Introduction
Neglected tropical diseases (NTDs) are a group of 20 ailments that, despite showing high prevalence in different developing areas around the world, do not receive enough attention to be completely controlled and eliminated (WHO, 2017). Among the parasitic NTDs, Chagas disease (CD, aka American trypanosomiasis), caused by the intracellular protozoan pathogen Trypanosoma cruzi, is a vector-borne infection that is naturally transmitted by the contaminated feces of blood-sucking triatomine bugs. In fact, the parasite is mainly transmitted by these reduviid vectors along the 21 Latin American countries where CD is currently endemic (WHO, 2017). However, this classical vectorial route is not the only mechanism of T. cruzi transmission: outbreaks of orally-acquired infections, associated either to contaminated food or beverage, have also been reported in several regions of the endemic area (e.g. the Amazon Basin) (Shikanai-Yasuda and Carvalho, Reference Shikanai-Yasuda and Carvalho2012) and other alternative routes, such as the iatrogenic and the congenital one, have significantly contributed to both the spreading and the introduction of the parasitic disease in non-endemic countries, like Spain (Pinazo and Gascón, Reference Pinazo and Gascón2015). Currently, the epidemiological scenario of CD comprises about 6–7 million of infected individuals and over 75 million people living at risk worldwide (WHO, 2017).
As in other NTDs caused by kinetoplastid parasites (i.e. sleeping sickness and leishmaniases), the necessity of identifying novel treatment strategies for combating T. cruzi infection is still a reality (Patterson and Wyllie, Reference Patterson and Wyllie2014; Scarim et al., Reference Scarim, Jornada, Chelucci, de Almeida, dos Santos and Chin2018). Indeed, CD chemotherapy is currently based on two old nitroaromatic drugs: the 2-nitroimidazole beznidazole (BZ) and the 5-nitrofuran nifurtimox, which show important disadvantages related to their effectiveness in the later chronic stage of the disease, besides the wide range of side-effects they induce in patients (Urbina, Reference Urbina2018; Ribeiro et al., Reference Ribeiro, Dias, Paiva, Hagström-Bex, Nitz, Pratesi and Hecht2020). Presently, more than five decades after the introduction of these two medicines, a promising drug candidate for CD therapy is fexinidazole, another nitroheterocyclic compound that is the first all-oral treatment approved for both stages of Trypanosoma brucei gambiense human infection, the most common form of sleeping sickness (Patterson and Wyllie, Reference Patterson and Wyllie2014; WHO, 2019). According to these findings, it is noteworthy to point out that nitroheterocycles are still considered as a tempting group of molecules to be explored for the identification of novel CD treatments (Patterson and Fairlamb, Reference Patterson and Fairlamb2018). Several families of synthetic compounds that contain nitro groups in their scaffolds and also commercialized drugs identified by repurposing strategies, such as the 5-nitroimidazole metronidazole, have been recently proposed as putative anti-T. cruzi agents, according to the promising activity they achieved in different experimental models of the disease, both in vitro and in vivo (Simões-Silva et al., Reference Simões-Silva, de Araújo, Oliveira, Demarque, Peres, d'Almeida-Melo, Batista, da Silva, Cardoso-Santos, da Silva, Batista, Bahia and Soeiro2017; Thompson et al., Reference Thompson, Blaser, Palmer, Anderson, Shinde, Launay, Chatelain, Maes, Franzblau, Wan, Wang, Ma and Denny2017; Almeida et al., Reference Almeida, Ribeiro, Ferreira dos Santos, da Silva, Branquinho, de Lana, Gadelha and de Fátima2018; Arias et al., Reference Arias, Herrera, Garay, Rodrigues, Forastieri, Luna, Bürgi, Prieto, Iglesias, Cravero and Guerrero2018; Leite et al., Reference Leite, Fontes, Bastos, Hoelz, Bianco, de Oliveira, da Silva, da Silva, Batista, Nefertiti, Peres, Villar, Soeiro and Boechat2018; Palace-Berl et al., Reference Palace-Berl, Pasqualoto, Zingales, Moraes, Bury, Franco, da Silva Neto, Murayama, Nunes, Silva and Tavares2018; Chaves e Mello et al., Reference Chaves e Mello, Quaresma, Pitombeira, de Brito, Farias, de Castro, Salomão, de Carvalho, de Paula, Nascimento, Cupello, Paes, Boechat and Felzenszwalb2020). Nevertheless, toxicity issues are usually attributed to this kind of compounds (Patterson and Wyllie, Reference Patterson and Wyllie2014) and thus, it is mandatory to discard any potential risk associated to the presence of a nitro group in the antichagasic prototype.
In this context, our research group has identified various trypanocidal agents among several families of 5-nitroindazole derivatives. The most promising molecules of a synthetic series of 1,2-disubstituted 5-nitroindazolinones showed remarkable activity over the replicative forms (i.e. extracellular epimastigotes and intracellular amastigotes) of T. cruzi strains that are representative of different discrete typing units (DTUs): the drug-susceptible CL and Tulahuen (both, DTU TcVI) and the moderately drug-resistant Y (DTU TcII). These prototypes also registered no toxic effects on different mammalian cells, such as J774 macrophages, L929 fibroblasts and primary cultures of cardiac cells (CC) (Vega et al., Reference Vega, Rolón, Montero-Torres, Fonseca-Berzal, Escario, Gómez-Barrio, Gálvez, Marrero-Ponce and Arán2012; Fonseca-Berzal et al., Reference Fonseca-Berzal, da Silva, da Silva, Vasconcelos, Batista, Escario, Arán, Gómez-Barrio and Soeiro2015). Among them, 2-benzyl derivatives were identified as the best anti-T. cruzi compounds of this synthetic series (Vega et al., Reference Vega, Rolón, Montero-Torres, Fonseca-Berzal, Escario, Gómez-Barrio, Gálvez, Marrero-Ponce and Arán2012). Several of these 1-substituted 2-benzyl-5-nitroindazolinones also induced important reductions in the parasitaemia levels of T. cruzi acute infected mice (Fonseca-Berzal et al., Reference Fonseca-Berzal, Escario, Arán and Gómez-Barrio2014). According to these results, other series of 5-nitroindazole derivatives were subsequently synthesized by our research group, all of them supporting a benzyl moiety at the N-2 position of the indazole scaffold, i.e. 3-alkoxy-2-benzyl-5-nitro-2H-indazoles and 1-alkyl-2-benzyl-5-nitroindazolin-3-ones. Among these two series, we have recently identified several molecules that show great selectivity against the causative agent of CD (Fonseca-Berzal et al., Reference Fonseca-Berzal, Ibáñez-Escribano, Reviriego, Cumella, Morales, Jagerovic, Nogal-Ruiz, Escario, da Silva, Soeiro, Gómez-Barrio and Arán2016a, Reference Fonseca-Berzal, Ibáñez-Escribano, Vela, Cumella, Nogal-Ruiz, Escario, da Silva, Batista, Soeiro, Sifontes-Rodríguez, Meneses-Marcel, Gómez-Barrio and Arán2018).
In the present study, we evaluated the activity profile of two of the most selective compounds of the aforementioned series of 1-alkyl-2-benzyl-5-nitroindazolin-3-ones: 1-(2-aminoethyl) derivative 16 and 1-(2-acetoxyethyl) derivative 24 (Fig. 1), against T. cruzi parasites of Y strain (DTU TcII). Aiming to further explore the trypanocidal spectrum of these 5-nitroindazole derivatives, we assayed them in vitro over the three main parasite forms [i.e. epimastigotes, bloodstream trypomastigotes (BT) and intracellular amastigotes] of a T. cruzi strain that naturally shows moderate resistance to nitroderivatives (Romanha et al., Reference Romanha, de Castro, Soeiro, Lannes-Vieira, Ribeiro, Talvani, Bourdin, Blum, Olivieri, Zani, Spadafora, Chiari, Chatelain, Chaves, Calzada, Bustamante, Freitas-Junior, Romero, Bahia, Lotrowska, Soares, Andrade, Armstrong, Degrave and Andrade2010; Soeiro et al., Reference Soeiro, de Souza, da Silva, Batista, Batista, Pavão, de Araújo, Fortes Aiub, da Silva, Lionel, Britto, Kim, Sulikowski, Hargrove, Waterman and Lepesheva2013). Furthermore, these molecules were moved to in vivo mouse models of both acute toxicity and T. cruzi infection, to assess their effectiveness when administered through different routes, either as monotherapy or combined with the reference drug BZ.

