Introduction
Digenean trematodes are a group of parasitic flatworms, also known as flukes, which parasitize wild and domestic animals, as well as humans (Schell, Reference Schell1970). Many digenean trematode species have been reported to cause disease in humans from several countries in Southeast Asia (Chai et al., Reference Chai, Shin, Lee and Rim2009; Hung et al., Reference Hung, Madsen and Fried2013; Chai, Reference Chai2019). In fact, human infection by the liver fluke Opisthorchis viverrini is a serious public health problem in Thailand and Lao PDR, where this fluke has demonstrated a causative relationship with cholangiocarcinoma or bile duct cancer (Sripa et al., Reference Sripa, Bethony, Sithithaworn, Kaewkes, Mairiang, Loukas, Mulvenna, Laha, Hotez and Brindley2011; Suwannatrai et al., Reference Suwannatrai, Saichua, Haswell, Sripa and Brindley2018). In addition to its human impacts, comparatively high echinostome trematode infection rates have been observed in free-grazing ducks from northern, central and northeastern Thailand, where these domestic ducks play an important role in the rural economy with regards to egg and meat production (Saijuntha et al., Reference Saijuntha, Duenngai and Tantrawatpan2013). Digenean trematodes have a complex life cycle that usually requires at least two animals as hosts: an invertebrate intermediate host for asexual reproduction of larval trematodes and a vertebrate definitive host for sexual reproduction of adult trematodes (Poulin and Cribb, Reference Poulin and Cribb2002). In brief, trematode eggs are released from adult trematodes in the definitive host. Miracidium-stage larvae hatch from the eggs and find a suitable first-intermediate host, usually snail faunas. Inside this host, the miracidium larvae develop and grow; larvae in sporocyst, redia and cercarial stages are commonly found in the first-intermediate host. Cercaria-stage larvae emerge from the first-intermediate host and swim in search of an appropriate second-intermediate host. In the second-intermediate host the cercaria develop into the metacercarial stage. The metacercaria-stage larvae inside the second-intermediate host must be ingested by an appropriate definitive host before they can develop into adults and complete their life cycle. Snail faunas play important roles as first-intermediate hosts in the trematode life cycle, harbouring miracidium-stage larvae and amplifying the sporocyst, redia and cercaria trematode stages. In addition, some snail species can serve as second-intermediate hosts and harbour metacercaria-stage trematodes.
Two major groups of snails, including prosobranchs and pulmonates, are common intermediate hosts of digenean trematodes. Prosobranchs are gill-breathing snails; they are commonly dioecious and their sexes are mostly inconvenient to be externally discriminated. Growth and longevity of the prosobranchs are highly variable among the different species. Their growth is not uniform during the year. Their longevity is quite long when compared with the pulmonates, ranging from 3 to 7 years and reaching to 20 years for some species (Fretter and Graham, Reference Fretter and Graham1962). About the pulmonates, they are a group of air-breathing snails and generally hermaphrodites with self-fertilization. However, both self-fertilization and cross-fertilization can occur in some freshwater pulmonates, i.e. lymnaeids, planorbids, physids and ancylid. Growth of freshwater pulmonates commonly decreases after their achievement of sexual maturity. For longevity, most of freshwater pulmonates inhabit about 1 year and die after their spawning season. From the biology of these snails, Esch and Fernandez (Reference Esch and Fernandez1994) pointed out that the differences of trematode community between prosobranchs and pulmonates could occur due to the marked differences of reproductive biology, growth characteristics and longevity of these two snail groups. Some freshwater snails determined as invasive species are capable of harbouring trematode larvae. In broadly, an invader Physella acuta (=Physa acuta) originates in North America (Dillon et al., Reference Dillon, Wethington, Rhett and Smith2002; Lydeard et al., Reference Lydeard, Campbell and Golz2016) and has been widely noted as a globally invasive freshwater snail from all continents, excluding Antarctica (Wethington and Lydeard, Reference Wethington and Lydeard2007; Bousset et al., Reference Bousset, Pointier, David and Jarne2014). This snail species is known to become a dominant species by replacing native snails with a short period of time (Dobson, Reference Dobson2004). In addition to acting as a first-intermediate host snail of several trematode cercariae reported in a few previous studies (Toledo et al., Reference Toledo, Muñoz-Antolí, Pérez and Esteban1998; Kraus et al., Reference Kraus, Brant and Adema2014; Ebbs et al., Reference Ebbs, Loker and Brant2018), the P. acuta was suggested that they may serve as a decoy host of native trematode species (Chipev, Reference Chipev1993; Muñoz-Antoli et al., Reference Muñoz-Antoli, Trelis, Gozalbo, Toledo, Haberl and Esteban2003). In Thailand, the P. acuta, is usually found in various habitats, such as assemblage in the border or attaching on aquatic vegetations of drainage ditches, ponds and canals, but the infective evidence of trematode larvae in this snail is still undiscovered. Also, the golden apple snail, Pomacea canaliculata, is an important invader in Thailand (Vidthayanon, Reference Vidthayanon, Bartley, Bhujel, Funge-Smith, Olin and Phillips2005) and has been never reported for cercarial infection.
Previous studies have reported approximately 30 species of eight freshwater snail families infected by trematode cercariae in Thailand: Ampullariidae, Bithyniidae, Lymnaeidae, Nassariidae, Pachynidae, Planorbidae, Thiaridae and Viviparidae (Dechruksa et al., Reference Dechruksa, Krailas, Ukong, Inkapatanakul and Koonchornboon2007; Ukong et al., Reference Ukong, Krailas, Dangprasert and Channgarm2007; Chuboon and Wongsawad, Reference Chuboon and Wongsawad2009; Chontananarth and Wongsawad, Reference Chontananarth and Wongsawad2013; Krailas et al., Reference Krailas, Namchote, Koonchornboon, Dechruksa and Boonmekam2014; Kulsantiwong et al., Reference Kulsantiwong, Prasopdee, Piratae, Khampoosa, Thammasiri, Suwannatrai, Boonmars, Viyanant, Ruangsitichai, Tarbsripair and Tesana2015; Namsanor et al., Reference Namsanor, Sithithaworn, Kopolrat, Kiatsopit, Pitaksakulrat, Tesana, Andrews and Petney2015; Anucherngchai et al., Reference Anucherngchai, Tejangkura and Chontananarth2016, Reference Anucherngchai, Tejangkura and Chontananarth2017; Veeravechsukij et al., Reference Veeravechsukij, Namchote, Neiber, Glaubrecht and Krailas2018), these studies have revealed 15 morphological types of trematode cercariae, such as amphistome, echinostome, gymnocephalous, parapleurolophocercous, pleurolophocercous and xiphidiocercariae, which belong to families of human intestinal flukes (Chai et al., Reference Chai, Shin, Lee and Rim2009; Hung et al., Reference Hung, Madsen and Fried2013; Chai, Reference Chai2019). Moreover, nine families of brackish-water snails have been observed to carry cercaria-stage digenean trematodes (Sri-Aroon et al., Reference Sri-Aroon, Lohachit and Harada2004). Interestingly, cercarial infection in natural freshwater snails has been reported in the central region of Thailand; for instance, Anucherngchai et al. (Reference Anucherngchai, Tejangkura and Chontananarth2016) reported nine cercaria types in eight species of infected snails from Chao-Phraya basin, in which thiarid snails exhibited the highest infection rate, whereas the assassin snail Anentome helena presented no infections. Meanwhile, seven types of trematode cercariae were observed in 12 snail species by Chontananarth et al. (Reference Chontananarth, Tejangkura, Wetchasart and Chimburut2017) in Nakhon Nayok Province. However, comprehensive investigations of cercarial infections in snails from the Bangkok area are rare.
