INTRODUCTION
The two morphologically similar hookworm species Ancylostoma braziliense Gomez de Faria 1910, and A. ceylanicum Looss, 1911 have been a source of confusion for many years. During most of the first half of the 20th century, A. ceylanicum was regarded as a synonym of A. braziliense (Skrjabin et al. 1951; Yorke and Maplestone, 1926), and often referred to as A. braziliense var ceylanicum (Wijers and Smit, 1966) until Biocca (1951) provided morphological evidence that they were different. Nevertheless, several authors continued to regard them as synonymous (Rep, 1963). In cross-breeding experiments using A. braziliense and A. ceylancium, Rep et al. (1968) provided evidence to show that the two populations failed to produce progeny and concluded that they were separate species. Yoshida (1971) provided additional morphological evidence to support this.
Both A. braziliense and A. ceylanicum have been reported in canids and felids in tropical regions of the Americas, Asia and South Africa (Baker et al. 1989). The distribution of these hookworms may overlap with each other as well as with A. caninum and A. tubaeforme. In Australia, A. braziliense has long been known to occur in cats and dogs in northern Queensland (Beveridge, 2002; Setasuban and Waddell, 1973). Stewart (1994) identified A. ceylanicum for the first time in Australia based on a morphological study of hookworms from cats in Townsville. Gasser et al. (1996) then provided genetic markers for the identification of 4 species of hookworms from dogs and cats, using specimens from the Stewart (1994) study to characterize A. ceylanicum.
Traub et al. (2004) identified species of hookworms present in dogs in India by the polymerase chain reaction – restriction fragment length polymorphism (PCR-RFLP) method using DNA from eggs in faecal samples. This study noted that the sequence of the internal transcribed spacer (ITS) region of a hookworm isolate from a cat from the Cocos (Keeling) Islands in the Indian Ocean, was identical to that of the sequences of A. ceylanicum (Reference number AHC 30168) isolated from a cat in Townsville (GenBank® Accession numbers Y19183, Z70740, AJ001593) (see Chilton and Gasser, 1999; Gasser et al. 1996; Stewart, 1994). Utilizing phylogenetic analysis of ITS sequences of the Townsville isolate of A. ceylanicum (Chilton and Gasser, 1999) and other Ancylostomatidae that parasitize canids, felids and humans; namely A. caninum, A. tubaeforme, Uncinaria stenocephala, A. duodenale, Necator americanus (GenBank® Accession numbers Y19181, AJ001591, Z70739, Y19182, AJ001592, Z70741, AJ001679, AJ001594, AF194145, AF217891), Traub et al. (2004) concluded that an unidentified Ancylostoma isolate found in dogs in tea-growing communities of Assam must be A. braziliense. Traub et al. (2004) also acknowledged the lack of a positive control in making this conclusion.