Fig. 1. Chemical structures of the two 5-nitroindazole derivatives assayed: 1-(2-aminoethyl)-2-benzyl-5-nitro-1,2-dihydro-3H-indazol-3-one, hydrochloride (16) and 1-(2-acetoxyethyl)-2-benzyl-5-nitro-1,2-dihydro-3H-indazol-3-one (24).
Material and methods
Compounds
The synthesis of the two 5-nitroindazole derivatives studied in the present work (Fig. 1) was previously described (Fonseca-Berzal et al., Reference Fonseca-Berzal, Ibáñez-Escribano, Reviriego, Cumella, Morales, Jagerovic, Nogal-Ruiz, Escario, da Silva, Soeiro, Gómez-Barrio and Arán2016a, Reference Fonseca-Berzal, Ibáñez-Escribano, Vela, Cumella, Nogal-Ruiz, Escario, da Silva, Batista, Soeiro, Sifontes-Rodríguez, Meneses-Marcel, Gómez-Barrio and Arán2018). The numbering initially used for the designation of these molecules in their respective references has been maintained in the present paper, as follows: 1-(2-aminoethyl)-2-benzyl-5-nitro-1,2-dihydro-3H-indazol-3-one (hydrochloride), compound 16 (Fonseca-Berzal et al., Reference Fonseca-Berzal, Ibáñez-Escribano, Vela, Cumella, Nogal-Ruiz, Escario, da Silva, Batista, Soeiro, Sifontes-Rodríguez, Meneses-Marcel, Gómez-Barrio and Arán2018) and 1-(2-acetoxyethyl)-2-benzyl-5-nitro-1,2-dihydro-3H-indazol-3-one, compound 24 (Fonseca-Berzal et al., Reference Fonseca-Berzal, Ibáñez-Escribano, Reviriego, Cumella, Morales, Jagerovic, Nogal-Ruiz, Escario, da Silva, Soeiro, Gómez-Barrio and Arán2016a). For all the in vitro assays, stock solutions of 16, 24 and BZ – purchased from LAFEPE, Laboratório Farmacêutico do Estado de Pernambuco, Brazil – were prepared in dimethyl sulfoxide (DMSO) and extemporaneously added to the cultures in a final concentration of the solvent lower than 1% (v/v), which has no toxic effect on cultures of mammalian cells and parasites (Fonseca-Berzal et al., Reference Fonseca-Berzal, da Silva, da Silva, Vasconcelos, Batista, Escario, Arán, Gómez-Barrio and Soeiro2015). Regarding the in vivo experiments, compounds 16 and 24 were diluted in either 10% DMSO (v/v) or 20% Trappsol (w/v), both prepared in distilled and sterile water. For the administration of BZ, the reference drug was always dissolved in distilled and sterile water supplemented with 3% Tween 80 (v/v). All the final concentrations used for preparing these vehicles do not cause any detectable toxic effect (i.e. behaviour and clinical parameters) on mice (da Silva et al., Reference da Silva, Batista, de Araújo, Cunha-Júnior, Stephens, Banerjee, Farahat, Akay, Fisher, Boykin and Soeiro2017).
Mammalian cells
Primary cultures of embryonic CC were obtained from Swiss Webster mice as described in Meirelles et al. (Reference Meirelles, de Araújo-Jorge, Miranda, de Souza and Barbosa1986). Purified CC cultures were incubated at 37°C in a humidified atmosphere of 5% CO2 and sustained in Dulbecco's Modified Eagle's Medium (DMEM) without phenol red, supplemented with 2.5 mm CaCl2, 1 mm L-glutamine, 2% chicken embryo extract and 5% fetal bovine serum (FBS) previously inactivated by heating at 56°C for 30 min (Fonseca-Berzal et al., Reference Fonseca-Berzal, da Silva, da Silva, Vasconcelos, Batista, Escario, Arán, Gómez-Barrio and Soeiro2015).
Parasites
In this study, all the experiments were performed using T. cruzi Y strain (DTU TcII), which was initially isolated from an acute human case in Brazil and characterized by Silva and Nussenzweig (Reference Silva and Nussenzweig1953). Log-phase cultures of epimastigotes were continuously maintained at 28°C in Liver Infusion Tryptose (LIT) medium, supplemented as described in Vega et al. (Reference Vega, Rolón, Montero-Torres, Fonseca-Berzal, Escario, Gómez-Barrio, Gálvez, Marrero-Ponce and Arán2012). BT were obtained from acute infected Swiss Webster mice by performing heart puncture at the parasitaemia peak day and after being purified, BT were suspended in Roswell Park Memorial Institute (RPMI) medium supplemented with 5% heat-inactivated FBS (Fonseca-Berzal et al., Reference Fonseca-Berzal, da Silva, da Silva, Vasconcelos, Batista, Escario, Arán, Gómez-Barrio and Soeiro2015). Intracellular forms were obtained by the infection of CC with BT in a 1:10 ratio (cell:parasite), as previously depicted (Fonseca-Berzal et al., Reference Fonseca-Berzal, da Silva, da Silva, Vasconcelos, Batista, Escario, Arán, Gómez-Barrio and Soeiro2015; da Silva et al., Reference da Silva, Batista, de Araújo, Cunha-Júnior, Stephens, Banerjee, Farahat, Akay, Fisher, Boykin and Soeiro2017).
Animals
The use of outbred stock Swiss Webster male mice and the experimental procedures were in accordance with the Brazilian Law 11.794/2008 and the regulations of the National Council for the Control of Animal Experimentation (CONCEA). The mice were housed at a maximum of six individuals per cage, kept in a specific-pathogen-free (SPF) room at 20–22°C and 50–60% humidity under a 12/12 h light/dark cycle, and provided with sterilized water and chow ad libitum. The animals were adapted to these conditions for 1 week before starting the experiments. All animal experimental procedures were performed following the license L-038/2017 approved by the Ethics Committee for Animal Use of Instituto Oswaldo Cruz (CEUA/IOC).
In vitro experiments
Unspecific cytotoxicity on CC cultures
The toxicity profile of compounds 16 and 24 was determined over CC, by applying a colorimetric method in the presence of the redox indicator Prestoblue®. The assay was performed in 96-well plates previously coated with 0.01% of gelatin (Meirelles et al., Reference Meirelles, de Araújo-Jorge, Miranda, de Souza and Barbosa1986), and 100 μL of DMEM containing 6 × 104 cells were seeded per well. These plates were incubated overnight at 37°C with 5% CO2 and then, the medium was replaced by serial solutions of each compound in fresh DMEM. Cultures of CC were treated for 24 and 48 h under the same conditions of temperature and humidity, and controls of non-treated and BZ-treated cells included in every plate. After each time point, both CC morphology and contractibility were examined by light microscopy, and cell viability monitored by adding 10% Prestoblue® (v/v). The plates were finally incubated for 5 h under the conditions aforementioned and absorbance read at 570 and 600 nm. Each assay was performed separately three times (n = 3) and LC50 (i.e. concentration that induces 50% of cell lethality) values determined after both 24 and 48 h of compound exposure, by plotting drug concentrations vs percentages of cytotoxicity (%C) (Fonseca-Berzal et al., Reference Fonseca-Berzal, da Silva, da Silva, Vasconcelos, Batista, Escario, Arán, Gómez-Barrio and Soeiro2015; da Silva et al., Reference da Silva, Batista, de Araújo, Cunha-Júnior, Stephens, Banerjee, Farahat, Akay, Fisher, Boykin and Soeiro2017).
Epimastigote susceptibility assay
The activity against epimastigotes was determined by applying the spectrofluorimetric method standardized by Rolón et al. (Reference Rolón, Vega, Escario and Gómez-Barrio2006). In a 96-well microplate, cultures of log-phase epimastigotes in LIT medium (3 × 106 epimastigotes mL−1) were seeded by adding 200 μL per well. The parasites were exposed to serial solutions of compounds 16 and 24 for 48 h at 28°C, and controls of non-treated and BZ-treated epimastigotes included in each assay. Finally, 10% resazurin solution (3 mm in PBS, pH 7.0) was added (v/v) and after incubating 5 h at 28°C, fluorescent intensity read at 535 (excitation) and 590 nm (emission) wavelengths. Each assay was run similarly for three (n = 3) and both IC50 and IC90 (i.e. concentration that inhibits 50 and 90% of epimastigote growth, respectively) estimated by plotting drug concentrations vs percentages of parasite growth inhibition (%PGI). For each compound, selectivity index (SI) on epimastigotes was calculated, as follows: SIEPI = LC50/IC50, where LC50 corresponds to the value obtained after 48 h of CC treatment.
