °C until use. Miracidia were obtained from hatching eggs after 10 days at 26 °C. NEJs were obtained from in vitro excystment of metacercaria as previously described (Carmona et al. Reference Carmona, Dowd, Smith and Dalton1993).
Parasite extracts
Adult fluke E/S products were obtained as previously described (Dalton and Heffernan, Reference Dalton and Heffernan1989). Briefly, live liver flukes were cultured in RPMI-1640, pH 7·3, 30 mm 4-(2-hydroxyethyl)piperazine-1-ethanesulfonic acid, 2% glucose, 25 mg L−1 gentamicin (1 adult mL−1 media), for 6 h at 37 °C. The resulting culture medium was centrifuged at 20 000 g for 1 h at 4 °C, filtered through a 0·22 µm pore size membrane (Millipore Co., MA, USA) and concentrated by ultra-filtration using a 10 kDa cut off membrane (Amicon, Millipore Co., MA, USA). Adult liver fluke somatic extract was prepared as previously described (Cancela et al. Reference Cancela, Carmona, Rossi, Frangione, Goni and Berasain2004). Briefly, 10 mL of cold PBS per gram (wet weight) of flukes was added and the parasites were homogenized in a glass tissue grinder and sonicated in ice bath five times with 60 s bursts at 20% power followed by 30 s pauses. After centrifugation at 20 000 g for 30 min at 4 °C, supernatant was stored at −80 °C until use. Adult deoxycholate (DOC) extract was prepared as previously described (Acosta et al. Reference Acosta, Goni and Carmona1998). Briefly, 10 adult flukes were incubated in a solution consisting of 10 mL of 1% w/v deoxycholic acid in 0·15 m glycine buffer (pH 9·0), 0·5 m NaCl for 60 min at room temperature (RT), 30 min at 37 °C and then 30 min at 4 °C. The material was then centrifuged at 20 000 g for 30 min and supernatant stored at −80 °C until use.
RNA isolation and cDNA synthesis
Total RNA was extracted from adult flukes, eggs, miracidia and NEJs using the TRIzol reagent (Thermo Fisher Scientific, MA, USA). Genomic DNA contamination was removed by DNase I digestion (Thermo Fisher Scientific, MA, USA). Two hundred microgram of total RNA were reverse transcribed using M-MLV reverse transcriptase cDNA Synthesis Kit (Thermo Fisher Scientific, MA, USA) according to the manufacturer's instructions.
Cloning and recombinant expression of F. hepatica stefins in E. coli
Coding DNA sequences (CDSs) of mature F. hepatica Stefin-1, -2 and -3 (FhStf-1, -2 and -3) were PCR amplified from adult cDNA (prepared from adult parasite collected from bovine naturally infected), using gene-specific primers (see Supplementary Table S1). PCR reactions were performed in 50 µL volumes using 1·5 U Taq DNA polymerase Hifi (Thermo Fisher Scientific, MA, USA), 1·5 mm MgCl2, 0·2 mm of each dNTP and 200 nm specific forward and reverse primers. Cycling conditions were as follow: denaturation at 94 °C for 180 s followed by 35 cycles at 94 °C for 60 s; 55 °C for 45 s and 72 °C for 60 s. Amplicons obtained were ligated into the pCR21-TA cloning vector and plasmid DNA extracted and confirmed by sequencing. CDSs were subcloned into the pGEX-4T2 expression vector using EcoRI restriction enzyme. Orientation of inserts was evaluated by PCR using gene- and vector-specific primers and confirmed by plasmid DNA sequencing using pGEX forward and reverse vector primers.
Expression of recombinant FhStf-1, -2 and -3 was carried out in BL21 E. coli RIL strain (GE Healthcare, LT, UK) using 0·1 mm isopropyl β-D-1-thiogalactopyranoside for 3 h at 37 °C. Soluble bacterial extracts were prepared by resuspending bacterial pellets in PBS and then applying five pulses of ultrasound (30 s each, potency 20%) with an ultrasonic homogenizer 4710 (Cole-Palmer Instruments, IL, USA). Recombinant proteins were purified from bacterial supernatants by affinity chromatography in glutathione-sepharose 4B (GE Healthcare, LT, UK), followed by thrombin cleavage to separate them from the glutathione-S-transferase (GST) tags. Protein concentration was determined by using the Qubit fluorometric quantification kit (Thermo Fisher Scientific, MA, USA) and sample purity assessed by sodium dodecyl sulphate-12% polyacrylamide gel electrophoresis (SDS-PAGE) (Laemmli, Reference Laemmli1970).