Differentiation of hookworm species in dogs and cats is important not only from a veterinary standpoint but also from a public health perspective. All hookworm species of dogs and cats are capable of causing a degree of skin irritation, referred to as ‘ground itch’ when occurring on the soles of the feet, or cutaneous larva migrans (CLM) or ‘creeping eruptions’ when elsewhere on the body (Maplestone, 1933). All these symptoms are attributable to hypersensitivity reactions caused by migrating nematode larvae, and may be exacerbated by the introduction of bacteria during skin penetration. Ancylostoma braziliense is the most frequently implicated aetiological agent (Chaudhry and Longworth, 1989; Malgor et al. 1996) and is the species responsible for prolonged CLM, with tracts in the skin being recorded for over 100 days in some cases (Dove, 1932). A. braziliense cannot penetrate deeper below the human epidermis (Sandground, 1939), but penetrates the skin of dogs considerably faster than either A. ceylanicum or A. caninum (Vetter and Linden, 1977). On the other hand A. ceylanicum penetrates human skin far more effectively, and cutaneous symptoms seldom last for long, other than in highly allergic subjects. In addition to this, A. ceylanicum is the only canine or feline hookworm that has been shown to produce both experimental and naturally patent infections in humans (Areekul et al. 1970; Chowdhury and Schad, 1972). In experimental infections, intermittent gastrointestinal symptoms such as severe abdominal pain and flatulence were observed by the 3rd to 4th week of infection and eggs in the stool a few days later. The infection was observed to be chronic but light for 30 weeks prior to termination of the infection (Carroll and Grove, 1986; Yoshida et al. 1971). Natural infections with A. ceylanicum have also been sporadically reported. With the exception of Dutch servicemen returning from West New Guinea, who suffered heavy infections with concurrent anaemia (Anten and Zuidema, 1964), natural and mostly light infections have been reported from humans in the Philippines (Velasquez and Cabrera, 1968), Taiwan (Yoshida et al. 1968) Thailand (Areekul et al. 1970) and India (Chowdhury and Schad, 1972). A. ceylanicum infections in dogs and cats have been shown to be endemic in the South East Asian region (Choo et al. 2000; Setasuban et al. 1976; Yoshida et al. 1968, 1973). The last report of A. ceylanicum in humans was within a review listing species of parasites reported in China from 1988–1997 (Xu et al. 1997). Since morphological differentiation of Ancylostoma eggs in faeces is impossible, it is probable that recent studies in this geographical region have not aimed to identify the species of hookworm and assumed the species found in human surveys to be A. duodenale.
The present study re-evaluates the identity of A. ceylanicum in Australia and A. braziliense in northeast India in light of discrepancies between the conclusions of Traub et al. (2004) and the examination of DNA from worms identified morphologically as A. ceylanicum from the laboratory of one of the authors (J.M.B.).
MATERIALS AND METHODS
Source of adult hookworms
The Nottingham strain of A. ceylanicum was acquired in 1984 from Dr Rajasekariah of Hindustan Ciba-Geigy Ltd, Bombay, India. This strain is thought to have originated from dogs in India (Ray et al. 1972) and was probably isolated in the late 1960s. It has been adapted to passage through hamsters, and in Nottingham has been maintained for the last 22 years by regular passage (3–4 times/annum) in DSN hamsters through oral administration of larvae (Garside et al. 1989).
Adult A. braziliense were obtained from the gastrointestinal tracts of feral cats trapped and humanely euthanased as part of a control programme on the Cocos (Keeling) Islands conducted by the Western Australian Department of Conservation and Land Management. The intestinal tracts were removed from euthanased cats as soon as possible before being cut into thirds and fixed in 70% ethanol prior to being shipped back to Murdoch University where the intestines were opened and examined for the presence of hookworms.
Morphological identification
The heads and tails of adult hookworms were fixed in 70% ethanol prior to being cleared in lactophenol on slides for microscopical examination. The most reliable difference between the species is the configuration of the lateral bursal rays of the male (Biocca, 1951; Norris, 1971; Yoshida, 1971; Yoshida et al. 1968). All three lateral rays of A. braziliense are clearly widely separated at their tips, whereas the mediolateral and posteriolateral are essentially parallel in A. ceylanicum so that the tips of these rays are very close together, but separated from the tip of the externolateral ray. Another reliable difference is the position of attachment of the externodorsal ray to the dorsal trunk (Biocca, 1951) which in A. braziliense is closer to the origin of the dorsal trunk. Yoshida (1971) noted that using the shape of the teeth to differentiate these species was difficult and that therefore differentiation of females is unreliable. He therefore provided 4 other criteria with which to differentiate females, but we found these differences to be ill-defined, so we only used male specimens for DNA extractions.
DNA extraction and Polymerase Chain Reaction (PCR)
A single adult male specimen of A. ceylanicum and 2 male specimens of A. braziliense with their anterior and posterior ends dissected for morphological identification, were washed in physiological saline and the DNA extracted using the DNeasy Tissue Kit (Qiagen) according to the manufacturer's instructions.
