INTRODUCTION
Trypanosoma cruzi is the aetiological agent of Chagas disease, also known as American trypanosomiasis. Chagas disease affects from 16 to 18 million people in the Americas, causing 21 000 deaths yearly and 40 million are at risk of infection throughout Central and South America (WHO, 2005). The life cycle of the parasite involves several stages of differentiation; in the mammalian host, the parasite has an obligate intracellular replicative form, the amastigote, and a non-replicative form, the bloodstream trypomastigote. The major forms present in the insect vector are also a replicative stage, the epimastigote, and a non-replicative stage, the infective metacyclic trypomastigote. The epimastigote form can be readily obtained in axenic culture, and has been used for this reason in most biochemical studies performed on the parasite (Cazzulo et al. Reference Cazzulo, Stoka and Turk2001).
Peptidases of microbial pathogens, including T. cruzi, have attracted the attention of many laboratories because of their roles in pathogenesis (Cazzulo et al. Reference Cazzulo, Stoka and Turk2001; Vermelho et al. Reference Vermelho, Giovanni-de-Simone, d'Avila-Levy, Santos, Melo, Silva, Bom and Branquinha2007), but less is known about the presence of a group of peptidases, the calpains. These enzymes constitute a large family of calcium-dependent cytosolic cysteine peptidases that have been characterized mainly in humans and that exist in 2 major isoforms, m-calpain and μ-calpain, which require millimolar and micromolar concentrations of Ca2+, respectively, for their activation. The role of calpain remains poorly understood, but it may participate in a variety of cellular processes, including the rearrangement of cytoskeletal proteins, different signal transduction pathways and apoptosis (Goll et al. Reference Goll, Thompson, Li, Wei and Cong2003). Calpain activation in humans seems to be increased during normal ageing and in muscular dystrophy, cataract, arthritis and Alzheimer's disease, and in many acute traumas. A variety of calpain inhibitors are under development and the potential clinical utility of these inhibitors has been shown to treat Alzheimer's disease (Battaglia et al. Reference Battaglia, Trinchese, Liu, Walter, Nixon and Arancio2003) and to minimize neuronal death after ischaemia (Hayes et al. Reference Hayes, Wang, Kampfl, Posmantur, Newcomb and Clifton1998).
The presence of large numbers of calpain-related proteins (CALPs) in trypanosomatids has been revealed by some groups, although there are no data as yet on the specific functions of the calpain-like proteins in these microorganisms (Hertz-Fowler et al. Reference Hertz-Fowler, Ersfeld and Gull2001; Ramos et al. Reference Ramos, Franco, Smith and Uliana2004; Ersfeld et al. Reference Ersfeld, Barraclough and Gull2005; Salotra et al. Reference Salotra, Duncan, Singh, Raju, Sreenivas and Nakhasi2006; Vergnes et al. Reference Vergnes, Gourbal, Gorard, Sundar, Drummelsmith and Ouellette2007; Andrade et al. Reference Andrade, Murta, Chapeaurouge, Perales, Nirdé and Romanha2008). Our group became involved in the study of these proteins though the purification of a proteolytically active CALP in the culture supernatant of the insect trypanosomatid Crithidia deanei, yet its function was not addressed (d'Avila-Levy et al. Reference d'Avila-Levy, Souza, Gomes, Vermelho and Branquinha2003). More recently, our group investigated the effect of calpain inhibitor III (MDL28170), a potent calpain inhibitor, on the growth of Leishmania amazonensis. This inhibitor promoted cellular alterations and arrested the cellular growth of the parasite, and a CALP was identified and detected on the cell surface of the flagellate (d'Avila-Levy et al. Reference d'Avila-Levy, Marinho, Santos, Martins, Santos and Branquinha2006a).
In this paper, we extended our studies on the presence of CALPs in trypanosomatids by investigating the inhibitory capability of MDL28170 against T. cruzi, aiming to show its effects on the growth and morphology of epimastigote forms. In addition, we have demonstrated the presence of calpain homologues in 3 distinct T. cruzi strains.
MATERIALS AND METHODS
Parasites and cultivation
Epimastigotes of Trypanosoma cruzi Dm28c (lineage TCII), Y (lineage TCI) and INPA 4167 (Z3 zymodeme) strains were grown in 3·7% brain heart infusion medium supplemented with 10% heat-inactivated fetal bovine serum at 28°C for 4 days to reach late-log phase growth. Alternatively, epimastigote forms of Dm28c were obtained from the differentiation of trypomastigotes after a blood passage in mice; in this case, epimastigotes were kept for no more than 3 passages in the axenic culture.