Preparation of polyclonal antisera
Polyclonal antisera against rFhStf-1 and -3 were prepared by immunizing rabbits with 150 µg of each recombinant protein, in emulsion with complete Freund's adjuvant (Sigma, MO, USA) for priming. Two weeks after priming, a booster with 150 µg of each antigen in emulsion with incomplete Freund's adjuvant (Sigma, MO, USA) was administered, followed by the administration of two additional identical boosters at 2-week intervals, in order to enhance specific IgG antibody titres.
Purification of native cystatin-like inhibitors from adult fluke extract
Adult fluke somatic extract was incubated at 100 °C for 10 min and then left on ice until cool. The heated extract was centrifuged at 20 000 g for 30 min at 4 °C, and the supernatant was loaded onto a carboxymethyl-papain (CP) sepharose 4B affinity chromatography column, as described (Abrahamson, Reference Abrahamson1994). After overnight (ON) incubation at 4 °C, the resin was washed with 20 volumes of 20 mm sodium phosphate buffer pH 7·6 and then with one volume of 1 m NaCl. Papain-bound proteins were eluted with 20 mm sodium phosphate buffer pH 11·5, 0·1 m NaCl and eluted fractions were then neutralized in PBS. Purity and inhibitory activity were assessed by SDS-PAGE and enzymatic assay, respectively.
Inhibitory activity of native and recombinant cystatins
The inhibitory activities of native FhStf-1 and rFhStf-1, -2 and -3 were assessed using commercial papain (Sigma, MO, USA) and mammalian lysosomal cysteine proteinase (Sigma, MO, USA): cathepsin B (bovine spleen), cathepsin L (human liver) and cathepsin C (bovine spleen). To test the inhibition of F. hepatica cysteine proteinases FhCL1 and 3 (FhCL1 and 3, respectively), these enzymes were expressed in the yeast Hansenula polymorpha as previously described (Corvo et al. Reference Corvo, Cancela, Cappetta, Pi-Denis, Tort and Roche2009). Assay buffers used were as follows: 100 mm sodium acetate, pH 5·5, 100 mm NaCl, 1 mm EDTA and 0·005% Triton X-100 for bovine cathepsin C, human cathepsin L and papain; 100 mm sodium acetate, pH 5·5, 60 mm NaCl, 1 mm EDTA for bovine cathepsin B and 50 mm phosphate buffer pH 7·0, 1 mm DL-dithiothreitol (DTT), 1 mm EDTA for FhCL1 and 3. Enzymatic activity were monitored using different 7-amido-4-methylcoumarin (MCA) fluorogenic substrates (Bachem, CA, USA): Z-Phe-Arg-MCA for cathepsin L and papain; Z-Arg-Arg-MCA for cathepsin B, H-Gly-Phe-MCA for cathepsin C, Boc-Val-Leu-Lys-MCA for FhCL1 and Tosyl-Gly-Pro-Arg-MCA for FhCL3. Enzymes (10–125 nm) were assayed at 37 °C in a microplate well (100 µL volume). FhCL1 and 3 were previously activated by incubation in 0·1 m citrate buffer pH 4 containing 1 mm DTT and 1 mm EDTA, for 3 h at 37 °C. The release of 4-MCA (NHMec) was monitored in a Spectramax Microplate Reader, Molecular Devices Corporation, CA, USA (355 nm excitation and 460 nm emission). For inhibition constant determinations, different concentrations of cystatins (0–1000 nm) were incubated for 30 min with each enzyme in the reaction buffer prior to the addition of substrate. Recombinant GST was used as a negative control (0–1000 nm) to test inhibitory specificity of F. hepatica stefins (FhStefins). Data analysis and Ki determinations were performed using non-lineal regression (Morrison, Reference Morrison1969) using the GraphPad Prism 5.0 Software (http://www.graphpad.com).
Western-blot and far-Western-blot analyses
After electrophoresis, proteins were electrotransferred onto nitrocellulose membranes (0·22 mm Hybond-ECL, GE Healthcare, LT, UK) for 60 min at 15 V, and the membranes were blocked in PBS containing 0·1% Tween 20 and 5% skimmed milk [blocking solution (BS)], ON at 4 °C. For immunoblot, the membrane was probed with rabbit polyclonal antisera raised against rFhStf-1 and -3, diluted (1:10·000) in BS, for 60 min at RT. For far-Western blot, the membrane was probed with 10 µg mL−1 E/S products diluted in BS, 3 h at RT; after five washes in PBS-0·1% Tween 20, the membrane was incubated with sheep polyclonal antiserum against FhCL1, diluted 1:5000 in BS, for 1 h at RT. To detect bound antibodies, membranes were washed as described above and probed with horseradish peroxidase-conjugated antirabbit IgG (GE Healthcare, LT, UK, 1:8000) or antisheep IgG (Sigma, MO, USA; 1:7000), during 1 h at RT. A chemiluminescent substrate (ECL Western-blot detection kit, GE Healthcare, LT, UK) was used for detection, in the Versadoc Imaging System (Bio-rad, CA, USA).