BT susceptibility assay
This assay was performed following the methodology described in previous works (Fonseca-Berzal et al., Reference Fonseca-Berzal, da Silva, Menna-Barreto, Batista, Escario, Arán, Gómez-Barrio and Soeiro2016b; da Silva et al., Reference da Silva, Batista, de Araújo, Cunha-Júnior, Stephens, Banerjee, Farahat, Akay, Fisher, Boykin and Soeiro2017). Briefly, a suspension containing 5 × 106 BT mL−1 in RPMI medium was distributed in a 96-well microplate by seeding 200 μL per well. Then, the parasites were incubated within serial solutions of compounds 16 and 24, for 24 h at 37°C and 5% CO2. Controls of non-treated and BZ-treated BT were included in each experiment. Finally, parasite death rates were determined with a light microscope, by directly counting the number of live BT in a Neubauer chamber. Each assay was run in similar conditions at least two times (n ⩾ 2) and both EC50 and EC90 (i.e. concentration that provokes 50 and 90% of parasite death, respectively) estimated by plotting drug concentrations vs percentages of parasite death (%PD). For each compound, SI on BT was also calculated, as follows: SIBT = LC50/EC50, where LC50 corresponds to the value obtained after 24 h of CC treatment.
Intracellular amastigote susceptibility assay
For this assay, 100,000 CC per well were seeded in a 24-well plate provided with rounded coverslips previously coated with 0.01% of gelatin and then, maintained in DMEM for 24 h at 37°C in a humidified atmosphere of 5% CO2. Afterwards, CC were infected with Y strain BT in a ratio 1:10 (cell:parasite) and this interaction was maintained for 24 h at 37°C with 5% CO2. These infected CC were washed to remove non-internalized parasites and then, incubated for 48 h within compounds 16 and 24 serially diluted in fresh medium. Controls of untreated infected CC were included in all the plates and BZ was tested in parallel. Finally, the infected CC were fixed with Bouin and stained with Giemsa and the trypanocidal activity determined by means of light microscopy, scoring the percentage of infected CC and the mean number of parasites per infected CC to estimate the endocytic index (EI). Each assay was run similarly at least two times (n ⩾ 2) and both IC50 and IC90 (i.e. concentration that inhibits 50 and 90% of the EI, respectively) obtained by plotting drug concentrations vs percentages of EI inhibition (%EII). The respective SI on amastigotes was also estimated: SIAMA = LC50/IC50, where LC50 is the value obtained after 48 h of CC treatment (Fonseca-Berzal et al., Reference Fonseca-Berzal, da Silva, da Silva, Vasconcelos, Batista, Escario, Arán, Gómez-Barrio and Soeiro2015; da Silva et al., Reference da Silva, Batista, de Araújo, Cunha-Júnior, Stephens, Banerjee, Farahat, Akay, Fisher, Boykin and Soeiro2017).
In vivo experiments
Acute toxicity in mice
Acute toxicity levels of derivatives 16 and 24 were determined in male Swiss Webster mice weighing 28 ± 4 g. Escalating doses of both compounds were administered every 2 h to groups of two mice, either orally (p.o.) or intraperitoneally (ip), until reaching a final cumulative dose of 200 mg kg−1. A group of mice treated with the vehicle alone was included in each experiment, as control. Conform to the Organization for Economic Cooperation and Development (OECD) guidelines, animal acute toxicity was evaluated every 2 h for a 24 h period, and also 48 h post-treatment, in order to detect any toxic and/or sub-toxic (i.e. mobility, motor locomotion, tremors, convulsions or pain signals, such as prostration and both facial and corporal expression) symptoms. Moreover, chow and water consumption was observed and body weight monitored. The possible cardiotoxicity was evaluated by exploring the integrity of the electrical conduction system by electrocardiographic (ECG) traces analysis. After the complete observation period of 48 h, NOAEL (no-observed-adverse-effect level) was determined. At this endpoint time, the animals were euthanized and their organs (i.e. kidney, liver, heart and spleen) inspected to look for morphological abnormalities. Serum samples were also collected for conducting biochemical analyses in ICTB/FIOCRUZ, to detect possible alterations in the plasmatic levels of both alanine (ALT) and aspartate (AST) aminotransferases, creatinine and creatine kinase (CK), which are indicative markers of hepatic, renal and muscular injury, respectively. Each experiment was performed twice (n = 2) in similar conditions (Timm et al., Reference Timm, da Silva, Batista, da Silva, da Silva, Tidwell, Patrick, Jones, Bakunov, Bakunova and Soeiro2014; da Silva et al., Reference da Silva, Batista, de Araújo, Cunha-Júnior, Stephens, Banerjee, Farahat, Akay, Fisher, Boykin and Soeiro2017; Simões-Silva et al., Reference Simões-Silva, de Araújo, Oliveira, Demarque, Peres, d'Almeida-Melo, Batista, da Silva, Cardoso-Santos, da Silva, Batista, Bahia and Soeiro2017).
Effectiveness on a murine model of acute infection
Male Swiss Webster mice with an average weight of 22 ± 2 g were infected with 104 BT forms of T. cruzi Y strain inoculated via ip. In all the assays, only those animals with positive parasitaemia at 5th day post-infection (dpi), which corresponds to the parasitaemia onset in this model, composed the experimental groups. In a first study (n = 5 mice/group), for the purpose of evaluating the effectiveness of compounds 16 and 24 when prepared by using two different vehicles (i.e. 10% DMSO or 20% Trappsol) and administered to mice either p.o. or ip, the animals were randomly divided in groups, as follows: (i) non-infected and untreated (i.e. negative control), (ii) infected and untreated, receiving the vehicle alone (i.e. control of infection), (iii) infected and treated p.o. with 25 mg kg−1 of 16 in 10% DMSO, (iv) infected and treated ip with 25 mg kg−1 of 16 in 10% DMSO, (v) infected and treated p.o. with 25 mg kg−1 of 16 in 20% Trappsol, (vi) infected and treated ip with 25 mg kg−1 of 16 in 20% Trappsol, (vii) infected and treated p.o. with 25 mg kg−1 of 24 in 10% DMSO, (viii) infected and treated ip with 25 mg kg−1 of 24 in 10% DMSO, (ix) infected and treated p.o. with 25 mg kg−1 of 24 in 20% Trappsol, (x) infected and treated ip with 25 mg kg−1 of 24 in 20% Trappsol, (xi) infected and treated p.o. with a combination of 16 in 10% DMSO and BZ (both, 25 mg kg−1) and (xii) infected and treated p.o. with 25 mg kg−1 of BZ. For each therapeutic scheme, the mice received two unique doses of 0.1 mL at 5th and 8th dpi. A second study (n = 6 mice/group), was also carried out, in which the mice were randomly distributed in the following groups: (i) non-infected and untreated (i.e. negative control), (ii) infected and untreated, receiving the vehicle alone (i.e. control of infection), (iii) infected and treated with 25 mg kg−1 of 16, (iv) infected and treated with 25 mg kg−1 of 24, (v) infected and treated with a combination of 16 and BZ (both, 25 mg kg−1), (vi) infected and treated with a combination of 24 and BZ (both, 25 mg kg−1) and (vii) infected and treated with 25 mg kg−1 of BZ. In this case, compounds were always diluted in 10% DMSO and given to the mice in five consecutive daily doses of 0.1 mL p.o., beginning at 5th dpi. In both experiments and for evaluating the effectiveness of the aforementioned treatments, parasitaemia levels were individually checked, by directly counting the number of BT in 5 μL of blood taken from the tail of each mouse, following the method described by Brener (Reference Brener1962). Moreover, body weights were controlled weekly (i.e. ponderal curve analysis) and mortality rates monitored daily until the 30th day post-treatment and expressed as the percentage of cumulative mortality (%CM) (de Araújo et al., Reference de Araújo, da Silva, Batista, da Silva, Meuser, Aiub, da Silva, Araújo-Lima, Banerjee, Farahat, Stephens, Kumar, Boykin and Soeiro2014; Timm et al., Reference Timm, da Silva, Batista, da Silva, da Silva, Tidwell, Patrick, Jones, Bakunov, Bakunova and Soeiro2014; da Silva et al., Reference da Silva, Batista, de Araújo, Cunha-Júnior, Stephens, Banerjee, Farahat, Akay, Fisher, Boykin and Soeiro2017; Simões-Silva et al., Reference Simões-Silva, de Araújo, Oliveira, Demarque, Peres, d'Almeida-Melo, Batista, da Silva, Cardoso-Santos, da Silva, Batista, Bahia and Soeiro2017). Finally, the cardiac function of those surviving mice that progressed to the chronic stage of the infection was explored by the evaluation of ECG parameters: frequency, PR, QRS and QT intervals, as described in Campos et al. (Reference Campos, Hoppe, Duque, de Castro and Oliveira2016). In order to preserve animal welfare, daily observation was supervised by a PhD veterinary doctor aiming to avoid any suffering and pain as a result of parasite infection and/or compound administration. The mice were humanely euthanized whenever early endpoints of animal suffering became apparent, such as motor disturbance, exploratory activity and/or moribund condition.
