Introduction
Global amphibian declines have been attributed to multiple factors including habitat loss and fragmentation, invasive species, pollution, climate change and disease (Kiesecker et al., Reference Kiesecker, Blaustein and Belden2001; Daszak et al., Reference Daszak, Cunningham and Hyatt2003; Blaustein et al., Reference Blaustein, Gervasi, Johnson, Hoverman, Belden, Bradley and Xie2012). Pathogens such as ranavirus and Batrachochytrium dendrobatidis, which have wide spatial distributions and contribute to mortality events that can affect population stability, are obvious targets for research (Skerratt et al., Reference Skerratt, Berger, Speare, Cashins, Mcdonald, Phillott, Hines and Kenyon2007; Duffus and Cunningham, Reference Duffus and Cunningham2010; Price et al., Reference Price, Garner, Nichols, Balloux, Ayres, de Alba and Bosch2014). However, many other pathogens affect free-living amphibians and may have population-level effects which are poorly understood or masked by co-infection with more virulent agents (Pessier Reference Pessier2008). One example is the Ichthyosporea, a class of protists in the Opisthokonta. Within the Ichthyosporea (junior synonym Mesomycetozoea), the order Dermocystida consists entirely of pathogens affecting mammals, birds, fish and amphibians (Cavalier-Smith, Reference Cavalier-Smith, Coombs, Vickerman, Sleigh and Warren1998; Mendoza et al., Reference Mendoza, Taylor and Ajello2002).
Until recently, Dermocystid parasites infecting amphibians were classified into two genera, Amphibiocystidium and Amphibiothecum (Pascolini et al., Reference Pascolini, Daszak, Cunningham, Tei, Vagnetti, Bucci, Fagotti and Di Rosa2003; Feldman et al., Reference Feldman, Wimsatt and Green2005; Pereira et al., Reference Pereira, Di Rosa, Fagotti, Simoncelli, Pascolini and Mendoza2005). Currently, amphibian dermocystids are assigned to six genera (Amphibiocystidium, Dermocystidium, Dermosporidium, Dermotheca, Sphaerothecum and Valentines) to ensure monophyly within each genus and reflect the discovery of Sphaerothecum caipira infecting frogs (Borteiro et al., Reference Borteiro, Baldo, Maronna, BaÊta, Sabbag, Kolenc, Debat, Haddad, Cruz, Verdes and Ubilla2018a). Representatives of these genera have been documented most frequently in European anurans and urodeles including common frogs (Rana temporaria), water frogs (Pelophylax esculentus complex), Italian stream frogs (Rana italica), common midwife toads (Alytes obstetricans), palmate newts (Lissotriton helveticus), common newts (Lissotriton vulgaris), marbled newts (Triturus marmoratus) and crested newts (Triturus cristatus) from Italy, France, Germany, Switzerland, Czechoslovakia, Scotland and the Netherlands (Pérez, Reference Pérez1907; Moral, Reference Moral1913; Pérez, Reference Pérez1913; Granata, Reference Granata1919; Guyénot and Naville, Reference Guyénot and Naville1922; Remy, Reference Remy1931; Poisson, Reference Poisson1937; Broz and Privora, Reference Broz and Privora1952; Broz and Kulda, Reference Broz and Kulda1954; Pascolini et al., Reference Pascolini, Daszak, Cunningham, Tei, Vagnetti, Bucci, Fagotti and Di Rosa2003; Pereira et al., Reference Pereira, Di Rosa, Fagotti, Simoncelli, Pascolini and Mendoza2005; Duffus and Cunningham, Reference Duffus and Cunningham2010; González-Hernández et al., Reference González-Hernández, Denoël, Duffus, Garner, Cunningham and Acevedo-Whitehouse2010; Courtois et al., Reference Courtois, Cornuau, Loyau and Schmeller2013; Stark and Guex, Reference Stark and Guex2014; Federici et al., Reference Federici, Rossi, Fidati, Paracucchi, Scargetta, Montalbani, Franzetti, La Porta, Fagotti, Simonceli, Cenci and Di Rosa2015; Fiegna et al., Reference Fiegna, Clarke, Shaw, Baily, Clare, Gray, Garner and Meredith2017; Fagotti et al., Reference Fagotti, Rossi, Canestrelli, La Porta, Paracucchi, Lucentini, Simoncelli and Di Rosa2019). North American dermocystids include Dermocystidium penneri, which affects American toads (Anaxyrus americanus), Fowler's toads (Anaxyrus fowleri), and Yosemite toads (Anaxyrus canorus) (Jay and Pohley, Reference Jay and Pohley1981; Green and Sherman, Reference Green and Sherman2001; Green et al., Reference Green, Converse and Schrader2002; Converse and Green, Reference Converse, Green, Majumdar, Huffman, Brenner and Panah2005), and Dermotheca viridescens, which affects eastern newts (Notophthalmus viridescens) from Pennsylvania, Massachusetts and West Virginia (Raffel et al., Reference Raffel, Bommarito, Barry, Witiak and Shackelton2008).
Amphibians infected with dermocystids develop single or multiple cutaneous lesions, typically appearing as either 1–5 mm round, c-shaped, u-shaped, or dumbbell-shaped white nodules or 1–7 mm vesicular structures which may coalesce and ulcerate. Lesions have also been observed infrequently within gastrointestinal mucosa and internal organs (Raffel et al., Reference Raffel, Bommarito, Barry, Witiak and Shackelton2008; Fiegna et al., Reference Fiegna, Clarke, Shaw, Baily, Clare, Gray, Garner and Meredith2017). Histologically, nodular foci consist of sporangia containing microscopic endospores (Mendoza et al., Reference Mendoza, Taylor and Ajello2002). Host response to the sporangia is typically minimal until they rupture, at which point a localized immune response can cause lesions to appear inflamed (Pérez, Reference Pérez1913; Broz and Kulda, Reference Broz and Kulda1954; Pascolini et al., Reference Pascolini, Daszak, Cunningham, Tei, Vagnetti, Bucci, Fagotti and Di Rosa2003; Raffel et al., Reference Raffel, Bommarito, Barry, Witiak and Shackelton2008; Fiegna et al., Reference Fiegna, Clarke, Shaw, Baily, Clare, Gray, Garner and Meredith2017). Information on recovery period is limited, though anecdotal evidence suggests that the complete resolution of these lesions in mildly affected individuals is possible within a few weeks (Raffel et al., Reference Raffel, Bommarito, Barry, Witiak and Shackelton2008).
Infection with dermocystid pathogens was initially believed to be fairly benign; however, heavily infected newts from France, Scotland, Germany and the USA have demonstrated more severe clinical signs and pathologic changes, including regional oedema, haemorrhages, extensive cutaneous ulceration, development of secondary bacterial infections and death (Moral, Reference Moral1913; Gambier, Reference Gambier1924; González-Hernández et al., Reference González-Hernández, Denoël, Duffus, Garner, Cunningham and Acevedo-Whitehouse2010; Raffel et al., Reference Raffel, Bommarito, Barry, Witiak and Shackelton2008; Fiegna et al., Reference Fiegna, Clarke, Shaw, Baily, Clare, Gray, Garner and Meredith2017). Amphibian skin plays a major role in osmoregulation, electrolyte balance and defence against pathogen invasion (Woodhams et al., Reference Woodhams, Rollins-Smith, Alford, Simon and Harris2007; Rollins-Smith, Reference Rollins-Smith2009). Disruption of these vital functions in animals with heavy dermocystid burdens may result in death due to fatal electrolyte disturbances, similar to chytridiomycosis (Campbell et al., Reference Campbell, Voyles, Cook and Dinudom2012). Indeed, an increased mortality rate was reported for captive N. viridescens infected with D. viridescens, compared to newts without visible lesions (Raffel et al., Reference Raffel, Bommarito, Barry, Witiak and Shackelton2008). Alternatively, development of opportunistic secondary infections may contribute to morbidity and mortality. Amphibian dermocystid infections have also been linked to cutaneous microbiome changes favouring fungal colonization and dermal granular gland shrinkage, which may indicate the reduction of antimicrobial peptide secretion and subsequent immune dysfunction (Federici et al., Reference Federici, Rossi, Fidati, Paracucchi, Scargetta, Montalbani, Franzetti, La Porta, Fagotti, Simonceli, Cenci and Di Rosa2015; Fiegna et al., Reference Fiegna, Clarke, Shaw, Baily, Clare, Gray, Garner and Meredith2017). Further study of dermocystid parasites is needed to understand the threat that these organisms may pose to vulnerable amphibian populations. This study seeks to characterize dermocystid infection in a North American salamander species of conservation concern.