Transcriptional analysis of FhStefins in different developmental stages
Transcriptional analysis of FhStefins was performed by semi-quantitative PCR using cDNA from adults, eggs, miracidia and NEJ and gene-specific primers for Fh cystatins (see Table 1). Fasciola hepatica actin gene was used as internal control. Briefly, equal amount of total RNA (200 ng) was reverse transcribed as describe above and PCR reactions were performed using Taq DNA polymerase (Thermo Fisher Scientific, MA, USA). PCR conditions included an initial denaturation at 94 °C for 30 s followed by 35 cycles of 30 s at 94 °C, 60 s at 60 °C, 90 s at 72 °C and a final extension at 72 °C for 10 min. Amplicons were analysed by 1·2% agarose gel electrophoresis.
Table 1. Inhibition kinetics parameters of recombinant F asciola hepatica stefins

Mass spectrometry
Native and recombinant stefins FhStf-1, -2 and -3 were trypsin-digested and peptides were analysed by liquid chromatography tandem-mass spectrometry as previously described (Reolon et al. Reference Reolon, Martello, Schrank and Ferreira2014) using Q-TOF Ultima API mass spectrometer (Micromass, Water Co., MA, USA). Peptide masses were compared with theoretical masses using the MASCOT software version 2.3.02 against a local F. hepatica database containing deduced amino acid sequences from genome annotation as available on WormBase parasite database and those obtained in this work.
Cross-linking assay
Cross-linking of recombinant stefins was carried out by using the bifunctional reagent glutaraldehyde, as previously described (Darawshe et al. Reference Darawshe, Tsafadyah and Daniel1987). Briefly, a 1 mg mL−1 protein solution was incubated with 0·1% glutaraldehyde at RT. At different times, 10 µL samples were withdrawn and reactions were stopped by heating in SDS sample buffer and analysed by SDS-12% PAGE under reducing conditions.
Immunolocalization of FhStf-1 and -3 in parasite tissue
Adult liver flukes were fixed ON in 4·5% paraformaldehyde in PBS. Parasites were washed, dehydrated and embedded in paraffin wax. Tissue sections were cut 10 µm thick, deparaffinized, hydrated and soaked in PBS for 5 min. Then the slides were blocked in 1% glycine in PBS and 4% BSA in PBS each for 30 min at RT. The sections were incubated in a humid chamber for 1 h at RT with anti-rFhStf-1 or anti-rFhStf-3 or non-immune sera diluted 1:300 v/v in 1% BSA in PBS. After three washes with PBS, sections were incubated in a humid chamber for 1 h at RT with goat anti-rabbit IgG conjugated with Alexa Fluor 488 (Molecular Probes, Thermo Fisher Scientific, MA, USA) that was diluted 1:250 v/v in 1% BSA in PBS. After three washes with PBS, the sections were then incubated with 25 nm 4′,6-diamidino-2-phenylindole (DAPI, Molecular Probes, Thermo Fisher Scientific, MA, USA) for 20 min at RT and mounted with Fluoromount. Sections were observed under the confocal microscope (Olympus FluoView 1000, Olympus, PA, USA). The images were digitally captured and processed using the Olympus FluoView version 2.1c and the Olympus FluoView version 3.0 Viewer software (http://www.photonics.com/).
RESULTS
Sequence identification and phylogenetic analysis of cystatin-like inhibitors in F. hepatica
By mining F. hepatica sequences in the databases (WormBase parasite, GenBank), we found at least three different sequences corresponding to putative single-domain cystatin-like inhibitors. The three sequences were encoded in genes composed by three exons and two introns (Supplementary Fig. S1A). The shortest sequence (D915_13848_Fhep), named FhStf-1, had a CDS of 300 bp and encoded a 99 amino acid residue polypeptide with no signal peptide, as expected for an I25A clade member (stefin) (Supplementary Fig. S1B and C). The second sequence, (D915_14396_Fhep) was identical to previously deposited mRNA sequence with accession AAV68752.1. This sequence and a third one (D915_01512_Fhep) had CDS of 351 and 348 bp, encoding proteins of 116 and 115 amino acid residues, respectively. These two proteins were named FhStf-2 and 3, respectively, for reasons that will be explained below. These three sequences were aligned with platyhelminth cystatin-like proteins and with human I25A and I25B subfamily members (Fig. 1A). The three FhStefins shared with human stefins A (P01040) and B (P04080) the conserved inhibitory domain signature QXVXG, the conserved N-terminal glycine residue and C-terminal LP or LY motif characteristic of I25A subfamily; also in line with their placement in the I25A subfamily, none of the three FhStefins contained the PW motif characteristic of the I25B subfamily. However, unusually for stefins, all three FhStefins contain cysteine residues: four in FhStf-1, and four and three in mature FhStf-2 and -3, respectively (Supplementary Fig. S1C). Further, FhStf-2 and -3 (but not FhStf-1) contain predicted N-terminal signal peptides, normally not found in stefins (Supplementary Fig. S1B and C).
