Introduction
Parasites reduce the fitness of their hosts through diverse mechanisms including leaching nutrients, utilizing host energy, causing tissue damage and physically occupying space in/on the host (Poulin and Morand, Reference Poulin and Morand2000). Consequently, hosts have evolved numerous defensive strategies to avoid or mitigate the deleterious and cumulative effects of parasitism (Schulenburg et al., Reference Schulenburg, Kurtz, Moret and Siva-Jothy2009). Broadly speaking, host defences can be morphological, physiological, and/or behavioural in nature. In addition to direct anti-parasite behaviours such as grooming (Zhukovskaya et al., Reference Zhukovskaya, Yanagawa and Forschler2013), avoidance of sites with high parasite densities can reduce the risk of exposure in both invertebrate (Lefèvre et al., Reference Lefèvre, de Roode, Kacsoh and Schlenke2012; de Roode and Lefèvre, Reference de Roode and Lefèvre2012) and vertebrate hosts (Smith et al., Reference Smith, White and Hutchings2006; Coulson et al., Reference Coulson, Cripps, Garnick, Bristow and Beveridge2018). Moreover, the ecological and evolutionary consequences of parasitism as well as investment in parasite resistance are likely environmentally mediated (Raffel et al., Reference Raffel, Rohr, Kiesecker and Hudson2006; Luong and Polak, Reference Luong and Polak2007). For example, nutritional food sources (namely, ad libitum access to dietary yeast) can significantly curtail the deleterious effects of parasitism by Macrocheles subbadius on host Drosophila nigrospiracula (Polak, Reference Polak1996).
Trade-off theory can potentially explain environmentally-mediated investment in anti-parasite traits (Stearns, Reference Stearns1989). Trade-offs occur when organisms with limited resources (e.g. energy, nutrients, time) invest in one trait at a cost to another trait (Stearns, Reference Stearns1989; Weiner, Reference Weiner1992). Negative correlations between immune function and reproduction have been observed in vertebrate and invertebrate species (French et al., Reference French, DeNardo and Moore2007; Schwenke et al., Reference Schwenke, Lazzaro and Wolfner2016). French et al. (Reference French, DeNardo and Moore2007) determined that the trade-off between reproduction and immune function in ornate tree lizards, Urosaurus ornatus, is due to the reallocation of limited resources from recovery to reproduction. There can likewise be trade-offs between different behavioural traits. For example, sheep may forgo foraging on nutritionally superior grasses which are contaminated with infective stages of gastrointestinal parasites and instead forage at nutritionally inferior sites (Smith et al., Reference Smith, White and Hutchings2006). Furthermore, environmental heterogeneity in nature can generate variation in habitat quality and, resultantly, habitat preference (Jaenike and Holt, Reference Jaenike and Holt1991; Davis and Stamps, Reference Davis and Stamps2004). In predator-prey ecology, trade-offs resulting from avoiding predation risk are generally considered a non-consumptive effect of predators on the prey population; the cumulative effects of which are often called the ‘ecology of fear’ (Peacor and Werner, Reference Peacor and Werner2008). Likewise, the negative trade-offs suffered by hosts avoiding parasites may represent similar non-consumptive effects, and the ecology of fear framework may explain host–parasite systems as well (Raffel et al., Reference Raffel, Martin and Rohr2008).
Reproductive behaviour affects insect population structure and ecology (Atkinson et al., Reference Atkinson, McVean and Stone2002; Arvanitis et al., Reference Arvanitis, Wiklund and Ehrlen2007), and Drosophila ovipositing behaviour is affected by biotic and abiotic factors at available sites (Jaenike, Reference Jaenike1982; Quan and Eisen, Reference Quan and Eisen2018). Chemicals that may be toxic to flies, such as peppermint oil, can repel females from ovipositing at a site (Jaenike, Reference Jaenike1982); whereas the presence of beneficial microbes can encourage ovipositing (Quan and Eisen, Reference Quan and Eisen2018). Likewise, parasite avoidance may influence host decision-making regarding habitat choice (Lefèvre et al., Reference Lefèvre, de Roode, Kacsoh and Schlenke2012). Previous research has shown that when given a choice between sites with or without parasitoid wasps (restrained behind mesh), Drosophila spp. select oviposition sites free of wasps (Lefèvre et al., Reference Lefèvre, de Roode, Kacsoh and Schlenke2012). Furthermore, investment in anti-parasite defences, including avoidance, may interact with other factors in the environment that indicate habitat quality. The outcome may be the result of a trade-off between infection risk and access to an otherwise superior habitat, i.e. the ecology of fear (Smith et al., Reference Smith, White and Hutchings2006). We hypothesize that the optimal levels of avoidance may vary locally depending on the level of infection risk and resource availability/quality (Bonneaud et al., Reference Bonneaud, Mazuc, Gonzalez, Haussy, Chastel, Faivre and Sorci2003; Lindstrom et al., Reference Lindstrom, Foufopoulos, Parn and Wikelski2004). Thus, it is reasonable to predict that the expression of parasite avoidance by hosts will depend on the quality of available habitats.
