INTRODUCTION
The Atlantic black skipjack Euthynnus alletteratus (Rafinesque, 1810) is a pelagic scombrid fish that inhabits the coastal tropical and subtropical waters of both sides of the Atlantic Ocean, including the Mediterranean Sea, Caribbean Sea and Gulf of Mexico (Collette and Nauen, Reference Collette and Nauen1983). Three main populations of E. alletteratus are known in the Atlantic Ocean, located in the central eastern, in the southeastern and in the western Atlantic Ocean (Yoshida, Reference Yoshida1979). Moreover, there is a population in the Mediterranean Sea, which is considered independent from the Atlantic ones (Gaykov and Bokhanov, Reference Gaykov and Bokhanov2008). The status of the Mediterranean population is scarcely known, although E. alletteratus is a species of commercial interest (El-Haweet et al. Reference El-Haweet, Sabry and Mohamed2013), whose fishery in the region represents up to 25% of the global catches of this species (5 230 t in 2013) (Catarci, Reference Catarci2004; FAO, 2014) and it plays a key role in the Mediterranean pelagic trophic webs (Falautano et al. Reference Falautano, Castriota, Finoia and Andaloro2007).
The parasite fauna of E. alletteratus has been investigated only in the western Atlantic Ocean (Alves and Luque, Reference Alves and Luque2006), remaining neglected in the central and southeastern Atlantic Ocean and in the Mediterranean Sea.
The aim of this study is to investigate the metazoan parasite populations of E. alletteratus from the western Mediterranean Sea, evaluating how the geographical, environmental and biological factors can influence their structure and inferring the biological and ecological traits of the species that can be derived from the spatial and temporal variations.
MATERIALS AND METHODS
Host and parasite collection
A total of 150 Atlantic black skipjacks, caught off the Iberian Peninsula in the western Mediterranean Sea between 2008 and 2011 were examined for parasites (Table 1). Environmental data, as bottom depth (Depth) and sea surface temperature (SST), from each collection site per sampling date were obtained as monthly means from the SeaWiFS dataset (http:\\oceancolor.gsfc.nasa.gov; Table 1).
Table 1. Sampling data and biological parameters of Euthynnus alletteratus from the western Mediterranean Sea

SST, sea surface temperature; FL, fish fork length, W, fish total weight, M/F/U, male, female, unknown sex; N, number of hosts according to mean FL classes (201–400/401–600/601–800 mm).
Immediately after landing, fish were measured (FL, fork length range 205–731 mm), weighed (110–6214 g) and sexed (54 males, 72 females and 44 unsexed/hybrid specimens). After each sampling, the heads (including gills and heart) of fish were excised, stored individually in plastic bags and frozen at −20 °C. Subsequently, the heads were defrosted and examined under a stereomicroscope for metazoan parasites. All parasites were identified according to their morphological characteristics, counted and stored in 70% ethanol.
Morphological and molecular analysis of didymozoid parasites
Molecular data were used to support the morphological identification of Didymozoidae, family of trematodes of fish with a highly complex and controversial taxonomy (Abe and Okamoto, Reference Abe and Okamoto2015). Parasites of each didymozoid morphotype were cut into two portions at about 500 µm to the tip of the posterior end: the anterior part was stored in ethanol 70% for morphological control and the rest dehydrated with absolute ethanol and processed according to the following protocol. Total DNA was isolated placing the samples in 200 µL of a 5% suspension of deionised water and Chelex containing 0·1 mg mL−1 proteinase K (Georgieva et al. Reference Georgieva, Selbach, Faltýnková, Soldánová, Sures, Skírnisson and Kostadinova2013). The polymerase chain reaction (PCR) amplification was performed on two loci of interest: D1-D2 domain of the ribosomal large-subunit (partial 28S) and second internal transcribed spacer (ITS-2) of DNA (Anderson and Barker, Reference Anderson and Barker1993; Chambers and Cribb, Reference Chambers and Cribb2006). Three microliters of DNA were used in 25 µL reactions using Ready-To-Go PCR beads (GE Healthcare, UK) and 2 µL of each primer. Partial 28S was amplified using primers ZX-1 (5′-ACCCGCTGAATTTAAGCATAT-3′) and 1500R (5′-GCTATCCTGAGGGAAACTTCG-3′), according to Palm et al. (Reference Palm, Waeschenbach, Olson and Littlewood2009). The ITS2 portion was amplified using primers 3S (5′-GGTACCGGTGGATCACTCGGCTCGTG-3′) and BD2 (5′-TATGCTTAAATTCAGCGGGT-3′) (Bowles et al. Reference Bowles, Hope, Tiu, Liu and McManus1993). PCR products were stained with Gel Red (Biotium, Italy) and visualized on 1·5% Tris-acetate-EDTA agarose gel electrophoresis. PCR products were purified with QIAquick PCR purification kit (QIAGEN, Italy) according to the manufacturer's instructions, and sequenced by an external core service (Macrogen Europe Inc, the Netherlands) using the PCR primers.