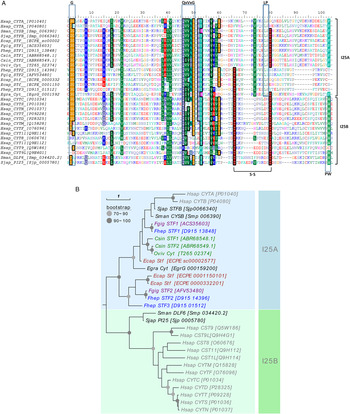
Fig. 1. Sequence and phylogenetic analysis of single-domain cystatins. (A) Alignment of the amino acid sequences of Fasciola hepatica single-domain cystatins here identified with other platyhelminth and mammalian cystatins. Cystatin motifs (GG, QxVxG and LP or PW) and disulfide bridges are highlighted. Alignment is coloured based on amino acid properties (back coloured if conserved over 60% of alignment). (B) Phylogenetic tree was generated using the maximum likelihood method and visualized using Evolview. Cystatins obtained from different sequence databases are described by their accession numbers (WormBase parasite, Uniprot). Species included (colour coded) are the trematodes Fasciola hepatica (Fhep), Fasciola gigantica (Fgig), Echinostoma caproni (Ecap), Clonorchis sinensis (Csin), Schistosoma japonicum (Sjap) and Schistosoma mansoni (Sman), Opisthorchis viverrini (Oviv), the cestode Echinococcus granulosus (Egran) and the mammalian Homo sapiens (Hsap).
We next carried out a phylogenetic analysis of F. hepatica stefins together with related sequences identified in platyhelminths and humans. In the resulting phylogenetic tree (Fig. 1B), FhStf-1 was assigned to the clade I25A, which comprises the classical stefin-like proteins. Within this clade, FhStf-1 grouped with its F. gigantica orthologue (ACS35603), with which it has almost 94% sequence similarity. The other two F. hepatica stefins, however, also grouped with the I25A subfamily but in a divergent clade, separate from classical stefins and together with two E. caproni stefins. Human cystatins sensu stricto as well as sequences from S. japonicum (Sjp_0005780), S. mansoni (Smp_034420.2) grouped together as a separated clade composed by members of the I25B subfamily. The F. gigantica orthologue for FhStf-2 was identified (AFV53480, with 94% similarity), whereas for FhStf-3 no orthologue sequence was identified. The facts that the phylogenetic analysis grouped FhStf-2 and -3 with subfamily I25A rather than subfamily I25B and that FhStf-2 is the orthologue of a F. gigantica protein previously named as stefin (Siricoon et al. Reference Siricoon, Grams and Grams2012) led us to name FhStf-2 and -3 as ‘stefins’. It must nonetheless be stressed that signal peptides, present in FhStf-2 and -3, are usually absent in stefins and instead present in true cystatins.
Several cysteine proteinase-binding proteins can be detected in F. hepatica extracts
In order to detect cysteine proteinase inhibitors in liver fluke extracts, a far-Western approach was performed. E/S products, somatic extract and DOC membrane extracts were separated by electrophoresis and then transferred to nitrocellulose membranes. These membranes were probed with E/S products (very rich in cysteine proteinase L) and then an anti-FhCL1 antibody was used to detect bound cysteine proteinase L. We observed several bands (8 to 20 kDa) corresponding to cysteine proteinase-binding proteins (Fig. 2A) and bands (~27 kDa) due to cathepsin L mature enzymes also present in the extracts being probed. In order to obtain more information on the cysteine proteinase-binding proteins, we fractionated adult E/S products, DOC extract and somatic extract by affinity chromatography on immobilized CP. Of these materials, only the somatic extract allowed us to obtain a purified protein. This protein did not precipitate upon boiling of the original extract and had an apparent molecular mass of 10 kDa, as expected for a cystatin-like molecule (Fig. 2B). Mass spectrometry analysis identified peptides corresponding to a F. hepatica FhStf-1 (Supplementary Fig. S2), and the purified native protein showed potent inhibitory activity against papain (Ki = 3·32 nm) and F. hepatica cysteine proteinases L1 (Ki = 22·42 nm) and L2 (Ki = 3·8 nm).