Drosophila nigrospiracula is a cactiphilic fly that feeds and oviposits in the necrotic tissue of the cactus Carnegiea gigantea (Markow, Reference Markow1988). This fly is capable of dispersing long distances, upwards of 900 m, between cacti in response to changing environmental conditions (Johnston and Heed, Reference Johnston and Heed1976). Macrocheles subbadius is a naturally occurring ectoparasite of D. nigrospiracula that feeds on the haemolymph of the adult fly and uses hosts for dispersal between temporally variable necrotic cactus (Polak, Reference Polak1996). The mite is a facultative parasite and can complete its life cycle as a free-living predator of nematodes (Perez-Leanos et al., Reference Perez-Leanos, Loustalot-Laclette, Nazario-Yepiz and Markow2017). Mites pose an infection risk to adult flies during mating, feeding, and ovipositing at cacti as well as to newly eclosed offspring. Infection with mites can reduce fly longevity by up to 50–67% (Polak, Reference Polak1996). Flies resist mite infection primarily through behavioural defences such as grooming and rapid bursts of movement, both of which are energetically demanding activities; for example grooming flies consume ~30% more energy than resting flies (Luong and Polak, Reference Luong and Polak2007; Horn and Luong, Reference Horn and Luong2019). Avoidance of infested habitats may play a role in minimizing both the costs of mounting a behavioural defence, and the risk of infection (Buck et al., Reference Buck, Weinstein and Young2018).
Here we investigate the role of parasite avoidance relative to resource quality in driving host reproductive behaviour and habitat choice. We measured the preference of female D. nigrospiracula for oviposition sites with varying degrees of parasite risk and environmental quality. We then asked if introducing ectoparasitic mites to an otherwise preferred oviposition site sufficiently alters the risk-reward ratio to induce a behavioural trade-off and eliminate preferences for particular sites. Because proximity to parasites negatively impacts fly survival and fecundity (Horn and Luong, Reference Horn and Luong2018), we hypothesize that the substantial costs of parasite exposure outweigh any potential benefits gained from utilizing a preferred habitat.
Methods
Fly and mite cultures
Drosophila nigrospiracula Patterson and Wheeler cultures were initiated from adult flies (~150 of each sex) collected from necrotic C. gigantea located in the Sonoran Desert (Arizona, USA). The culture medium consisted of 3:1 instant mashed potato flakes to Drosophila medium (Formula 4–24 Instant Drosophila Medium; Carolina Biological Supply Company, Burlington, NC). Larval D. nigrospiracula fails to pupate in the absence of cactus, thus autoclaved necrotic cactus (4–6 cm3) was added to the medium along with nutritional yeast. Newly emerged adult flies were separated by sex and maintained on agar medium until the experiments. Flies were stored in an incubator (Percival Scientific, Perry, IA, USA) at 24 °C and 50% relative humidity with a 12 L:12 D cycle.
Macrocheles subbadius Berlese cultures were initiated with ~200–300 female mites found on wild-caught D. nigrospiracula. Bacteriophagic nematodes were provided as a food source and co-cultured in a 2:1 mix of wheat bran to wood chips. Mite cultures were maintained at 26 °C and 70% relative humidity with a 12 L:12 D cycle (Percival Scientific, Perry, IA, USA). Mites were collected from culture using a Berlese funnel and temporarily stored on a moistened paper towel or moistened plaster-of-Paris until use.
Mite avoidance
This experiment was designed to test if female D. nigrospiracula preferentially oviposit on sites based on the presence or absence of parasites (illustrated in Fig. 1). For a given trial, five female flies and three male flies were introduced into an observation arena (7.5 cm radius × 1.5 cm high petri dish) containing two small dishes (3 cm radius × 0.5 cm height). Each dish contained a comparable thin layer (to facilitate egg counting) of oviposition media (culture medium with yeast and cactus supplement); the only difference was that one dish contained mites and the other was mite-free. Mites were not in direct contact with the flies; five adult female mites were haphazardly selected from the mass culture and sequestered inside a chamber consisting of a cropped pipette tip with mesh screens on the ends to allow cues to pass through the chamber. The mite-free dish had an identical chamber present except it did not contain mites. A small, distilled-water-moistened piece of sterile sponge (~1 cm2) was placed onto each oviposition dish to minimize desiccation. A damp paper towel was placed in the centre of the arena to provide additional humidity. The arenas were then parafilmed and placed inside a rectangular plastic bin (30 cm × 15 cm base, 12 cm height) containing a beaker of distilled water to ensure high humidity. This process prevented the media from desiccating over the experimental period. To control for idiosyncratic preferences of flies, we orientated the arenas such that each condition was on the left and right side ~50% of the time.