Effects of the calpain inhibitor on growth rate and cell morphology
The effects of calpain inhibitor III (MDL28170; Z-Val-Phe-CHO; purchased from Calbiochem, San Diego, CA, USA) on epimastigotes of T. cruzi were assessed by a method similar to that previously described elsewhere (d'Avila-Levy et al. Reference d'Avila-Levy, Marinho, Santos, Martins, Santos and Branquinha2006a). Briefly, epimastigotes were counted using a Neubauer chamber and resuspended in fresh medium to a final concentration of 5×106 viable epimastigotes per ml. The viability was assessed by mobility and lack of staining after challenging with trypan blue. MDL28170 was added to the culture at final concentrations in the 10–70 μM range (starting from a 5 mM solution in dimethylsulfoxide (DMSO) that was serially diluted in culture medium). A dilution of DMSO corresponding to that used to prepare the highest drug concentration was assessed in parallel. After incubation for 24, 48, 72 and 96 h at 28°C, the number of viable, motile epimastigotes was quantified by counting the flagellates in a Neubauer chamber. Alternatively, parasites grown for 72 h in the absence and in the presence of the calpain inhibitor were washed 5 times in cold phosphate-buffered saline (PBS; 150 mM NaCl, 20 mM phosphate buffer, pH 7·2) prior to resuspension in a drug-free fresh medium and allowed to grow for another 72 h, in order to evaluate the trypanocidal or trypanostatic effect. The number of live epimastigotes was evaluated as well as cell morphology (after Giemsa staining) under optical microscopy at 24-h intervals (Mendonça-Filho et al. Reference Mendonça-Filho, Rodrigues, Alviano, Santos, Soares, Alviano, Lopes and Rosa2004). The 50% inhibitory concentration (IC50) was evaluated after 72 h. This value was determined by linear regression analysis, by plotting the number of viable epimastigotes versus log drug concentration by use of Origin Pro 7.5 computer software.
Identification of CALPs by Western blotting
T. cruzi Dm28c cells were collected by centrifugation (500 g/5 min/4°C) and washed 3 times with cold PBS. Trypanosomes (108 cells) were resuspended in 100 μl of PBS and lysed by the addition of 1% SDS (Santos et al. Reference Santos, d'Avila-Levy, Dias, Ribeiro, Pereira, Elias, Souto-Padrón, Lopes, Alviano, Branquinha and Soares2006). The Drosophila extracts (kindly provided by Dr Alexandre Afranio Peixoto, FIOCRUZ, RJ, Brazil) were prepared in the extraction buffer (25 mM Hepes pH 7·2, 5 mM EDTA, 5 mM EGTA, 10 mM β-mercaptoethanol and 250 mM sucrose). About 10 frozen flies were crushed, suspended with 200 ml of cold extraction buffer and homogenized in a Teflon/glass homogenizer with 10–12 strokes at 4°C. The homogenate was carefully mixed and centrifuged at 100 000 g for 1 h at 4°C. The supernatant was stored at −80°C. Protein concentration was determined by the method described by Lowry et al. (Reference Lowry, Roseborough, Farr and Randall1951), using BSA as standard.
Immunoblot analysis was performed with total cellular extracts equivalent to 100 μg of protein, as previously described (d'Avila-Levy et al. Reference d'Avila-Levy, Araújo, Vermelho, Soares, Santos and Branquinha2005). The primary antibody used was a rabbit antiserum raised against Drosophila melanogaster calpain (anti-Dm-calpain; Emori and Saigo, Reference Emori and Saigo1994), at a dilution of 1:500. The secondary antibody used was peroxidase-conjugated goat anti-rabbit IgG at a dilution of 1:25 000 followed by chemiluminescence immunodetection after reaction with ECL reagents (d'Avila-Levy et al. Reference d'Avila-Levy, Araújo, Vermelho, Soares, Santos and Branquinha2005). The relative molecular mass of the reactive polypeptide was calculated by comparison with the mobility of SDS–PAGE standards.