Fig. 2. Detection and purification of cystatin-like inhibitors in adult F asciola hepatica extracts. (A) Detection of cystatin-like inhibitors by far-Western blotting, using as probe adult ES product (ES, containing high levels of cathepsin L proteinases, CLs) followed by anti-FhCL1 antibody (see Methods). Proteins from adult fluke extracts or egg cystatin (used as a control) were mixed in SDS-sample buffer (without reducing agent) and were not boiled before electrophoresis. The reactivity observed only in lanes probed with ES corresponds to cysteine proteinase binding-, cystatin-like proteins. The reactivity observed both in lanes probes with ES and in control lanes corresponds to CLs found in the parasite extracts loaded in the gel. EggCys, egg white cystatin (positive control); DOC, deoxycholate extract; S, somatic extract. (B) Purification of cystatins using carboxymethyl-papain agarose, assessed by SDS-15% PAGE. S, somatic extract; bS, somatic extract after boiling; Nb, non-bound fraction; E1 and E2, eluted fractions.
Expression of functional F. hepatica cystatins in E. coli
To perform a functional characterization of F. hepatica cysteine proteinase inhibitors, we cloned the full length CDS (excluding nucleotides encoding signal peptide residues) and expressed the corresponding recombinant mature stefin-like proteins (rFhStf-1, -2 and -3) in E. coli as GST fusion proteins (Fig. 3A). High yields (~10 mg L−1 culture medium) of the pure recombinant proteins were obtained, with the expected apparent molecular weights (MW) of ~10 kDa in the three cases (Fig. 3B). Mass spectrometry analysis confirmed the identity of the three recombinant F. hepatica stefins (Supplementary Fig. S2).

Fig. 3. Recombinant expression of functional Fasciola hepatica stefins in Escherichia coli. (A) The SDS-12% PAGE analysis illustrating the recombinant expression of rFhStf-1, -2 and -3. Selected purification steps are shown: induction (I), fusion protein bound to glutathione-sepharose 4B (R) and protein recovery after thrombin cleavage. rGST-FhStf, recombinant stefins fused to GST; rFhStf, recombinant stefin free of GST; MW, molecular weight markers. (B) Inhibition of papain activity by recombinant FhStf-1, -2 and -3. Activity is given in terms of arbitrary fluorescence units (RFL) per unit time (min).
Fluorogenic enzymatic assays were performed with each of the three recombinant F. hepatica stefins. A strong inhibition of papain activity (nm range) was observed for all of them (Fig. 3C), confirming that active recombinant cysteine proteinase inhibitors were produced in E. coli. Inhibitory activity was not observed when we used GST, instead of cystatin, in the enzymatic assay.
Inhibition kinetics of recombinant F. hepatica cystatins
Inhibitory assays were performed in order to assess specificity of F. hepatica stefins vs parasite and host enzymes. Potent inhibition of the proteolytic activities of recombinant FhCL1 or 3 was observed for rFhStf-1, -2 and -3. The Ki values for the inhibition of FhCL1 (an enzyme predominantly expressed by the adult stage) by the three recombinant inhibitors were similar and in the nm range (Table 1), whereas those for the juvenile-specific FhCL3 enzyme were an order of magnitude higher. A similar potent inhibition profile (with Ki in the nm range for the three rFhStefins) was observed with human cathepsin L. In contrast, only FhStf-2 was capable to inhibit host cathepsin B with a Ki in the nm range. Interestingly, both rFhStf-1 and -2 inhibited bovine cathepsin C with a high affinity (35 and 57 nm, respectively) whereas rFhStf-3 displayed no significant inhibition (Ki > 1 µ m).
Oligomerization behaviour of F. hepatica cystatins
Cross-linking experiments showed differences in the oligomerization tendency of the rFhStf-1, -2 and -3. For rFhStf-1, monomers and dimers (~20 kDa) were the predominant forms detected, and forming tetramers as the highest MW species detectable (Fig. 4A). rFhStf-2 exhibited an apparently balanced distribution from monomers (10 kDa) to oligomeric (18–50 kDa) and higher mass multimeric forms (Fig. 4B). rFhStf-3 had a clearly stronger tendency to form very high molecular mass oligomers (over 100 kDa) appearing mostly in the form of high MW species towards the end of the assay (Fig. 4C). Moreover, rFhStf-3 monomeric and dimeric forms disappeared towards the end of assay, while for rFhStf-1 and -2 they remained detectable.