The triploid, unisexual silvery salamander (Ambystoma platineum) occurs in the Northeastern and Midwestern United States and originated through hybridization of blue-spotted salamanders (A. laterale) and Jefferson salamanders (A. jeffersonianum), resulting in retention of two sets of A. jeffersonianum chromosomes and one set of A. laterale chromosomes (JJL; Uzzell, Reference Uzzell1964,; Uzzell and Goldblatt, Reference Uzzell and Goldblatt1967). Silvery salamanders typically coexist in habitats with one of their parental species and act as sexual parasites on the males of that species. However, some populations, including those in Illinois, do not occur with either parental species. In these populations, silvery salamanders utilize sperm from small-mouthed salamanders (A. texanum) for kleptogenic reproduction (Uzzell and Goldblatt, Reference Uzzell and Goldblatt1967; Morris and Brandon, Reference Morris and Brandon1984; Spolsky et al., Reference Spolsky, Phillips and Uzzell1992; Phillips et al., Reference Phillips, Uzzell, Spolsky, Serb, Szafoni and Pollowy1997; Teltser and Greenwald, Reference Teltser and Greenwald2015).
Silvery salamanders are found in only two counties in Illinois and are considered state-endangered (Illinois Endangered Species Protection Board, 2015). Threats to the persistence of silvery salamanders in Illinois are largely unknown, but an ongoing demographic and health assessment study has been initiated to examine conservation challenges for this species. The primary objective of this study is to provide a morphologic, histologic and molecular description of dermocystid infection in free-living silvery salamanders in Illinois, and secondarily, to describe the incidental detection of dermocystids in sympatric small-mouthed salamanders.
Methods
Demographic survey
Silvery salamanders were evaluated at six ephemeral wetlands distributed among three study sites including Collison (wetland 71), Middle Fork (MF, wetlands 76 & 285) and Kickapoo State Park (KSP, wetlands 67, 282, and 283) in Vermilion County, Illinois. Exact wetland locations are not provided to protect the animals but are available upon request. In 2016, salamanders were captured using minnow traps and dip-nets with a standardized number of traps and dip-net sweeps used based on wetland size. In 2017 and 2018, study wetlands were encircled with drift-fences and associated pitfall traps to monitor salamander ingress and egress during breeding migrations. Traps were checked daily from February 1st to April 15th 2017 and 2018 and salamanders were toe-clipped using a wetland specific numbering system to estimate fence trespass rates.
Health assessments
Salamander health assessments were performed for 3–4 days each year during breeding migrations. Animals were measured (snout-vent length and total length; mm) and weighed (g) and a complete physical examination was performed by a veterinarian (LA) noting appearance of the eyes, nose, oral cavity, integument, musculoskeletal system and cloaca. During health assessments in 2017 white cutaneous nodules consistent with dermocystid infection were observed on multiple animals. Digits with nodules from two different salamanders were opportunistically excised during routine toe-clipping and immediately placed in 100% ethanol. A single male small-mouthed salamander from wetland 67 was found with white cutaneous nodules on the trunk. The skin overlying the nodules was swabbed and the animal was released. In 2018 one heavily affected silvery salamander (21 nodules) and one heavily affected small-mouthed salamander (>50 nodules) from pond 283 were euthanized using an overdose of buffered MS-222 (3-Aminobenzoic Acid Ethyl Ester, Sigma Chemical Co. St. Louis, MO 61378). An additional heavily affected silvery salamander (>50 nodules) was found dead within a bucket trap at the same site. Two skin nodules from each animal were collected using a sterile scalpel blade and dry-frozen at −20°C. The bodies were then preserved in 10% buffered formalin and submitted for histopathology.
Pathologic evaluation
Fixed tissues collected during health assessments were processed routinely for histopathology and stained with hematoxylin and eosin. Slides were evaluated via light microscopy by an anatomic pathologist. Images were taken using an Olympus DP71 digital microscope camera.
DNA extraction, polymerase chain reaction (PCR) and sequencing
DNA was extracted from the skin swab and frozen and ethanol-preserved tissue samples using a commercial kit (DNeasy Blood & Tissue Kit, Qiagen, Valencia, CA, USA). Quality and purity of resulting DNA samples was evaluated spectrophotometrically (NanoDrop 1000, Thermo Fisher Scientific, 260 Waltham, MA, USA). Two sets of existing consensus PCR primers targeting the 18S rRNA gene were used to screen tissue samples for the presence of dermocystids (González-Hernández et al., Reference González-Hernández, Denoël, Duffus, Garner, Cunningham and Acevedo-Whitehouse2010; Fiegna et al., Reference Fiegna, Clarke, Shaw, Baily, Clare, Gray, Garner and Meredith2017). Each reaction contained 1 × PCR buffer, 2.5 mm magnesium chloride, 0.2 mm dNTPs, 2.5 U Platinum Taq polymerase, 0.2 μ m of each primer, 1 μL template DNA (<1μg) and sterile deionized water to bring the final volume to 50 μL (Platinum Taq DNA Polymerase, Invitrogen, Carlsbad, CA 92008). Thermocycler settings consisted of a 5-minute initial denaturation at 95°C followed by 40 cycles of 95°C for 30 s, 57.7°C for 30 s and 72°C for 120 s, then a final elongation step of 72°C for 7 minutes. The optimal annealing temperature of 57.7°C was determined using a thermal gradient testing 10 temperatures from 53 to 65°C (data not shown). A positive control consisting of sequence-confirmed D. penneri from a Fowler's toad (Anaxyrus fowleri) and a non-template control (sterile water) were included in each run. Products were resolved on a 1% agarose gel and samples with appropriately sized bands (1400 bp) were treated with ExoSAP-IT (USB Corporation, 26111 miles Road, Cleveland, OH, USA) and commercially sequenced in both directions. Sequences were trimmed of primers and low-quality base-pair calls in Geneious v. 11.1.3 (Kearse et al., Reference Kearse, Moir, Wilson, Stones-Havas, Cheung, Sturrock, Buxton, Cooper, Markowitz, Duran, Thierer, Ashton, Mentjies and Drummond2012) and compared to known sequences in the NCBI GenBank database using BLASTN (Benson et al., Reference Benson, Karsch-Mizrachi, Lipman, Ostell and Wheeler2008).
Phylogenetic analysis
18s rRNA sequences that were 92–99% similar to the silvery and small-mouthed salamander dermocystids were recovered from GenBank using BLASTN. Sequences were aligned using MAFFT v. 7.388 (Katoh and Standley, Reference Katoh and Standley2013) with default parameters as implemented in Geneious. The ends of the resulting alignment file were manually adjusted and trimmed in Geneious. In order to estimate the best substitution model for phylogenetic analysis, PartitionFinder v. 2.1.1 (Lanfear et al., Reference Lanfear, Senfeld, Frandsen, Wright and Calcott2017) was used to search through all 195 nucleotide models using the corrected Akaike Information Criterion (AICc; Sugiura Reference Sugiura1978). Maximum likelihood phylogenetic reconstruction was performed in RAxML v.8.1.3 using a GTR GAMMA model with 100 bootstrap replicates (Stamatakis, Reference Stamatakis2014). Bootstrap support (BS) was then summarized on the best tree. Bayesian phylogenetic reconstruction was performed using MrBayes v. 3.2.6 (Ronquist et al., Reference Ronquist, Teslenko, van der Mark, Ayres, Darling, Höhna, Larget, Liu, Suchard and Huelsenbeck2012) with a GTR + I + G substitution model and 20 million generations of Markov Chain Monte Carlo (MCMC) models for three runs of four chains each, sampling every 1000 trees. Trace files were viewed in Tracer v. 1.6 (Rambaut and Drummond, Reference Rambaut and Drummond2007) to assess parameter convergence. To assess topological convergence, .t files were assessed using the R package RWTY v. 1.0.1 (Warren et al., Reference Warren, Geneva and Lanfear2017). Based on these assessments, the first 10% (2000 trees) were discarded as burn-in.