Bangkok, the highly urbanized capital of Thailand, is in a central plain divided into two by the Chao Phraya River. Bangkok is nearly entirely penetrated by canals that diverge from the river and lead to widely dispersed and varied aquatic faunas. The canals benefit the people greatly, enabling agriculture delivery and drainage, local aquaculture and fisheries, open-field livestock husbandry (particularly ducks), water traffic, floating tourist markets, etc. These anthropogenic activities indicate high contact between humans and water resources as well as the completeness of host components. Therefore, the success of trematode transmission from aquatic animals to various vertebrate animals, including humans, is highly probable. Moreover, the migration of humans and especially labourers from provincial areas into Bangkok, likely provides a pathway for the spread of trematodes. Investigation of the diversity, distributions, infection rates and host ranges of trematode faunas is important to our understanding of the general biology of trematodes and our ability to prevent and control trematode transmission. In this regard, Bangkok is an interesting area in which to study larval trematode infection in aquatic animals, particularly freshwater snails that can distribute and exist in a variety of habitats. Throughout Bangkok, water resources comprising ponds and canals have been identified as sampling localities for snails harbouring cercaria-stage digenean trematodes. Our results elucidate the prevalence of cercarial infections in these snail intermediate hosts, the morphology of each cercaria and the phylogenetic relationship between these trematode cercariae and their related digenean trematodes.
Materials and methods
Snail collection and parasitic investigation
Freshwater snails were randomly collected from natural water resources, canals and ponds in almost all areas of Bangkok, comprising 59 sampling sites in 32 districts (Fig. 1). The global positioning system coordinates of all sampling sites were recorded and subsequently identified in a study area map using QGIS software version 3.6.1 (QGIS Development Team, 2020) and the WGS84 coordinate system. Snail specimens were collected by hand and net during May 2018 and March 2019. Collection from each sampling site took approximately 15–20 min based on the counts per unit of time-sampling method (Olivier and Schneiderman, Reference Olivier and Schneiderman1956). The collected snails were temporarily reared in 2-L plastic boxes, with an appropriate proportion per box during the cercarial infection observation period. Morphological characters of the snail shells were used to identify each species level, as described by Brandt (Reference Brandt1974) and Upatham et al. (Reference Upatham, Sormani, Kitikoon, Lohachit and Burch1983). Cercarial infection in these freshwater snails was investigated using a crushing technique (Caron et al., Reference Caron, Rondelaud and Losson2008). In brief, the snail body was removed from the shell and depressed within a pair of Petri dishes. Thereafter, the cercaria larvae were observed under a stereomicroscope at high magnification. The numbers of examined snails and infected snails were recorded and used to calculate the prevalence (%) of infection by dividing the number of infected snails by the number of examined snails and multiplying the resulting number by 100.

Fig. 1. Map of snail sampling sites in Bangkok, Thailand.
Morphological study
Using a glass dropper, live cercariae were collected from each crushed snail body and placed in a drop of water on a microscope slide covered with a coverslip. The cercariae were morphologically examined from stained and fixed specimens. Neutral red solution with 0.5% and neutral-buffered formalin with 10% were used as the staining and fixative agents, respectively. The stained and fixed cercariae were thoroughly examined under a compound light microscope for their morphological characteristics and individually photographed using an Olympus BX51 compound light microscope with a DP70 camera (Olympus Corporation, Japan). Following Schell (Reference Schell1970) and Frandsen and Christensen (Reference Frandsen and Christensen1984), types of cercariae were identified based on their morphological characters. The family level of each cercaria type was also morphologically determined. Several cercaria morphological parameters were measured in the photomicrographs using an ImageJ program (Abramoff et al., Reference Abramoff, Magalhaes and Ram2004). All parameters were measured as minimum-to-maximum range values in micrometres (μm), and they are followed by their arithmetic means in parentheses.
Extraction, amplification, purification and sequencing of cercarial DNA
Fresh specimens of cercariae were preserved in 1.5-mL microcentrifuge tubes containing absolute ethanol. The preserved cercariae were washed by centrifuging twice in ultrapure water at 10 000 × g for 1 min. The genomic DNA of each cercaria type was extracted using a GF-1 Tissue DNA Extraction Kit (Vivantis, Malaysia) following the protocol of the manufacturer. The extracted cercaria DNA was amplified for the internal transcribed spacer 2 (ITS2) region using a polymerase chain reaction (PCR) methodology and the universal primers, as specified by Barber et al. (Reference Barber, Mkoji and Loker2000) and Chontananarth et al. (Reference Chontananarth, Tejangkura, Wetchasart and Chimburut2017), including ITS3 (5′-GCA TCG ATG AAG AAC GCA GC-3′) and ITS4 (5′-TCC TCC GCT TAT TGA TAT GC-3′) for forward and reverse primers, respectively. The PCR amplification of the cercaria ITS2 fragment employed a total reaction volume of 25 μL, comprising 1× PCR buffer, 2 mm MgCl2, 0.4-μ m of each primer, 100-μ m dNTP mixture, 2-units μL−1 Taq polymerase and 4 μL of the cercarial DNA template. Using a thermal cycler (Mastercycler Pro, Eppendorf, Germany), the PCR conditions were as follows: 94 °C for 5 min of predenaturation, followed by 35 cycles of denaturation, annealing and extension at 94 °C for 1 min, 56 °C for 1 min and 72 °C for 30 s, respectively. A final extension step was set at 72 °C for 10 min. The expected ITS2 fragment of cercarial DNA from each PCR product was separated by gel electrophoresis at 50 volts for 60 min using a 1.5% agarose gel stained with SYBR safe (Invitrogen). Gel visualization was accomplished using the Gel Doc™ XR+ imaging system (Bio-Rad Laboratories, USA). Purification and sequencing were performed by Macrogen, Korea. The same primer set used for PCR was used to sequence the ITS2 amplicons via the Sanger (chain-termination) method. The novel ITS2 sequences of trematode cercariae obtained in the current study were submitted to the GenBank database and their accession numbers are provided in Table 1.
Table 1. ITS2 sequence data of cercariae obtained in this study and trematodes acquired from GenBank database for the phylogenetic analyses

Phylogenetic tree analyses
The sequence data obtained for the ITS2 regions from forward and reverse primers were trimmed and then assembled as contiguous sequences using the CAP3 sequence assembly programme (Huang and Madan, Reference Huang and Madan1999). The megablast algorithm in the standard nucleotide basic local alignment search tool (BLAST) from the NCBI database was used to confirm the presence of the ITS2 region in the sequence data. For phylogenetic analysis, 17 novel sequences of cercaria samples obtained in the current study and 44 sequences of several families of trematodes acquired from the GenBank database (Table 1) were used to construct a phylogenetic tree. A sequence of Aspidogastrea, Aspidogaster ijimai, was placed as an outgroup. Before construction, datasets of all sequences were aligned using ClustalW (Thompson et al., Reference Thompson, Higgins and Gibson1994). The best-fitting model of nucleotide substitution based on Akaike information criterion was analysed using jModelTest version 2.1.10 (Guindon and Gascuel, Reference Guindon and Gascuel2003; Darriba et al., Reference Darriba, Taboada, Doallo and Posada2012). The phylogenetic tree was constructed using Bayesian inference (BI) analyses based on the general time reversible substitution model with gamma-distributed rate variation across sites and a proportion of invariable sites (GTR + G + I). MrBayes version 3.2.7 software (Ronquist et al., Reference Ronquist, Teslenko, van der Mark, Ayres, Darling, Höhna, Larget, Liu, Suchard and Huelsenbeck2012) was utilized to implement the BI analyses with 10 million generations of Markov chain Monte Carlo and to sample trees every 1000 generations; the first 2500 trees (25%) were discarded as the burn-in phase. Additionally, pairwise genetic distance was analysed using MEGA X software (Kumar et al., Reference Kumar, Stecher, Li, Knyaz and Tamura2018) to estimate evolutionary divergence between sequences. A prior assumption of a 5% cut-off in sequence divergence was applied for species delimitation (Gordy and Hanington, Reference Gordy and Hanington2019).
