Introduction
The general formula of the pyrochlore-supergroup minerals (Atencio et al., Reference Atencio, Andrade, Christy, Gieré and Kartashov2010) is A 2–mB 2X 6–wY 1–n, where A typically is a large [8]-coordinated cation with a radius exceeding ~1.0 Å (Na, Ca, Ag, Mn, Sr, Ba, Fe2+, Pb2+, Sn2+, Sb3+, Bi3+, Y, Ce (and other lanthanides), Sc, U and Th), a vacancy (□), or H2O. B is a [6]-coordinated cation, typically of a high field-strength (Ta, Nb, Ti, Sb5+, W, V5+, Sn4+, Zr, Hf, Fe3+, Mg, Al and Si). X typically is O, but can include subordinate OH and F. Y typically is an anion (OH–, F– or O2–), but can also be a vacancy, H2O, or a very large (typically, with an ionic radius >1.3 Å) monovalent cation (K, Cs or Rb). The symbols m, w, and n represent parameters that indicate incomplete occupancy of the A, X and Y sites, respectively.
The pyrochlore supergroup comprises seven groups: betafite, coulsellite, elsmoreite, microlite, pyrochlore, ralstonite and roméite, depending on the nature of the predominant B cation (Ti, Mg, W, Ta, Nb, Al and Sb, respectively) and X anion (O2– in all groups except coulsellite and ralstonite, where the dominant anion is F–) (Atencio et al., Reference Atencio, Andrade, Bastos Neto and Pereira2017).
The names of the particular species are constructed from two prefixes and one root name (identical to the name of the group). The first prefix refers to the dominant anion (or cation) of the dominant valence (or H2O or □) at the Y site. The second prefix refers to the dominant cation of the dominant valence (or H2O or □) at the A site (Atencio et al., Reference Atencio, Andrade, Christy, Gieré and Kartashov2010). In the case of our new mineral, the Y site is dominated by O (the prefix is ‘oxy-’), the A site is dominated by Bi3+ (the prefix is ‘bismuto-’), and the B site is dominated by Ta, hence the root name is ‘microlite’. The whole combination leads, therefore, to the name oxybismutomicrolite.
The new mineral and its name were approved by the Commission on New Minerals, Nomenclature and Classification (CNMNC) of the International Mineralogical Association (IMA) (IMA2019-047, Kasatkin et al., Reference Kasatkin, Britvin, Peretyazhko, Chukanov, Škoda and Agakhanov2019). The type specimen is deposited in the collection of the Fersman Mineralogical Museum of the Russian Academy of Sciences, Moscow, Russia, with the registration number 5409/1.
The current IMA list of minerals (updated in November, 2019; http://cnmnc.main.jp/) includes nine valid species belonging to the microlite group. Three of them, hydroxykenomicrolite, oxystannomicrolite and oxystibiomicrolite have been redefined by Atencio et al. (Reference Atencio, Andrade, Christy, Gieré and Kartashov2010) from former species ‘cesstibtantite’, ‘sukulaite’ (= stannomicrolite) and ‘stibiomicrolite’, respectively, while six others were published as new species: fluorcalciomicrolite (Andrade et al., Reference Andrade, Atencio, Persiano and Ellena2013a), fluornatromicrolite (Witzke et al., Reference Witzke, Steins, Doering, Schuckmann, Wegner and Pöllmann2011), hydrokenomicrolite (Andrade et al., Reference Andrade, Atencio, Chukanov and Ellena2013b), hydroxycalciomicrolite (Andrade et al., Reference Andrade, Yang, Atencio, Downs, Chukanov, Lemée-Cailleau, Persiano, Goeta and Ellena2017), kenoplumbomicrolite (Atencio et al., Reference Atencio, Andrade, Bindi, Bonazzi, Zoppi, Stanley and Kristiansen2018) and oxynatromicrolite (Fan et al., Reference Fan, Ge, Li, Yu and Shen2016). Thus oxybismutomicrolite is the tenth member of the microlite group.
One more name, oxycalciomicrolite, is listed in the table of present members of the microlite group (Atencio et al., Reference Atencio, Andrade, Christy, Gieré and Kartashov2010) and has a ‘P’ status meaning ‘possible new species’ (Christy and Atencio, Reference Christy and Atencio2013). Published analyses corresponding to this mineral exist but its formal description has not yet been done.