Newly-generated sequences were aligned using Clustal W as implemented in MEGA v6 (Tamura et al. Reference Tamura, Stecher, Peterson, Filipski and Kumar2013) and then deposited in the GenBank database (http://www.ncbi.nlm.nih.gov/Genbank/GenbankSearch.html). Species boundaries were assessed via maximum likelihood (ML) analyses of Tamura-Nei parameter distances using MEGA v6 with nodal support estimated using 1000 bootstrap resamplings (Tamura et al. Reference Tamura, Stecher, Peterson, Filipski and Kumar2013). The outgroup-rooted trees were drawn using the species Quadrifoliovarium pritchardae (GenBank accession number AY897561 for ITS2 and AY897567 for 28S) as outgroup. A six-rates custom model (general time reversible) of sequence evolution was set, allowing the estimation of proportion of invariable sites and the gamma distribution. Nodes with a percentage of bootstrap <70% were considered to be not statistically supported (Hillis and Bull, Reference Hillis and Bull1993). Voucher specimens were deposited in the Parasite Collection of the Cavanilles, Institute of Biodiversity and Evolutionary Biology, University of Valencia, Spain (PCICBiBE).
Data analysis
Differences between mean fork lengths of host specimens were evaluated with Student's t-test; the mean FL of the specimens from the Gulf of Valencia of 2011 was significantly lower than that of tuna from the southeastern Spain of the same year (Student's t = −11·824, P-value < 0·0001), the mean FL of tuna from the southeastern Spain differed among the years 2008, 2009 and 2011 (t = 5·36–7·55, P < 0·0001). Fish samples were grouped according to size as juvenile (FL = 200–400 mm) and adult specimens (401–800 mm), and then according to locality and year. Hosts were divided into five groups (Table 1): juvenile fish from the Gulf of Valencia of 2011, adult fish from the southeastern Spain of 2008, 2009 and 2011, and adult fish from the Strait of Gibraltar of 2008.
Prevalence (P%), abundance and mean abundance (mA) of each parasite species were calculated according to Bush et al. (Reference Bush, Lafferty, Lotz and Shostak1997). The confidence intervals of prevalence and mean abundance were estimated with the Sterne exact method and the bias-corrected and accelerated Efron-Tibshirani's bootstrap. Differences between the parasite assemblages of the five host groups and between host sexes (including all adult specimens) were evaluated using the Fisher's exact test for prevalence and the bootstrap rank Welch test for mean abundance. Results were compared with previously published quantitative data on the parasites of E. alletteratus from the western Atlantic Ocean (Alves and Luque, Reference Alves and Luque2006). Correlations between abundance of parasite species and host size were evaluated with the Spearman rank correlation coefficient. All these analyses were performed using the free software Quantitative Parasitology web 1·0·9 (Reiczigel et al. Reference Reiczigel, Rózsa and Reiczigel2013).
Bayesian hierarchical spatial models (Muñoz et al. Reference Muñoz, Pennino, Conesa, López-Quílez and Bellido2013) were used to examine whether the abundance of each parasite species was related to independent variables, namely the bottom depth, SST, length and weight of the hosts. Moreover, a random spatial effect was introduced in the analysis in order to verify the spatial variability of the data within each area. Specifically, the number of each parasite in each location sampled, Y i was considered Poisson distributed with rate t i λ i. , where t i is the observation time at site i and λ i is proportional to a relative species abundance at the site i and measures the expectation of samplings for a unit observation time, according to the general formulation,

where α is the intercept, X ij is the vector of the explanatory covariates at the year j and the location i, Z j is the component of the temporal unstructured random effect at the year j, and W i represents the spatially structured random effect at the location i.