Fig. 4. Oligomer formation by Fasciola hepatica stefins. Recombinant FhStf-1 (rFhStf-1) (A), FhStf-2 (rFhStf-2) (B) and FhStf-3 (rFhStf-3) (C) were incubated with 0·1% glutaraldehyde for the times indicated (min). Cross-linked products were analysed by SDS-12% PAGE followed by Coomassie Brilliant Blue R staining. Lanes indicated ‘C’ correspond to controls without glutaraldehyde. MW, molecular weight markers. T, tetramer; t, trimer; d, dimer; m, monomer.
Transcriptional profile of cystatins in different developmental stages
We performed RT-PCR analysis using specific primers in order to gain insight into the transcriptional pattern of FhStf-1, -2 and -3 across fluke developmental stages. All three FhStefins mRNAs were detected in all developmental stages tested, namely adult, eggs, miracidia and NEJs (Fig. 5). Both FhStf-1 and -3 were constitutively transcribed, but FhStf-1 appeared to have a higher level of transcription than FhStf-3. Interestingly, the transcription of FhStf-2 mRNA gradually increased during parasite maturation, from the egg to the adult stage.

Fig. 5. RT-PCR analysis of FhStefins expression in different larval stages of Fasciola hepatica. PCR reactions were carried out using gene-specific primers (FhStf-1, -2 and -3, and actin as an internal control), and cDNA prepared from adult (A), egg (E), miracidia (M) and newly excysted juvenile (N), as described in Material and Methods.
Detection of native FhStf-1 and -3 in E/S products and extracts of adult flukes
To demonstrate whether the differences observed at the transcriptional level of FhStf-1 and -3 are correlated with a lower protein expression of FhStf-3 in parasitic extracts, we performed a Western-blot and immunolocalization analyses (see below). Using an antiserum raised against rFhStf-1, we detected a band of the expected molecular mass (10 kDa) in E/S products, as well as somatic and DOC extracts of adult flukes (Fig. 6A). In contrast, the anti-FhStf-3 serum, in spite of clearly recognizing rFhStf-3, showed only faint reactivity against the membrane-enriched DOC extract (Fig. 6B), in lanes loaded with the same protein amounts as for FhStf-1.

Fig. 6. Detection of FhStf-1 and -3 in adult Fasciola hepatica extracts. Western-blot analysis of adult F. hepatica protein extracts (20 µg lane−1) using anti-rFhStf-1 (A) and anti-rFhStf-3 (B). ES, E/S products; D, soluble deoxycholate extract; S, soluble somatic extract. The recombinant FhStf-1 (Stf-1), FhStf-2 (Stf-2) and FhStf-3 (Stf-3) (100 ng of each) were loaded as controls. Arrows indicate the migration of FhStf-1 in SDS-PAGE.
Immunolocalization of F. hepatica cystatins in parasite tissues
FhStf-1 and -3 were immunolocalized in paraffin-tissue sections from adult parasites (Fig. 7). The FhStf-3 antiserum gave only a weak signal, restricted to the intestinal epithelium and the tegument (Fig. 7A). In contrast, FhStf-1 was detected clearly, in the intestinal epithelium, the tegumental cells including specifically those of ventral suckers (Fig. 7B, F and G), and in the reproductive organs, in the ovary (Fig. 7D) and the periphery of testes containing primary spermatogonia (Fig. 7E).

Fig. 7. Immunolocalization of FhStf-1 and -3 in adult F. hepatica. Paraffin-embedded tissue sections (5 µm thick) were probed with 1:300 dilutions of anti-rFhStf-3 (A), anti-rFhStf-1 serum (B, D–G), and pre-immune serum (C) and revealed with Alexa 488 bound secondary IgG antibody (green). Nuclei were counterstained with DAPI (blue). Ca, caecum; Ov, ovarium; Tc, tegumental cells; Te, testis; Tg, tegument; Vs, ventral sucker.