Flow cytometry and immunofluorescence microscopy for calpain detection
Epimastigotes (1×107 cells) used for these experiments were fixed at 4°C in 0·4% paraformaldehyde in PBS (pH 7·2) for 30 min, followed by extensive washing in the same buffer. Alternatively, the fixed cells were permeabilized by 0·01% Triton X-100 in PBS for 15 min at room temperature and then washed twice in PBS. The fixed and permeabilized cells maintained their morphological integrity, as verified by optical microscopic observation. After this step, the cells were incubated for 1 h at room temperature with a 1:500 dilution of the following polyclonal antibodies: rabbit anti-Dm-calpain polyclonal antibody (Emori and Saigo, Reference Emori and Saigo1994); anti-C21, raised against the whole molecule of human brain m-calpain (Grynspan et al. Reference Grynspan, Griffin, Cataldo, Katayama and Nixon1997); and anti-CAP5.5, raised against the cytoskeleton-associated protein from Trypanosoma brucei (Hertz-Fowler et al. Reference Hertz-Fowler, Ersfeld and Gull2001). Cells were then incubated for an additional hour with a 1:200 dilution of fluorescein isothiocyanate (FITC)-labelled goat anti-rabbit IgG (d'Avila-Levy et al. Reference d'Avila-Levy, Dias, Nogueira de Melo, Martins, Lopes, Santos, Vermelho and Branquinha2006b). The cells were then washed 3 times in PBS and observed in a Zeiss epifluorescence microscope (Axioplan 2). Alternatively, the parasite-associated fluorescence was excited at 488 nm and quantified on a flow cytometer (FACSCalibur, BD Bioscience, USA) equipped with a 15 mW argon laser emitting at 488 nm. Non-treated cells and those treated with the secondary antibody alone were run in parallel as controls. Each experimental population was then mapped by using a two-parameter histogram of forward-angle light scatter versus side scatter. The mapped population (n=10 000) was then analysed for log green fluorescence by using a single-parameter histogram.
Effects of the calpain inhibitor on peptidase expression
Epimastigotes (5×106 cells) of Dm28c were incubated with MDL28170 at the IC50 concentration for 24 h at 28°C. Thereafter, cells were fixed and processed for flow cytometry analysis as previously described, employing a 1:500 dilution of the polyclonal antibodies anti-Dm-calpain and anti-cruzipain, the latter raised against the major cysteine peptidase of T. cruzi epimastigote forms (Cazzulo et al. Reference Cazzulo, Stoka and Turk2001).
Statistical analysis
All experiments were performed in triplicate, in 3 independent experimental sets. The data were analysed statistically by means of Student's t-test using EPI-INFO 6.04 (Database and Statistics Program for Public Health) computer software. P values of 0·05 or less were considered statistically significant.
Sequence data analysis
A search for Dm-calpain homologous proteins in T. cruzi was performed using the BlastP algorithm and the nr database at NCBI (GenBank). The theoretical molecular mass of homologous proteins was calculated using the ExPASy Server facilities (http://expasy.org). Identification of conserved domains was performed using the CD-Search Tool and Conserved Domain Database (CDD 27036 PSSMs) at NCBI.
RESULTS
The calpain inhibitor III (MDL28170) was added to T. cruzi clone Dm28c epimastigote forms in concentrations ranging from 20 to 70 μM, and the cellular growth was monitored for 4 days in vitro. Our results showed that MDL28170 arrested the growth in a dose-dependent manner (Fig. 1A). The lowest concentration of the drug (20 μM) presented a significant inhibitory effect only after 72 h of growth, but the remaining concentrations promoted reduced levels of growth that were statistically significant after 48 h (Fig. 1A). The calpain inhibitor at 50 μM decreased the growth rate by approximately 70–75% in the 48–96 h interval, and at 70 μM promoted a reduction in the cellular growth rate of around 91% after 48 h, and this ratio was also maintained up to 96 h of growth (Fig. 1A). Conversely, DMSO did not significantly affect the parasite growth behaviour (Fig. 1A). The same conditions performed for Dm28c were employed for the analysis of the effects of MDL28170 upon Y and INPA4167 strains, and similar profiles of growth inhibition were observed (data not shown). These strains were chosen to represent members of distinct T. cruzi lineages: TCI (Y strain), TCII (Dm28c) and Z3 zymodeme (INPA4167 strain). The IC50 was calculated for each strain, and similar values were detected: 31·7 μM (Dm28c), 34·3 μM (Y) and 37·4 μM (INPA4167).