Historical background
The name ‘bismutomicrolite’ was first introduced in the mineralogical literature by Zalashkova and Kukharchik (Reference Zalashkova and Kukharchik1957) applied to a “new variety of microlite” from an unspecified pegmatite vein in Altay Mts., Russia. This mineral was described as abundant roughly formed dark-green to emerald-green crystals up to 2 cm across embedded in light-purple scaly lepidolite masses. However, wet chemical analysis of this mineral gave only 3.25 wt.% of Bi2O3 (vs. 5.27 wt.% Na2O and 8.60 wt.% CaO) that resulted in 0.08 Bi atoms per formula unit (apfu) vs. 0.94 Na and 0.84 Ca apfu. According to the approved nomenclature of pyrochlore-supergroup minerals by Atencio et al. (Reference Atencio, Andrade, Christy, Gieré and Kartashov2010), this mineral should be considered as fluornatromicrolite.
Afterwards von Knorring and Mrose (Reference von Knorring and Mrose1963) reported on a new bismuth mineral ‘westgrenite’, (Bi,Ca)(Ta,Nb)2O6(OH), found as yellow, pink or brown veinlets that replaced bismutotantalite during late hydrothermal crystallisation of the lithium pegmatite at Wampewo Hill, Busiro County of Buganda, Uganda. Later on, ‘westgrenite’ was renamed by Hogarth (Reference Hogarth1977) and, in accordance with his classification of pyrochlore-group minerals, was given the name ‘bismutomicrolite’.
Thirty-three years later, ‘bismutomicrolite’ was discredited by Atencio et al. (Reference Atencio, Andrade, Christy, Gieré and Kartashov2010) because its published composition probably refers to a mixture. Indeed, regardless that some data reported by von Knorring and Mrose (Reference von Knorring and Mrose1963) (in particular, strongest X-ray lines and intensities and optical parameters), conform to microlite-group minerals, the chemical data given in this publication seem to be wrong. Though the constituent elements could correspond to a member of microlite group, our attempts to recalculate the chemical analysis in accordance with the general crystal-chemical formula of pyrochlore-supergroup minerals, completely failed. It is obvious that the material from Uganda needs to be restudied by modern analytical methods.
Christy and Atencio (Reference Christy and Atencio2013) confirmed the discredited status of ‘bismutomicrolite’ referring to the fact that analysed instances represent a zero-valent-dominant microlite-group mineral, or probable mixtures.
In particular, ‘westgrenite’ has also been described in a specimen from the Tromba pegmatite, Estado de Goiás, Brazil and studied by wet chemistry and powder X-ray diffraction (Erichsen de Oliveira et al., Reference Erichsen de Oliveira, Rocha Baptista and Baptista1970). It occurs there as dark-grey to black crystal fragments associated closely with native bismuth and bismutite. The recalculation of chemical data given in the cited paper following the normalisation constraints to Ta + Nb + Fe3+ = 2 pfu leads to the formula (Bi0.64Ca0.35)Σ0.99(Ta1.69Nb0.30Fe3+0.01)Σ2.00O5.81(OH)0.98. The current nomenclature (Atencio et al., Reference Atencio, Andrade, Christy, Gieré and Kartashov2010) assigns this composition to zero-valent-dominant microlite but just at the boundary with ‘hydroxybismutomicrolite’, a potentially new species. However, the authors of the cited paper on ‘westgrenite’ state that this mineral is intimately intergrown with native bismuth thus collected powder X-ray diffraction data include reflections of both species. This means that the Bi2O3 content in the chemical analysis is probably overestimated and, in fact, this mineral is even more obviously zero-valent-dominant microlite.
‘Bismutomicrolite’ from the beryl pegmatite #265 belonging to the Separation Rapids pegmatite group, NW Ontario, Canada was described by Tindle and Breaks (Reference Tindle and Breaks1998) as a product of the late-stage alteration of tungsteniferous wodginite and contains 9.63 wt.% of Bi2O3 leading to 0.233 Bi apfu only. The presence in the given chemical analysis of FeO (4.75 wt.%, or 0.373 apfu) and MnO (2.77 wt.%, or 0.220 apfu) clearly demonstrates that this material is, most likely, a mixture of zero-valent-dominant microlite with a wodginite-group mineral.