The internal transcribed spacer (ITS)-1, 5·8S and ITS2 regions of A. ceylanicum and A. braziliense controls were amplified by PCR using previously published primers RTGHF1 (5′-CGTGCTAGTCTTCAGGACTTTG-3′) and RTGHR1 (5′-CGTTGTCATACTAGCCACTGC-3′) (Traub et al. 2004). According to sequence information available on GenBank® the PCR was expected to amplify an approximate 693 bp region of A. ceylanicum (GenBank® Accession numbers Y19183, Z70740, AJ001593). The sequence information of A. braziliense was not available on GenBank®. The PCR conditions were identical to those described by Traub et al. (2004).
Sequencing and analysis of PCR products
The PCR products were purified using Qiagen spin columns (Qiagen) and sequenced using an ABI 3730 48 capillary DNA sequencer (Applied Biosystems) using Big Dye version 3.1 terminators. DNA sequences were analysed using Finch TV Version 1.3.1 (Geospiza Inc.) and aligned using Clustal W (Kyoto University Bioinformatics Center). Distance (neighbour-joining) and Maximum Parsimony analyses were performed using the ITS2 of related hookworms on MEGA 3.1 (Kumar et al. 2004). ITS2 sequences of A. duodenale (GenBank® Accession number AJ001594), A. tubaeforme (GenBank® Accession number AJ001592), A. caninum (GenBank® Accession number AJ001591) and A. ceylanicum submitted by Chilton and Gasser (GenBank® Accession number AJ001593) were included with Bunostomum trigonocephalum (GenBank® Accession number AJ001595) as an out-group. The ITS2 sequence data for the dog hookworm presumed to be A. braziliense from Assam, India by Traub et al. (2004) was also included in the tree (GenBank® Accession number DQ780009). Sequence comparisons were made between mitochondrial cytochrome oxidase I sequences submitted for A. ceylanicum (Townsville isolate) by Hu et al. (2002) (GenBank® Accession number AJ407937) and A. ceylanicum (also a Nottingham isolate) submitted by McDonnell and Matthews (GenBank® Accession number AF225917).
RESULTS
Morphological identification of parasites
Copulatory bursae of male hookworms from the laboratory of J. M. Behnke conform to the typical A. ceylanicum morphology in having the mediolateral and posteriolateral rays running parallel to their tips, and in having the externodorsal ray attached relatively distally along the dorsal trunk (Fig. 1). The average width of the cuticular striations just anterior to the bursa was 5·66 μm. Specimens have been deposited in the Western Australian Museum (WAM V4615). The structure of the bursae of male hookworms collected from a Cocos Island cat conformed to the characteristic morphology of A. braziliense, with all 3 lateral rays diverging, and in having a relatively proximal attachment of the externodorsal ray to the dorsal trunk (Fig. 2). The average width of the cuticular striations just anterior to the bursa was 3·45 μm. Specimens have been deposited in the Western Australian Museum (WAM V4616).

Fig. 1. Lateral view of bursa of male Ancylostoma ceylanicum Nottingham laboratory strain. Lateral rays are marked as externolateral (el), mediolateral (ml) and posteriolateral (pl), and the attachment point of the externodorsal to the dorsal trunk as (ed).
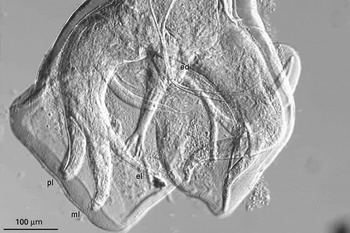
Fig. 2. Ventral view of bursa of male Ancylostoma braziliense from a cat from Cocos Island. Rays are marked as in Fig. 1.