Fig. 1. Effect of calpain inhibitor III (MDL28170) on the growth rate and cellular morphology of Trypanosoma cruzi Dm28c. The growth pattern of the parasite was followed at 28°C in the absence (control) or in the presence of MDL28170 (A) at concentrations ranging from 20 and 70 μM. The inhibitor was added to the cultures at 0 h, and the cells were counted daily. DMSO, used as the drug diluent, did not interfere with growth behaviour. Data shown are the mean of 3 independent experiments performed in triplicate. The dashed boxes highlight growth rates significantly different from control (P values ⩽0·05). (B) Microscopic observations of the viability of epimastigote forms when incubated in the absence or in the presence of MDL28170. Control cells were cultured in BHI medium (a). Cells cultured in the presence of MDL28170 at 20–70 μM showed similar morphological alterations (b-i).
The anti-trypanosomal activity of this inhibitor was reversible, since T. cruzi cells pre-treated for 72 h with the calpain inhibitor at 70 μM resumed growth when subcultured in a drug-free fresh medium (data not shown). Optical microscopy observations of T. cruzi Dm28c cells treated with the calpain inhibitor in the 20–70 μM range revealed, irrespective to the drug concentration, an increase in the cell volume, with the flagellates becoming round and some of them presenting no detectable flagellum (Fig. 1C, b–i), but no cell lysis was observed, corroborating the trypanostatic effect on the epimastigote growth. Cells cultured in the presence of DMSO at a dose used to dissolve the highest concentration of MDL28170 did not present any detectable morphological alterations (data not shown).
As demonstrated by flow cytometry and fluorescence microscopy analyses, the anti-Dm-calpain antibody was found to react with the cell surface of T. cruzi (Fig. 2A, B). In fixed/permeabilized cells, the antibody binding was stronger, being intensely detected in the whole cell body but not in the flagellum (Fig. 2A, B). In addition, a polypeptide band migrating at approximately 80 kDa was detected in the trypanosomatid cell lysate by cross-reactivity with the same antibody (Fig. 2A, inset). As a positive control, lysates of D. melanogaster cells presented a polypeptide with the same molecular mass that was recognized by anti-Dm-calpain antibody (Fig. 2A, inset).

Fig. 2. Detection of cross-reactivity between a calpain-like protein from Trypanosoma cruzi Dm28c and Drosophila melanogaster calpain (Dm-calpain). (A) Flow cytometric analysis showing the anti-Dm-calpain antibody binding to T. cruzi. Paraformaldehyde-fixed cells were permeabilized (p) or not (np) with Triton X-100 and then incubated in the absence (autofluorescence) or in the presence of anti-Dm-calpain antibody at 1:500 dilution, in order to be analysed by flow cytometry. For simplicity, only the autofluorescence of non-permeabilized cells is shown, since the permeabilized cells presented similar values (data not shown). When treated only with the secondary-FITC antibody, both permeabilized and non-permeabilized cells generated similar curves to that observed in the autofluorescence of cells (not shown). Representative data of the analysis of 10 000 cells from 1 of 3 experiments are shown. The inset shows the Western blotting analysis of polypeptides from T. cruzi (lane a) and D. melanogaster cell extract (lane b) probed with anti-Dm-calpain antibody. In lane c, the blotted proteins from T. cruzi were incubated only with the secondary-peroxidase antibody, and no reactive polypeptide was detected. The number on the left indicates the apparent molecular mass, expressed in kDa, of the reactive polypeptide. (B) Fluorescence microscopy showing the labelling of T. cruzi with anti-Dm-calpain antibody. Fixed cells (a–d) and fixed-permeabilized cells (e, f) were analysed under differential interferential contrast images (a, c, e) and immunofluorescence (b, d, f). Parasites treated only with the secondary antibody presented no fluorescence intensity (b). The bars represent 1 μm.
Since we cannot exclude the possibility that the anti-Dm-calpain polyclonal antibody may cross-react with proteins unrelated to CALPs in T. cruzi, a search for homologues of D. melanogaster CALP in T. cruzi genome was performed. The fragment of the D. melanogaster protein CAA55297.1 that was employed to generate the antibody used in this work (Emori and Saigo, Reference Emori and Saigo1994) was compared in a BlastP analysis with T. cruzi proteins found in GenBank data base (data not shown). The first 15 hits (homologues with e-value ranging from 2e-8 to 0·003) all corresponded to calcium-dependent cysteine peptidases and had their theoretical molecular mass determined, and 4 out of these 15 homologues presented a molecular mass around 80 kDa: XP_816697.1 (78·3 kDa), XP_803757.1 (80·8 kDa) kDa), XP_820102.1 (82·4 kDa) and XP_816696.1 (82·6 kDa). These 4 proteins presented only 2 conserved domains (cd00044 and pfam09149) and showed high similarity to each other, which indicates the possibility of being isoenzymes. The conserved domain cd00044 is shared with the fragment of the protein CAA55297.1, which supports the recognition of T. cruzi 80 kDa CALPS by the anti-Dm-calpain antibody.