Museum specimen survey
A total of 125 A. platineum specimens collected from Vermilion County, Illinois and maintained in the Illinois Natural History Survey (INHS) and University of Illinois Museum of Natural History (UIMNH) collections were visually examined for the presence of white skin nodules consistent with dermocystid infection.
Statistical analysis
All statistical evaluations were performed in R v 3.5.1 (R Core Team 2018). Descriptive statistics for skin nodule prevalence were tabulated by year and wetland. Ninety-five per cent confidence intervals for binomial probabilities were constructed around prevalence estimates using the binconf function in the Hmisc package. The effect of wetland ID and study site on the probability of dermocystid detection was assessed in each year using generalized linear models, package brglm (Kosmidis Reference Kosmidis2017). Post-hoc between group differences were evaluated using the contrasts function in the lsmeans package (Lenth Reference Lenth2016).
Sample size calculations were performed to determine the number of salamander examinations needed to detect varying levels of dermocystid prevalence (1%, 2%, 3%, 4%, 5%, 10%, 20%), at different salamander population sizes (50, 100, 200, 300, 500, 1000 and 5000). The specificity for visual detection of dermocystid skin nodules was assumed to be one, while a conservative value of 0.8 was chosen for sensitivity, based on previous studies (Raffel et al., Reference Raffel, Bommarito, Barry, Witiak and Shackelton2008, González-Hernández et al., Reference González-Hernández, Denoël, Duffus, Garner, Cunningham and Acevedo-Whitehouse2010; Fiegna et al., Reference Fiegna, Clarke, Shaw, Baily, Clare, Gray, Garner and Meredith2017). Herd sensitivity, or the probability of infected salamanders being detected at a wetland where dermocystid infection is actually present, was set at 0.95. These calculations were performed using an online tool provided at epitools.ausvet.com.au, based on an approximation of the hypergeometric distribution modified to account for imperfect diagnostic test sensitivity (MacDiarmid Reference MacDiarmid1988).
Results
Sample population
In total 36 silvery salamanders were examined on March 3–4th 2016, 390 individuals were assessed on February 24th, February 28th and March 7th, 2017 and 220 were examined on February 19–21st and 25th, 2018. The proportion of salamanders examined compared to the total number of unique captures per wetland each year ranged from 12 to 83%, reflecting somewhat uneven sampling effort for the health assessment component of this study (Table 1).
Table 1. Sample size and Dermotheca sp. prevalence in adult silvery salamanders (Ambystoma platineum) surveyed in Vermilion County, Illinois in 2017 and 2018.
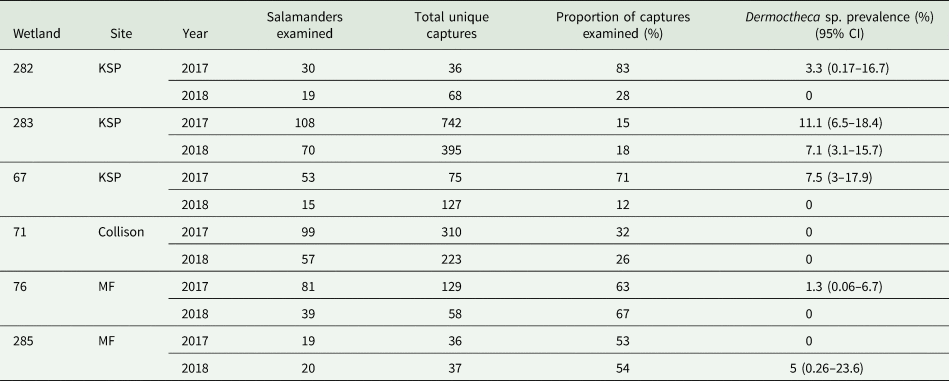
White cutaneous nodules consistent with dermocystid infection were not observed in 2016. In 2017, the prevalence of nodules was higher at KSP than MF (OR = 6.65, 95% CI = 3.59–36.18, P = 0.028) and Collison (OR = 20, 95% CI = 1.2–340, P = 0.039). Prevalence was also higher at wetland 283 than wetlands 76 (OR = 6.95, 95% CI = 1.23–39.19, P = 0.028) and 71 (OR = 25.8, 95% CI = 1.45–448.8, P = 0.026). In 2018, the prevalence of nodules was not significantly different between sites or wetlands. Skin nodules consistent with dermocystid infection were not detected in Collison (wetland 71) at any point during the study.
Sample size calculations indicate that the number of salamanders examined per wetland prior to 2017 was inadequate to detect dermocystids at a prevalence of 20% or lower (Table 2). Using a total number of unique captures as a (limited) proxy for population size, sampling effort was also likely inadequate to detect dermocystids at a prevalence of 20% or lower at wetland 285 in 2017 and wetlands 67 and 282 in 2018.
Table 2. Number of silvery salamander (Ambystoma platineum) examinations needed to detect Dermotheca sp. at different levels of prevalence and different salamander population sizes
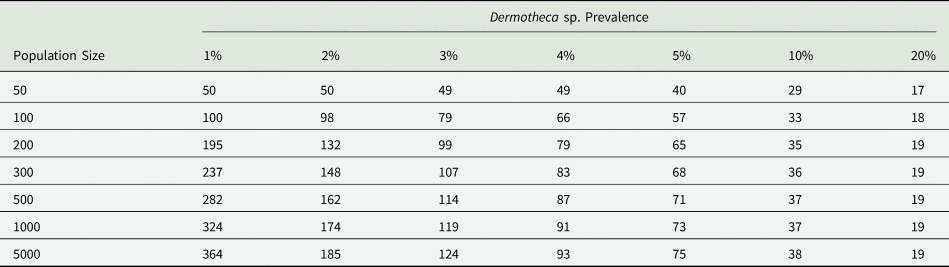
Calculations were performed using an approximation of the hypergeometric distribution modified to account for imperfect diagnostic test sensitivity. Sensitivity = 0.8, specificity = 1, herd sensitivity = 0.95.
Gross and histologic lesion description
Skin nodules consistent with dermocystid infection in silvery salamanders consisted of 1–3 mm white, raised, slightly firm subcutaneous nodules concentrated on the trunk (Figs 1 and 2). Individual nodules were round to dumbbell-shaped and occasionally curved or C-shaped. Most salamanders had fewer than 10 lesions. Hepatic nodules were identified in the most heavily affected silvery salamander at necropsy, but internal lesions were not identified in the other two necropsied animals.