Results
Overall cercaria infections in freshwater snails
We examined 15 621 individual freshwater snails collected from natural ponds and canals in the Bangkok area (Fig. 1) during May 2018 and March 2019 and determined infections of the cercarial stage. Of these, 517 snails were found to be infected with trematode cercariae, amounting to a 3.31% overall prevalence of infection (Table 2). The infected snails were classified into 11 species/subspecies (Fig. 2), including seven prosobranch snails (A. helena, Bithynia siamensis siamensis, Filopaludina martensi martensi, Filopaludina sumatrensis polygramma, Melanoides tuberculata, Tarebia granifera and Thiara scabra) and four hygrophila snails (Gyraulus siamensis, Indoplanorbis exustus, P. acuta and Radix auricularia). The B. siamensis siamensis snail exhibited the highest prevalence of infection (16.16%), whereas the P. acuta snail exhibited the lowest prevalence (0.08%). The most diverse infectivity of snails was observed in the B. siamensis siamensis snail, which was infected with seven morphological types of cercariae. The next highest diversities of infection were observed in F. sumatrensis polygramma and R. auricularia snails, which were infected with five and four cercaria types, respectively. However, no trematode cercaria infections were observed in nine species/subspecies of the remaining snails (Table 2), including eight prosobranch snails (Cyclotropis carinata, F. martensi cambodiensis, F. sumatrensis speciosa, F. sumatrensis peninsularis, Idiopoma umbilicata, P. canaliculata, Sermyla riqueti and Sulcospira housei) and a hygrophila snail (Austropeplea viridis). A total of eight types of trematode cercaria morphologies were found in the entire collection of infected snails (Fig. 3): brevifurcate-pharyngeate-clinostomatoid, echinostome, monostome, mutabile, parapleurolophocercous, strigea, vivax and xiphidiocercariae. Cercaria infection was highest in the mutabile (1.86%). Infections with the following five cercariae were low (≤0.1% prevalence of infection): monostome, brevifurcate-pharyngeate-clinostomatoid, parapleurolophocercous, strigea and vivax. Infections with xiphidiocercariae were frequently observed, such that 8 of all 11 infected snail species were observed with this cercaria. The next highest frequencies of infections were observed in echinostome and mutabile cercariae, which were found in seven and six infected snail species, respectively.
Table 2. Prevalence of cercarial infection in examined freshwater snails from Bangkok, Thailand with the numbers of examined snails and infected snails

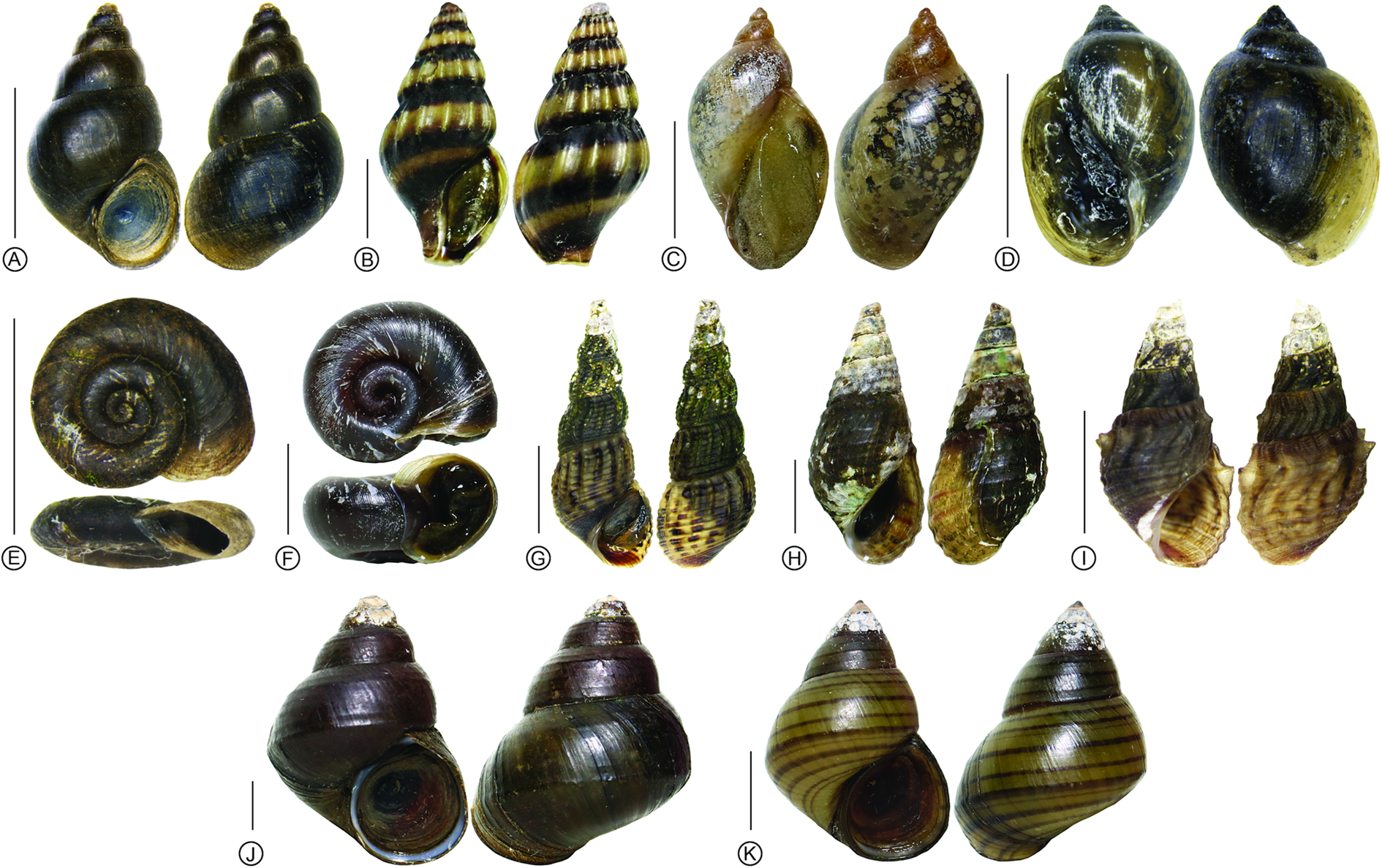
Fig. 2. General morphology of infected freshwater snails in 11 species/subspecies, including (A) Bithynia siamensis siamensis, (B) Anentome helena, (C) Radix auricularia, (D) Physella acuta, (E) Gyraulus siamensis, (F) Indoplanorbis exustus, (G) Melanoides tuberculata, (H) Tarebia granifera, (I) Thiara scabra, (J) Filopaludina martensi martensi and (K) Filopaludina sumatrensis polygramma. Scale bars: 5 mm.

Fig. 3. General morphological photomicrographs of eight trematode cercariae infecting in freshwater snail intermediate hosts, including (A) brevifurcate-pharyngeate-clinostomatoid, (B) echinostome, (C) monostome, (D) mutabile, (E) parapleurolophocercous, (F) strigea, (G) vivax and (H) xiphidiocercariae. The character abbreviations are as follows: C, caecum; CS, collar spines; DMF, dorso-median finfold; DVF, dorso-ventral finfold; E, oesophagus; EB, excretory bladder; ES, eyespots; LF, lateral finfold; MCT, main collecting tube; OS, oral sucker; P, pharynx; PG, penetration grands; S, stylet; T, tail; TF, tail furca; TS, tail stem; VS, ventral sucker. Scale bars: 100 μm.
Regarding concurrent infections (Table 3), 10 individuals of the B. siamensis siamensis snail were found to harbour two or three types of cercariae at the same time (double or triple infections). Double infection was found in eight B. siamensis siamensis snails: four individuals harboured a pair of xiphidiocercariae and mutabile types; three individuals harboured a pair of monostome and mutabile types; and one individual harboured a pair of mutabile and parapleurolophocercous types. Meanwhile, two B. siamensis siamensis individuals exhibited triple infections (one snail with xiphidiocercariae, monostome and mutabile; and another snail with xiphidiocercariae, vivax and mutabile).