Pekov and Memetova (Reference Pekov and Memetova2008) reported on cation-deficient ‘bismutomicrolite’ from the Mikheevskaya vein at the Lipovka granite pegmatites, Middle Urals, Russia, where it forms a continuous solid-solution system with ‘plumbomicrolite’ and ‘uranmicrolite’ in rims of concentric-zoned crystals of ‘ordinary microlite’ (i.e. ‘calciomicrolite’ and ‘natromicrolite’). Despite the only given chemical analysis showing a formal dominance of Bi3+ as the dominant cation of the dominant valence at the A site, it is impossible to assign a particular species name to this mineral on the basis of the nomenclature of Atencio et al. (Reference Atencio, Andrade, Christy, Gieré and Kartashov2010) because of a low analytical total (89.74 wt.%) and the absence of analytical data for F– and H2O.
Zagorsky and Peretyazhko (Reference Zagorsky and Peretyazhko1992a) studied Bi-rich ‘microlites’ from various veins of the Malkhan pegmatite field, Central Transbaikalia, Russia and, in particular, reported on ‘bismutomicrolite’ from the Solnechnaya pegmatite vein. The empirical formula of this mineral calculated as an average of 38 different microprobe analyses, has been given as (Bi0.74Na0.88Ca0.31Sb0.03Pb0.01Mn0.05)Σ2.02(Ta1.25Nb0.18Ti0.45Sn0.12)Σ2.00(O6.38F0.62)Σ7.00. This composition, based on 29.57 wt.% of Bi2O3, still falls within the fluornatromicrolite field according to the nomenclature of Atencio et al. (Reference Atencio, Andrade, Christy, Gieré and Kartashov2010). However, the authors gave a maximal local Bi2O3 content of 39.29 wt.% in ‘microlites’ from the Solnechnaya vein which indicates the possible existence of a new, Bi-dominant member of the microlite group. One particular composition given by the authors and interpreted as ‘bismutobetafite’ in accordance with Hogarth (Reference Hogarth1977), shows Bi dominance at the A site: (Bi0.96Na0.49Ca0.40Mn0.09□0.06)Σ2.00(Ta1.05Nb0.16Ti0.69Sn0.10)Σ2.00(O6.38F0.44□0.18)Σ7.00. According to Atencio et al. (Reference Atencio, Andrade, Christy, Gieré and Kartashov2010) this composition might be attributed to ‘fluorbismutomicrolite’, another potentially new species with the end-member formula BiTa2O6F.
Excellent crystallinity of the unusually high-bismuth ‘microlites’ from the Malkhan pegmatite field (due to the almost complete absence of U and Th which are responsible for the radiation-induced damage and metamict state of many samples of pyrochlore-supergroup minerals) made it possible to investigate them in detail and to reinstate the status of ‘bismutomicrolite’ as a valid mineral species under the new name ‘oxybismutomicrolite’.
Occurrence
Oxybismutomicrolite was found in the Solnechnaya (‘Sunny’) pegmatite vein of the Malkhan pegmatite field, Krasnochikoyskiy District, Zabaykalskiy Kray, Central Transbaikalia, Russia. The Malkhan field of complex granitic miarolitic pegmatites (50°38′50″N, 109°55′07″E) is located in the south-western part of Zabaykalskiy Kray, approximately 320 km south-west of Chita, the regional capital, and 200 km south-east of Ulan-Ude, capital of neighbouring Republic of Buryatia (Fig. 1). The Malkhan pegmatite field was discovered in the 1980s and is particularly famous for its world-class quality gem and collection tourmalines. It occupies an area of ~60 km2 on the southern slopes of the Malkhan ridge and contains more than 300 pegmatite veins (Fig. 2). The Malkhan pegmatite field has been dated radiometrically by the 40Ar/39Ar method to 128–124 Ma (Zagorsky and Peretyazhko, Reference Zagorsky and Peretyazhko2010).

Fig. 1. Geographic location of the Malkhan pegmatite field.