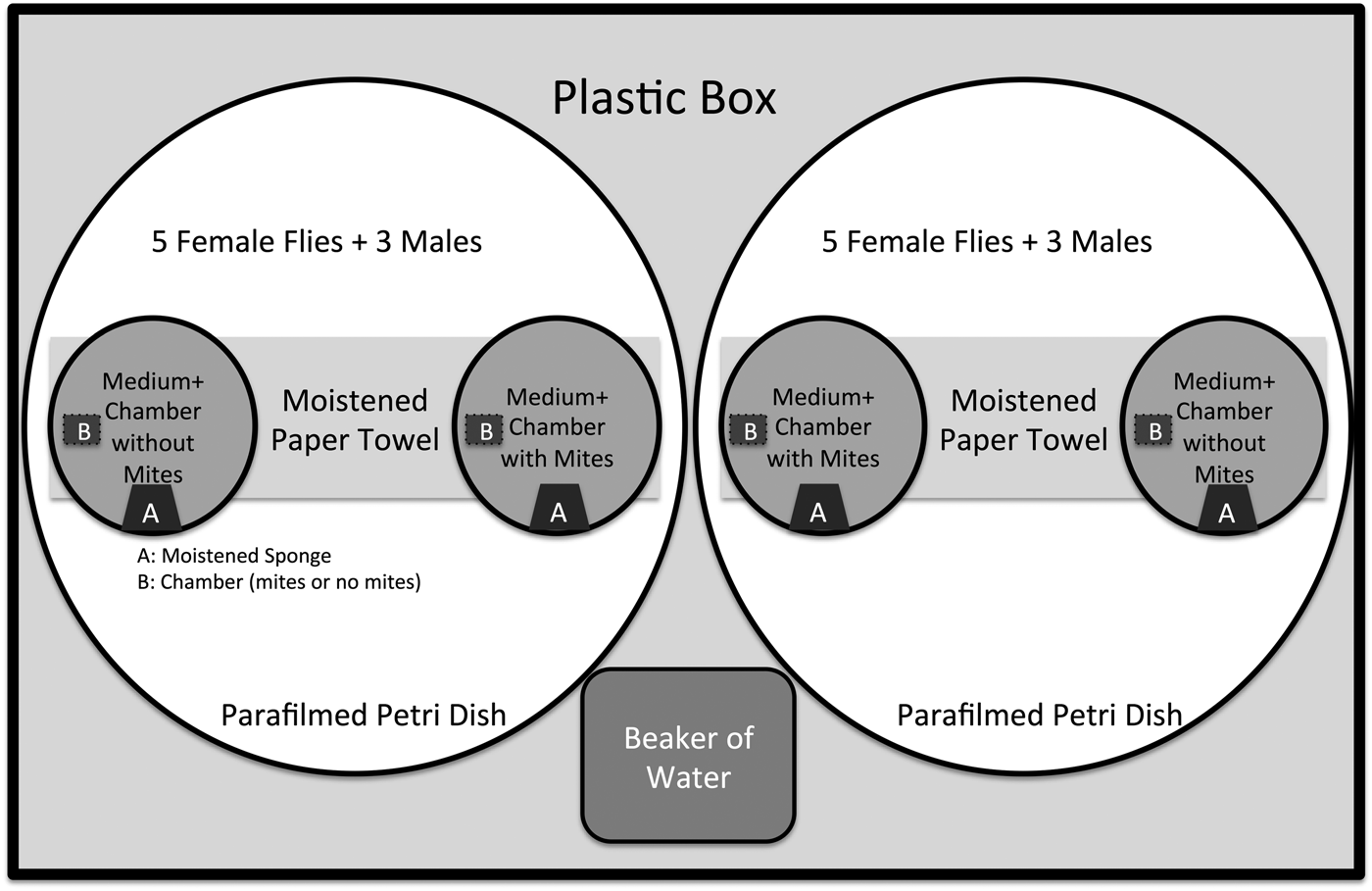
Fig. 1 Illustration of experimental setup in the mite-avoidance experiment. Two sites (watch glasses) are in each individual arena (petri dish closed with parafilm). The sites contain media (instant potatoes, Drosophila media, nutritional yeast, autoclaved cactus), distilled-water-moistened sponge (A), and a central chamber that either contained five adult female mites restrained with mesh or no mites (B). Five female flies and three male flies were then introduced into each arena. Multiple arenas were placed into a plastic box along with a beaker of water (humidifier) and stored for 48 h in an incubator (24 °C and 50% relative humidity with a 12 L:12 D cycle), after which the number of eggs at each site was counted under a dissecting microscope.
Flies were permitted 48 h to oviposit in the dishes, after which time adult flies were removed and the number of eggs deposited in the media were counted. The degree of bias was quantified as the proportion of the total number of eggs deposited on the dish with mites. Thus, for each arena we determined if flies preferentially oviposited on the mite side (bias >0.5), the mite-free side (bias <0.5), or showed no preference (bias = 0.5). Extra replicates, 18 arenas each containing two dishes, were conducted in order to compare the hatch rate (no. of larvae/no. of eggs) of eggs on the mite-infested and the mite-free sites. Dishes in which 10 or more eggs were deposited (in order to get a reasonable measure of hatch rate for the dish) were retained for 48 additional hours, and the number of larva per dish was then recorded under a dissecting microscope.
Mite–environment interaction
In two preliminary trials, we validated the importance of two environmental indicators that flies may use to select ovipositing sites. We anticipated that flies would show a strong attraction to sites supplemented with cactus and a moderate attraction to sites seeded with eggs from conspecifics. Previous research has shown that chemical cues deposited along with eggs can encourage ovipositing by other females (Duménil et al., Reference Duménil, Woud, Pinto, Alkema, Jansen, Van der Geest, Roessingh and Billeter2016). Arenas were prepared as in the mite avoidance experiment, but without mite chambers, to assess preferences for oviposition media. In the cactus experiment, we tested if flies prefer medium containing cactus (~0.5 g) over medium without cactus but were otherwise comparable (Drosophila media + instant potatoes + yeast). In the egg-seeding experiment media was prepared with formula, instant potato, yeast, and cactus, but one dish was augmented with 10 intact D. nigrospiracula eggs; eggs were transferred directly from an oviposition dish of a ‘donor’ female using a Pasteur pipette. These additional eggs were subtracted from the total before analysis. In both experiments, female flies were allowed 48 h to oviposit on the media before the eggs were counted. A bias >0.5 represented a preference for the treatment factor (cactus or augmented eggs), while a bias <0.5 represented an aversion to that factor.