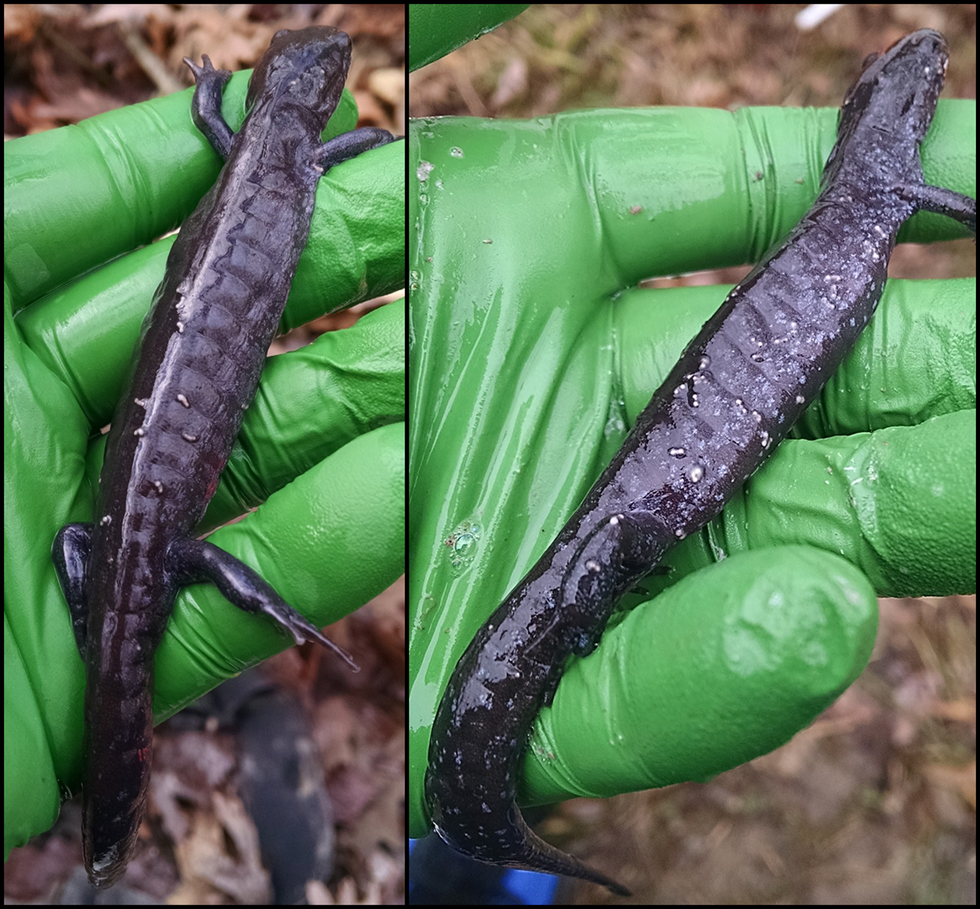
Fig. 1. Gross appearance of skin lesions associated with Dermotheca sp. infection in free-living silvery salamanders (Ambystoma platineum) from Vermilion County, IL 2017–2018.
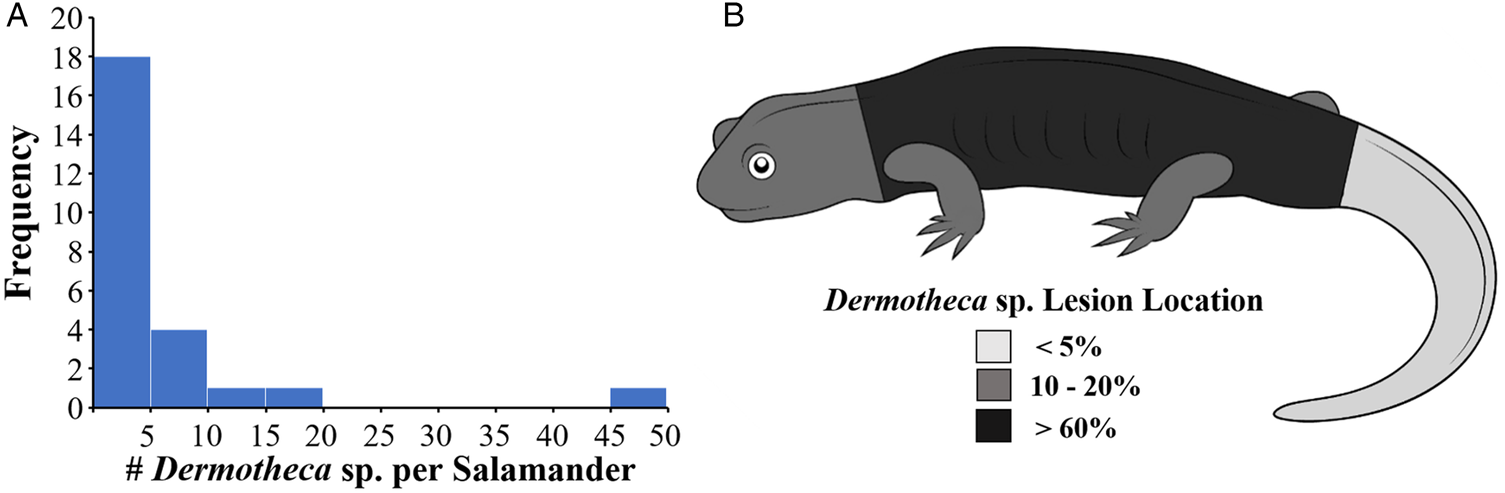
Fig. 2. Intensity (A) and distribution (B) of Dermotheca sp. lesions in 24 affected adult silvery salamanders (Ambystoma platineum) from Vermilion County, IL 2017–2018.
On histologic examination, grossly apparent skin and hepatic nodules were consistent with ichthyosporean sporangia (Fig. 3). Sporangia were located within the superficial dermis and subcapsular hepatic parenchyma and mildly compressed surrounding tissue. Each sporangium ranged from 300 to 1000 μm in diameter and had a thin (5–10 μm) eosinophilic, hyalinized wall. Sporangia contained numerous 6–10 μm round endospores with 5 μm round eosinophilic cytoplasmic bodies, colorless cytoplasm and small round peripheral nuclei. Only minimal tissue reaction was observed in association with these sporangia and consisted primarily of mild hyperplasia (up to seven cell layers) in the overlying epidermis. Rare sporangia were segmentally ruptured with low numbers of free endospores and minimal heterophilic inflammation in the immediately adjacent dermis or hepatic parenchyma. Most observed sporangia were intact with no associated inflammation, though a few larger sporangia contained low numbers of central viable and necrotic heterophils. The sporangia capsules stained strongly with Gomori Methenamine-Silver Nitrate Stain (GMS), and the endospores had diffusely strong reactions with Periodic acid–Schiff (PAS) stain.
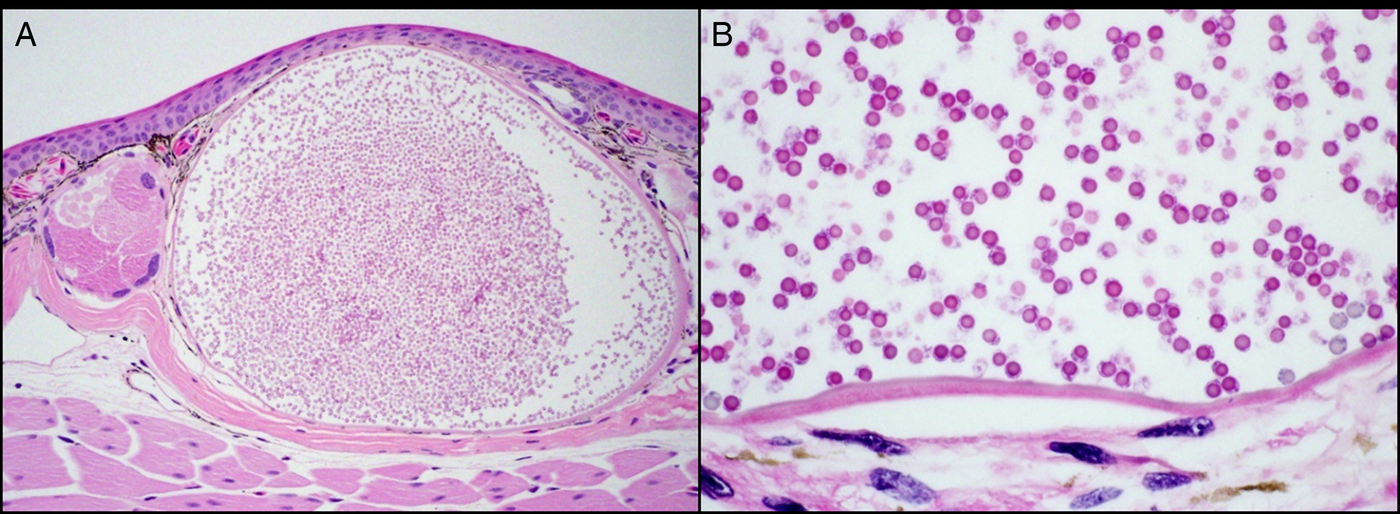
Fig. 3. Skin from Ambystoma platineum. Within the superficial dermis is a single ichthyosporean sporangium that elevates the epidermis and slightly compresses surrounding tissues (A, hematoxylin and eosin stain, 100X). The sporangium has a thin eosinophilic hyalinized wall and contains numerous 6–10 μm round endospores (B, hematoxylin and eosin stain, 600X).