Once the model was determined, the next step was to estimate its parameters. Following Bayesian reasoning, the parameters were treated as random variables, and prior knowledge was incorporated via the corresponding prior distributions of the selected parameters. In particular, for the parameters involved in the fixed effects, non-informative Gaussian distributions N(0, 100) were used. A prior Gaussian distribution with a zero mean and covariance matrix was assumed for the spatial effect. This component depends on two hyperparameters k and τ, which determined, respectively, the range and the total variance of the spatial effect (see Muñoz et al. Reference Muñoz, Pennino, Conesa, López-Quílez and Bellido2013 for more detailed information about spatial effects). In addition, a Log Gamma prior distribution was assumed on the log-precision λy (a = 1, b = 5e–05) for the temporal component.
A model selection with both backwards and forwards approaches was used to select relevant variables. Specifically, the Deviance Information Criterion (DIC), a well-known Bayesian model-choice criterion for comparing complex hierarchical models (Spiegelhalter et al. Reference Spiegelhalter, Best, Carlin and Van Der Linde2002), was used. Lower values of DIC represented the best compromise between fit and parsimony.
The Integrated Nested Laplace Approximation (INLA) methodology (Rue et al. Reference Rue, Martino and Chopin2009) and software (http:\\ www.r-inla.org), which is implemented in the R software (R Development Core Team, 2015), were used as an alternative to the Markov chain Monte Carlo methods for speed of calculation (Roos et al. Reference Roos, Carvalho, Lopes and Pennino2015).
RESULTS
A total of 10 parasite species/taxa were found in the head of E. alletteratus from the western Mediterranean Sea (Table 2). The monogenean Hexostoma thunninae was the most prevalent species (P% = 55%) and the didymozoid Melanocystis cf. kawakawa the most abundant one (mA = 14·4).
Table 2. Prevalence (P%), mean abundance (mA), 95% confidence intervals (in brackets), Spearman rank correlation index (Rs) between abundance and fish length and microhabitat, of the parasites of the head of Euthynnus alletteratus from the western Mediterranean Sea.
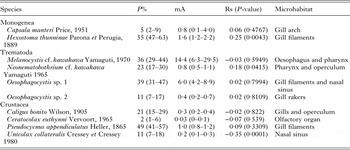
Morphological and molecular analysis of didymozoid parasites
The 3 236 didymozoid specimens found in the head tissues of E. alletteratus were morphologically classified into four species/morphotypes (Fig. 1): Melanocystis cf. kawakawa (66% of all specimens), Neonematobothrium cf. kawakawa (4%), Oesophagocystis sp. 1 (28%), and Oesophagocystis sp. 2 (2%). All these parasites consisted of a capsule including a couple of similar hermaphroditic individuals, encapsulated in host tissues. Neonematobothrium cf. kawakawa had a very long, ribbon-like body, not divided into regions. Oral sucker and pharynx were present, whereas prepharynx and ventral sucker were absent. Testes parallel, reaching genital junction level. Ovary and vitellaria simple, thin and long, located in the anterior and posterior parts of the body, respectively. Uterus three looped. Melanocystis cf. kawakawa, Oesophagocystis sp. 1, and Oesophagocystis sp. 2 had the body divided into two regions. The anterior regions were lancelolate, filiform and racket–like, respectively, and attached to the middle of the ventral surface of the posterior regions. The posterior regions, which included ovarium, vitellaria and testes, were disk-like in M. cf. kawakawa, conical in Oesophagocystis sp. 1, and bell-shaped in Oesophagocystis sp. 2. Oral sucker and pharynx were present, whereas prepharynx and ventral sucker absent. Two blind caeca with black amorphous contents were visible in M. cf. kawakawa.

Fig. 1. Micrographs and phylogenetic tree of didymozoids of the head of Euthynnus alletteratus from the western Mediterranean Sea inferred by using the maximum likelihood method based on the Tamura-Nei model of internal transcribed spacer 2 (ITS-2) sequences. Bootstrap support (>70% for 1000 replicates) of the percentage of trees in which the associated taxa clustered together is shown next to the branches. Bar in microns: A-B, 500; C, 100; D, 1000.