Molecular characterization
Clear and readable sequences were obtained for the PCR products RT-GHF1-RTGHR1 from the hookworm controls of A. ceylanicum and A. braziliense. Nucleotide sequence data for A. braziliense (Cocos Islands) and A. ceylanicum (Nottingham) were deposited into GenBank® under Accession numbers DQ359149 and DQ381541 respectively. A. braziliense and A. ceylanicum controls produced a PCR product of 693 and 681 base pairs respectively. The ITS1, 5·8S and ITS2 sequences of A. braziliense isolated from the cat in the Cocos Islands, Australia, was found to be identical to the previously published sequence of the A. ceylanicum by Chilton and Gasser (1999) isolated from a cat in Townsville (GenBank® Accession numbers Y19183, Z70740, AJ001593) except for a transversional change at position 189 in the ITS2. The ITS2 sequence of A. ceylanicum (Nottingham strain) was found to be 92·0% similar to A. ceylanicum isolated from Townsville and identical to the hookworm species previously presumed to be ‘A. braziliense’ (Traub et al. 2004) from a dog in Assam, India.
Phenogram construction of the ITS2 region of hookworms using neighbour-joining and Maximum Parsimony (Fig. 3), produced strong bootstrap support (100%) for the placement of A. braziliense (Cocos Islands isolate) and ‘A. ceylanicum’ (Townsville isolate) within a single operational taxonomic unit (OTU) in the Ancylostoma species group and strong support (100%) for this OTU as a separate group lying basal to the other 4 species. Taken together, there is now strong evidence to suggest that isolate ‘A. ceylanicum’ from a cat in Townsville genetically belonged to A. braziliense and that the difference between the latter and former isolates is suggestive of geographical diversity within a single OTU.

Fig. 3. Phylogenetic analysis of the aligned ITS2 sequences of related canine, feline and human hookworms using Bunostomum trigonocephalum as an out-group with bootstrap values for distance (Neighbour joining, maximum parsimony) analyses in parentheses. Species names of misidentified species places in single quotation marks (‘..’).
DISCUSSION
The morphological determination of the Townsville isolate of Ancylostoma by Stewart (1994) as A. ceylanicum appears to have been an error. Although the present authors have been unable to see the original specimens, Fig. 4.25 of Stewart (1994) clearly shows the radiation of the 3 lateral rays, and the proximal attachment of the externodorsal ray to the dorsal trunk. Stewart (1994) discounted the importance of lateral rays in favour of the shape of the lateral lobes, and the structure of the teeth on the buccal cutting plates. The study considered the arrangement of the lateral rays to be dependent on the orientation of the specimen, whereas in agreement with Biocca (1951), Yoshida et al. (1968) and Yoshida (1971), we found this character to be highly consistent and distinctive. The structure of the teeth on the cutting plates on the other hand provided ill-defined and inconsistent characters. Yoshida (1971) found that the width of the striae at any part of the body could be used to differentiate these species, but it was not clear if he measured the width at the extremities. We only measured the width of the striae next to the bursa, which was 5·7 μm for A. ceylanicum and 3·5 μm for A. braziliense. This compares to Yoshida's means of 8·7 μm and 4·7 μm respectively for ethanol-fixed male hind bodies.
Genetic data presented herein also clearly support morphological criteria and clearly place the Townsville isolate of A. ceylanicum into the same terminal clade (species group) as A. braziliense. In addition, a sequence comparison of a partial fragment of the mitochondrial cytochrome oxidase I gene from the A. ceylanicum from Townsville (GenBank Accession number AJ407937; Hu et al. 2002) and that of an unpublished sequence for A. ceylanicum derived from the Nottingham isolate (GenBank Accession number AF225917; submitted by McDonnell and Matthews in 2001) revealed differences at 42 nucleotide positions over an alignment length of 393 bp. This magnitude of genetic difference exceeds that for this locus reported among morphologically distinct species of hookworm (Hu et al. 2002), which raises further doubt as to the identification of hookworms from Townsville as A. ceylanicum.