Table 3. Double and triple cercarial infections of the snail B. siamensis siamensis

General morphology and infection of cercariae
The general morphological characters, including measured characters, of eight cercaria types were recorded from stained and unstained specimens. Several characters, such as oral and ventral suckers, stylet, eyespots, collar spines, tail and finfold, were utilized to morphologically classify these eight cercaria types. Snail intermediate hosts and infection rates of each cercaria, including overall and per a snail host, were also mentioned in the descriptions.
Type 1. Brevifurcate-pharyngeate-clinostomatoid cercaria (Fig. 3A)
The body shape of brevifurcate-pharyngeate-clinostomatoid cercaria is oval-elongated. The oral sucker is spherical and located at the terminal anterior part of the body. A few characters – pharynx, oesophagus and intestinal caeca – are quite small and difficult to thoroughly observe. A pair of eyespots is situated at one-third of the body measured from the proximal end. Penetration glands are located in the middle of body. The ventral sucker is vestigial. A small excretory bladder is located at the posterior end of the body. Externally, a dorso-median finfold is situated in the longitudinal middle of the body. The tail is substantially longer than the body and is bifurcated into two furcae. Each furca is obviously shorter than the tail stem and is attached with a lateral finfold at the furca margin. All measured characters are listed in Table 4. The following three species of snails were infected with brevifurcate-pharyngeate-clinostomatoid cercaria with a 0.06% overall prevalence of infection: B. siamensis siamensis (0.23%), R. auricularia (0.41%) and I. exustus (0.16%) (Table 2). Among these three snail species, no different characters of the cercaria morphology were observed.
Table 4. Dimensions of measured morphological characters of cercariae larvae (in μm) are represented as minimum and maximum (range) values followed by arithmetic mean within parentheses

Number of cercaria individuals measured is shown within parentheses after each cercaria type.
Type 2. Echinostome cercaria (Fig. 3B)
The body of echinostome cercaria is often ovate-shaped. The oral sucker lacks a stylet and is circular-shaped, surrounded by collar spines and located at the subterminal of the anterior body. The pharynx is vertically elongated in shape. The oesophagus is long, divided into bifurcate caeca near the middle of the body (before the ventral sucker) and filled with fine brownish granules. The bifurcate caeca were prominently observed along both lateral sides, from the middle of the body to the posterior end. Main collecting tubes are distinctively located along both sides of the body from the pharynx to the ventral sucker and contain large, blackish-bordered granules. The ventral sucker is significantly larger than the oral sucker and is spherical in shape, located at approximately two-thirds of the body, measured from the anterior end. The excretory bladder is horizontally ovate and located at the posterior end of the body. The tail is tapered and is longer than the body. The measured characters are shown in Table 4. Echinostome cercaria was found in the following snail species with an overall infection prevalence of 0.52%: B. siamensis siamensis (0.23%), A. helena (1.20%), R. auricularia (2.26%), G. siamensis (0.23%), I. exustus (7.23%), T. granifera (0.06%) and F. sumatrensis polygramma (0.06%) (Table 2). However, the echinostome cercaria was found to be morphologically similar among their snail hosts.
Type 3. Monostome cercaria (Fig. 3C)
The monostome cercaria is ovate in body shape and contains scattered brownish pigments inside the body. The oral sucker is spherical in shape and situated in the subterminal region of the anterior body. The pharynx size is small, and the oesophagus separates into two long branches of intestinal caeca that reach the excretory bladder. A pair of globular/ovate eyespots is present near the level of the pharynx. The ventral sucker is absent. The main collecting tubes are fused after the oral sucker and terminate at the excretory bladder, filling it with excretory granules. The excretory bladder is located at the posterior end of the body. The tail is unforked, thick and shorter than the body. Several characters of this cercaria were measured, as shown in Table 4. Only one snail species, B. siamensis siamensis (0.46%), was infected with monostome cercaria, and the overall prevalence of infection was 0.06% (Table 2).
Type 4. Mutabile cercaria (Fig. 3D)
The mutabile cercaria is fusiform in shape. The oral sucker is situated in the subterminal region of the anterior part of the body, whereas the ventral sucker is located in the middle of the body. The oral and ventral suckers are equal or slightly different sizes. A prepharynx was not observed. A large, globular-shaped pharynx is present and located near the oral sucker. The oesophagus is bifurcated into two intestinal caeca and extends two-thirds the length of the body, measured from the front. Main collecting tubes filled with fine brownish granules extend to the excretory bladder located at the posterior end of the body. We did not observe a tail in this cercaria. The measured characters are reported in Table 4. Mutabile cercaria infections were observed at a 1.86% overall infection prevalence in six freshwater snail species: B. siamensis siamensis (12.89%), A. helena (1.51%), G. siamensis (0.23%), T. granifera (0.06%), F. martensi martensi (0.07%) and F. sumatrensis polygramma (0.03%) (Table 2). The mutabile cercaria is not different in their morphological characters among six species of the snail hosts.
Type 5. Parapleurolophocercous cercaria (Fig. 3E)
Parapleurolophocercous cercaria are oval to elongated in shape. The oral sucker is rounded and located in the subterminal region of the anterior part of the body. The oral sucker is distinctively large compared to the ventral sucker. The pharynx is small and ovate and situated between two eyespots. The eyespots vary in shape, ranging from globular to elliptical to polygonal. An oesophagus was not observed. The posterior two-thirds of the body is filled with seven pairs of penetration glands arranged along both lateral sides and terminating at the posterior end of the body. The ventral sucker is small, circular and situated near three-thirds the length of the body, measured from the anterior end. A large excretory bladder filled with brownish granules is located at the posterior end of the body. The tail is not forked and is longer than the body. The processes of lateral finfolds occurred at one-third of the body length measured from the proximal end of the tail, whereas the dorso-ventral finfold attached at two-thirds of the body length. The measured characters are shown in Table 4. Three freshwater snails, B. siamensis siamensis (0.05%), M. tuberculata (2.36%) and T. scabra (0.91%), were found to harbour parapleurolophocercous cercaria with 0.04% overall prevalence of infection (Table 2). No different morphology of parapleurolophocercous cercaria was observed in these three snail hosts.
Type 6. Strigea cercaria (Fig. 3F)
The bodies of strigea cercaria are ovate to elongated in shape. The oral sucker, which is globular shaped and situated at the anterior terminal end of the body, seems to be equal or slightly smaller in size compared to the ventral sucker. The prepharynx is present and extends over a short distance compared to the oesophagus. The pharynx is roundish in shape and small. The oesophagus is longer than the pharynx and bifurcated into two intestinal caeca. The caeca are distinctly observed and sinuously extend to the posterior end of the body. The ventral sucker is well developed and located in the middle of the body. Penetration glands are present near and posterior to the ventral sucker. The excretory bladder is located at the posterior end of the body. The tail is divided into two furcae and is longer than the body. The furcae seem to be equal in length or longer than the tail stem. The measured characters of strigea cercaria are shown in Table 4. Strigea cercaria was found to infect two snail species, R. auricularia (0.10%) and F. sumatrensis polygramma (0.06%), with a 0.02% overall prevalence of infection (Table 2). The morphological characters of strigea cercaria between these two snail species are not different.
Type 7. Vivax cercaria (Fig. 3G)
The body of vivax cercaria is ovate in shape. A spherically shaped oral sucker is located at the anterior end of the body. The pharynx is small in size and almost globular shaped. The oesophagus is longer than the pharynx. Large bifurcate intestinal caeca directly connect the oesophagus to the approximate posterior end of the body. The ventral sucker is vestigial and smaller than the oral sucker and exists at around two-thirds of the body length, as measured from the front. The excretory bladder is located at the posterior end of the body. The tail of the cercaria is forked and longer than the body. The tail furcae are shorter than the tail stem and are attached via a lateral finfold in the furca margin. Excretory pores are located at ends of the furcae. The characters measured for this cercaria are shown in Table 4. Two snails, B. siamensis siamensis (0.41%) and F. sumatrensis polygramma (0.23%), were found to be infected with this cercaria with 0.11% overall prevalence infection (Table 2). The vivax cercaria observed between these two snail species is similar in morphological characters.