Molecular & phylogenetic analysis
An approximately 1350 bp product was amplified from the skin swab and two digits collected in 2017 and the euthanized/deceased silvery and small-mouthed salamanders collected in 2018 using both sets of primers. The aligned portions of all sequences were identical, and representative data were deposited in GenBank (Accession numbers MK330881.1, MK757499.1, MK757500.1, MK757501.1). Sequences from the present study were 99% similar to D. viridescens and a Dermotheca sp. infecting Italian stream frogs in Europe (Accession # EU650666).
After the 10% burn-in, all parameters and topologies from the Bayesian phylogenetic analysis had ESS values >>200 or average standard deviation of split frequencies <0.01, indicating the MCMC runs had converged to stationarity. BS values in the maximum likelihood analysis averaged 67%, and posterior probability (PP) support averaged 97% in the Bayesian analysis. The maximum likelihood and Bayesian phylogenetic analyses produced similar trees, recovering Sphaerothecum as sister to Rhinosporidium, Valentines, Dermocystidium, Amphibiocystidium and Dermotheca with >66% BS and >97% PP (Figs 4 and 5). The silvery and small-mouthed salamander sequences were recovered in a monophyletic group with Dermotheca spp. (70 BS/0.99 PP).

Fig. 4. Phylogenetic tree for ichthyosporean organisms produced by maximum-likelihood analytic methods.

Fig. 5. Phylogenetic tree for ichthyosporean organisms produced by Bayesian analytic methods.
Historic occurrence
A total of 125 A. platineum specimens collected in Vermilion county, Illinois from 1973 to 2018 were visually inspected for the presence of white nodules consistent with dermocystid infection. Sampling effort during this time period was haphazard, with the most specimens collected in the early 1980s (18 salamanders in 1980, 32 in 1981). Following this peak, sample sizes only exceeded 10 individuals per year in 2000 and 2018. Cutaneous nodules were not identified in any specimen. Tissue sampling for molecular screening was not pursued due to initial fixation of specimens in formalin prior to long-term storage in ethanol.
Discussion
This is the first report of Dermotheca sp. in the Midwestern United States and the first detection of this organism in ambystomatid salamanders. The gross appearance of Dermotheca sp. is consistent with D. viridescens and slightly different from all other dermocystid species (Raffel et al., Reference Raffel, Bommarito, Barry, Witiak and Shackelton2008). Lesions in Amphibiocystidium ranae infections are similar in size and color, but mature into more dramatic U-shaped sporangia (Guyénot and Naville, Reference Guyénot and Naville1922; Remy, Reference Remy1931; Broz and Privora, Reference Broz and Privora1952; Broz and Kulda, Reference Broz and Kulda1954; Pascolini et al., Reference Pascolini, Daszak, Cunningham, Tei, Vagnetti, Bucci, Fagotti and Di Rosa2003; Pereira et al., Reference Pereira, Di Rosa, Fagotti, Simoncelli, Pascolini and Mendoza2005). Dermocystidium pusula lesions start out round and similarly sized (1–3 mm), but have varying coloration and can progress to much larger (5–8 mm) cysts with 1–4 openings prior to ulceration. These lesions are also occasionally hemorrhagic and may induce localized or systemic oedema, which has not been described for other dermocystid infections (Pérez, Reference Pérez1907, Reference Pérez1913; Moral, Reference Moral1913; Granata, Reference Granata1919; González-Hernández et al., Reference González-Hernández, Denoël, Duffus, Garner, Cunningham and Acevedo-Whitehouse2010; Fiegna et al., Reference Fiegna, Clarke, Shaw, Baily, Clare, Gray, Garner and Meredith2017). Dermocystidium penneri lesions are large (4–6 mm) and range in shape from round to oval to serpiginous tracts of divided chambers (Jay and Pohley, Reference Jay and Pohley1981; Feldman et al., Reference Feldman, Wimsatt and Green2005). Sphaerothecum caipira, Dermosporidium hylarum and Valentines rwandae all produce variably colored, 0.5–1 mm round lesions with or without ulceration (Carini, Reference Carini1940; Borteiro et al., Reference Borteiro, Cruz, Kolenc, Verdes, Moraña, Martínez Debat, Kun, Ubilla and Okada2014; Scheid et al., Reference Scheid, Balczun, Dehling, Ammon and Sinsch2015; Borteiro et al., Reference Borteiro, Baldo, Maronna, BaÊta, Sabbag, Kolenc, Debat, Haddad, Cruz, Verdes and Ubilla2018a). Elongated, curved and dumbbell-shaped lesions have not been reported for these three dermocystid species.
Cutaneous Dermotheca sp. nodules in silvery salamanders are distributed across the body in a similar way to most other dermocystids. The dorsal trunk is most commonly affected by S. caipira. V. rwandae, and D. pusula, and the ventral trunk by A. ranae, D. viridescens and D. penneri infections (Moral, Reference Moral1913; Broz and Privora, Reference Broz and Privora1952; Jay and Pohley, Reference Jay and Pohley1981; Pascolini et al., Reference Pascolini, Daszak, Cunningham, Tei, Vagnetti, Bucci, Fagotti and Di Rosa2003; Feldman et al., Reference Feldman, Wimsatt and Green2005; Pereira et al., Reference Pereira, Di Rosa, Fagotti, Simoncelli, Pascolini and Mendoza2005; Raffel et al., Reference Raffel, Bommarito, Barry, Witiak and Shackelton2008; González-Hernández et al., Reference González-Hernández, Denoël, Duffus, Garner, Cunningham and Acevedo-Whitehouse2010; Scheid et al., Reference Scheid, Balczun, Dehling, Ammon and Sinsch2015; Fiegna et al., Reference Fiegna, Clarke, Shaw, Baily, Clare, Gray, Garner and Meredith2017; Borteiro et al., Reference Borteiro, Baldo, Maronna, BaÊta, Sabbag, Kolenc, Debat, Haddad, Cruz, Verdes and Ubilla2018a). The head is a common site for lesion development in S. caipira and V. rwandae infections, the limbs are frequently affected by A. ranae and D. pusula, and the tail often hosts nodules in D. viridescens and D. pusula infections (Moral Reference Moral1913; Guyénot and Naville, Reference Guyénot and Naville1922; Raffel et al., Reference Raffel, Bommarito, Barry, Witiak and Shackelton2008; González-Hernández et al., Reference González-Hernández, Denoël, Duffus, Garner, Cunningham and Acevedo-Whitehouse2010; Fiegna et al., Reference Fiegna, Clarke, Shaw, Baily, Clare, Gray, Garner and Meredith2017; Borteiro et al., Reference Borteiro, Baldo, Maronna, BaÊta, Sabbag, Kolenc, Debat, Haddad, Cruz, Verdes and Ubilla2018a). Internal dermocystid infections have also been described, with D. viridescens sporangia identified in the liver, V. rwandae sporangia in cardiac muscle, Dermotheca sp. sporangia in palpebral conjunctivae and oral mucosa, and D. pusula sporangia in muscle tissue, oral mucosa, liver and cloacal stroma (Pérez Reference Pérez1907; Gambier, Reference Gambier1924; Raffel et al., Reference Raffel, Bommarito, Barry, Witiak and Shackelton2008; Fiegna et al., Reference Fiegna, Clarke, Shaw, Baily, Clare, Gray, Garner and Meredith2017; Borteiro et al., Reference Borteiro, Baldo, Maronna, BaÊta, Sabbag, Kolenc, Debat, Haddad, Cruz, Verdes and Ubilla2018a; Fagotti et al., Reference Fagotti, Rossi, Canestrelli, La Porta, Paracucchi, Lucentini, Simoncelli and Di Rosa2019). Systemic dissemination of D. penneri spores to the liver, kidneys and fat pads has also been identified in a Yosemite toad (Green and Sherman, Reference Green and Sherman2001). While hepatic sporangia were detected in a single silvery salamander with heavy cutaneous dermocystid infection, the frequency and intensity of internal and disseminated infection in ambystomatid salamanders cannot be assessed without further necropsy surveillance.