Statistical analysis
SPSS Statistics Software (version 22, IBM) was used for the statistical analysis. The non-parametric Mann–Whitney U test was applied for comparing the activity and cytotoxic profile in vitro of both 5-nitroindazole derivatives 16 and 24 with those of the reference drug BZ. The same test was run for analysing the trypanocidal activity of these compounds among representative strains of DTUs TcII and TcVI. Moreover, the non-parametric Kruskal–Wallis test was used for conducting comparative studies among schemes assessed in the acute toxicity model. Regarding the effectiveness in vivo, the impact of these treatments over parasitaemia levels, body weight and cardiac function of T. cruzi-infected mice was analysed by applying one-way ANOVA, with the subsequent post-hoc analysis. In all the studies, P < 0.05 was considered statistically significant.
Results
In vitro experiments
Unspecific cytotoxicity on CC cultures
None of these 5-nitroindazole derivatives induced important cytotoxic effects over primary cultures of CC. After both 24 and 48 h of compounds exposure, neither morphology nor contractibility affectation was observed: only derivative 16 seemed to be slightly cytotoxic at the highest concentration tested (i.e. 200 μ m) after 48 h of incubation (%C = 46.45%). In fact, 16 and 24 showed LC50 values higher than 200 μ m at these two time points and cytotoxic profiles comparable to the one exhibited by the reference drug BZ (Supplementary Fig. S1).
Activity against epimastigotes
The activity of derivatives 16 and 24 over the extracellular and replicative form of T. cruzi is depicted in both Fig. 2 and Table 1. These compounds were considerably more active on Y strain epimastigotes than BZ (P < 0.05), showing IC50 values lower than 10 μ m and higher selectivity than the reference drug (Table 1). As it can be seen in Fig. 2, none of them achieved the IC90 value at the higher concentration tested. However, at 20 μ m both derivatives inhibited epimastigote growth in a percentage higher than that of the reference drug, showing %PGI of 87.29, 83.36 and 63.84% (i.e. derivatives 16, 24 and BZ, respectively). Among these two molecules, compound 16 displayed the most outstanding trypanocidal profile, with IC50 = 0.49 μ m (P < 0.05) and SIEPI > 408.16.

Fig. 2. Trypanocidal profile of 5-nitroindazole derivatives 16 (left), 24 (middle) and BZ (right) against Y strain epimastigotes (A), bloodstream trypomastigotes (B) and intracellular amastigotes (C).
Table 1. Activity of 5-nitroindazole derivatives 16 and 24 against epimastigotes, bloodstream trypomastigotes and intracellular amastigotes of T. cruzi Y strain, expressed as IC50 and IC90 (EC50 and EC90 for bloodstream trypomastigotes)

Results are expressed as the mean ± s.d. of at least two independent assays (n ⩾ 2). Selectivity indexes (SI) on each parasite form are also estimated.
ND, not determined.
*Significant differences (P < 0.05) with BZ, Mann–Whitney U test.
#Significant differences (P < 0.05) with the activity over a DTU TcVI strain (see Fonseca-Berzal et al., Reference Fonseca-Berzal, Ibáñez-Escribano, Reviriego, Cumella, Morales, Jagerovic, Nogal-Ruiz, Escario, da Silva, Soeiro, Gómez-Barrio and Arán2016a, Reference Fonseca-Berzal, Ibáñez-Escribano, Vela, Cumella, Nogal-Ruiz, Escario, da Silva, Batista, Soeiro, Sifontes-Rodríguez, Meneses-Marcel, Gómez-Barrio and Arán2018), Mann–Whitney U test.
Activity against BT
Regarding the activity on BT (Fig. 2 and Table 1) after 24 h of incubation at 37°C, compounds 16 and 24 did not have any impact over the extracellular and non-replicative form of T. cruzi, being both molecules less effective than BZ (EC50 = 7.43 μ m). Indeed, none of these 5-nitroindazole derivatives reached the EC50 at the concentrations tested (EC50 > 50 μ m) and thus, their SIBT could not be determined (ND).
Activity against intracellular amastigotes
Concerning the activity over intracellular amastigotes, both 5-nitroindazole derivatives exhibited outstanding trypanocidal profiles against the intracellular and replicative form of T. cruzi (Fig. 2 and Table 1). Certainly, these compounds were more active than the reference drug BZ, being derivative 16 the most promising one: IC50 = 0.41 μ m (P < 0.05), SIAMA > 487.80.
In vivo experiments
Acute toxicity in mice
As Supplementary Table S1 reflects, neither death nor apparent toxic effects were found in mice by clinical observation, as a consequence of the administration of derivatives 16 and 24. Certainly, compound 16 showed NOAEL > 200 mg kg−1 when it was given either p.o. or ip, and the same toxicity profile was observed with the oral administration of compound 24. However, some solubility problems were detected when this derivative was prepared in doses >100 mg kg−1. Accordingly, the administration of compound 24 via ip was interrupted after giving the third dose (i.e. 50 mg kg−1), reaching a cumulative dose of 100 mg kg−1 and thus, considering NOAEL > 100 mg kg−1. Furthermore, those mice that every 2 h received 0.1 mL of the vehicle alone (both, p.o. and ip) did not exhibit detectable side-effects too (data not shown).
Regarding the biochemical analysis, plasmatic levels of ALT, AST, creatinine and CK did not show significant alterations after the administration of derivatives 16 and 24 (Supplementary Table S2), pointing to the absence of any hepatic, renal and cardiac dysfunction, respectively. Similarly, those control mice that received the vehicle alone maintained these parameters unaltered (data not shown).
Finally, at the endpoint time (i.e. 48 h post-treatment), loss of body weight (Fig. 3A) and morphological alterations in kidney, liver, heart and spleen (Fig. 3B) were not registered in any group. Moreover, all these treated mice showed ECG profiles with frequencies, PR, QRS and QT intervals similar to those of the non-treated group, which indicated the absence of abnormalities in the cardiac function during the observation period. In fact, significant differences were not noticed when comparing the ECG profiles obtained 48 h post-treatment with those recorded before the administration of these compounds (Fig. 3C). As it can be seen in Fig. 3, all the parameters studied in the groups treated with derivatives 16 and 24, administered either p.o. or ip, were similar to those of the respective control group that received the vehicle alone.

Fig. 3. Variations in body weight (A), normalized organ weight (B) and electrocardiographic parameters (C) of male Swiss Webster mice 48 h after concluding the treatments with 5-nitroindazole derivatives 16 and 24. Bars represent the mean ± s.d. of the results obtained in two independent assays (n = 2). *Significant differences (P < 0.05) with the respective control group, Kruskal–Wallis. #Significant differences (P < 0.05) with the parameters observed at day 1, Kruskal–Wallis.
Effectiveness on a murine model of acute infection
Since none of these 5-nitroindazole derivatives induced acute toxicity effects when administered to mice either p.o. or ip, different therapeutic schemes of both 16 and 24 were tested in a mouse model of acute infection caused by T. cruzi. In a first experiment, the infected mice were treated with two unique doses of 25 mg kg−1, which were administered at 5th (i.e. parasitaemia onset) and 8th (i.e. parasitaemia peak) dpi. The results obtained with derivatives 16 and 24 are reflected in Figs 4 and 5, respectively.

Fig. 4. Parasitaemia levels (A) and mortality rates (B) of different groups of male Swiss Webster mice (n = 5) treated with two unique doses of the 5-nitroindazole derivative 16, administered by using two different vehicles (i.e. 10% DMSO and 20% Trappsol) and given at 5th and 8th dpi (black arrows), either p.o. or ip. Results are expressed as the mean ± s.d. Individual levels of parasitaemia at the peak day (i.e. 8th dpi) were included as an inset. *Significant differences (P < 0.05) with the untreated group, one-way ANOVA.