Fig. 2. Geological map of the Malkhan pegmatite field (after Zagorsky and Peretyazhko, Reference Zagorsky and Peretyazhko1992a, with additions). 1 – Quarternary deposits (gravels, sands); 2 – non-dissected sequence of metapararocks of Malkhan series of Upper Proterozoic, PR2m (biotite, biotite-amphibole and amphibole schists); 3–8 metaorthorocks of Malkhan Lower Proterozoic complex, PZ1m: 3 – basic metagabbroids, 4 – less basic metagabbroids, metadiorites, 5 – quartz metadiorites, 6 – ultrametamorphic biotite leicogranites, 7 – amphibole-biotite granites (gneissic granites), 8 – biotite gneissic granites, aplite-like granites; 9–11 Mesozoic granites (MZ): 9 – biotite porphyritic granites γ1, 10 – two-mica leicogranites γ22, 11 – dyke biotite two-mica granites and aplites γ3; 12 – pegmatite veins (bodies); red star – the Solnechnaya (“Sunny”) pegmatite vein; 13 – tectonic disturbances; 14 – facies contours.
The general survey of the Malkhan pegmatite field, its geology, types of pegmatites and their composition, geochemistry, mineralogy and formation conditions of pegmatite bodies are given in detail in many publications (e.g.: Peretyazhko, Reference Peretyazhko2009, Reference Peretyazhko2010; Peretyazhko et al., Reference Peretyazhko, Zagorskiy and Bobrov1989, Reference Peretyazhko, Zagorsky, Sapozhnikov, Bobrov and Rakcheev1992, Reference Peretyazhko, Prokof'ev, Zagorsky and Smirnov2000, Reference Peretyazhko, Zagorsky, Smirnov and Mikhailov2004; Peretyazhko and Zagorsky, Reference Peretyazhko and Zagorsky2002; Zagorsky, Reference Zagorsky2010, Reference Zagorsky2012, Reference Zagorsky2015; Zagorsky at al., Reference Zagorsky, Peretyazhko and Shmakin1999, Reference Zagorsky, Peretyazhko, Sapozhnikov, Zhukhlistov and Zvyagin2003, Reference Zagorsky, Peretyazhko and Dmitrieva2016; Zagorsky and Peretyazhko, Reference Zagorsky and Peretyazhko1992a,Reference Zagorsky and Peretyazhkob, Reference Zagorsky and Peretyazhko2006, Reference Zagorsky and Peretyazhko2008, Reference Zagorsky and Peretyazhko2010).
One of the characteristic features of several pegmatite bodies of the Malkhan field is their enrichment in bismuth. Tourmaline–lepidolite–albite complexes that have developed around miaroles contain up to 300 ppm of Bi in bulk. This results in the development of diverse Bi mineralisation in the pegmatites that includes native bismuth, bismuthinite, bismutite and tantalo–niobates such as bismutocolumbite (a new mineral species discovered in the Danburitovaya pegmatite vein, Peretyazhko et al., Reference Peretyazhko, Zagorsky, Sapozhnikov, Bobrov and Rakcheev1992), bismutotantalite and Bi-rich minerals of the pyrochlore supergroup. Moreover, Bi content in elbaite from the miaroles reaches 0.5 wt.% (Peretyazhko et al., Reference Peretyazhko, Zagorskiy and Bobrov1989).
The Solnechnaya pegmatite vein is located in the central part of the Malkhan field, in the valley of a right tributary of the small Skakunia river. It lies in quartz metadiorites of the Malkhan complex representing a cigar-shaped body 55 m long, up to 5–6 m in wide, running to the north-west (Fig. 3). The vein was uncovered by mining workings (ditches) Nos. 613 and 615 (Fig. 3a). Their mineral composition is given in Fig. 3b.

Fig. 3. Contour geological plan of the Solnechnaya (‘Sunny’) pegmatite vein (a), and distribution of normative minerals compositions by blocks and sections (b) of vein after Zagorsky and Peretyazhko, Reference Zagorsky and Peretyazhko1992a, with additions. 1 – metagabbroids – metadiorites (PZ1m), 2 – pegmatitic zones productive on tourmaline with miarole (black) and albite-lepidolite-elbaite complex, 3–10 – normative minerals: 3 – quartz, 4 – albite, 5 – anorthite, 6 – potassium feldspar, 7 – black tourmaline (schorl), 8 – garnet (almandine + spessartine), 9 – lepidolite, 10 – pollucite and accessory minerals; 11 – attitude elements of pegmatite vein contacts; 12 – mine workings (ditches). The procedure for calculating the normative minerals composition of pegmatite body by blocks and sections are given in Zagorsky and Peretyazhko, Reference Zagorsky and Peretyazhko1992a,Reference Zagorsky and Peretyazhkob.