Parasite cells, either grown in axenic cultures without passages in vivo or obtained after the differentiation of trypomastigotes recently isolated from infected mice, were processed for flow cytometric analysis using anti-Dm-calpain in order to estimate the influence of in vitro maintenance of T. cruzi Dm28c epimastigote forms upon the expression of CALPs. A decrease in the relative CALPs expression between newly isolated and in vitro-maintained cells did take place, as demonstrated in Fig. 3A. In addition, 2 anti-calpain antibodies with distinct specificities were also employed in order to detect the possible binding of different anti-calpain antibodies to calpain-like molecules of T. cruzi Dm28c: anti-C21, raised against the whole molecule of human brain m-calpain (Grynspan et al. Reference Grynspan, Griffin, Cataldo, Katayama and Nixon1997); and anti-CAP5.5, raised against the cytoskeleton-associated protein from T. brucei (Hertz-Fowler et al. Reference Hertz-Fowler, Ersfeld and Gull2001). In both cases, cells were fixed and permeabilized, or not, with Triton X-100. When anti-C21 antibodies were employed, there were no significant differences in labelling among fixed and permeabilized cells and the labelling itself is very close to the autofluorescence of cells, which indicates the lack of immunological similarity between human brain m-calpain and T. cruzi CALPs. Conversely, fixed/permeabilized T. cruzi cells showed an intense labelling with anti-CAP5.5; the antibody binding was drastically diminished when fixed cells were not permeabilized (Fig. 3B).
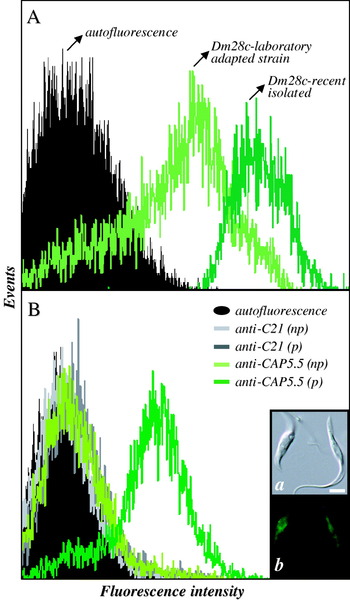
Fig. 3. Detection of binding of anti-calpain antibodies to Trypanosoma cruzi Dm28c epimastigote forms by flow cytometric analysis. (A) Cells regularly kept in BHI axenic culture (Dm28c-laboratory adapted strain) and cells obtained from the differentiation of trypomastigotes after a blood passage in mice (Dm28c-recent isolated) were fixed with paraformaldehyde and incubated in the absence (autofluorescence) or in the presence of anti-Dm-calpain antibody at 1:500 dilution. (B) Cells regularly kept in BHI axenic culture were fixed with paraformaldehyde, permeabilized (p) or not (np) with Triton X-100 and then incubated in the absence (autofluorescence) or in the presence of anti-C21 or anti-CAP5.5 antibodies at 1:500 dilution. Representative data of the analysis of 10 000 cells from 1 of 3 experiments are shown. The data from autofluorescence, fixed/permeabilized cells incubated with anti-C21 and fixed cells incubated with anti-CAP5.5 overlapped. The inset shows the fluorescence microscopy of T. cruzi permeabilized cells labelled with anti-CAP5.5 antibody. Note the fluorescence all over the cell body, but not in the flagellar region. The bar represents 1 μm.
A flow cytometric analysis was also performed in order to compare the relative levels of expression of CALPs in T. cruzi strains belonging to different phylogenetic lineages. To accomplish this, anti-Dm calpain antibodies were employed in fixed and/or permeabilized cells from members of each parasite lineage. In the 3 strains studied, the permeabilization with Triton X-100 raised significantly the mean of fluorescence intensity (MFI) values, which indicates that CALPs are located mainly intracellularly but also on the cell surface. In addition, distinct levels of expression of CALPs were detected: higher levels (twice as many) were found for Y strain in comparison to Dm28c, and a reduced labelling was found in INPA4167 strain (Fig. 4).