Type 8. Xiphidiocercariae (Fig. 3H)
Xiphidiocercariae are oval-elongated in shape. The oral sucker is round and located in the subterminal region of the anterior part of the body. A stylet was found within the centre of the oral sucker. The pharynx is quite large and globular in shape. The oesophagus is short, slender and divided into two intestinal caeca that extend a relatively short distance. The ventral sucker is approximately equal in size to the oral sucker and is situated in the middle of the body. Penetration glands are dense near the pharynx and the ventral sucker. The excretory bladder is thin walled and located near the posterior end of the body. The tail is tapered and shorter than the body in length. The characters measured are reported in Table 4. The following eight snail species were infected with this cercaria with a 0.71% overall prevalence of infection: B. siamensis siamensis (2.44%), R. auricularia (0.72%), P. acuta (0.08%), G. siamensis (0.90%), M. tuberculata (0.94%), T. granifera (0.06%), F. martensi martensi (1.01%) and F. sumatrensis polygramma (0.43%) (Table 2). Xiphidiocercariae are morphologically similar among these snail hosts investigated.
Phylogenetic analyses and molecular identification
Seventeen samples of eight morphological types of cercariae were successfully amplified and sequenced for ITS2. A total of 17 novel sequences of the ITS2 region (approximately 350–560 base pairs) were identified, and 44 trematode sequences were acquired from the GenBank database for phylogenetic assessment using BI analyses. Regarding the general topology of the phylogram (Fig. 4), a monophyletic group of digenean trematodes was revealed by applying the Aspidogastrea, A. ijimai, as an outgroup. Seventeen novel sequences of eight current cercariae types were arranged into at least nine different families of digenean trematodes. For xiphidiocercariae, three of the four samples were clearly clustered into an individual family with high posterior probability supports. The 3TLC sample (from the B. siamensis siamensis snail) was found to be intimately related to the family Lecithodendriidae (clade A), and a clade of 6.1BB1 and 30.1NK4 (from the R. auricularia snail) was arranged as the sister clade of a clade of the sequences in family Telorchiidae (clade C). However, the sample 47.1PCR1 (from B. siamensis siamensis) was closely clustered with a clade of two families, Lecithodendriidae and Pleurogenidae. Based on the BLAST result, the sample 47.1PCR1 belonged to the family Pleurogenidae (similarity ~90%). A sequence of monostome cercaria (10.2MBR2 from B. siamensis siamensis) was highly supported to congregate with the Catatropis vietnamensis species (MH750020) within family Notocotylidae (clade E); the genetic divergence between these two identical sequences was 0.0%. Three samples of echinostome cercaria were arranged within family Echinostomatidae (clade F) and were proximally related to three different species with high posterior probability supports, including close relationships between a couple of 13.1SPS1 (from I. exustus) and Petasiger exaeretus (KY283998), demonstrating 10.0% of genetic divergence; 1NC1 (from R. auricularia) and Hypoderaeum conoideum (AJ564385), demonstrating 0.2% genetic divergence; and 25.1BKN1 (from I. exustus) and Echinostoma malayanum (JF412730), demonstrating 0.0% genetic divergence. A clade of family Heterophyidae (clade G) was built by including two samples of parapleurolophocercous cercaria; sample 28.1MBR1 (from M. tuberculata) was highly correlated with Acanthotrema tridactyla (MH991954), demonstrating 0.3% genetic divergence, whereas sample 4.1BN2 (from M. tuberculata) was observed to relate closely with Haplorchis pumilio (KP165437), demonstrating 0.0% genetic divergence. The brevifurcate-pharyngeate-clinostomatoid cercaria (1.1BN1 from R. auricularia) was included within a clade of family Clinostomidae (clade H) demonstrating close correlation with Clinostomum phalacrocoracis (KJ786982), and the genetic divergence between sequences was 3.3%. A family Diplostomidae clade (clade I) revealed that the strigea cercaria (1.2LS2 from R. auricularia) was positioned as a basal clade of the family, with no close relationship to any specific species. Three samples of vivax cercaria were grouped in family Cyathocotylidae (clade J) without specific species of the close relationship. Among these vivax cercaria, 33.2SM1 (from the F. sumatrensis polygramma snail) was highly correlated with 8SM2 (from the F. sumatrensis polygramma) (0.0% genetic divergence) compared to 48.1MBR1 (from F. sumatrensis polygramma); genetic divergences of a pair of 33.2SM1 and 48.1MBR1, and a pair of 8SM2 and 48.1MBR1 were 16.9 and 16.6%, respectively. Regarding mutabile cercaria (clade D), two samples (10BKN1 and 42BKN1 from the same snail species, B. siamensis siamensis) were clustered together and showed no correlation with species in the same family; because the ITS2 data of organisms in family Lissorchiidae have never been entered into the GenBank database, 0.0% sequence divergence was observed between these two identical samples. Nevertheless, mutabile cercaria was predominantly identified and classified based on their morphological characters.

Fig. 4. Phylogenetic tree of the current cercariae and related trematodes based on the ITS2 region using BI analyses. The support value of posterior probability was represented at each node. The bold and coloured taxa represent the cercaria samples obtained from the current study. A–J alphabets within coloured boxes refer to a clade of each family of digenean trematodes. Scale bars were drawn to measure the branch length as expected number of substitutions per site.
Discussion
A wide range of infective investigations of cercaria-stage trematodes in freshwater snails have been performed in Thailand, particularly in northern and northeastern regions, and these studies have demonstrated high diversities of both trematode larvae and freshwater snail intermediate hosts (Krailas et al., Reference Krailas, Namchote, Koonchornboon, Dechruksa and Boonmekam2014; Kulsantiwong et al., Reference Kulsantiwong, Prasopdee, Piratae, Khampoosa, Thammasiri, Suwannatrai, Boonmars, Viyanant, Ruangsitichai, Tarbsripair and Tesana2015; Anucherngchai et al., Reference Anucherngchai, Tejangkura and Chontananarth2016, Reference Anucherngchai, Tejangkura and Chontananarth2017; Sripa et al., Reference Sripa, Kiatsopit and Piratae2016; Wongsawad et al., Reference Wongsawad, Wongsawad, Sukontason, Phalee, Noikong-Phalee and Chai2016; Chontananarth and Wongsawad, Reference Chontananarth and Wongsawad2017; Chontananarth et al., Reference Chontananarth, Tejangkura, Wetchasart and Chimburut2017; Kulsantiwong et al., Reference Kulsantiwong, Prasopdee, Labbunruang, Chaiyasaeng and Tesana2017; Chantima et al., Reference Chantima, Suk-Ueng and Kampan2018; Veeravechsukij et al., Reference Veeravechsukij, Namchote, Neiber, Glaubrecht and Krailas2018; Haruay and Piratae, Reference Haruay and Piratae2019). A variety of types of cercariae have been revealed in snail intermediate hosts collected from several provinces in central Thailand. However, cercarial infection in naturally occurring freshwater snail species has never been comprehensively investigated in the Bangkok province. The current study found an overall 3.31% prevalence of cercarial infection in freshwater snails collected from natural ponds and canals in the Bangkok area. This overall prevalence of infection differs slightly from previous reports investigating cercarial infection in central Thailand. Anucherngchai et al. (Reference Anucherngchai, Tejangkura and Chontananarth2016) surveyed the cercarial stages of trematodes in freshwater snails from the Chao-Phraya Basin and reported an overall cercarial infection rate of 5.90%, whereas Chontananarth et al. (Reference Chontananarth, Tejangkura, Wetchasart and Chimburut2017) determined a 4.70% overall prevalence of trematode cercariae infection in freshwater snails from Nakhon Nayok Province. The lower infection rate determined in this current study may reflect the low diversity of animal hosts (second-intermediate hosts and definitive hosts) investigated because of the lack of suitable habitats in urbanization areas such as the one in this study. McKinney (Reference McKinney2008) has suggested that diversities in species of invertebrates, amphibians, reptiles and mammals tend to be lower in extreme urbanization areas. Furthermore, the lack of certain anthropogenic activities, such as open-field defecation and urination, and a comparatively low proportion of livestock farming can lead to low trematode infection rates in freshwater snails (Mereta et al., Reference Mereta, Bedewi, Yewhalaw, Mandefro, Abdie, Tegegne, Birke, Mulat and Kloos2019). Species diversity of snail faunas served as first-intermediate hosts may also reflect the low infection rate in this study. Snail species diversity of the current study (20 snail taxa observed) is quite higher than the studies of Anucherngchai et al. (Reference Anucherngchai, Tejangkura and Chontananarth2016) and Chontananarth et al. (Reference Chontananarth, Tejangkura, Wetchasart and Chimburut2017) (14 snail taxa observed) mentioned above, concordant with a previous study (Johnson et al., Reference Johnson, Lund, Hartson and Yoshino2009) that they investigated the effect of snail diversity on transmission of Schistosoma mansoni and indicated reduction of parasitic transmission into snail hosts due to increasing of snail community diversity.