In the next experiments, we tested if mite avoidance would overwhelm the preference for sites with attractants (cactus or presence of conspecific eggs). Oviposition arenas were prepared as described in the mite avoidance experiment. In the mite–cactus experiment we prepared one dish with cactus and a chamber containing five mites, and the other dish contained regular media (no cactus) and an empty mite chamber. In the mite-seeding experiment one dish was augmented with eggs (as described above) and a chamber containing mites, whereas the other dish was not artificially seeded with eggs and had only an empty chamber. After a 48-h ovipositing period, the bias in each arena was quantified such that bias >0.5 represented a greater proportion of eggs deposited on the side with mites and either cactus or seeded eggs.
Nutritional cactus and fly fitness
This additional experiment confirmed ovipositing on cactus increased the fitness of flies; two male and female flies were placed into vials with ~2.5 of culture media with a cactus supplement or in vials with identical media sans cactus. After 4 days the adult flies were removed, and the vials were followed for 2 weeks after which the number of pupae and adult offspring were counted.
Statistics
Statistics were performed in R studio (R Studio Team, 2015)
A one-group Wilcoxon test was used to test if a group showed significant bias in egg laying towards one environment (wilcox.test, two-sided, H 0: bias = μ = 0.5; R Stats). Hatch rates were also compared using a Wilcoxon test. A Kruskal–Wallis test was used to test if the mean number of eggs deposited differed between experiments.
Results
Mite avoidance
This experiment tested if flies selectively oviposit at parasite-free habitats when provided with oviposition sites that were otherwise comparable. The mean bias against mite-infested sites was 0.24 ± 0.05 (mean ± 1 standard error) (N = 46) (Fig. 2). The average number of eggs on the dish with sequestered mites was 49.9 ± 10.6 and 114.3 ± 11.3 on the mite-free site. This bias is significantly different from random ovipositing (wilcox.test, μ = 0.5, P < 0.001). Flies showed a strong aversion to ovipositing on sites where M. subbadius mites were present. In 18 trials, the dishes were retained and the hatch rate of eggs at the site containing mites and the site without mites was compared, 12% (N = 11) and 18% (N = 13) respectively (not significantly different, wilcox.test, P = 0.79). However, a low number of hatch rates was recorded due to (1) only including dishes with ⩾10 eggs and (2) heavy bacterial/mould growth after 4 days.
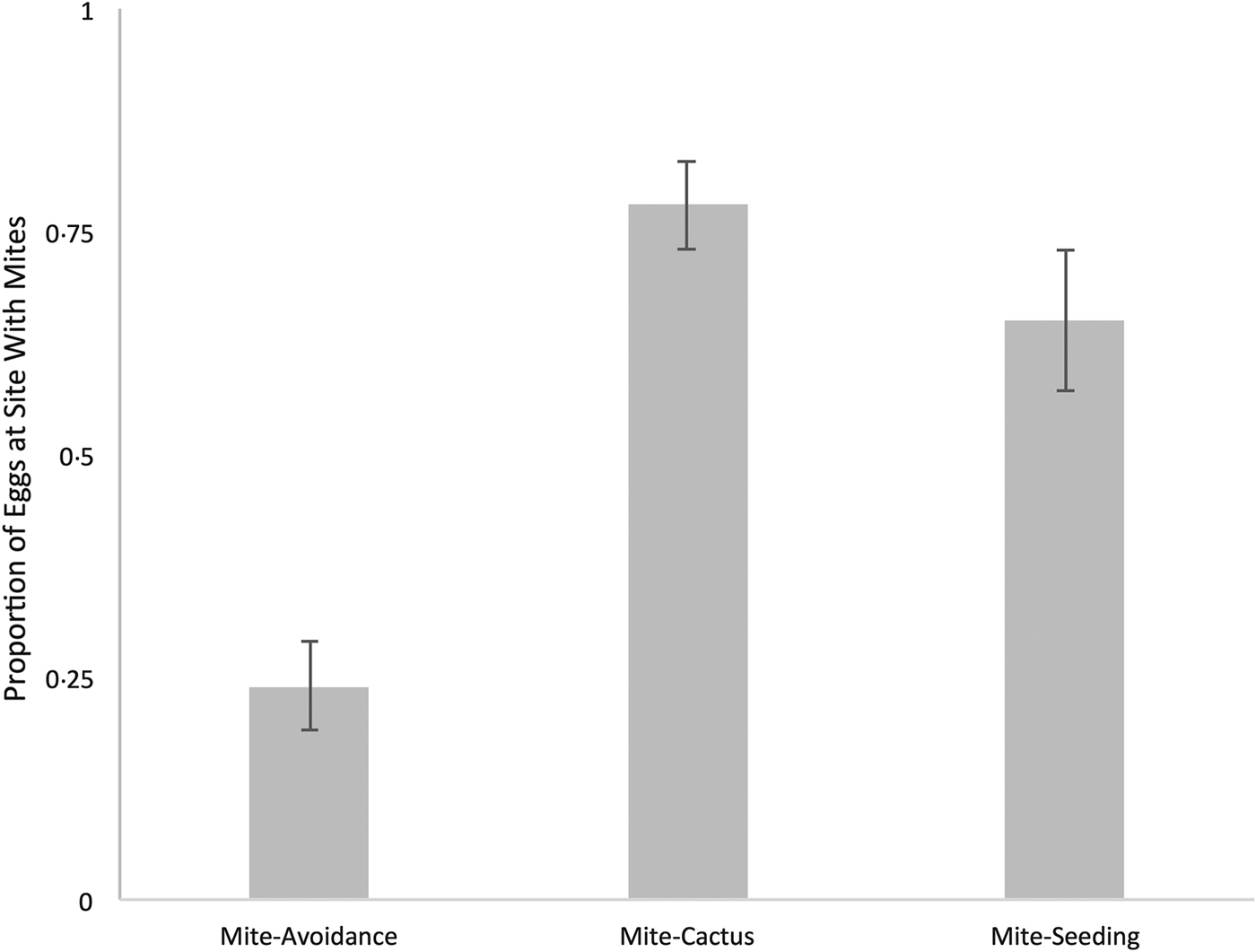
Fig. 2 Mean proportion of eggs laid at mite-infested sites in mite-avoidance and mite–environment interaction experiments. In the mite-avoidance experiment (N = 46), sites were otherwise identical except for the presence of mites restrained behind a mesh. In the mite–cactus experiment (N = 16), the site with mites also contained cactus, while the mite-free side lacked cactus. In the mite-seeding experiment (N = 18), the mite-infested side also contained 10 Drosophila nigrospriacula eggs from stock flies. Error bars represent standard error.