Fig. 4. The mean of the fluorescence intensity (MFI) calculated from the flow cytometric analysis of the anti-Dm-calpain antibody binding to Trypanosoma cruzi strains belonging to lineage TCI (Y strain), lineage TCII (Dm28c) and zymodeme Z3 (INPA4167 strain). Paraformaldehyde-fixed cells were permeabilized or not with Triton X-100 and incubated in the absence or in the presence of anti-Dm-calpain antibody at 1:500 dilution, and then analysed by flow cytometry. Representative data of the analysis of 10 000 cells from 1 of 3 experiments are shown. The asterisks highlight that MFI values from fixed and permeabilized cells are significantly different from each other (P values ⩽0·05). Note that the MFI was also distinct among each strain.
We also aimed to assess whether the MDL28170-treated cells of Dm28c presented any change in the levels of expression of CALPs and cruzipain in comparison to non-treated cells. Flow cytometry analyses revealed that both peptidases had their expression altered significantly when parasites were subjected to the presence of the calpain inhibitor: a reduction in the contents of CALPs was paralleled by an increased expression of cruzipain (Table 1).
Table 1. Detection of binding of anti-Dm-calpain and anti-cruzipain antibodies to untreated and MDL28170-treated Trypanosoma cruzi Dm28c epimastigote forms by flow cytometric analysis

DISCUSSION
In a previous study from our group, we showed that MDL28170, which is a potent, reversible and cell-permeable human calpain inhibitor (Rami et al. Reference Rami, Ferger and Krieglstein1997), arrested the growth of L. amazonensis in a dose-dependent manner (d'Avila-Levy et al. Reference d'Avila-Levy, Marinho, Santos, Martins, Santos and Branquinha2006a). In this paper, a similar approach was employed in order to explore the effects of this calpain inhibitor upon T. cruzi growth and to establish the differences amongst both trypanosomatids with respect to the parasites’ growth behaviour. Our results showed that the calpain inhibitor also arrested the growth of T. cruzi in a dose-dependent manner. When the IC50 values of MDL28170 for T. cruzi strains are compared to the calculated value for L. amazonensis, it is clear that the latter parasite, to which the IC50 value is 19 μM (d'Avila-Levy et al. Reference d'Avila-Levy, Marinho, Santos, Martins, Santos and Branquinha2006a), is more sensitive to MDL28170 than T. cruzi. These results are validated through the observation that the anti-trypanosomal activity of this inhibitor was reversible, while the anti-leishmanial activity of this compound at 25 μM is irreversible (d'Avila-Levy et al. Reference d'Avila-Levy, Marinho, Santos, Martins, Santos and Branquinha2006a). Similar optical microscopy observations (round-shaped cells) were described in other trypanosomatids when treated with cysteine peptidase inhibitors, due to osmotic stress caused by the peptidase inhibitor (Troeberg et al. Reference Troeberg, Morty, Pike, Lonsdale-Eccles, Palmer, McKerrow and Coetzer1999; Santos et al. Reference Santos, d'Avila-Levy, Dias, Ribeiro, Pereira, Elias, Souto-Padrón, Lopes, Alviano, Branquinha and Soares2006).