With regards to snail intermediate hosts, B. siamensis siamensis was the highest and most frequently infected snail species, demonstrating an infection rate of 16.16%, including seven of the eight identified cercaria types. A previous study (Chanawong and Waikagul, Reference Chanawong and Waikagul1991) has emphasized that this snail species is highly susceptible to trematode infection compared to B. siamensis goniomphalos, so it is possible that the former is easily infected by several types of trematodes. However, the previous study investigated only one trematode (O. viverrini) infection in Bithynia spp., and the susceptibility of B. siamensis siamensis to trematode infection is still uncertain. In contrast, the lowest rate of cercarial infection was observed in the P. acuta snail (0.08%). Based on field observations, the pulmonate snail P. acuta was commonly found to exist near/at the water surface level by attaching to floating aquatic plants (particularly water hyacinth) or floating objects that move with the water current. Therefore, it is possible that the low cercarial infection rate observed herein results from a low probability of encounter between this pulmonate snail species and miracidium-stage trematodes. Interestingly, the current study revealed that P. acuta snail has been observed to be infected by cercarial trematode (xiphidiocercariae) in Thailand for the first time, indicating the potential role of invasive snail species to serve as intermediate hosts of trematode cercariae in the non-native region. The snail P. acuta considered as a globally invasive snail species was officially reported to distribute in Thailand in 2018; this invader snail was found to live in various habitat types, consisting of rivers, paddy field and attaching on ornamental aquatic plants in concrete pots or ponds (Ng et al., Reference Ng, Limpanont, Chusongsang, Chusongsang and Panha2018). Even though no infection of cercarial trematodes in P. acuta were previously recorded in Thailand, this snail was found to harbour furcocercous cercaria (type 4) from Valencia, Spain with a low infection rate of 0.04% (Toledo et al., Reference Toledo, Muñoz-Antolí, Pérez and Esteban1998), and paramphistome and brevifurcate-apharyngeate cercariae from the Middle Rio Grande, New Mexico (Kraus et al., Reference Kraus, Brant and Adema2014). Infection of this invader snail by indigenous parasites in invasive range, except for North America, is another possible reason to describe the low proportion of cercarial-infected P. acuta in invasive range, also in Thailand. Ebbs et al. (Reference Ebbs, Loker and Brant2018) elucidated the potential of P. acuta from native and invasive regions to act as an intermediate host of trematode larvae and demonstrated that the cercarial infection rate of this snail from the native region was found to be significantly greater than that from the invasive region, in concordance with the enemy-release hypothesis – invasive species encounters a decrease of regulation by predators, parasites and other natural enemies in the invasive region, leading to great distribution and abundance of an invader (Keane and Crawley, Reference Keane and Crawley2002; Torchin et al., Reference Torchin, Lafferty, Dobson, McKenzie and Kuris2003).
Of the eight cercaria types identified in the freshwater snails investigated, mutabile cercaria exhibited the highest prevalence of infection (1.86%), possibly because definitive and intermediate hosts for this cercaria type exist in the same ecosystem. Mutabile cercaria usually requires several freshwater fish as definitive hosts in order to develop into adult-stage intestinal flukes (Schell, Reference Schell1970; Frandsen and Christensen, Reference Frandsen and Christensen1984; Shimazu, Reference Shimazu2016; Ramakant et al., Reference Ramakant, Rahul and Anand2018). Transmission of trematode larvae from intermediate hosts to definitive hosts is greatly facilitated if both host types simultaneously inhabit the same aquatic ecosystem, particularly compared to trematodes requiring terrestrial animals (such as reptiles, birds and mammals) as their definitive hosts. Conversely, five cercaria types (brevifurcate-pharyngeate-clinostomatoid, monostome, parapleurolophocercous, strigea and vivax) displayed low infection rates with a prevalence approximating or below 0.1%. These five cercaria morphologies appeared to infect a few species of snail hosts, whereas the remaining cercariae infected in at least six snail species, suggesting that the capability of the parasite to infect a wide range of hosts can facilitate the success of parasitic transmission. To consider transmission success of parasites due to diversity of trematode taxa within each cercaria type, more than one single taxon per morphotype was observed in some cercariae (echinostome, parapleurolophocercous, vivax and xiphidiocercariae) based on the molecular identification. The taxon diversity within each cercaria type seems to be an unworthy reason for determining the parasitic transmission success because both low and high infection rates can be herein found in the cercaria types represented more than one single taxon; for instead, low infection rate was found in parapleurolophocercous and vivax cercariae, in the same time, high infection rate was also found in echinostome cercaria and xiphidiocercariae. Xiphidiocercariae commonly infected a variety of snail species, 8 of the 11 infected snail species identified. Xiphidiocercariae can be subdivided into several subgroups based on their morphological and molecular characters (Hall and Groves, Reference Hall and Groves1963; Schell, Reference Schell1970; Frandsen and Christensen, Reference Frandsen and Christensen1984; Shchenkov et al., Reference Shchenkov, Denisova, Kremnev and Dobrovolskij2019). Shchenkov et al. (Reference Shchenkov, Denisova, Kremnev and Dobrovolskij2019) recently identified five xiphidiocercariae; four of these corresponded to actual digenean taxa (Lecithodendriidae, Microphallidae, Pleurogenidae and Prosthogonimidae), and the remaining subtype was classified as undescribed taxa, for the first time, based on molecular evidence. Therefore, the appearance of xiphidiocercariae in several snail hosts may be the manifestation of each subtype's different adaptations for infecting different snail hosts. The xiphidiocercariae identified in the current study could be classified into three families of digenean trematodes based on molecular evidence, in agreement with a previous report suggesting that the larger the number of snail species infected, the richer the diversity of resulting trematode faunas and communities (Selbach et al., Reference Selbach, Soldánová, Feld, Kostadinova and Sures2020).
For double and triple infections, 10 B. siamensis siamensis snails were infected with two or three cercaria types at the same time. Mutabile cercaria was commonly observed in all cases of these two phenomena. A rare proportion of the concurrent infections observed in the current study agree with the results of previous studies that report concurrent infections of cercarial trematodes in other freshwater snails, i.e. M. tuberculata, Radix natalensis and I. exustus (Devkota et al., Reference Devkota, Budha and Gupta2011; Krailas et al., Reference Krailas, Namchote, Koonchornboon, Dechruksa and Boonmekam2014; Outa et al., Reference Outa, Sattmann, Köhsler, Walochnik and Jirsa2020). Furthermore, Sewell (Reference Sewell1922) suggested that concurrent infections in individual snails occurred accidentally and more commonly in snails that serve as first-intermediate hosts, agreeable with the current study that all mixed cercariae were observed to utilize the snail B. siamensis siamensis as their first-intermediate host. Nevertheless, some trematode requires facilitation by prior infection of other trematodes in the same snail hosts. Previous studies (Walker, Reference Walker1979; Appleton, Reference Appleton1983) revealed concurrent infection of schistosomatid (Austrobilharzia terrigalensis) cercariae and other trematode cercariae in a marine snail (Velacumantus australis), for which high infection of the schistosomatid was usually observed in the prior-infected snails; Walker (Reference Walker1979) predicted that this schistosomatid is able to inhabit in the snails that were priorly infected by other trematodes and their host's defense mechanism was switched off.