DISCUSSION
During host infection, parasitic organisms release a diverse repertoire of molecules for successful establishment and reproduction inside their hosts. In the case of F. hepatica, the most prominent among such molecules are papain-family cysteine proteinases (Collins et al. Reference Collins, Stack, O'Neill, Doyle, Ryan, Brennan, Mousley, Stewart, Maule, Dalton and Donnelly2004; Cancela et al. Reference Cancela, Acosta, Rinaldi, Silva, Duran, Roche, Zaha, Carmona and Tort2008; Robinson et al. Reference Robinson, Dalton and Donnelly2008a ; Beckham et al. Reference Beckham, Piedrafita, Phillips, Samarawickrema, Law, Smooker, Quinsey, Irving, Greenwood, Verhelst, Bogyo, Turk, Coetzer, Wijeyewickrema, Spithill and Pike2009). Because these proteinases are potentially harmful for both host and parasite tissues, mechanisms to regulate their activity have evolved. Previous studies demonstrated the presence of two I25A cystatins (FgStefin-1 and -2) in somatic extracts and excretion–secretion products of adult F. gigantica (Tarasuk et al. Reference Tarasuk, Vichasri Grams, Viyanant and Grams2009; Siricoon et al. Reference Siricoon, Grams and Grams2012). It was suggested that both of these inhibitors may have extracorporeal functions, inhibiting both parasite and host cysteine proteinases (Tarasuk et al. Reference Tarasuk, Vichasri Grams, Viyanant and Grams2009; Siricoon et al. Reference Siricoon, Grams and Grams2012). In the present work, we identified three single-domain cystatin-like cysteine proteinase inhibitors expressed by F. hepatica, named FhStf-1, -2 and -3. Phylogenetic analysis revealed that the identified inhibitors fall in two different clades. FhStf-1 grouped with F. gigantica Stefin-1 (Tarasuk et al. Reference Tarasuk, Vichasri Grams, Viyanant and Grams2009) (of which it is the probable orthologue), and most other I25A members previously identified in platyhelminths (Cuesta-Astroz et al. Reference Cuesta-Astroz, Scholte, Pais, Oliveira and Nahum2014; Kang et al. Reference Kang, Ju, Lee, Kim, Pak, Sohn and Na2014; Guo, Reference Guo2015), as well as their mammalian orthologues. In contrast, FhStf-2 and -3, together with certain E. caproni stefins, grouped in a separate clade, within the I25A subfamily but clearly separate from classical stefins. Our analysis also suggested that FhStf-2 is the orthologue of previously described F. gigantica Stefin-2 (Siricoon et al. Reference Siricoon, Grams and Grams2012). FhStf-3 represents a novel cystatin from the genus Fasciola, but it should not be ruled out that an orthologue is present in F. gigantica, species of which the genome has not been sequenced.
The identification of the atypical subgroup of I25A proteins in Fasciola (comprising FhStf-2 and -3) with the presence of signal peptide and absence of PW motif is in line with previous evidence that the evolution of cystatin-like inhibitors in platyhelminths does not follow the patterns known in mammals. Other examples exist of platyhelminth I25 family members displaying combinations of features that were deemed exclusive of different subfamilies from studies in mammals (Cuesta-Astroz et al. Reference Cuesta-Astroz, Scholte, Pais, Oliveira and Nahum2014; Guo, Reference Guo2015). This evolutionary divergence in the extracellular cystatins could reflect functional diversification. In agreement with this idea, enzymatic inhibition assays demonstrated that F. hepatica cystatins have different target enzyme specificities. FhStf-2 was capable to inhibit not only mammalian cathepsin B, as previously demonstrated for its F. gigantica orthologue (Siricoon et al. Reference Siricoon, Grams and Grams2012), but also cathepsins C and L, suggesting that this inhibitor may regulate a broad range of host proteinases. Interestingly, FhStf-3 displayed a more restricted activity, inhibiting human cathepsin L and papain but not human cathepsins B and C. The three rFhStfs tested were capable of inhibiting human cathepsin L (as well as FhCL1 and 3, as discussed below), perhaps reflecting that inhibition of cathepsin Ls is an evolutionarily conserved function for this class of inhibitors. The possibility of inhibition by F. hepatica stefins of host proteinases, including cysteine cathepsins involved in antigenic processing, may have implications for immune modulation in fasciolosis. Lysosomal cathepsin L and cathepsin B can be inhibited by parasitic nematodes Nippostrongylus brasiliensis inducing suppression of antigen specific immune responses (Dainichi et al. Reference Dainichi, Maekawa, Ishii, Zhang, Nashed, Sakai, Takashima and Himeno2001). In the parasitic nematode Heligmosomoides polygyrus cystatin can interfere with dendritic cell maturation (Sun et al. Reference Sun, Liu, Li, Chen, Liu, Liu and Su2013) whereas an I25B cystatin from S. japonicum was capable to induce macrophage activation to M2 phenotype and an anti-inflammatory response (Yang et al. Reference Yang, Liu, Yue, Chen, Song, Zhan and Wu2014). In vivo inhibition of lysosomal cysteine proteinases by FhStefin and its role in immunoregulation warrant further investigations. In the present work, we found potent inhibitory activity of recombinant FhStf-1, -2 and -3 against F. hepatica cysteine proteinases FhCL1 and 3. In addition, we observed inhibition of F. hepatica cysteine proteinase FhCL2 by native FhStf-1. The expression of these three single-domain cystatins together with a multidomain cystatin previously reported (Khaznadji et al. Reference Khaznadji, Collins, Dalton, Bigot and Moire2005) could reflect the need to control unwanted proteolysis by the diverse complement of cysteine proteinases highly expressed by F. hepatica.