Dermotheca sp. sporangia from the present study were similar in size and structure compared to other dermocystid species, with the possible exception of A. ranae, D. pusula, D. penneri and (in a single instance) D. viridescens. Sporangia in these species contain internal septations dividing endospores into chambers – apparently based on spore developmental stage; this was not observed in the Dermotheca sp. from silvery salamanders (Granata 1919; Guyénot and Naville, Reference Guyénot and Naville1922; Broz and Privora, Reference Broz and Privora1952; Broz and Kulda, Reference Broz and Kulda1954; Jay and Pohley, Reference Jay and Pohley1981; Raffel et al., Reference Raffel, Bommarito, Barry, Witiak and Shackelton2008; González-Hernández et al., Reference González-Hernández, Denoël, Duffus, Garner, Cunningham and Acevedo-Whitehouse2010; Fiegna et al., Reference Fiegna, Clarke, Shaw, Baily, Clare, Gray, Garner and Meredith2017; Fagotti et al., Reference Fagotti, Rossi, Canestrelli, La Porta, Paracucchi, Lucentini, Simoncelli and Di Rosa2019). Dermotheca sp. endospores share similar histologic features with other dermocystids except the endospores of D. viridescens, D. hylarum, A. ranae, D. penneri and D. pusula, which contain variable numbers of cytoplasmic granules in addition to the larger cytoplasmic inclusion body (Carini, Reference Carini1940; Broz and Privora, Reference Broz and Privora1952; Broz and Kulda, Reference Broz and Kulda1954; Jay and Pohley, Reference Jay and Pohley1981; Green and Sherman, Reference Green and Sherman2001; Pascolini et al., Reference Pascolini, Daszak, Cunningham, Tei, Vagnetti, Bucci, Fagotti and Di Rosa2003; Feldman et al., Reference Feldman, Wimsatt and Green2005; Raffel et al., Reference Raffel, Bommarito, Barry, Witiak and Shackelton2008; González-Hernández et al., Reference González-Hernández, Denoël, Duffus, Garner, Cunningham and Acevedo-Whitehouse2010; Fiegna et al., Reference Fiegna, Clarke, Shaw, Baily, Clare, Gray, Garner and Meredith2017). Sporangia in all dermocystid species, including the Dermotheca sp. of the present study, are associated with mild epidermal hyperplasia. Most sporangia induced a minimal host inflammatory response that became more intense around ruptured or degenerating structures, similar to previous reports (Granata, Reference Granata1919; Pérez, Reference Pérez1913; Pascolini et al., Reference Pascolini, Daszak, Cunningham, Tei, Vagnetti, Bucci, Fagotti and Di Rosa2003; Pereira et al., Reference Pereira, Di Rosa, Fagotti, Simoncelli, Pascolini and Mendoza2005; Fiegna et al., Reference Fiegna, Clarke, Shaw, Baily, Clare, Gray, Garner and Meredith2017; Fagotti et al., Reference Fagotti, Rossi, Canestrelli, La Porta, Paracucchi, Lucentini, Simoncelli and Di Rosa2019).
The gross appearance, lesion distribution and histologic characteristics of Dermotheca sp. infection in ambystomatid salamanders from Illinois are generally consistent with those of other dermocystid parasites. However, these characteristics alone are inadequate for species identification within this group, and more recent classifications have relied upon molecular phylogenetics instead (Pascolini et al., Reference Pascolini, Daszak, Cunningham, Tei, Vagnetti, Bucci, Fagotti and Di Rosa2003; Feldman et al., Reference Feldman, Wimsatt and Green2005; Pereira et al., Reference Pereira, Di Rosa, Fagotti, Simoncelli, Pascolini and Mendoza2005; Raffel et al., Reference Raffel, Bommarito, Barry, Witiak and Shackelton2008; González-Hernández et al., Reference González-Hernández, Denoël, Duffus, Garner, Cunningham and Acevedo-Whitehouse2010; Scheid et al., Reference Scheid, Balczun, Dehling, Ammon and Sinsch2015; Fiegna et al., Reference Fiegna, Clarke, Shaw, Baily, Clare, Gray, Garner and Meredith2017; Borteiro et al., Reference Borteiro, Baldo, Maronna, BaÊta, Sabbag, Kolenc, Debat, Haddad, Cruz, Verdes and Ubilla2018a). These analyses have resulted in multiple species reclassifications and their placement within several new genera, with the most recent report proposing a major taxonomic restructuring to ensure monophyly within each genus (Borteiro et al., Reference Borteiro, Baldo, Maronna, BaÊta, Sabbag, Kolenc, Debat, Haddad, Cruz, Verdes and Ubilla2018a). While molecular phylogenetics has improved dermocystid taxonomic resolution compared to morphology alone, limitations persist due to the restricted number and size of available sequences and the reliance upon one gene target (18S) for phylogenetic assessment (Feldman et al., Reference Feldman, Wimsatt and Green2005). These obstacles may be overcome with the publication of more 18S sequences and/or the analysis of sequence data from additional gene targets. However, until additional data is available, interpretation of dermocystid taxonomy should be performed cautiously.
With these caveats in mind, the phylogenetic analysis conducted in the present study recovered similar dendrogram topology to that of Borteiro et al. (Reference Borteiro, Baldo, Maronna, BaÊta, Sabbag, Kolenc, Debat, Haddad, Cruz, Verdes and Ubilla2018a). Clades including amphibian Dermotheca spp., Amphibiocystidium ranae, Rhinosporidium spp., Valentines rwandae and Sphaerothecum spp. were reliably recovered with high support (>85% BS, >96% PP), however, monophyly was not fully consistent between analyses. Unlike the analysis of Borteiro et al. (Reference Borteiro, Baldo, Maronna, BaÊta, Sabbag, Kolenc, Debat, Haddad, Cruz, Verdes and Ubilla2018a), the present study recovered Dermocystidium pusula and Dermocystidium penneri in separate, well-supported clades (100% BS, 100% PP) in every dendrogram. This may indicate that these parasites belong in different genera; however, sequencing of additional gene targets would likely be necessary to improve phylogenetic resolution and confidently settle this issue. Similarly, while branch support was high for amphibian Dermotheca spp. and Dermotheca percae, resolution was poorer for other Dermotheca species infecting fish, including D. fenicum, D. salmonis and D. borealis. This illustrates the phylogenetic uncertainty within the order Dermocystida and highlights the need for additional evolutionary history research within this taxon.