Mite–environment interaction
Cactus experiment
This experiment confirmed that flies preferentially oviposit on sites containing necrotic cactus as opposed to plain media. On average flies laid 131.8 ± 26.3 eggs on the site with cactus and 25.6 ± 10.5 eggs on the site with no cactus. This represents a significant 0.84 ± 0.05 (N = 17) bias towards the site with cactus (one sample wilcox.test, P < 0.001) (Fig. 3). As predicted, flies exhibited a strong attraction towards sites with media containing necrotic saguaro cactus, their natural host plant and oviposition substrate.
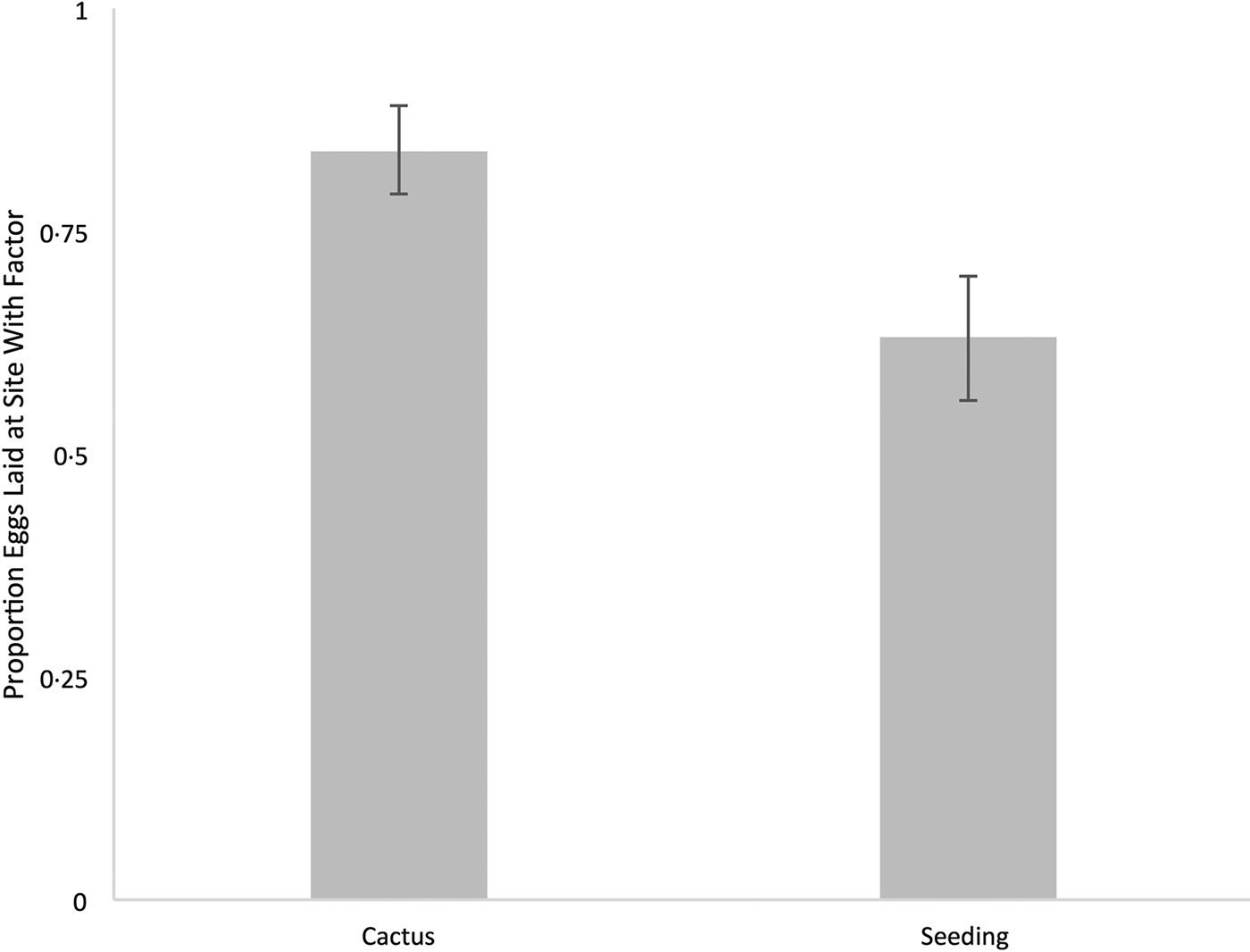
Fig. 3 Mean proportion of eggs in environmental preference experiments. In the cactus experiment (N = 17) flies were given a choice between a site containing cactus and a site containing only potato-fly instant medium. In the seeding experiment (N = 23) flies were given a choice between a site seeded with conspecific eggs and a site without seeding. Error bars represent standard error.