A total of 384 bp of ITS2 and 595 bp of 28S fragments of 18 DNA sequence were generated (GenBank accession number: KU216165-KU216182 and KU290344-KU290361; PCICBiBE voucher numbers: 12126–12143). The comparison of ITS2 fragments showed that there was no genetic variation within M. cf. kawakawa and N. cf. kawakawa groups, whereas 0·001 ± SD 0·001 of difference was found within Oesophagocystis sp. 1 and Oesophagocystis sp. 2 groups. The ML analysis of these fragments revealed four well-supported genetic clusters within the family Didymozoidae (Fig. 1), which were consistent with the morphological types previously identified. ITS2 fragments of M. cf. kawakawa were more similar to those of Oesophagocystis sp. 1 (0·035 ± SD 0·009) than to those of Oesophagocystis sp. 2 (0·047 ± SD 0·011) and those of N. cf. kawakawa (0·255 ± SD 0·030). The ITS2 sequences of N. cf. kawakawa were more similar to those of M. cf. kawakawa than to those of Oesophagocystis sp. 1 (0·270 ± SD 0·011) and those of Oesophagocystis sp. 2 (0·279 ± SD 0·032). Finally, the sequences of Oesophagocystis spp. were more similar between them (0·039 ± SD 0·010) than to those of the other species. The phylogenetic tree from 28S region (not shown) had similar topology to that deduced from ITS2. Bootstrap support values were generally lower than the ITS2 ones, with some basal nodes of the tree not resolved.
Parasite population analysis
The abundance of H. thunninae and N. cf. kawakawa was positively correlated with the host length (Spearman rank correlation index, Rs = 0·25, P = 0·0043 and Rs = 0·18, P = 0·0415, respectively), whereas that of Unicolax collateralis was negatively correlated with host length (Rs = –0·35, P = 0·0001). Bayesian GLM analyses showed a positive relationship between the abundance of Capsala manteri and North eastward latitude and longitude (posterior mean = 0·0021, 95% CI = –0·0439–0·0483; 0·1079, 95% CI = –0·568–0·7758, respectively), whereas a negative one between that of Ceratocolax euthynni and the eastward longitude (posterior mean = –0·3646, 95% CI = –1·4649- 0·8126). Positive relationships were also found between the abundance of most of parasites and SST (Table 3), except that negative of Pseudocycnus appendiculatus. The relationship between the abundance of C. manteri, N. cf. kawakawa and Caligus bonito and the sea bottom depth was also negative. No significant differences in P% and mA of each parasite species were found between host sexes.
Table 3. Bayesian analysis of the relationship between environmental factors and the abundance of the parasites of the head of Euthynnus alletteratus from the western Mediterranean Sea

SST, sea surface temperature.
95% confidence intervals in brackets. Only significant data are shown (P ⩽ 0·05).
Figures 2–3 show the prevalence and mean abundance of parasites according to the locality. Ceratocolax euthynni was found only in the tuna from the southeastern Spain; whereas three species were not collected in those of the Strait of Gibraltar: C. manteri, N. cf. kawakawa and C. bonito. This caligid parasite and U. collateralis had the highest P% and mA in tuna of the Gulf of Valencia; H. thunninae and P. appendiculatus had the highest P% and mA in the samples of the southeastern Spain. Melanocystis cf. kawakawa and Oesophagocystis sp. 1 had the highest P% in the Gulf of Valencia and in the southeastern Spain, and had the highest mA in the southeastern Spain and in the Gulf of Valencia, respectively.

Fig. 2. Prevalence of the parasites of the head of Euthynnus alletteratus according to locality. Black squares, southeastern Spain samples; white squares, Gulf of Valencia; and grey squares, Strait of Gibraltar. Lines indicate 95% confidence intervals. Greek letters indicate significant differences (P ⩽ 0·05).
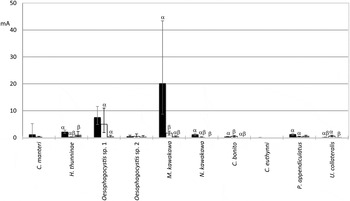
Fig. 3. Mean abundance of the parasites of the head of Euthynnus alletteratus according to locality. Black squares, southeastern Spain samples; white squares, Gulf of Valencia; and grey squares, Strait of Gibraltar. Lines indicate 95% confidence intervals. Greek letters indicate significant differences (P ⩽ 0·05).
Table 4 shows the P% and mA of the parasites found in the southeastern Spain according to the year. The P% of didymozoids and of U. collateralis was higher in 2011 than in 2008 and 2009, being higher in 2009 than in 2008, but Oesophagocystis sp. 2 had similar prevalences among years. The mA of M. cf. kawakawa was higher in 2009 than in 2008 and 2011; the mA of P. appendiculatus were higher in 2008 than 2011, and that of N. cf. kawakawa was higher in 2011 than in 2008.