The dermocystids infecting silvery salamanders and small-mouthed salamanders from Illinois were recovered in a monophyletic clade with D. viridescens and a currently un-named Dermotheca sp. from Italy with high BS and PP. The sequence variation within amphibian Dermotheca spp. is similar to or lower than that of R. seeberi and D. percae, potentially indicating that all amphibian Dermotheca spp. should be classified as D. viridescens. We did not assign our organism a specific epithet due to the aforementioned phylogenetic uncertainty associated with single-gene analyses, however, future multi-gene sequencing may confirm that the ambystomatid Dermotheca sp. from Illinois is actually D. viridescens. Dermotheca viridescens was originally identified in eastern newts from Pennsylvania, Massachusetts and West Virginia (Raffel et al., Reference Raffel, Bommarito, Barry, Witiak and Shackelton2008). Eastern newts occur in northern Illinois but are not present at the wetlands sampled in the present study. In fact, the closest known eastern newt population is approximately 64 km away in Montgomery County, Indiana (Minton Reference Minton2001). Movement of this parasite across large distances may have been facilitated by human transport of infected amphibians, as has been previously documented for ranavirus and chytridiomycosis (Jancovich et al., Reference Jancovich, Davidson, Parameswaran, Mao, Chinchar, Collins, Jacobs and Storfer2005; Picco et al., Reference Picco, Brunner and Collins2007; Picco and Collins, Reference Picco and Collins2008; Farrer et al., Reference Farrer, Weinert, Bielby, Garner, Balloux, Clare, Bosch, Cunningham, Weldon, du Preez, Anderson, Pond, Shahar-Golan, Henk and Fisher2011; Schloegel et al., Reference Schloegel, Toledo, Longcore, Greenspan, Vieira, Lee, Zhao, Wangen, Ferreira, Hipolito, Davies, Cuomo, Daszak and James2006). Alternatively, infection with Dermotheca sp. may be more widespread than currently recognized and could be naturally present in understudied amphibian populations linking affected areas. Broader surveillance efforts will be necessary to define the current spatial distribution of Dermotheca sp. and to characterize the host range of this poorly understood parasite.
The origin of Dermotheca sp. in ambystomatid salamanders from Illinois is unclear, and so is the length of time this parasite has been present in affected populations. Sampling effort for focused health assessments prior to 2017 was insufficient to detect Dermotheca sp. at a wetland prevalence of 10% or lower. Observed Dermotheca sp. prevalence in 2017 and 2018 ranged from 1 to 11%, indicating that previous health surveys could easily have missed the parasite if it was present at similarly low levels. Salamander ecology studies at the same wetlands occasionally had large enough sample sizes to detect Dermotheca sp., but the primary focus of these studies was not physical examination and cutaneous nodules may have been present but not detected (Phillips et al., Reference Phillips, Uzzell, Spolsky, Serb, Szafoni and Pollowy1997; Phillips et al., Reference Phillips, Johnson, Dreslik and Petzing2002; McAllister et al., Reference McAllister, Bursey, Crawford, Kuhns, Shaffer and Trauth2010). While it may be tempting to assume that Dermotheca sp. was suddenly introduced into affected salamander populations in 2017, it appears equally possible that this is an endemic parasite normally occurring at a low prevalence in ambystomatids. Ultimately, freedom from Dermotheca sp. infection prior to 2017 cannot be confidently assumed due to a lack of historic parasite surveillance data.
This limitation is not confined to the present study. Comprehensive dermocystid surveillance is rarely undertaken in wild amphibian populations and many existing reports depend on opportunistic observations of infected animals. While robust epidemiologic data for amphibian dermocystids is limited, a few consistent trends emerge when all available literature is considered together. Several studies demonstrate that dermocystid prevalence is highest in cooler seasons including late fall, winter and early spring (Pérez, Reference Pérez1907; Pérez, Reference Pérez1913; Guyénot and Naville, Reference Guyénot and Naville1922; Remy, Reference Remy1931; Poisson, Reference Poisson1937; Broz and Privora, Reference Broz and Privora1952; Jay and Pohley, Reference Jay and Pohley1981; Green et al., Reference Green, Converse and Schrader2002; Raffel et al., Reference Raffel, Bommarito, Barry, Witiak and Shackelton2008; Borteiro et al., Reference Borteiro, Gobel, Kolenc, Laufer, Martínez Debat and Ubilla2018b). This could be due to temperature-dependent alterations in host immunity, seasonal behavioral changes facilitating infection and/or cooler temperatures promoting parasite replication, as documented for B. dendrobatidis (Berger Reference Berger, Speare, Hines, Marantelli, Hyatt, McDonald, Skerratt, Olsen, Clarke, Gillespie, Mahony, Sheppard, Williams and Tyler2004; Kriger and Hero, Reference Kriger and Hero2007; Rowley and Alford, Reference Rowley and Alford2007). Salamanders were only sampled during February and March in the present study, potentially pairing search effort with periods of maximal dermocystid prevalence and increasing the likelihood of detecting infections. Dermocystid prevalence was not investigated during other time points because ambystomatids are fossorial and impractical to locate in large numbers outside of the breeding season. While this will likely remain a significant limitation to the study of dermocystids in ambystomatids, more thorough characterization of seasonal prevalence in other species may provide insights into the factors driving lesion development in amphibians.
Dermocystid prevalence can vary between wetlands and years, indicating that host and environmental factors likely influence lesion development (Broz and Privora, Reference Broz and Privora1952; Raffel et al., Reference Raffel, Bommarito, Barry, Witiak and Shackelton2008; Courtois et al., Reference Courtois, Cornuau, Loyau and Schmeller2013; Fiegna et al., Reference Fiegna, Clarke, Shaw, Baily, Clare, Gray, Garner and Meredith2017; Borteiro et al., Reference Borteiro, Gobel, Kolenc, Laufer, Martínez Debat and Ubilla2018b; Fagotti et al., Reference Fagotti, Rossi, Canestrelli, La Porta, Paracucchi, Lucentini, Simoncelli and Di Rosa2019). Furthermore, dermocystid prevalence can vary between sympatric species examined within a single sampling period. Scheid et al. (Reference Scheid, Balczun, Dehling, Ammon and Sinsch2015) examined 16 anuran species within the same swamp and detected V. rwandae in only two species, one of which (Hyperolius lateralis) had a much higher dermocystid prevalence (18.2%) than the other (H. viridiflavus, 0.7%). Prevalence of A. ranae was significantly higher in a population of declining R. lessonae (45.5–52.4%) than a stable population of R. esculenta (14.3–17.2%) from the same wetland complex in Italy (Pascolini et al., Reference Pascolini, Daszak, Cunningham, Tei, Vagnetti, Bucci, Fagotti and Di Rosa2003). Borteiro et al. (Reference Borteiro, Gobel, Kolenc, Laufer, Martínez Debat and Ubilla2018b) identified D. hylarum in Scinax fuscovarius, but not in any other amphibian species examined; Jay and Pohley (Reference Jay and Pohley1981) found D. penneri in A. americanus, but not in six other species from the same study site; and Broz and Privora (Reference Broz and Privora1952) identified A. ranae in R. temporaria, but not R. esculenta or any other species occupying the same habitat. The present study documented Dermotheca sp. infection in silvery salamanders and small-mouthed salamanders, but the infection was not observed in several other sympatric amphibian species including two ambystomatid salamanders (Ambystoma maculatum and Ambystoma opacum) and several anurans. This may suggest a difference in exposure or susceptibility between species, underscoring the need for additional research documenting dermocystid host range, life history characteristics, modes of transmission and pathogenesis.