In addition, the determination that the Assam isolates of Traub et al. (2004) were not A. ceylanicum was based on sequence comparison with the data of Chilton and Gasser (1999). Although Traub et al. (2004) stated that A. ceylanicum adult worms were sourced from feral cats in Western Australia to act as a positive control, this statement was made in error. The DNA was sourced from hookworm eggs from feral cats and not adult worms. Since the ITS1 5·8S and ITS2 sequences of this DNA were 100% identical to those of the A. ceylanicum Townsville isolate of Chilton and Gasser (1999), the specimen was treated as an A. ceylanicum positive control in Traub et al. (2004). In addition, the determination that the Assam isolates of Traub et al. (2004) were not A. ceylanicum was based on sequence comparison with the data of Chilton and Gasser (1999), now known to be erroneously identified. It is now apparent that the hookworms found in north Indian dogs have an identical rDNA sequence to the sequenced laboratory strains, and can therefore be considered to be A. ceylanicum.
A. braziliense together with A. caninum and A. tubaeforme have been commonly isolated from dogs and cats in northern Queensland, Northern Territory and the north west of Western Australia (Beveridge, 2002). There is no evidence to support the occurrence of A. ceylanicum in Australia to date. The majority of ancylostomatoid fauna in Australia is represented by introduced species in human and domestic animals (Beveridge, 2002), including A. duodenale in humans, A. tubaeforme and A. braziliense in cats and A. caninum in dogs and dingoes.
From the limited data published, we know that A. ceylanicum appears to be present in India (Traub et al. 2004), Taiwan (Yoshida et al. 1968), central Thailand (Setasuban et al. 1976), Malaysia (Sheikh et al. 1985; Yoshida et al. 1973), Borneo (Choo et al. 2000), Indonesia (Margono et al. 1979) and Surinam in South America (Rep, 1975). There are no recent studies of hookworms of dogs and cats from Papua New Guinea; however, older evidence suggests that A. ceylanicum was present (Anten and Zuidema, 1964). The distribution of A. braziliense in Asia appears to be restricted to the south, below latitude 10 °N. It has, to date, only been reported in Malaysia (Yoshida et al. 1973; Sheikh et al. 1985), Borneo (Choo et al. 2000) and Indonesia (Margono et al. 1979). In the Americas, A. braziliense has been reported in the southeast of the United States, extending up to Florida (23 °–31 °N) (Anderson et al. 2003) as well as Surinam (Rep and Heinemann, 1976), Brazil (Labarthe et al. 2004), Uruguay (Malgor et al. 1996) and Cuba (Prieto Fernandez et al. 1978). Why A. braziliense should be restricted to the southern tropics in Asia remains unknown, especially given the intermingling of human populations and their domestic animals, via trade routes and migrations that have occurred in the region. This is a fascinating question that may be due to, as yet, unidentified aspects of the biology of these two closely related ancylostomids in Asia, which are allopatric for a large part of their distribution ranges. Moreover, the apparent absence of A. ceylanicum from Australia despite the presence of A. braziliense and A. caninum in canids and felids and A. duodenale in humans also remains intriguing. Additional studies are required to determine the prevalence and host distribution of hookworms in wild and domesticated dogs and cats in Australia and Papua New Guinea. With the advent of rapid and highly specific PCR-based screening methods (Traub et al. 2004) for canine and feline hookworms directly from faeces, epidemiological studies can now be conducted with ease and accuracy to shed further light on this matter.
This study serves an important example of why detailed parasite morphology must accompany initial generation of molecular data for the diagnosis of nematodes. Once morphological characters have been validated by molecular studies, they provide a simple, cost-effective means of identifying parasites in field studies with a high level of sensitivity and specificity, particularly for those parasitic stages that are morphologically similar or identical (e.g. Echinococcus granulosus). However, caution must be utilized when interpreting molecular results for epidemiological studies without the support of conventional parasitological data.