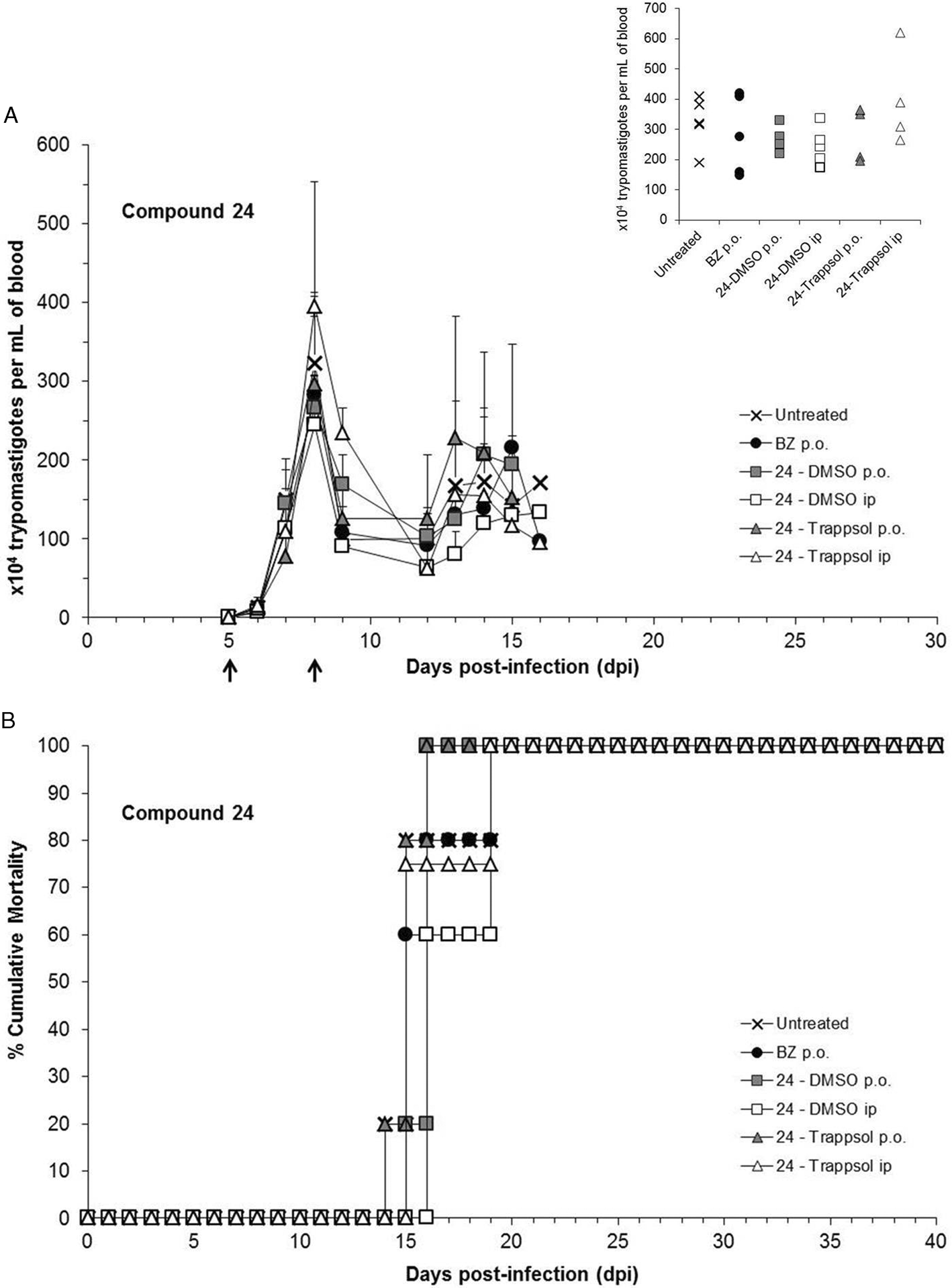
Fig. 5. Parasitaemia levels (A) and mortality rates (B) of different groups of male Swiss Webster mice (n = 5) treated with two unique doses of the 5-nitroindazole derivative 24, administered by using two different vehicles (i.e. 10% DMSO and 20% Trappsol) and given at 5th and 8th dpi (black arrows), either p.o. or ip. Results are expressed as the mean ± s.d. Individual levels of parasitaemia at the peak day (i.e. 8th dpi) were included as an inset. *Significant differences (P < 0.05) with the untreated group, one-way ANOVA. Note: n = 4 for the group treated with 24 – Trappsol ip, since one of the animals initially included had no positive parasitaemia at 5th dpi and then, it was excluded from the experiment.
As it can be seen in Fig. 4A, none of the treatments carried out with compound 16 suppressed the parasitaemia of acute infected mice. Focusing on the results obtained at the day of the parasitaemia peak (i.e. 8th dpi), the combined therapy of this 5-nitroindazole derivative with the reference drug was the only effective regimen, reducing the parasitaemia levels more successfully (ca. 30%) than the administration of BZ alone (i.e. 12.48%). However, this combination did not protect animals against the mortality triggered by T. cruzi infection, which in this group happened between 15th and 19th dpi (Fig. 4B). Moreover, the ponderal curves registered for all the infected animals showed lower rates than that of the group of non-infected mice, pointing to an evident impact of the infection over the body weight (Supplementary Fig. S2). Generally, all the infected mice suffered important loss of body weight during the second week post-infection (P < 0.05), being this fact undoubtedly connected with the beginning of mortality (Fig. 4B). However, the surviving mice – i.e. 20% (n = 1/5) of the animals belonging to the two groups orally treated – began to recover body weight during the fourth week post-infection, reaching at 28th dpi percentages of variation comparable to that of the non-infected group (Supplementary Fig. S2). Regarding the vehicles, the oral administration of derivative 16 led to similar profiles of effectiveness, mortality and variation of body weight, independently of the approach (i.e. 10% DMSO or 20% Trappsol). Such a behaviour was also observed when this treatment was given ip (Fig. 4 and Supplementary Fig. S2). Concerning the effectiveness of compound 24, the best parasitaemia reduction at the peak day was obtained by the dilution of this derivative in 10% DMSO (v/v), which reached 17.80 and 24.23% of reduction when administered p.o. and ip, respectively. Otherwise, this latter compound showed no trypanocidal effect when 20% Trappsol (w/v) was selected as a vehicle, since all the treated mice (both, p.o. and ip) exhibited parasitaemia levels at 8th dpi similar to that of the untreated group (Fig. 5A). Besides, none of the schemes carried out with derivative 24 neither induced protection against the mortality associated to the parasitic infection (Fig. 5B) nor prevented the significant weight loss (P < 0.05) ordinarily registered at 14th dpi for T. cruzi-infected animals (Supplementary Fig. S2).
These preliminary outcomes guided us to choose the most promising combination of each 5-nitroindazole derivative, vehicle and route, to be assayed in a subsequent experiment in which prolonged regimens of five consecutive doses of 25 mg kg−1 administered at 5–9th dpi were studied. Accordingly, 10% DMSO (v/v) and the oral administration were selected as the most suitable vehicle and route, respectively (Figs 4 and 5). As reflected in Fig. 6A, none of these treatments was able to induce parasitaemia suppression in T. cruzi-infected mice. However, after giving the first three doses, derivative 16 attained ca. 60% of reduction in the parasitaemia levels of the peak day (P < 0.05), being as effective as BZ. Otherwise, the effectiveness of derivative 24 did not improve with the application of this new regimen, since this treatment reached a minor parasitaemia reduction at 8th dpi (9.79%) and did not induce significant gains in the body weight of infected mice during the second week post-infection (Fig. 6A and Supplementary Fig. S3, respectively), unlike the reference drug (P < 0.05). Regarding the mortality associated to the infection, these monotherapies did not reduce death rates, since the groups of mice treated with compounds 16 and 24 showed 0 (n = 0/6) and 16.67% (n = 1/6) of survival, respectively (Fig. 6B). However, both 5-nitroindazole derivatives exhibited a positive impact over T. cruzi infection when they were co-administered with the reference drug (Fig. 6A): the parasitaemia peak decreased 79.24% when compound 16 was given in combination with BZ (P < 0.05) and 91.11% with the co-administration of derivative 24 and BZ (P < 0.05). These two activity profiles were better than the one obtained with the administration of BZ alone, which reduced 57.61% the parasitaemia of the peak day. These combined treatments also protected mice against mortality, which did not occur until the third week post-infection (i.e. 21st dpi): in combination with BZ, derivatives 16 and 24 yielded 83.33 (n = 5/6) and 66.67% (n = 4/6) of survival, respectively (Fig. 6B). Moreover, both groups of mice that received these combined therapies did not suffer weight loss during the second week post-infection (Supplementary Fig. S3), showing at 14th dpi healthier profiles than the one typically exhibited by infected and untreated mice (P < 0.05).