Common methods for diagnosing amphibian dermocystid infections include visualization of grossly consistent cutaneous nodules, microscopic examination of tissue sections via histology or transmission electron microscopy and PCR performed on tissue samples. Many of these methods require biopsy or sacrifice of affected animals, making visual examination an attractive option. Several studies rely on visual detection of dermocystids exclusively, however, few have critically evaluated the sensitivity and specificity of this method (Courtois et al., Reference Courtois, Cornuau, Loyau and Schmeller2013; Diego-Rasilla Reference Diego-Rasilla2017; Galán and Dopereiro, Reference Galán and Dopereiro2017; Borteiro et al., Reference Borteiro, Gobel, Kolenc, Laufer, Martínez Debat and Ubilla2018b). Raffel et al. (Reference Raffel, Bommarito, Barry, Witiak and Shackelton2008) dissected 106 eastern newts and found that 12 newts with visible cutaneous D. viridescens lesions had consistent sporangia in the skin on histologic examination. Four of these animals also had sporangia in the liver, while three newts had hepatic sporangia with no evidence of cutaneous disease. Overall sensitivity for visual examination in this report was 0.8 (95% CI: 0.51–0.95) and specificity was 1 (95% CI: 0.95–1). González-Hernández et al. (Reference González-Hernández, Denoël, Duffus, Garner, Cunningham and Acevedo-Whitehouse2010) dissected 21 palmate newts and detected D. pusula DNA in skin biopsies from all animals with visibly apparent cutaneous nodules (N = 10) and one newt which had histologic skin changes consistent with recent dermocystid infection, but no cutaneous nodules. In this case, sensitivity of visual examination was 0.91 (95% CI: 0.57–1) and specificity was 1 (95% CI: 0.66–1). Fiegna et al. (Reference Fiegna, Clarke, Shaw, Baily, Clare, Gray, Garner and Meredith2017) dissected 30 palmate newts and found D. pusula sporangia in 18 animals with and two animals without visually apparent cutaneous lesions. Sensitivity for visual examination in this report was 0.9 (95% CI: 0.67–0.98) and specificity was 1 (95% CI: 0.66–1). Amphibian dermocystid infections produce very characteristic cutaneous lesions, and visual identification of these lesions has high specificity for dermocystid detection. However, animals with internal dermocystid infections and those with early or resolving lesions may be misclassified as dermocystid negative using visual examination alone. The prevalence estimates obtained in the present study relied heavily upon visual examination and may be underestimates of true Dermotheca sp. prevalence in silvery salamanders.
PCR screening of skin swabs is one non-invasive diagnostic option which may reduce false-negative rates in dermocystid surveys. González-Hernández et al. (Reference González-Hernández, Denoël, Duffus, Garner, Cunningham and Acevedo-Whitehouse2010) found that skin swabs from 24 newts with cutaneous dermocystid lesions were PCR positive for D. pusula, while swabs from 16 animals with no gross lesions were PCR negative. Additional studies are needed to determine the sensitivity and specificity of this technique, however, this may provide a practical means of dermocystid detection to augment existing methodologies. Non-invasive antemortem diagnostics are especially useful for threatened and endangered species, where invasive testing is not feasible or ethical in large numbers of animals.
From a disease ecology perspective, ichthyosporeans share a suite of biological characteristics with parasites capable of causing host extinction, including low host specificity, the potential for high virulence, presence of a free-living infectious stage and the ability to survive prolonged adverse environmental conditions (Mendoza et al., Reference Mendoza, Taylor and Ajello2002; Fisher et al., Reference Fisher, Henk, Briggs, Brownstein, Madoff, McCraw and Gurr2012; Rowley et al., Reference Rowley, Gleason, Andreou, Marshall, Lilje and Goslan2013). Unfortunately, studies documenting the population level impacts of dermocystid infections are extremely limited (González-Hernández et al., Reference González-Hernández, Denoël, Duffus, Garner, Cunningham and Acevedo-Whitehouse2010; Raffel et al., Reference Raffel, Bommarito, Barry, Witiak and Shackelton2008; Fiegna et al., Reference Fiegna, Clarke, Shaw, Baily, Clare, Gray, Garner and Meredith2017). As a result, the real-world importance of these parasites for amphibian conservation is hard to determine, and likely context-dependent. Long-term research on the Pelophylax esculentus complex in central Italy documented steep population declines in P. lessonae with severe A. ranae infections, while sympatric P. esculentus with lower dermocystid burdens remained stable (Simoncelli et al., Reference Simoncelli, Fagotti, Dall'Olio, Vagnetti, Pascolini and Di Rosa2005; Fagotti et al., Reference Fagotti, Morosi, Di Rosa, Clarioni, Simoncelli, Pascolini, Pellegrino, Guex and Hotz2005; Di Rosa et al., Reference Di Rosa, Simoncelli, Fagotti and Pascolini2007). Extreme weather events, elevated tissue organochlorine levels and chytridiomycosis were also identified during these declines, and it is difficult to disentangle the complex interaction between disease and other anthropogenic and climactic stressors in this case (Simoncelli et al., Reference Simoncelli, Fagotti, Dall'Olio, Vagnetti, Pascolini and Di Rosa2005; Fagotti et al., Reference Fagotti, Morosi, Di Rosa, Clarioni, Simoncelli, Pascolini, Pellegrino, Guex and Hotz2005; Di Rosa et al., Reference Di Rosa, Simoncelli, Fagotti and Pascolini2007). If A. ranae was not the proximate cause of the P. lessonae declines, then monitoring dermocystid prevalence may provide information on the overall wellness of infected populations, rising as health status deteriorates secondary to multiple stressors. However, a 7-year study in Italian stream frogs failed to demonstrate differences in weight, survival probability, or capture probability between Dermotheca sp. infected and uninfected frogs, despite high parasite prevalence (>50% infected) (Fagotti et al., Reference Fagotti, Rossi, Canestrelli, La Porta, Paracucchi, Lucentini, Simoncelli and Di Rosa2019). This outcome disparity demonstrates the importance of characterizing natural dermocystid infection dynamics and identifying pathogen, host and environmental factors promoting severe disease sequelae.
In the present study, salamander health status did not differ based on the presence of Dermotheca sp. infection, however, all animals were released immediately after physical examination and long-term survival and fitness consequences are unknown. Furthermore, only animals healthy enough to attempt breeding were evaluated, and it is possible that more heavily affected salamanders remained in burrows and were not examined. The cross-sectional nature of this study and the possibility of sampling bias ultimately makes it difficult to infer the effects of Dermotheca sp. on salamander health. The importance of other amphibian species for the maintenance of Dermotheca sp. in Vermilion County wetlands is also unknown. Small-mouthed salamanders are common at all study sites and could potentially serve as a reservoir for Dermotheca sp. infection in silvery salamanders. This is potentially concerning, as the presence of an abundant reservoir host can promote high levels of infection in rarer species, releasing pathogens from density dependence and contributing to differentially large disease impacts (McCallum and Dobson, Reference McCallum and Dobson1995). This has been demonstrated for dermocystids infecting fish (Gozlan et al., Reference Gozlan, St-Hilaire, Feist, Martin and Kent2005; Hershberger et al., Reference Hershberger, van der Leeuw, Gregg, Grady, Lujan, Gutenberger, Purcell, Woodson, Winton and Parsley2010; Andreou et al., Reference Andreou, Arkush, Guégan and Gozlan2012). Future research should incorporate dermocystid surveillance in sympatric species to determine relative host susceptibility and assess the potential for reservoir host development. Longitudinal evaluation of infected salamanders in laboratory or mesocosm settings will be necessary to determine the impacts of Dermotheca sp. on individual health and survival. Continued population monitoring is needed to evaluate whether dermocystid parasites threaten silvery salamander conservation efforts.
Emerging infectious diseases can pose a significant threat to amphibians, especially species of conservation concern. Dermocystid parasites can affect individual survival and have the potential to impact population stability alone or in conjunction with other stressors. The identification of Dermotheca sp. in a state-endangered salamander is concerning and further robust studies are needed to determine its effects on individual and population health. The present study provides useful baseline information on morphology, pathology, phylogeny and prevalence that can be used to direct future research efforts and to monitor changes in the affected populations over time. Understanding the health of free-living amphibians may promote more effective management strategies and ultimately improve conservation outcomes.
Acknowledgements
We thank Andrew D. Sweet, PhD for guidance in phylogenetic analyses. We thank Connor T. Dempsey, Jaclyn M. Adams, Rachel Neubauer and Victoria Alessa Laserna for their assistance with collecting and sampling salamanders. We thank Jason P. Ross, John Warner, Allison Sieia, Eleanor Moen and Linsday Hseih for constructing the drift fences around each wetland.
Financial support
This work was supported by Illinois State Wildlife Grants (grant numbers T-104-R-1 and T-108-R-1).
Ethical standards
All animal handling and procedures were approved by the University of Illinois Institutional Animal Care and Use Committee (protocol #15180) and Illinois Endangered and Threatened Species Permits 05-11S and 1042.
Conflicts of interest
None