On the flanks of the vein, the contacts with metadiorites are sharp and steep, whereas in the swell zone both (1) hanging and steep (80°); and (2) lying and sloping (20°) contacts are present. The vein has a distinctly zoned structure. The lying and sloping side is dominated by a coarse- and medium-grained oligoclase pegmatite with small blocky aggregates of potassic feldspar and rare inclusions of schorl and garnet (almandine–spessartine series). In the hanging and steep side, instead, potassic feldspar dominates over plagioclase. Large biotite plates up to 10 cm across are common at the contacts of all textural-paragenetical pegmatite units with the host metadiorite. The central part of the vein is composed of a K-feldspar graphic pegmatite with blocky aggregates of potassic feldspar and quartz up to 0.5–1 m across (Zagorsky and Peretyazhko, Reference Zagorsky and Peretyazhko1992a).
In 1985, the mining workings in the swell zone, at the north-western side of the vein, uncovered a big (3 m × 2.5 m × 1.5 m) miarolitic cavity. Walls of the cavity were overgrown by excellent druses of smoky quartz up to 50 cm × 25 cm, gemmy crystals of ‘watermelon’ tourmaline up to 18 cm × 15 cm and perfect crystals of cleavelandite and lepidolite. At 1–2 m distance from the miarole the potassic feldspar pegmatite is intensively albitised and transformed into albitite with inclusions of lepidolite, orange spessartine, topaz and pink beryl (var. vorobyevite). The coarse-grained albite–lepidolite–elbaite complex is developed here and there around the miarole. Numerous samples of that complex were collected by one of the authors (ISP) in September 1985, shortly after the opening of the cavity. Their study revealed the following paragenesis: native bismuth, bismutite, bismutotantalite, fluorapatite, fluorite, fluornatromicrolite, pollucite, stibiotantalite, topaz, xenotime-(Y), Hf-rich zircon and the new mineral described herein.
Physical, chemical and optical properties
Oxybismutomicrolite forms rough octahedral crystals up to 1 mm across and equant grains up to 2 mm across embedded in albite–lepidolite–elbaite matrix (Fig. 4). Smaller crystals and grains (<0.2 mm across) are homogenous and monomineralic whereas the majority of the bigger crystals show well-defined zonation with zones of Bi-rich fluornatromicrolite, well observed in back-scattered electron microscopy (Fig. 5). Other associated minerals are bismutotantalite and stibiotantalite. Oxybismutomicrolite is black. It is translucent in thin splinters and exhibits resinous lustre and a greyish-white streak. The new mineral is brittle, with uneven fracture. Cleavage and parting are not observed. It is non-fluorescent under ultraviolet light. The Vickers hardness (VHN100) is 609 kg/mm2 (range 551–689 kg/mm2, load 100 g, n = 5) corresponding to a Mohs hardness of ~5. Density measured by helium pycnometry is 6.98(2) g cm–3. Density calculated using the empirical formula and unit-cell volume determined from single-crystal X-ray diffraction data is equal to 7.056 g cm–3.

Fig. 4. Black crystals and grains of oxybismutomicrolite embedded in albite, with elbaite (photo by Anatoly Kasatkin). Specimen #265O.

Fig. 5. Scanning electron microscopy back-scattered electron image showing chemical zonation of oxybismutomicrolite (lighter zones) with Bi-rich fluornatromicrolite (darker zones). Specimen #290O.
The mineral does not react with cold hydrochloric and nitric acids.
Reflectance values for oxybismutomicrolite have been measured in air relative to a WTiC standard by means of a Universal Microspectrophotometer UMSP 50 (Opton-Zeiss, Germany). The reflectance values (R, %) are given in Table 1 and plotted in Fig. 6.
Table 1. Reflectance values for oxybismutomicrolite.

The reference wavelengths required by the Commission on Ore Microscopy (COM) are given in bold type.

Fig. 6. Reflectance spectrum of oxybismutomicrolite in air.
In reflected light, oxybismutomicrolite is light grey and isotropic. No internal reflections and pleochroism are observed.