FhStf-1 was shown by RT-PCR to be constitutively transcribed throughout F. hepatica life cycle. At the protein level, we found FhStf-1 not only in somatic extracts, but also in E/S products, and DOC extracts of adult flukes, suggesting that this stefin is secreted by non-classical secretory pathways. This conclusion is in agreement with a previous proteomics analysis that identified FhStf-1 in extracellular vesicles released by adult F. hepatica (Cwiklinski et al. Reference Cwiklinski, de la Torre-Escudero, Trelis, Bernal, Dufresne, Brennan, O'Neill, Tort, Paterson, Marcilla, Dalton and Robinson2015) and with evidence that its F. gigantica orthologue is found in extracorporeal secretions (Tarasuk et al. Reference Tarasuk, Vichasri Grams, Viyanant and Grams2009). Within the (adult) parasite tissues, FhStf-1 was detected in the surface lamella of the gut and in the reproductive organs, localizations that coincide with those previously reported for the cathepsin Ls (Dalton et al. Reference Dalton, Neill, Stack, Collins, Walshe, Sekiya, Doyle, Mulcahy, Hoyle, Khaznadji, Moire, Brennan, Mousley, Kreshchenko, Maule and Donnelly2003; Collins et al. Reference Collins, Stack, O'Neill, Doyle, Ryan, Brennan, Mousley, Stewart, Maule, Dalton and Donnelly2004; Zawistowska-Deniziak et al. Reference Zawistowska-Deniziak, Wasyl, Norbury, Wesolowska, Bien, Grodzik, Wisniewski, Baska and Wedrychowicz2013), suggesting that part of the biological role of this inhibitor may be to control cathepsin L activity. The F. gigantica orthologue of the mentioned F. hepatica multidomain cystatin was predominantly localized in testis and sperm (Geadkaew et al. Reference Geadkaew, Kosa, Siricoon, Grams and Grams2014), supporting the relevance of Fasciola cystatins in reproduction.
For FhStf-2, our analysis of the transcriptional profile across the parasite life cycle was in rough agreement with the previous proposal, for the F. gigantica orthologue, of an important function in the juvenile stage followed by a function in the adult stage (Tarasuk et al. Reference Tarasuk, Vichasri Grams, Viyanant and Grams2009; Siricoon et al. Reference Siricoon, Grams and Grams2012). In that proposal, the inhibitor would be in the juvenile stage control cathepsin B, which is the predominant cysteine proteinase in this stage, and function as an inhibitor of cathepsin Ls in adults; our enzymological data for FhStf-2 are compatible with this idea. FhStf-3 appeared, by RT-PCR analysis, Western blotting and immunolocalization, to have a lower and/or more restricted expression than FhStf-1. This suggests a more specialized function for this protein as compared with FhStf-1 and -2. The oligomerization experiments suggested that FhStf-2 and -3 self-associate more strongly than the FhStf-1. In C. sinensis, CsStefin-2 (more closely related to FhStf-1 than to -2) was shown to form a tetramer, which the authors suggest is the native and active state of this protein (Kang et al. Reference Kang, Ju, Lee, Kim, Pak, Sohn and Na2014). It is conceivable that the active form(s) of FhStf-2 and/or -3 are oligomeric.
In the present work, we reported and functional characterized three cystatin-like molecules expressed by the trematode F. hepatica. A distinct inhibitory profile against host lysosomal cysteine proteinases and expression pattern in different developmental stages was also demonstrated for these FhStefins. RNAi technology in F. hepatica is now possible (McGonigle et al. Reference McGonigle, Mousley, Marks, Brennan, Dalton, Spithill, Day and Maule2008; Rinaldi et al. Reference Rinaldi, Morales, Cancela, Castillo, Brindley and Tort2008) and specific gene silencing of each of the cystatins described in this work could help to assign them functions in the biology of the parasite including the cross-talk with its host. Moreover, immunomodulatory properties of recombinant FhStefins could be assessed in order to evaluate their therapeutic potential against inflammatory diseases and as new intervention targets against fasciolosis.
SUPPLEMENTARY MATERIAL
The supplementary material for this article can be found at https://doi.org/10.1017/S0031182017001093.
ACKNOWLEDGEMENTS
We would like to thank Dr Pablo Smircich and Dr Gabriel Rinaldi for helpful discussion and comments to the manuscript.
FINANCIAL SUPPORT
This work was possible due to the support of CSIC-UDELAR, ANII and PEDECIBA in Uruguay and CNPq and CAPES in Brazil (AUX-PE-PARASITOLOGIA 1278/2011). MC and EDS was supported by CAPES and CNPq.