Fig. 6. Parasitaemia levels (A) and mortality rates (B) of different groups of male Swiss Webster mice (n = 6) treated with five consecutive doses (black arrows) of 5-nitroindazole derivatives 16 and 24, either in monotherapy or co-administered with BZ, vehiculized in 10% DMSO and given p.o. Results are expressed as the mean ± s.d. Individual levels of parasitaemia at the peak day (i.e. 8th dpi) were included as an inset. *Significant differences (P < 0.05) with the untreated group, one-way ANOVA.
Finally, the cardiac function of the animals that entered into the chronic stage of the infection was explored by the study of ECG parameters and their comparison with those of the non-infected mice (Fig. 7). It is noteworthy to point out that at 120th dpi, the percentages of survival of those mice treated with BZ, either alone or in combination with derivatives 16 or 24, were the same previously obtained at 40th dpi (Fig. 6B), not occurring any deaths during the 40–120th dpi period. Indeed, these infected and treated animals did not exhibit significant alterations in cardiac frequency, PR, QRS and QT intervals, when compared with the values shown by the group of non-infected mice (Fig. 7). Otherwise, these parameters could not be monitored for the group of infected and non-treated mice, since none of these animals overcame the acute experimental infection caused by T. cruzi (Fig. 6B).
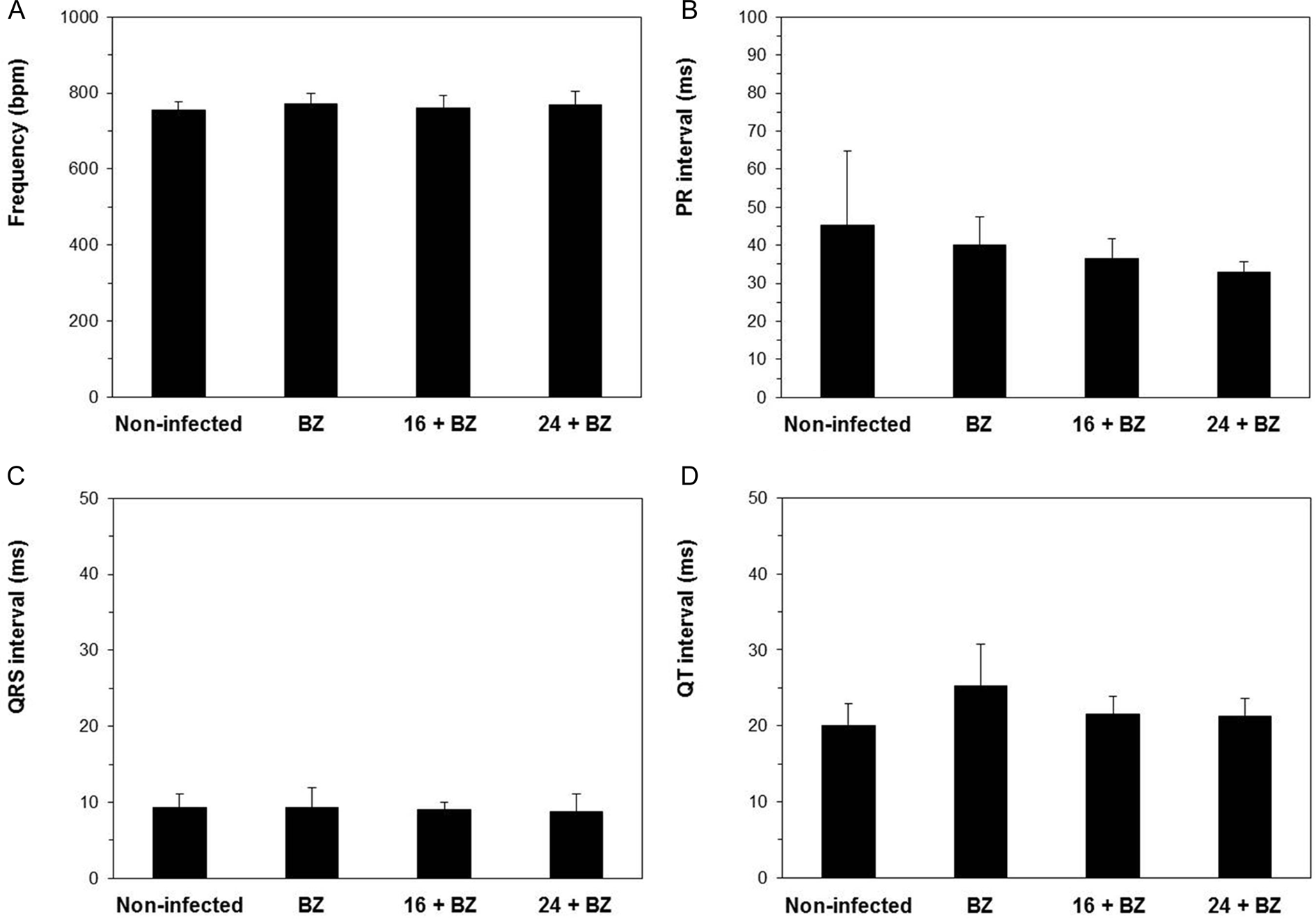
Fig. 7. Electrocardiographic parameters of the surviving mice obtained at 120th dpi: n = 6/6 (non-infected mice), n = 4/6 (BZ), n = 5/6 (compound 16 plus BZ) and n = 4/6 (compound 24 plus BZ). Frequency (A), PR (B), QRS (C) and QT (D) intervals. Bars represent the mean ± s.d. #Significant differences (P < 0.05) with the group of non-infected mice, one-way ANOVA.
Discussion
Trypanosoma cruzi population is currently divided into six DTUs (TcI–TcVI), according to their genetic background, geographical location, ecotopes and mammalian hosts distributed along the different endemic areas (Zingales et al., Reference Zingales, Miles, Moraes, Luquetti, Guhl, Schijman and Ribeiro2014). This high parasite diversity prompted researchers to investigate the association between the different T. cruzi strains and/or DTUs and drug susceptibility, since variable effectiveness of both reference antichagasic drugs occurs according to the geographical origin of patients (Coura and de Castro, Reference Coura and de Castro2002; Francisco et al., Reference Francisco, Jayawardhana, Lewis, Taylor and Kelly2017; Sales Junior et al., Reference Sales Junior, Molina, Murta, Sanchez-Montalvá, Salvador, Corrêa-Oliveira and Carneiro2017). In fact, DTU TcVI strains, such as CL Brener and Tulahuen, are representative drug-sensitive parasites, while those belonging to DTU TcI (e.g. Colombiana) are usually designated as high drug-resistant strains. Among these naturally resistant parasites, T. cruzi Y (DTU TcII) has been defined as a moderately drug-resistant strain, according to the medium resistance it displays to both nifurtimox and BZ (Romanha et al., Reference Romanha, de Castro, Soeiro, Lannes-Vieira, Ribeiro, Talvani, Bourdin, Blum, Olivieri, Zani, Spadafora, Chiari, Chatelain, Chaves, Calzada, Bustamante, Freitas-Junior, Romero, Bahia, Lotrowska, Soares, Andrade, Armstrong, Degrave and Andrade2010; Soeiro et al., Reference Soeiro, de Souza, da Silva, Batista, Batista, Pavão, de Araújo, Fortes Aiub, da Silva, Lionel, Britto, Kim, Sulikowski, Hargrove, Waterman and Lepesheva2013). Recent studies conducted by Zingales et al. (Reference Zingales, Araujo, Moreno, Franco, Aguiar, Nunes, Silva, Ienne, Machado and Brandão2015) and Petravicius et al. (Reference Petravicius, Costa-Martins, Silva, Reis-Cunha, Bartholomeu, Teixeira and Zingales2019) have pointed to the involvement of specific ATP-binding cassette (ABC) transporters in the natural resistance of T. cruzi to BZ, according to the over-expression of one ABCG-like protein in BZ-resistant strains (e.g. Colombiana, DTU TcI), unlike in BZ-susceptible ones, such as CL Brener (DTU TcVI). These authors have also proposed variations in the levels of type-I nitroreductases (i.e. NTR-1 and Old Yellow Enzime) among those mechanisms responsible for the different sensitivity of trypanosomatids to this reference drug (Petravicius et al., Reference Petravicius, Costa-Martins, Silva, Reis-Cunha, Bartholomeu, Teixeira and Zingales2019). However, a clear correlation between parasite DTUs and the presumable response of T. cruzi remains to be fully understood (Francisco et al., Reference Francisco, Jayawardhana, Lewis, Taylor and Kelly2017).