Table 4. Percent prevalence, mean abundance and their 95% confidence intervals of the parasites of the head of Euthynnus alletteratus from the southeastern Spain according to the sampling year

Greek letters indicate significant differences (P ⩽ 0·05).
DISCUSSION
This study describes for the first time the parasite assemblage of the head of E. alletteratus in the western Mediterranean Sea, adding information on the parasite fauna of this tuna, which has been previously investigated only in the western Atlantic Ocean (Alves and Luque, Reference Alves and Luque2006, Table 5). This is also the first contribution to the study of the molecular taxonomy of the didymozoids of this host. Considering the parasites recorded in formerly published studies, the known metazoan parasite fauna of the head of the Atlantic black skipjack includes a total of 24 species/taxa. Prior to this study, only three parasitic species had been reported in E. alletteratus from the Mediterranean Sea: the hexostomatid monogenean H. thunninae in tuna from the Ligurian Sea and the bomolochid copepods Unicolax collateralis and Unicolax mycterobius in hosts caught off Lebanon (Palombi, Reference Palombi1949; Lin and Ho, Reference Lin and Ho2006). The finding of the first two species in E. alletteratus from the westernmost areas of the Mediterranean Sea enlarges their range of distribution, confirming their presence in the whole Mediterranean Sea. Unicolax mycterobius was not observed in this study, but it has been found in Auxis rochei (Scombridae) from the southeastern of Spain and the Strait of Gibraltar during a contemporary parasitological survey (Mele et al. Reference Mele, Saber, Gómez-Vives, Garippa, Alemany, Macías and Merella2015). In addition, the capsalid monogenean C. manteri and the bomolochid copepod C. euthynni, which have been observed in E. alletteratus from the Atlantic Ocean (Cressey and Cressey, Reference Cressey and Cressey1980; Chisholm and Whittington, Reference Chisholm and Whittington2007), were found for the first time in the western Mediterranean Sea, suggesting that these parasites can infect the Atlantic black skipjack throughout its range of distribution. Each single species of the families Bomolochidae and Hexostomidae infects a different tuna species, showing a high degree of host specificity that is an uncommon character for the ectoparasites of the western Mediterranean tunas (Mele et al. Reference Mele, Merella, Macías, Gómez, Garippa and Alemany2010, Reference Mele, Macías, Gómez, Garippa, Alemany and Merella2012; Culurgioni et al. Reference Culurgioni, Mele, Merella, Addis, Figus, Cau, Karakulak and Garippa2014). For instance, the other ectoparasites found, Caligus bonito and P. appendiculatus, have been reported in several tuna species from the Mediterranean Sea, as well as from the Atlantic Ocean (Culurgioni et al. Reference Culurgioni, Mele, Merella, Addis, Figus, Cau, Karakulak and Garippa2014; Mele et al. Reference Mele, Saber, Gómez-Vives, Garippa, Alemany, Macías and Merella2015). However, the host specificity is not always straightforward, for example three ectoparasites from the western Mediterranean Sea, Allopseudaxine macrova of Auxis rochei and Capsala magronum and Caligus coryphaenae of Thunnus thynnus, were absent in E. alletteratus from the western Mediterranean Sea, whereas they have been found in some tuna species from the Atlantic Ocean, including E. alletteratus (Alves and Luque, Reference Alves and Luque2006; Culurgioni et al. Reference Culurgioni, Mele, Merella, Addis, Figus, Cau, Karakulak and Garippa2014; Mele et al. Reference Mele, Saber, Gómez-Vives, Garippa, Alemany, Macías and Merella2015). This fact suggests that the habitat overlapping of E. alletteratus and T. thynnus in the western Mediterranean Sea does not allow the sharing of these parasites. Probably for the same reason, several parasites observed in tunas from the western Atlantic Ocean, including E. alletteratus, were not found in the Mediterranean Sea: i.e. Capsala maccallumi, Hexostoma lintoni, Udonella caligorum, Sibitrema poonoui and Unicolax anonymus (Alves and Luque, Reference Alves and Luque2006; Justo and Kohn, Reference Justo and Kohn2015; Mele et al. Reference Mele, Saber, Gómez-Vives, Garippa, Alemany, Macías and Merella2015).