Although MDL28170 is a relatively specific calpain inhibitor, it cannot be ruled out that this inhibitor acts on other cysteine peptidases to a lesser extent, mainly cathepsins B (Rami et al. Reference Rami, Ferger and Krieglstein1997). Nevertheless, our results suggest that, as it occurs with L. amazonensis (d'Avila-Levy et al. Reference d'Avila-Levy, Marinho, Santos, Martins, Santos and Branquinha2006a), T. cruzi possesses molecules that share antigens with invertebrate calpain-related enzymes, which suggests that CALPs may be the major target of MDL28170. The cross-reactivity of anti-Dm calpain antibodies and trypanosomatid proteins was already detected in C. deanei extracellular calpain-like peptidase (d'Avila-Levy et al. Reference d'Avila-Levy, Souza, Gomes, Vermelho and Branquinha2003). Recently, our group has demonstrated that a CALP that cross-reacted with the same antibody was detected in both the cellular body and flagellum of Herpetomonas samuelpessoai promastigote cells, and its presence is enhanced in DMSO-induced paramastigote cells (Pereira et al. Reference Pereira, Elias, d'Avila-Levy, Branquinha and Santos2009). Based on sequence analyses, our study identified 4 T. cruzi CALPs of around 80 kDa that share the same conserved domain (cd00044) with the fragment of the protein CAA55297.1 that was employed to generate the anti-Dm-calpain antibody. For this reason, it is reasonable to presume that this antibody is capable of recognizing these 4 T. cruzi proteins. In this sense, it is already known that anti-Dm-calpain antibody does not recognize mammalian m-calpain and μ-calpain (Emori and Saigo, Reference Emori and Saigo1994). Recently, a member of the family of CALPs in T. cruzi was characterized (Giese et al. Reference Giese, Dallagiovanna, Marchini, Pavoni, Krieger and Goldenberg2008). The gene encoding TcCALPx11 corresponds to the protein XP_816697.1 and it is epimastigote-specific, being expressed mainly in epimastigotes subjected to the nutritional stress that precedes metacyclogenesis. No proteolytic activity was detected for this 80 kDa protein, and its differential expression may suggest that this protein may have a role in the T. cruzi stress response and/or in signal transduction.
In this study, we have also presented evidence that the maintenance of T. cruzi in axenic culture for a long time led to a decrease in the expression of CALPs. It has been reported that the long-term maintenance of T. cruzi cells in axenic cultures can select subpopulations less virulent than the parental strains, and the loss of virulence is associated with modification of the biological properties of this parasite that might lead to changes in the expression of some proteins (Contreras et al. Reference Contreras, Lima and Zorrilla1998). Although a direct relationship between the expression of CALPs and parasite virulence cannot be established, our results raise the interesting possibility that this correlation might occur. Simultaneously, the absence of cross-reactivity between CALPs in T. cruzi and anti-human m-calpain is suggestive of substantial structural differences among these molecules. In this sense, Ersfeld et al. (Reference Ersfeld, Barraclough and Gull2005) showed the presence of a large and diverse family of CALPs in T. brucei, Leishmania major and in T. cruzi displaying altered amino acid residues essential for catalytic activity and a moderate overall degree of sequence identity, which suggests that most CALPs do not have proteolytic activity. This large family exceeds the numbers found in most other organisms, which may point to organism-specific functions for these proteins.
Our results also indicate that CALPs in T. cruzi that cross-react with anti-CAP5.5 are located mainly in the intracellular milieu. CAP5.5 protein was the first characterized member of calpain-related genes in a trypanosomatid, specifically in T. brucei (Hertz-Fowler et al. Reference Hertz-Fowler, Ersfeld and Gull2001). This protein is characterized by the similarity to the catalytic region of calpain-type peptidases and it is detected exclusively in procyclic forms. CAP5.5 has been shown to be both myristoylated and palmitoylated, suggesting a stable interaction with the cell membrane through interactions with the underlying microtubule cytoskeleton as well. Interestingly, in our study the anti-CAP5.5 antibody labelled the whole cell body excluding the flagellum, as it occurs with T. brucei. Our results may indicate a possible correlation regarding the location of a subset of CALPs in both trypanosomes.
It is well established that many T. cruzi strains display a high level of biological divergence, which led to the development of many criteria for the grouping of these strains; early studies revealed substantial isozymic variability among isolates, defining 3 major groups or zymodemes – Z1, Z2 and Z3 (Miles et al. Reference Miles, Souza, Povoa, Shaw, Lainson and Toye1978). Subsequently, the use of DNA markers (Souto et al. Reference Souto, Fernandes, Macedo, Campbell and Zingales1996) proved the division of T. cruzi into 2 major phylogenetic lineages: lineage TCI (to which Z2 stocks belong) and lineage TCII (into which Z1 stocks fall). On the other hand, the position of Z3 zymodeme is still under review. In this sense, our results demonstrate a direct correlation between relative levels of CALP detection and T. cruzi strain classification, so it is possible that modulation in the expression of these proteins does take place. Nevertheless, the lineage-associated differences in CALPs expression must be confirmed by the use of a larger number of strains.