Seeding experiment
This experiment confirmed that flies preferentially oviposit at sites seeded with conspecific fly eggs. Flies exhibited a significant bias (0.63 ± 0.07, N = 23, one sample wilcox.test, P = 0.012) towards sites augmented with eggs. On average 102.7 ± 12.7 eggs were deposited on augmented sites and 87.8 ± 19.5 eggs were laid on untampered media (Fig. 3).
Mite–cactus experiment
Flies preferentially laid more eggs (119.8 ± 19.2) on sites with cactus and mites than on sites with neither mites or cactus (27.3 ± 7.3). We observed a 0.78 ± 0.05 (N = 16) bias towards the side with mites and cactus, and this bias was statistically significant (one sample wilcox.test, P = 0.0017). The bias observed in the mite–cactus experiment was similar to the bias observed in the cactus experiment (0.84 ± 0.05). Flies exhibited a strong preference for oviposition sites with cactus, even when that site presented a risk of infection by mites.
Mite-seeding experiment
Female flies were given a choice between a site seeded with conspecific eggs and mites vs a site without eggs or mites. An average of 121.8 ± 27.5 eggs were laid on sites seeded with eggs and mites, whereas 68.1 ± 18.5 eggs were deposited on the mite-free sites (sans egg augmentation) (Fig. 2). The mean bias was 0.65 ± 0.08 (N = 18) towards the seeded sites infested with mites (one sample wilcox.test, P = 0.055). The bias observed in the mite-seeding experiment was nearly indistinguishable from the bias observed in the seeding experiment (0.63 ± 0.07). These results show that flies preferentially oviposited at sites seeded with eggs, even if doing so required exposure to mites. Amongst the five preference experiments, there were no significant differences in the total number of eggs laid per arena (Kruskal–Wallis, H = 2.38, P = 0.67).
Nutritional cactus and fly fitness
Pairs of female flies, with two males, were given 4 days to mate and oviposit in vials with media containing cactus (N = 15) or without cactus (N = 15). Vials were maintained for 2 weeks, and the number of offspring was counted (pupae + adults). Vials with cactus supplement produced on average 6.3 ± 2.5 offspring, and the vials without cactus produced 0.0 ± 0.0 offspring. In the absence of cactus fly larvae overwhelmingly failed to pupate.
Discussion
We hypothesized that the fitness costs of parasite exposure would outweigh the benefits of ovipositing at environmentally preferred sites, and fly decision-making would, therefore, favour ovipositing at environmentally inferior sites in order to avoid parasite exposure. Hence we predicted that flies would avoid parasite exposure at all costs, even if it required ovipositing in a less than optimal media. Initial results showed that female D. nigrospiracula preferentially oviposited at sites free of mites when the substrates were otherwise comparable. This result agrees with previous studies on the interactions between Drosophila and restrained parasitoid wasps (Leptopilina boulardi), in which D. melanogaster and D. simulans laid ~60% of the eggs on parasitoid-free sites (Lefèvre et al., Reference Lefèvre, de Roode, Kacsoh and Schlenke2012). We observed a relatively stronger effect (86% of eggs laid at mite-free sites). If flies are more likely to encounter mites than parasitoid wasps, i.e. stronger parasite-mediated selection, flies may show a stronger adaptation to avoid mites (Koskella, Reference Koskella2018). Alternatively, this difference may be explained by the larger arenas used in the study by Lefèvre et al. (Reference Lefèvre, de Roode, Kacsoh and Schlenke2012), which may dilute the visual and/or olfactory cues that Drosophila use to detect wasps.
The sensory mechanism(s) by which D. nigropsiracula detect mites is not currently known. Flies are able to detect and respond to mites across semi-translucent mesh, suggesting the mechanism is olfactory or visual in nature (Luong et al., Reference Luong, Horn and Brophy2017). Given the overall robustness of the fly olfactory system to detect positive and negative cues and its ability to trigger fly behavioural responses (Jaenike, Reference Jaenike1982; Gaudry et al., Reference Gaudry, Nagel and Wilson2012; Quan and Eisen, Reference Quan and Eisen2018), we suggest that flies likely detect mites through chemosensory mechanisms. However, D. melanogaster show increased movement when exposed to predators such as spiders and mantises, and they appear to detect the predators through visual cues (de la Flor et al., Reference de la Flor, Chen, Manson-Bishop, Chu, Zamora, Robbins, Gunaratne and Roman2017). Further research on Drosophila detection of ectoparasites is warranted.