Table 5. Summary of the parasite fauna of the head of Euthynnus alletteratus

Capital letter represent sources: A, Palombi (Reference Palombi1949); B, Bussiéras (Reference Bussiéras1972); C, Cressey and Cressey (Reference Cressey and Cressey1980); D, Hendrix (Reference Hendrix1994); E, Alves and Luque (Reference Alves and Luque2006); F, Lin and Ho (Reference Lin and Ho2006); G, Chisholm and Wittington (Reference Chisholm and Whittington2007); X, present results. Asterisk indicates significant differences between the present results and Alves and Luque (Reference Alves and Luque2006) (P ⩽ 0·05).
Morphological and genetic analyses of the didymozoid trematodes revealed the presence of four species that had not been previously described in E. alletteratus. Among them, Melanocystis cf. kawakawa and Neonematobothrium cf. kawakawa have been also found in Euthynnus affinis and Euthynnus lineatus (Yamaguti, Reference Yamaguti1970; Madhavi and Ram, Reference Madhavi and Ram2000), whereas the other two, Oesophagocystis sp. 1 and Oesophagocystis sp. 2, can be considered as putative new species. Conversely, Lobatozoum multisacculatum, reported in E. alletteratus from the Brazilian waters of the Atlantic Ocean (Alves and Luque, Reference Alves and Luque2006) was not found in the present study. However, in a contemporary parasitological survey Mele et al. (Reference Mele, Macías, Gómez, Garippa, Alemany and Merella2012) reported this species in one specimen of Katsuwonus pelamis, a migratory species of tuna between the Atlantic Ocean and the western Mediterranean Sea. Didymozoids are food-borne parasites with a partial known life cycle that presumably involves several possible intermediate hosts, including molluscs, crustaceans and fish, and one or more definitive hosts, mainly scombrid fish (Nikolaeva, Reference Nikolaeva and Hargis1985; Pozdnyakov and Gibson, Reference Pozdnyakov, Gibson, Bray, Gibson and Jones2008). The fact that no one of the didymozoids found in E. alletteratus have been reported in other scombrids of the western Mediterranean Sea shows that this species plays a specific key role as host reservoir for the maintenance of these infections in this area. On the other side, the absence of L. multisacculatum in E. alletteratus of western Mediterranean Sea can be attributed to the lack of suitable environmental conditions and/or the absence of the intermediate hosts in this area.
The limited number of parasite species shared between the parasite faunas of the head of E. alletteratus from the western Mediterranean Sea and the western Atlantic Ocean indicates that the distance between these areas could represent a barrier to the dispersion of both the host and its species-specific parasites. This is in accordance with other studies on scombrids, e.g. Scomber colias that showed differences in the helminth fauna between the opposite sides of the Atlantic Ocean (Mele et al. Reference Mele, Pennino, Piras, Garippa and Merella2014). Moreover, the difference between the Atlantic and Mediterranean domains can influence the dispersion and recruitment of the generalist parasites infecting large migrant fish, e.g. the common dolphinfish Coryphaena hippurus (Carbonell et al. Reference Carbonell, Massutí, Castro and Garcıá1999). Therefore, the presence of several parasites exclusively either in the western Atlantic Ocean (i.e. Hexostoma keokeo, H. lintoni, Hexostoma euthynni, S. poonoui) or in the western Mediterranean Sea (all the didymozoids and Hexostoma thunninae), as well as the differences in the structure of the parasite assemblages between these areas, could be useful to distinguish the fish populations from the opposite coasts of the Atlantic Ocean.
Concerning the differences between the parasite assemblages of the fish from the Gulf of Valencia and southeastern Spain, it must be considered that the samples from Valencia had the lowest fork length, and this fact could influence the occurrence of some parasites (Madhavi and Ram, Reference Madhavi and Ram2000). Particularly, the abundance of the gill monogenean H. thunninae appears to be positively correlated with the fish length, because of the enlargement of the suitable habitat (gill area). Conversely, the abundance of U. collateralis, a copepod that lives embedded in the mucous of the nasal sinus, was negatively correlated with the fish length, suggesting that the parasite find an ideal microhabitat in the nasal cavities of the small hosts, as indicated for other nasal copepods (Cressey et al. Reference Cressey, Collette and Russo1983; Sharples and Evans, Reference Sharples and Evans1995). Moreover, the infection of didymozoids is related to the diet of E. alletteratus, that changes as fish grow, becoming the large specimens more piscivorous (Manooch et al. Reference Manooch, Mason and Nelson1985; Falautano et al. Reference Falautano, Castriota, Finoia and Andaloro2007).