Fluctuations of the expression levels of CALPs were also observed when Dm28c cells were treated for 24 h with MDL28170 at IC50: reduced labelling was found in treated cells, and a simultaneous increase in cruzipain expression was detected. Cruzipain is the major cysteine peptidase in T. cruzi, being expressed at different levels by distinct parasite stages, and much evidence points to its participation in penetration into the host cell, escape from the immune system and in the differentiation processes (Cazzulo et al. Reference Cazzulo, Stoka and Turk2001). The lethal effect of cruzipain inhibitors was due to inhibition of the auto-catalytic processing of the enzyme, leading to a decrease of its amount in lysosomes and its accumulation in the Golgi (Engel et al. Reference Engel, Doyle, Palmer, Hsieh, Bainton and McKerrow1998). Resistance of T. cruzi strains to cruzipain inhibitors is correlated with negligible levels of cruzipain activity and secretion of cruzipain precursors into the medium, with the concomitant upregulation of expression of a distinct 30 kDa cysteine peptidase (Engel et al. Reference Engel, Torres, Hsieh, Doyle and McKerrow2000; Yong et al. Reference Yong, Schmitz, Vannier-Santos, Lima, Lalmanach, Juliano, Gauthier and Scharfstein2000). In this case, it was supposed that the metabolic needs for cruzipain may be partly compensated by the increased expression of the 30 kDa enzyme (Yong et al. Reference Yong, Schmitz, Vannier-Santos, Lima, Lalmanach, Juliano, Gauthier and Scharfstein2000). Interestingly, overexpression of cruzipain in T. cruzi transfectants has been associated with enhanced metacyclogenesis but not with increased infectivity (Tomas et al. Reference Tomas, Miles and Kelly1997). Collectively, these biochemical changes may demonstrate a correlation of the expression levels of cysteine peptidases in T. cruzi, and it is tempting to speculate that, as previously suggested by Yong et al. (Reference Yong, Schmitz, Vannier-Santos, Lima, Lalmanach, Juliano, Gauthier and Scharfstein2000), the overexpression of a certain cysteine peptidase may be necessary in order to titrate out the toxic levels of the inhibitor compound that is active against a different peptidase from the same class.
There are no data as yet on the specific functions of any CALPs in trypanosomatids. The investigation of changes in parasite gene expression between drug resistant/sensitive strains and in the up-regulation of virulence-related genes in infective forms has brought to the fore the involvement of calpain-like proteins in distinct processes in trypanosomatids (Salotra et al. Reference Salotra, Duncan, Singh, Raju, Sreenivas and Nakhasi2006; Vergnes et al. Reference Vergnes, Gourbal, Gorard, Sundar, Drummelsmith and Ouellette2007; Andrade et al. Reference Andrade, Murta, Chapeaurouge, Perales, Nirdé and Romanha2008). Life cycle-specific expression may also demarcate the search for specific functions of these CALPs (Saxena et al. Reference Saxena, Worthey, Yan, Leland, Stuart and Myler2003; Giese et al. Reference Giese, Dallagiovanna, Marchini, Pavoni, Krieger and Goldenberg2008). Altogether, the results presented herein may contribute to the investigation of the presence and the functions of CALPs in trypanosomatids. The global analyses concerning the functions of these CALPs may provide new understandings on the mechanisms that gave rise to such a diverse family of proteins in these organisms.
This study was supported by MCT/CNPq (Conselho Nacional de Desenvolvimento Científico e Tecnológico), FUJB (Fundação Universitária José Bonifácio), CEPG/UFRJ, FAPERJ (Fundação Carlos Chagas Filho de Amparo à Pesquisa do Estado do Rio de Janeiro) and Fundação Oswaldo Cruz (FIOCRUZ). The authors are grateful to Dr Yasufumi Emori (Department of Biophysics and Biochemistry, Faculty of Sciences, University of Tokyo, Japan) for donating the anti-Dm-calpain, Dr Ralph Nixon (Department of Psychiatry and Program in Neuroscience, Harvard Medical School, USA) for donating the anti-C21 antibody, Dr Keith Gull (School of Biological Sciences, University of Manchester, UK) for donating the anti-CAP5.5 antibody and Dr Juan Jose Cazzulo (Instituto de Investigaciones Biotecnologicas, Universidad Nacional de General San Martin, Buenos Aires, Argentina) for donating the anti-cruzipain. The authors wish to thank Ana Carolina de Siqueira Couto de Oliveira (IMPPG, UFRJ, Brazil) for her technical assistance in the flow cytometry analysis and Dr Adeilton A Brandão (FIOCRUZ, Rio de Janeiro, Brazil) for supplying the INPA4167 strain used in this study.