Grostal and Dicke (Reference Grostal and Dicke1999) found that herbivorous mites (Tetranychus urticae) avoid living on and ovipositing on plant material that had come into contact with a natural predator, even when those predators were no longer present. Likewise, the parasitoid wasp Leptopilina heterotoma can olfactorily detect a closely related competitor and avoids rots where competition is present (Janssen et al., Reference Janssen, Vanalphen, Sabelis and Bakker1995). Furthermore, investment in parasite/pathogen avoidance can be altered by the hosts’ infection status. For example, flies infected with Drosophila C Virus show decreased avoidance of infected conspecific carcasses relative to uninfected controls when given a choice between high-quality sites with the remains of infected conspecific and uninfected sites with lower quality food (Siva-Jothy et al., Reference Siva-Jothy, Monteith and Vale2018). As exposure to multiple natural enemies can have complex synergistic or antagonistic effects on the victim (Dainese et al., Reference Dainese, Schneider, Krauss and Steffan-Dewenter2017), further studies should consider the relative effects of predators, parasites/pathogens, and competitors on the behaviour of Drosophila (Koprivnikar and Urichuk 2017).
When another environmental factor was introduced to the decision-making process, mite avoidance could have been inhibited by environmental cues or masked by strong preferences for particular conditions. However, in the mite-avoidance experiment cactus was present at both sites and flies avoided mite infested sites indicating that environmental conditions do not inhibit mite avoidance and instead overpower it. Our results also suggest that the benefits of ovipositing in these latter environments outweigh the risk of parasite exposure. Others studies have also shown that naive female fruit flies are attracted to food sources on which other flies previously laid eggs (Sarin and Dukas, Reference Sarin and Dukas2009). Female D. melanogaster are attracted to sites where other flies have oviposited through a combination of chemical cues derived from mated female flies and residual male sperm (Duménil et al., Reference Duménil, Woud, Pinto, Alkema, Jansen, Van der Geest, Roessingh and Billeter2016). In our study, these cues may have been present on, and transferred with, the eggs and used to seed the treatment sites (Duménil et al., Reference Duménil, Woud, Pinto, Alkema, Jansen, Van der Geest, Roessingh and Billeter2016). Preference for oviposition sites with pre-existing eggs may be adaptive (Wertheim et al., Reference Wertheim, Marchais, Vet and Dicke2002; Rosa et al., Reference Rosa, van Nouhuys and Saastamoinen2017). When flies oviposited at sites where previous eggs were deposited by female flies, low densities of larvae had higher survival than single larva (~85% vs ~45% respectively); whereas larvae at high densities had survival roughly equal to single individuals (Wertheim et al., Reference Wertheim, Marchais, Vet and Dicke2002). Similarly, a recent study of Melitaea cinxia observed that larvae under higher densities obtained larger sizes, developed faster, and without any negative effect on survival (Rosa et al., Reference Rosa, van Nouhuys and Saastamoinen2017).
Although the mites in this study were restrained behind a mesh screen, the presence of mites affected host decision-making. A previous study with D. nigrospiracula showed chronic exposure to mites across a mesh screen reduced female fecundity by 13% (Horn and Luong, Reference Horn and Luong2018). Hence, parasites can have significant trait-mediated impacts on host fitness even if infection does not occur. The altered habitat use (i.e. oviposition behaviour) observed in this study may in part explain the previously observed losses in host fitness. However, mite presence did not influence host preference for ovipositing substrate when the environment differed in other respects. D. nigrospiracula tolerated mite presence while avoiding ovipositing at sites without cactus; this tolerance may be driven by an apparently overwhelming developmental requirement for specific host plants among cactus flies observed here and in prior studies (Fellows and Heed, Reference Fellows and Heed1972; Fanara et al., Reference Fanara, Fontdevila and Hasson1999). Cactiphilic flies are typically adapted to survive and reproduce on specific host plants with unique biochemical (e.g. specific pH) and nutritional properties (Fanara et al., Reference Fanara, Fontdevila and Hasson1999; Soto et al., Reference Soto, Carreira, Corio, Padro, Soto and Hasson2014). By specializing in saguaro cactus D. nigrospracula may not be able to exploit resources other than the natural host plant (Fellows and Heed, Reference Fellows and Heed1972; Soto et al., Reference Soto, Carreira, Corio, Padro, Soto and Hasson2014). Consequently, highly host-specific organisms may remain ‘trapped’ at parasite-infested habitats if other suitable host plants are not available (Fellows and Heed, Reference Fellows and Heed1972). Since hosts may not necessarily escape mite infested environments the non-consumptive effects of parasites, whereby parasites adversely impact hosts even in the absence of infection, may become even more important (Geraldi and Macreadie, Reference Geraldi and Macreadie2013; Horn and Luong, Reference Horn and Luong2018). These results suggest the impacts of ecology of fear in host–parasite systems may be environmentally-mediated, and future work should investigate the relative impacts of consumptive and non-consumptive effects in heterogeneous environments (Geraldi and Macreadie, Reference Geraldi and Macreadie2013).
The aversion to mites was higher in magnitude than attraction towards seeded sites – only 24% of eggs (76% avoidance) were deposited at mite-infested sites vs 37% of eggs (63% avoidance) at unseeded sites. Thus it was surprising that the presence of mites had no significant effect on the preference of flies between seeded and unseeded sites. We suggest two possible reasons why the attraction to substrates with conspecific eggs outweighed the risk of mite exposure. First, reliable fitness benefits (e.g. larger pupae and adult stages) from the presence of conspecifics may outweigh the variable fitness costs of parasitism (Rosa et al., Reference Rosa, van Nouhuys and Saastamoinen2017; Koskella, Reference Koskella2018). If the odds of parasite exposure are inconsistent due to environmental heterogeneity, it may lead to decreased selection for and/or plasticity in mite avoidance behaviours (de Jong, Reference de Jong1995; Vilcinskas, Reference Vilcinskas2013). Second, the presence of conspecifics may reduce the offspring's risk of infection through dilution and/or improved avoidance. For instance, increased host density arising from aggregation or grouping behaviours can reduce the probability of individual hosts becoming infected (Hall et al., Reference Hall, Scanlan, Morgan and Buckling2011; Pascua et al., Reference Pascua, Hall, Best, Morgan, Boots and Buckling2014). A recent meta-analysis found that, perhaps counterintuitively, larger host populations had lower mean infection intensities of mobile parasites (Patterson and Ruckstuhl, Reference Patterson and Ruckstuhl2013). Additionally, hosts living in groups may have improved detection and/or avoidance of parasites. Mikheev et al. (Reference Mikheev, Pasternak, Taskinen and Valtonen2013) found that fish in groups were more effective at avoiding habitats containing infective stages of parasites and accumulated fewer infections as a consequence.