In previous works, our research group has introduced the 5-nitroindazole scaffold as a promising template for developing novel antichagasic compounds. Several 5-nitroindazole derivatives have demonstrated outstanding activity profiles, not only against drug-sensitive T. cruzi strains (i.e. CL Brener and Tulahuen), but also towards the moderately drug-resistant Y strain, either in vitro (Fonseca-Berzal et al., Reference Fonseca-Berzal, da Silva, da Silva, Vasconcelos, Batista, Escario, Arán, Gómez-Barrio and Soeiro2015, Reference Fonseca-Berzal, Ibáñez-Escribano, Reviriego, Cumella, Morales, Jagerovic, Nogal-Ruiz, Escario, da Silva, Soeiro, Gómez-Barrio and Arán2016a, Reference Fonseca-Berzal, Ibáñez-Escribano, Vela, Cumella, Nogal-Ruiz, Escario, da Silva, Batista, Soeiro, Sifontes-Rodríguez, Meneses-Marcel, Gómez-Barrio and Arán2018) or in vivo (Fonseca-Berzal et al., Reference Fonseca-Berzal, Escario, Arán and Gómez-Barrio2014, Reference Fonseca-Berzal, da Silva, Batista, Guedes-da-Silva, Vasconcelos, Demarque, Escario, Arán, Soeiro and Gómez-Barrio2017, Reference Fonseca-Berzal, da Silva, Batista, Guedes-da-Silva, Vasconcelos, Demarque, Escario, Arán, Gómez-Barrio and Soeiro2019a, Reference Fonseca-Berzal, da Silva, Batista, Guedes-da-Silva, Vasconcelos, Demarque, Escario, Arán, Gómez-Barrio and Soeiro2019b). Here, the effectiveness of two promising drug candidates, i.e. 5-nitroindazolinone derivatives 16 and 24, whose trypanocidal profiles in vitro against DTU TcVI parasites have been recently reported (Fonseca-Berzal et al., Reference Fonseca-Berzal, Ibáñez-Escribano, Reviriego, Cumella, Morales, Jagerovic, Nogal-Ruiz, Escario, da Silva, Soeiro, Gómez-Barrio and Arán2016a, Reference Fonseca-Berzal, Ibáñez-Escribano, Vela, Cumella, Nogal-Ruiz, Escario, da Silva, Batista, Soeiro, Sifontes-Rodríguez, Meneses-Marcel, Gómez-Barrio and Arán2018), were deeper explored upon T. cruzi using the naturally resistant parasite strain Y (i.e. DTU TcII) under distinct experimental models, both in vitro and in vivo. Accordingly, we followed those recommendations that point to the importance of testing experimental compounds over DTUs TcII and TcVI parasites at the initial stage of the screening (Don and Ioset, Reference Don and Ioset2014; Zingales et al., Reference Zingales, Miles, Moraes, Luquetti, Guhl, Schijman and Ribeiro2014), providing this way new evidences of the potential that these 5-nitroindazolinone derivatives possess as putative trypanocidal agents.
In this framework, we have previously identified 16 and 24 as promising anti-T. cruzi compounds, according to the great activity these molecules showed against both epimastigotes (IC50 = 0.35 and 5.43 μ m, respectively) and intracellular amastigotes (IC50 = 0.29 and 0.25 μ m, respectively) of a DTU TcVI strain (Fonseca-Berzal et al., Reference Fonseca-Berzal, Ibáñez-Escribano, Reviriego, Cumella, Morales, Jagerovic, Nogal-Ruiz, Escario, da Silva, Soeiro, Gómez-Barrio and Arán2016a, Reference Fonseca-Berzal, Ibáñez-Escribano, Vela, Cumella, Nogal-Ruiz, Escario, da Silva, Batista, Soeiro, Sifontes-Rodríguez, Meneses-Marcel, Gómez-Barrio and Arán2018). Interestingly, the trypanocidal profile that these derivatives displayed here over both replicative forms of this DTU TcII strain (Table 1) was quite similar, having no differences among the activity towards these two representative T. cruzi strains (i.e. CL Brener and Y). However, such an outstanding profile was not observed after treating in vitro the non-replicative form of T. cruzi (Table 1). This fact has also been detected with other molecules belonging to these two families of 5-nitroindazole derivatives (Fonseca-Berzal et al., Reference Fonseca-Berzal, Ibáñez-Escribano, Reviriego, Cumella, Morales, Jagerovic, Nogal-Ruiz, Escario, da Silva, Soeiro, Gómez-Barrio and Arán2016a, Reference Fonseca-Berzal, Ibáñez-Escribano, Vela, Cumella, Nogal-Ruiz, Escario, da Silva, Batista, Soeiro, Sifontes-Rodríguez, Meneses-Marcel, Gómez-Barrio and Arán2018). This particular behaviour has not been observed in 5-nitroindazole derivatives that are substituted at the positions 1 and 3 of the heterocyclic ring, which show similar anti-T. cruzi profiles in vitro against the two clinically relevant forms of the parasite (Muro et al., Reference Muro, Reviriego, Navarro, Marín, Ramírez-Macías, Rosales, Sánchez-Moreno and Arán2014; Martín-Escolano et al., Reference Martín-Escolano, Aguilera-Venegas, Marín, Martín-Montes, Martín-Escolano, Medina-Carmona, Arán and Sánchez-Moreno2018), being this fact also appreciated in other 1,3-disubstituted 5-nitroindazoles previously studied by us (data not published). Otherwise, substitutions at the positions 1 and 3 of the 5-nitroindazole scaffold do not usually lead to such a remarkable IC50 over amastigotes and thus, these derivatives are generally not as active as compounds 16 and 24 against intracellular T. cruzi forms (Muro et al., Reference Muro, Reviriego, Navarro, Marín, Ramírez-Macías, Rosales, Sánchez-Moreno and Arán2014; Martín-Escolano et al., Reference Martín-Escolano, Aguilera-Venegas, Marín, Martín-Montes, Martín-Escolano, Medina-Carmona, Arán and Sánchez-Moreno2018).