The discussion below mostly details various aspects of each of the trematode cercaria types investigated in terms of the family level assigned to those cercariae. However, important species of digenean trematodes in certain families are discussed as well.
According to morphological and molecular investigations of the trematode cercariae investigated, eight morphological types of cercariae were molecularly classified into at least nine families of digenean trematodes. The phylogram indicated that four xiphidiocercariae samples may be classified into three families, namely, Lecithodendriidae, Pleurogenidae and Telorchiidae. Shared by these families, this cercarial morphotype can yield a high diversity of trematode fauna, congruent with the previous study (Shchenkov et al., Reference Shchenkov, Denisova, Kremnev and Dobrovolskij2019) mentioned above. Of these three families, the family Lecithodendriidae is noteworthy as a group of intestinal flukes in humans (Chai et al., Reference Chai, Shin, Lee and Rim2009; Chai, Reference Chai2019) and is generally found to infect amphibians, mammals (especially bats) and occasionally birds (Schell, Reference Schell1970; Frandsen and Christensen, Reference Frandsen and Christensen1984; Chai, Reference Chai2019). In Thailand, a Lecithodendriid trematode, Phaneropsolus spinicirrus, was reported to parasitize a human host and was observed in a postpraziquantel treatment stool sample from a female patient in Kalasin Province, northeastern Thailand (Kaewkes et al., Reference Kaewkes, Elkins, Haswell-Elkins and Sithithaworn1991). The family Pleurogenidae was known to parasitize amphibians, particularly anurans; and two pleurogenid trematodes (Pleurogenes chiangmaiensis and Pleurogenoides sphaericus) have been observed in a few species of adult frogs from Chiang Mai Province, northern Thailand (Wongsawad et al., Reference Wongsawad, Rojtinnakorn, Wongsawad, Rojanapaibul, Marayong, Suwattanacoupt, Sirikanchana, Sey and Jadhav2004). The Telorchiidae is a family of intestinal flukes infecting amphibian and reptile definitive hosts (Schell, Reference Schell1970; Zhytova et al., Reference Zhytova, Romanchuk, Guralska, Andreieva and Shvets2019).
The mutabile cercaria in the current study was identified and classified based on their morphological characters and belong to the family Lissorchiidae. Based on the phylogram, a clade of two mutabile samples was positioned as a sister clade of the xiphidiocercariae clade. No correlations among the current mutabile samples and other lissorchiid species were determined because the GenBank database does not contain ITS2 data from any lissorchiid species. However, the family Lissorchiidae of trematodes infects distinct regions of the digestive tracts of fish definitive hosts, i.e. the rectum, gut and especially intestine (Shimazu, Reference Shimazu2016). Trematode faunas of this family are rarely mentioned in Thailand, which may be due to a lack of understanding of the negative impact of this trematode in terms of economic importance, livestock farming and human public health.
For the monostome, this cercaria was molecularly classified into a clade of the family Notocotylidae with high posterior probability support for a close relationship with C. vietnamensis, which has been reported to infect the intestinal caeca of the Mallard (Anas platyrhyncha) under experimental conditions and to utilize the freshwater thiarid snail M. tuberculata as an intermediate host (Izrailskaia et al., Reference Izrailskaia, Besprozvannykh, Tatonova, Nguyen and Ngo2019). Depending on posterior probability and genetic divergence, it is possible that the monostome cercaria (10.2MBR2) has an identical sequence and is conspecific with the C. vietnamensis. Furthermore, no differences are observed in the morphological characters of the monostome cercaria investigated and the C. vietnamensis cercaria. Basically, notocotylid trematodes require birds and mammals as their definitive hosts and inhabit the small intestine, colon and caeca (Schell, Reference Schell1970; Frandsen and Christensen, Reference Frandsen and Christensen1984; Zhytova et al., Reference Zhytova, Romanchuk, Guralska, Andreieva and Shvets2019; Selbach et al., Reference Selbach, Soldánová, Feld, Kostadinova and Sures2020). Several studies in Thailand, particularly northern and northeastern regions, have reported monostome cercaria infections in bithyniid snails, as discovered in the current study (Ngern-klun et al., Reference Ngern-klun, Sukontason, Tesana, Sripakdee, Irvine and Sukontason2006; Chontananarth and Wongsawad, Reference Chontananarth and Wongsawad2013; Tesana et al., Reference Tesana, Thabsripair, Suwannatrai, Haruay, Piratae, Khampoosa, Thammasiri, Prasopdee, Kulsantiwong, Chalorkpunrut and Jones2014; Kiatsopit et al., Reference Kiatsopit, Sithithaworn, Kopolrat, Namsanor, Andrews and Petney2015; Kulsantiwong et al., Reference Kulsantiwong, Prasopdee, Piratae, Khampoosa, Thammasiri, Suwannatrai, Boonmars, Viyanant, Ruangsitichai, Tarbsripair and Tesana2015; Namsanor et al., Reference Namsanor, Sithithaworn, Kopolrat, Kiatsopit, Pitaksakulrat, Tesana, Andrews and Petney2015; Anucherngchai et al., Reference Anucherngchai, Tejangkura and Chontananarth2016; Kulsantiwong et al., Reference Kulsantiwong, Prasopdee, Labbunruang, Chaiyasaeng and Tesana2017; Chantima et al., Reference Chantima, Suk-Ueng and Kampan2018).
A clade of family Clinostomidae was built by including the current sample of brevifurcate-pharyngeate-clinostomatoid cercaria. This sample was closely correlated with C. phalacrocoracis; however, the posterior probability support determined does not seem to support the mutual identity and conspecificity of these two sequences. The family Clinostomidae of gastrointestinal parasites infects the oral cavities, pharynxes and oesophagi of reptiles and birds (especially piscivorous birds) that serve as definitive hosts (Schell, Reference Schell1970; Frandsen and Christensen, Reference Frandsen and Christensen1984).