Differences of didymozoid infections between the fish from the southeastern Spain and the Strait of Gibraltar can be due to differences in environmental conditions, which influence the presence of infected preys in the areas. Although no specific information is available on the metacercariae of the didymozoids of E. alletteratus, it is important to note that several prey items of E. alletteratus (Falautano et al. Reference Falautano, Castriota, Finoia and Andaloro2007) have been reported infected by didymozoid metacarcariae also in the areas herein studied (Culurgioni et al. Reference Culurgioni, Mele, Merella, Addis, Figus, Cau, Karakulak and Garippa2014, PhD thesis at http://veprints.unica.it/1010/ and author personal comments; Mateu et al. Reference Mateu, Nardi, Fraija-Fernández, Mattiucci, de Sola, Raga, Fernández and Aznar2015). Because most of these intermediate hosts are pelagic species, it is more probable that E. alletteratus acquires the didymozoids, as well as other pelagic parasites as P. appendiculatus (Mladineo et al. Reference Mladineo, Segvić and Petrić2011; Culurgioni et al. Reference Culurgioni, Mele, Merella, Addis, Figus, Cau, Karakulak and Garippa2014), in the offshore pelagic areas close to the sampling points than in the adjacent inshore coastal regions.
The abundance of several parasites, especially of M. cf. kawakawa, is likely associated to the heating of the western Mediterranean waters in the warm season (Álvarez et al. Reference Álvarez, Rodríguez, Catalán, Hidalgo, Álvarez-Berastegui, Balbín, Aparicio-González and Alemany2015). Analogously, Lester et al. (Reference Lester, Barnes and Habib1985) and Jones (Reference Jones1991), studying the parasites of tuna that seasonally migrate from the warm tropical Pacific Ocean to the temperate southern Pacific areas of New Zealand, observed higher abundance of didymozoids in tropical areas than in the temperate ones, suggesting that these trematodes infect tuna only in the warm waters. Conversely, P. appendiculatus is found in the western Mediterranean Sea during the winter and early spring seasons, and its abundance seems to be favoured by the low SST (Hayward et al. Reference Hayward, Aiken and Nowak2008). In fact, this parasite showed significant differences of abundance between sampling years that can be related with the regional oceanography conditions. According to Álvarez-Berastegui et al. (Reference Álvarez-Berastegui, Ciannelli, Aparicio-Gonzalez, Reglero, Hidalgo, López-Jurado, Tintoré and Alemany2014) the westernmost areas of the Mediterranean Sea are characterized by highly dynamic oceanographic process that promote a seascape shaped by filaments, fronts and eddies, whose location varies between years and this variability can influence the presence of direct life cycle parasites.
In conclusion, molecular and morphological results evidence the findings of two putative new species of Oesophagocystis in E. alletteratus, which motivate further efforts aimed at their morphological description and systematics analysis. Interannual changes of the environmental factors, such as temperature, are the main factors influencing the abundance of most of the parasite species of E. alletteratus in the western Mediterranean Sea, but also the changing of these variables between sampling areas and the host length modify the presence of monogeneans and bomolochid copepods. The parasite fauna of E. alletteratus differs between the western Mediterranean Sea and the western Atlantic Ocean, suggesting that the parasite assemblages are isolated because this host does not seem able to cross the Atlantic Ocean. Although E. alletteratus is considered a near-shore species, the finding of pelagic parasites, like didymozoids and P. appendiculatus, suggests that this tuna can make local migrations between inshore and offshore pelagic areas in the western Mediterranean Sea.
ACKNOWLEDGEMENTS
We wish to express our gratitude to the technicians of the laboratory of Mazarrón of the IEO Centro Oceanográfico de Murcia, Spain. We are very grateful to the two anonymous reviewers for their critical suggestions that helped to improve the manuscript.
FINANCIAL SUPPORT
This work was supported by the Spanish research project (GPM-4 to D.M. and M. J. G-V., GPM1213 to D. M. and M. J. G-V., and PARATUN AGL2010-20892 to D. M., F. E. M. R., P. M. and G. G.) and the Regione Autonoma della Sardegna grant (to S. M., Master and Back 2012–2013 PRR-MAB-A2013-17605).