After confirming in vitro the outstanding activity of these molecules towards intracellular forms of Y strain, both 16 and 24 were moved to a mouse model of acute infection caused by this DTU TcII strain, in order to explore their effectiveness in vivo. Prior to these assays, acute toxic effects manifested as a consequence of their administration to mice were successfully discarded (Fig. 3 and Supplementary Tables S1 and S2). In this regard, several studies of acute toxicity previously conducted by us, have explored the suitability of 10% DMSO (v/v) to solubilize increasing doses of 5-nitroindazolinones (Fonseca-Berzal et al., Reference Fonseca-Berzal, da Silva, Batista, Guedes-da-Silva, Vasconcelos, Demarque, Escario, Arán, Soeiro and Gómez-Barrio2017, Reference Fonseca-Berzal, da Silva, Batista, Guedes-da-Silva, Vasconcelos, Demarque, Escario, Arán, Gómez-Barrio and Soeiro2019a, Reference Fonseca-Berzal, da Silva, Batista, Guedes-da-Silva, Vasconcelos, Demarque, Escario, Arán, Gómez-Barrio and Soeiro2019b). However, some solubility problems were detected when this vehicle was selected for administering low water-soluble derivatives (Fonseca-Berzal et al., Reference Fonseca-Berzal, da Silva, Batista, Guedes-da-Silva, Vasconcelos, Demarque, Escario, Arán, Soeiro and Gómez-Barrio2017). In order to avoid these difficulties, 20% Trappsol (w/v) was chosen here, aiming to improve drug solubility and to obtain a proper administration of compounds 16 and 24. The results obtained in these experiments (Fig. 3 and Supplementary Tables S1 and S2) corroborated the lack of toxicity previously registered in vitro over both L929 (Fonseca-Berzal et al., Reference Fonseca-Berzal, Ibáñez-Escribano, Reviriego, Cumella, Morales, Jagerovic, Nogal-Ruiz, Escario, da Silva, Soeiro, Gómez-Barrio and Arán2016a, Reference Fonseca-Berzal, Ibáñez-Escribano, Vela, Cumella, Nogal-Ruiz, Escario, da Silva, Batista, Soeiro, Sifontes-Rodríguez, Meneses-Marcel, Gómez-Barrio and Arán2018) and CC (Supplementary Fig. S1). In fact, none of the parameters recorded exhibited significant alterations after concluding the total observation period of 48 h, pointing to healthy profiles for all the experimental groups (Supplementary Tables S1 and S2). Also, the cardiological monitoring of the animals and therefore, any change noticed in their ECG waves and intervals is particularly important in this kind of toxicological studies (Botelho et al., Reference Botelho, Joviano-Santos, Santos-Miranda, Menezes-Filho, Soto-Blanco, Cruz, Guatimosim and Melo2019). Certainly, such alterations were not recorded after the administration of these derivatives, either p.o. or ip (Fig. 3) and the animals showed ECG parameters similar to those previously found for healthy Swiss Webster mice (Campos et al., Reference Campos, Hoppe, Duque, de Castro and Oliveira2016). According to these results, which indicated NOAEL > 200 mg kg−1 for both compounds – some solubility problems observed with compound 24 led to NOAEL > 100 mg kg−1 when given ip (Supplementary Table S1), different protocols were applied administering to T. cruzi-infected mice non-toxic doses of 25 mg kg−1, as mono- and combined therapy with BZ. The reference drug was also given in suboptimal doses of 25 mg kg−1, which correspond to 1/4 of the regimen standardized for this model of acute infection: BZ optimal doses of 100 mg kg−1 completely suppress the parasitaemia of T. cruzi Y-infected Swiss Webster mice, either with two unique administrations (de Araújo et al., Reference de Araújo, da Silva, Batista, da Silva, Meuser, Aiub, da Silva, Araújo-Lima, Banerjee, Farahat, Stephens, Kumar, Boykin and Soeiro2014; Timm et al., Reference Timm, da Silva, Batista, da Silva, da Silva, Tidwell, Patrick, Jones, Bakunov, Bakunova and Soeiro2014) or five consecutive daily doses (Fonseca-Berzal et al., Reference Fonseca-Berzal, Escario, Arán and Gómez-Barrio2014; Timm et al., Reference Timm, da Silva, Batista, da Silva, da Silva, Tidwell, Patrick, Jones, Bakunov, Bakunova and Soeiro2014; da Silva et al., Reference da Silva, Batista, de Araújo, Cunha-Júnior, Stephens, Banerjee, Farahat, Akay, Fisher, Boykin and Soeiro2017; Simões-Silva et al., Reference Simões-Silva, de Araújo, Oliveira, Demarque, Peres, d'Almeida-Melo, Batista, da Silva, Cardoso-Santos, da Silva, Batista, Bahia and Soeiro2017, Reference Simões-Silva, Peres, Britto, Cascabulho, Oliveira, da Gama, da Silva, da Costa, Araújo, Campos, Batista, Demarque, Moreira and Soeiro2019). According to this, the administration of both derivatives and BZ in such a low dosage allows better comparison among the impact of these experimental treatments over T. cruzi infection (Martín-Escolano et al., Reference Martín-Escolano, Aguilera-Venegas, Marín, Martín-Montes, Martín-Escolano, Medina-Carmona, Arán and Sánchez-Moreno2018). Indeed, trypanocidal effectiveness was not so relevant when 16 and 24 were given alone in two unique doses of 25 mg kg−1, as happened with the reference drug (Figs 4 and 5). However, important effects were evidenced when these derivatives were co-administered with BZ in a prolonged scheme of five consecutive daily doses of 25 mg kg−1 (Fig. 6). These combined treatments improved both the effectiveness and the survival rate observed when the respective compound (i.e. 16, 24 and also BZ) was given in monotherapy under the same regimen. Besides, CD commonly courses with important damages of the electrogenic cardiac system, being the ECG an important tool for the diagnosis and prognosis of the disease (Martinez et al., Reference Martinez, Perna, Perrone and Sosa Liprandi2019). In fact, atrioventricular blockage and arrhythmias are frequent disturbances detected also in T. cruzi-infected mice (Campos et al., Reference Campos, Hoppe, Duque, de Castro and Oliveira2016). Here, those infected and treated animals that overcame the acute infection showed ECG tracings similar to those of the non-infected mice (Fig. 7), suggesting that these combined therapies of derivatives 16 and 24 with BZ protected mice against this kind of cardiac alterations. Considering all the results obtained in vivo, these monotherapy protocols conducted with both 5-nitroindazole derivatives did not lead to better effectiveness than BZ; however, the co-administration schemes showed superior anti-parasitic profile than the reference drug. Recently, other pre-clinical studies have revealed important findings of trypanocidal effectiveness in vivo by co-administering different compounds with the reference drug BZ (Guedes-da-Silva et al., Reference Guedes-da-Silva, Batista, da Silva, Pavão, Batista, Moreira, Souza, Britto, Rachakonda, Villalta, Lepesheva and Soeiro2019; Simões-Silva et al., Reference Simões-Silva, Peres, Britto, Cascabulho, Oliveira, da Gama, da Silva, da Costa, Araújo, Campos, Batista, Demarque, Moreira and Soeiro2019). The implementation of combined therapies is an alternative actually considered for the treatment of CD (Sales Junior et al., Reference Sales Junior, Molina, Murta, Sanchez-Montalvá, Salvador, Corrêa-Oliveira and Carneiro2017; Ribeiro et al., Reference Ribeiro, Dias, Paiva, Hagström-Bex, Nitz, Pratesi and Hecht2020), and already in clinical use for combating many illnesses, including those triggered by parasitic infections, such as malaria (WHO, 2015) and sleeping sickness (WHO, 2019), among others.
Concluding remarks
The outcomes introduced in the present work corroborate the 5-nitroindazole scaffold as a promising template for developing novel anti-T. cruzi drugs. Concretely, 1-(2-aminoethyl)-2-benzyl-5-nitro-1,2-dihydro-3H-indazol-3-one (hydrochloride) – compound 16 – and 1-(2-acetoxyethyl)-2-benzyl-5-nitro-1,2-dihydro-3H-indazol-3-one – compound 24 – bore out remarkable selectivity in vitro on the replicative forms of the moderately drug-resistant T. cruzi Y strain (DTU TcII). Furthermore, the lack of toxic effects that both derivatives induced in vivo and the effectiveness obtained with subcurative doses of 25 mg kg−1, encourage our research group to consider higher doses and/or more prolonged therapeutic regimens for further investigating the suitability of both 5-nitroindazolinone derivatives as alternative trypanocidal agents, administered either as monotherapy or in combination with reference antichagasic drugs. Regarding the latter, our findings also reinforce the plausible use of drug co-administration for treating CD, as already used in other parasitic diseases. Finally, other works directed to evaluate ADMETox properties of these molecules are desirable and planned, aiming to explore both the pharmacokinetics and safety profile of these 5-nitroindazole derivatives.
Supplementary material
The supplementary material for this article can be found at https://doi.org/10.1017/S0031182020000955.
Acknowledgements
The authors thank the Program for Technological Development in Tools for Health (PDTIS-FIOCRUZ) for use of their facilities. C.F.-B. acknowledges M.N.C.S. and FIOCRUZ for the stay in Laboratório de Biologia Celular (LBC/IOC/FIOCRUZ).
Financial support
This work was supported by the Spanish Ministry of Economy, Industry and Competitiveness (MINEICO, ref. SAF2015-66690-R), the National Council for Scientific and Technological Development of Brazil (CNPq, ref. 301372/2015-2) and Fundação Carlos Chagas Filho de Amparo à Pesquisa do Estado do Rio de Janeiro (FAPERJ, ref. E02/2017). The 911120 UCM-CEI Moncloa Research Group ‘Epidemiología, Diagnóstico y Terapia Antiparasitaria’ and the Spanish Ministry of Science, Innovation and Universities (MICIU, ref. RTI-2018-093940-B-I00) are also acknowledged. M.N.C.S. is a research fellow of CNPq and CNE researcher.
Conflict of interest
None.
Ethical standards
The authors assert that all procedures contributing to this work comply with the ethical standards of the relevant national and institutional guides on the care and use of laboratory animals.