With regards to human parasites, five of the trematode cercaria families identified herein – Cyathocotylidae, Diplostomidae, Echinostomatidae, Heterophyidae and Lecithodendriidae – have been reported to play an important role as human intestinal flukes (Schell, Reference Schell1970; Frandsen and Christensen, Reference Frandsen and Christensen1984; Chai, Reference Chai2019). The Lecithodendriidae was already mentioned in the previous paragraph for their important role in human parasites. Among these, the family Heterophyidae, comprised of intestinal flukes that infect birds and mammals, is the largest group reported to appear in humans in Southeast Asia (Chai et al., Reference Chai, Shin, Lee and Rim2009). Heterophyidae flukes are also highly distributed throughout Thailand (Chontananarth et al., Reference Chontananarth, Wongsawad, Chomdej, Krailas and Chai2014). The current phylogram indicates that two parapleurolophocercous cercaria samples are closely related to two different heterophyid species, A. tridactyla and H. pumilio, each displaying a high support value and low genetic divergence. The cercarial stages of these two heterophyid species have also been found to infect thiarid snails in several areas of Thailand (Dechruksa et al., Reference Dechruksa, Krailas, Ukong, Inkapatanakul and Koonchornboon2007; Krailas et al., Reference Krailas, Namchote, Koonchornboon, Dechruksa and Boonmekam2014; Veeravechsukij et al., Reference Veeravechsukij, Namchote, Neiber, Glaubrecht and Krailas2018). Humans can become infected with heterophyid flukes by consuming uncooked or semicooked fish containing heterophyid metacercariae. A previous study (Krailas et al., Reference Krailas, Veeravechsukij, Chuanprasit, Boonmekam and Namchote2016) revealed high infections of H. pumilio metacercaria in red-tailed tinfoil barbs (Barbonymus altus) taken from the Pasak Cholasid Reservoir, Thailand; and this fish is utilized for human consumption. Additionally, adult H. pumilio were observed in the postpraziquantel treatment stools of 12 patients from northeastern, Thailand (Radomyos et al., Reference Radomyos, Bunnag and Harinasuta1983). Heterophyid trematode infections have been observed in humans from other counties in Southeast Asia as well, including Lao PDR, South Korea and the Philippines (Belizario et al., Reference Belizario, de Leon, Bersabe, Baird and Bangs2004; Chai et al., Reference Chai, Sohn, Yong, Eom, Min, Lee, Lim, Insisiengmay, Phommasack and Rim2013, Reference Chai, Kim and Seo2014). With regards to aquatic cultivations, Clausen et al. (Reference Clausen, Madsen, Murrell, Phan Thi, Nguyen Manh, Viet and Dalsgaard2012) studied the relationship of freshwater snails and juvenile rohu fish (Labeo rohita) collected from cultured ponds and infected with heterophyid cercariae and metacercariae, respectively. They concluded that an abundance of infected snails can increase fish infections and suggested that the density of heterophyid-infected snail hosts (as well as potential snail hosts) is a useful indicator for predicting juvenile fish infections.
Trematode faunas in the family Echinostomatidae are intestinal parasites that generally infect reptiles, birds and mammals, including humans (Schell, Reference Schell1970; Chai et al., Reference Chai, Shin, Lee and Rim2009; Toledo and Esteban, Reference Toledo and Esteban2016; Chai, Reference Chai2019). In the current study, three echinostome cercaria samples were grouped within an Echinostomatidae clade with a close relationship to different echinostomatid species, indicating a greater diversity of echinostomatids in Bangkok, Thailand. Two of the three echinostome cercaria samples were determined to have possible conspecificity with two echinostomatid species. This potentially makes a couple of the 25.1BKN1 sample and E. malayanum and another couple of the 1NC1 sample and H. conoideum, based on the low sequence divergence and high support value obtained for each couple. Echinostoma malayanum has been found to parasitize humans from several Southeast Asian countries, including Malaysia, the Philippines, Singapore and Thailand, where this echinostomatid species constitutes a human public health problem (Lie and Virik, Reference Lie and Virik1963; Bhaibulaya et al., Reference Bhaibulaya, Charoenlarb and Harinasuta1964; Belizario et al., Reference Belizario, Geronilla, Anastacio, de Leon, Suba-an, Sebastian and Bangs2007; Chai, Reference Chai2019). In addition, Toledo and Esteban (Reference Toledo and Esteban2016) revealed that at least 24 species could be causative agents of human echinostomiasis, particularly in Southeast Asia and the Far East. Diarrhoea, fatigue, epigastric and abdominal pain, and weight loss are common symptoms when humans are infected with echinostomatids (Graczyk and Fried, Reference Graczyk and Fried1998; Toledo et al., Reference Toledo, Esteban and Fried2006). In addition to their negative effects on humans, three echinostomatid species (Echinostoma revolutum, Echinoparyphium recurvatum and H. conoideum) have been found to infect free-grazing ducks from central, northern and northeastern Thailand with relatively high infection rates (approximating 57%); and these ducks are economically important for egg and meat production (Saijuntha et al., Reference Saijuntha, Duenngai and Tantrawatpan2013).
For the family Diplostomidae, the phylogram revealed that the strigea cercaria observed was included in a clade of the family Diplostomidae, with no correlation with any specific diplostomid species. This family is generally known as a group of intestinal flukes in birds and mammals, including humans (Schell, Reference Schell1970; Chai, Reference Chai2019; Zhytova et al., Reference Zhytova, Romanchuk, Guralska, Andreieva and Shvets2019). However, diplostomid trematodes found in humans from Thailand are rarely reported. In the matter of diplostomid metacercariae, two previous studies revealed natural infections of Euclinostomum metacercariae in osphronemid fish (Trichopsis vittata, Trichopsis schalleri, Betta splendens and Betta imbellis) from northern, northeastern and southern Thailand (Purivirojkul and Sumontha, Reference Purivirojkul and Sumontha2013; Senapin et al., Reference Senapin, Phiwsaiya, Laosinchai, Kowasupat, Ruenwongsa and Panijpan2014).
Three samples of vivax cercaria were clustered within a clade of the family Cyathocotylidae and displayed no close relationships with any of the specific cyathocotylid species considered. The genetic divergences observed among three vivax cercaria samples indicated that at least two different taxa of the cyathocotylids were found in the current study. Basically, cyathocotylids are a group of intestinal trematodes that infect reptiles, birds and mammals, including humans (Schell, Reference Schell1970; Frandsen and Christensen, Reference Frandsen and Christensen1984; Chai, Reference Chai2019). However, no human in Thailand has been reported to be infected with this trematode group. Only one cyathocotylid, Prohemistomum vivax, has been found parasitizing a single patient from Cairo, Egypt; five adult trematodes were collected from this patient's small intestine (Nasr, Reference Nasr1941; Chai, Reference Chai2019).
With regards to the study area, Patarwut et al. (Reference Patarwut, Chontananarth, Chai and Purivirojkul2020) recently reported the infections of metacercaria-stage Posthodiplostomum sp. (diplostomatid) and Cyathocotylidae sp. (cyathocotylid) in a Wrestling Halfbeak (Dermogenys pusilla) from the Bangkok Metropolitan Region of Thailand; because they utilized the same study area, these observations could be related to our observations regarding the diplostomid and cyathocotylid life cycles of strigea and vivax cercariae reported herein.
In conclusion, cercarial trematode infections in freshwater snail intermediate hosts from Bangkok were comprehensively investigated for the first time in this study area and displayed a slightly different prevalence of infection compared to previous studies evaluating cercarial infections in freshwater snails from central Thailand. Over 50% of the snail species were determined to be infected with cercarial trematodes. Three snail species, including B. siamensis siamensis, F. sumatrensis polygramma and R. auricularia, demonstrated the ability to harbour at least 50% of all cercaria types, indicating the important role of the freshwater snails in the successful transmission of digenean trematodes. A globally invasive snail, P. acuta, was recorded to be infected by trematode cercariae (xiphidiocercariae) in Thailand for the first time, revealing the potential role of an invasive snail to serve as an intermediate host of trematode cercariae in non-native region. Of the eight morphological cercaria types identified, five cercariae belonging to five trematode families were noted to be human parasites, and two of these families, Heterophyidae and Echinostomatidae, are highly distributed in Thailand. Adult trematodes of these two families have been frequently reported in several vertebrate hosts, namely, aquatic birds, rats, cats, dogs and humans. Although, excluding the schistosome cercaria that causes cercarial dermatitis, cercaria-stage digenean trematodes do not directly injure humans, knowing and understanding the general biology of trematode cercariae (including diversity, distribution, infection rate and host range) is important and necessary for preventing and controlling parasitic transmission that impacts aquatic cultivations, livestock farming and human health.
Acknowledgements
We thank our colleagues for their assistance about snail collection, and anonymous reviewers for their valuable recommendations.
Financial support
This study was funded by the Center of Excellence on Biodiversity (BDC), Office of Higher Education Commission (BDC-PG2-161004), the Department of Zoology, Faculty of Science, Kasetsart University, and the Science Achievement Scholarship of Thailand (SAST).
Conflict of interest
The authors declare there are no conflicts of interest.
Ethical standards
The temporary rearing of snail specimens during observations of cercarial infection and the investigation of the cercarial stage in freshwater snails were approved by the ethics committee of Kasetsart University (approval no. ACKU61-SCI-033).