The spatial positioning behaviour of adult flies, i.e. habitat distribution during feeding, mating, and rest, may differ from their ovipositing behaviour. A study on the D. melanogaster response to acetic acid found that flies detected the same cue through two different sensory inputs (Joseph et al., Reference Joseph, Devineni, King and Heberlein2009). Gustatory inputs lead to an oviposition attraction towards sites with higher concentrations of acetic acid, while olfactory inputs induced positional avoidance (Joseph et al., Reference Joseph, Devineni, King and Heberlein2009). Female flies laid ~90% of their eggs at sites with higher acetic acid concentrations while simultaneously spending significantly less time at those sites (Joseph et al., Reference Joseph, Devineni, King and Heberlein2009). This may be a result of fly larva requiring different conditions/nutrition than adult flies (Sang and King, Reference Sang and King1961; Rodrigues et al., Reference Rodrigues, Martins, Balance, Broom, Dias, Fernandes, Rodrigues, Sucena and Mirth2015; Horn and Luong Reference Horn and Luong2018). These results suggest that flies may be adapted to oviposit at sites that are subpar for the adult survival but beneficial for larval development, while also avoiding those sites when not laying eggs. This prediction is supported by previous observations that older flies may have higher risks of infection and experience larger fitness costs if they become infected (Polak, Reference Polak1996). Additionally, there may be age-mediated risks of infection such that older flies are at greater risk of infection than younger flies. Mites prefer hosts with higher metabolic rate flies, and D. nigrospiracula metabolic rate increases for approximately 2 weeks post eclosion maximizing during the period of peak reproductive output (Luong and Polak, Reference Luong and Polak2007; Horn et al., Reference Horn, Mierzejewski and Luong2018). This may indicate that older flies are either more likely to be attacked and/or mount weaker defences against infection. Newly emerged adults, therefore, may have higher fitness at environmentally superior sites even if mites are present compared to older flies which experience greater risks from mite exposure. Future work could measure if, when given a choice between environmentally preferred sites with mites and ill-favoured sites without mites, flies spend more time at the inferior site but oviposit at the preferred site.
Ovipositing behaviour in insects is heritable and can have significant implications for the ecology and evolution of host species (Singer et al., Reference Singer, Ng and Thomas1988; Thompson, Reference Thompson1988; Atkinson et al., Reference Atkinson, McVean and Stone2002). Parasitism is hypothesized to have influenced the evolution of animal personality by leading to consistently expressed behaviours across time (Barber and Dingemanse, Reference Barber and Dingemanse2010; Kortet et al., Reference Kortet, Hedrick and Vainikka2010). Egg-laying behaviour may be an example of how the selective pressures exerted by parasitism can lead to the evolution of animal personalities (Kortet et al., Reference Kortet, Hedrick and Vainikka2010; Klemme and Karvonen, Reference Klemme and Karvonen2016). Furthermore, different selective pressures on host reproduction may lead to host populations with different habitat use and reproductive behaviour, both of which can lead to reduced gene flow and pre-zygotic reproductive isolation (Karvonen and Seehausen, Reference Karvonen and Seehausen2012). Alterations in gene flow due to anti-parasite behaviours may have consequences for parasite-mediated speciation (Coyne and Orr, Reference Coyne and Orr1997; Brunner and Eizaguirre, Reference Brunner and Eizaguirre2016).
In conclusion, we found that although flies avoided ovipositing at sites with mites when the environments were otherwise identical, environmental preferences tended to overwhelm parasite aversion. In the field of parasite ecology, there has been an increased focus on the behavioural responses of hosts and the resulting non-consumptive effects of parasites (Raffel et al., Reference Raffel, Martin and Rohr2008; Horn and Luong, Reference Horn and Luong2019). However, the non-consumptive consequences of living in close proximity with parasites on host behaviour are not fully understood. Our results show that host decision-making in response to parasites is context-dependent and strongly influenced by a heterogeneous environment. Future studies in the ecology of fear as it pertains to parasites should investigate potential inter-specific variation in parasite avoidance due to the variable costs of infection parasites have on their hosts which may alter the risk-reward ratio of avoiding otherwise suitable habitats with parasites. Our study expands on the behavioural ecology of host–parasite systems by considering the relative effects parasites have on host reproductive behaviour compared to other environmental considerations.
Author ORCIDs
Collin J. Horn, 0000-0002-6384-4193
Acknowledgements
Flies and mites used in this experiment were cultured by Maesha Elahi and Taylor Brophy respectively. We would like to thank two anonymous reviewers for feedback on this manuscript.
Financial support
Lien Luong is funded by an NSERC Discovery Grant (#435245).
Conflict of interest
None.
Ethical standards
Not applicable.