The refractive index could not be measured because of the absence of corresponding immersion liquids. The mean n value, calculated from the Gladstone–Dale equation using the empirical formula and the calculated density, is 2.184.
Infrared spectroscopy
In order to obtain an infrared (IR) absorption spectrum (Fig. 7), a powdered oxybismutomicrolite sample was mixed with anhydrous KBr, pelletised, and analysed using an ALPHA FTIR spectrometer (Bruker Optics) at a resolution of 4 cm–1. Sixteen scans were collected. The IR spectrum of an analogous pellet of pure KBr was used as a reference.

Fig. 7. Powder IR absorption spectrum of oxybismutomicrolite.
The strong IR bands at 570 cm–1 (with the shoulders at 650 and 705 cm–1) and 375 cm–1 correspond to stretching and bending vibrations of the octahedral framework, respectively, and are typical for microlite-group minerals (Andrade et al., Reference Andrade, Atencio, Chukanov and Ellena2013b, Reference Andrade, Yang, Atencio, Downs, Chukanov, Lemée-Cailleau, Persiano, Goeta and Ellena2017; Chukanov, Reference Chukanov2014; Geisler et al., Reference Geisler, Berndt, Meyer, Pollok and Putnis2004). The shoulder at 880 cm–1 corresponds to a combination mode (a combination of stretching vibrations of the octahedral framework and lattice vibrations involving extra-framework cations). No bands are observed in the range 1000–3800 cm–1 which indicates the absence of H2O, OH–, CO3– and B-bearing groups in the mineral.
A high IR radiation scattering observed above 1200 cm–1 reflects a high refractive index of the mineral.
Chemical data
The chemical composition of the new mineral was determined using a Cameca SX-100 electron microprobe (wavelength dispersive spectroscopy mode, 15 kV, 20 nA and 5 μm beam diameter). A synthetic multi-layered pseudocrystal (W/Si layers, d = 60 Å; PC1 in Cameca terminology) was used as a reflector for the fluorine analysis. Prior to analysis, special care was taken to optimise the precise determination of peak maxima of the FKα line and background positions. An empirically determined correction factor was applied to the coincidence of 2nd-order of TaM ζ with the FK α line. Analytical data (average for seven spot analyses) are given in Table 2. Contents of other elements with atomic numbers >8 are below detection limits.
Table 2. Chemical composition of oxybismutomicrolite.

S.D. – Standard deviation
The empirical formula on the basis of (Ta + Ti + Nb + Sn) = 2 apfu is (Bi0.79Na0.68Ca0.32Mn0.03Pb0.02□0.16)Σ2.00(Ta1.42Ti0.30Nb0.21Sn0.07)Σ2.00O6.00(O0.52F0.38□0.10)Σ1.00. The simplified formula is (Bi,Na,Ca)2(Ta,Ti,Nb,Sn)2O6(O,F), based on Atencio et al. (Reference Atencio, Andrade, Christy, Gieré and Kartashov2010).
X-ray diffraction and crystal structure
Single-crystal X-ray studies were carried out using a Bruker Smart Apex II DUO diffractometer equipped with a CCD detector (MoKα radiation) and gave the following data: cubic, a = 10.4746(11) Å, V = 1149.2(4) Å3 (Z = 8) and space group Fd–3m.
Powder X-ray diffraction data (Table 3) were collected using a Rigaku RAXIS Rapid II diffractometer equipped with cylindrical image plate detector and rotating anode with microfocus optics (CoKα-radiation), using Debye-Scherrer geometry, at accelerating voltage of 40 kV, current of 15 mA and exposure time of 15 min. The distance between sample and detector was 127.4 mm. The image-plate data were processed using the ocs2xrd program (Britvin et al., Reference Britvin, Dolivo-Dobrovolsky and Krzhizhanovskaya2017). Diffraction peaks are perfectly indexed in the cubic unit cell, space group Fd–3m. The unit-cell parameters refined from the powder data (STOE WinXPOW v. 1.06 software, Stoe and Cie GmbH, Darmstadt, Germany) are: a = 10.4872(6) Å and V = 1153.40(12) Å3.
Table 3. Powder X-ray diffraction data (in Å for CoKα) for oxybismutomicrolite.
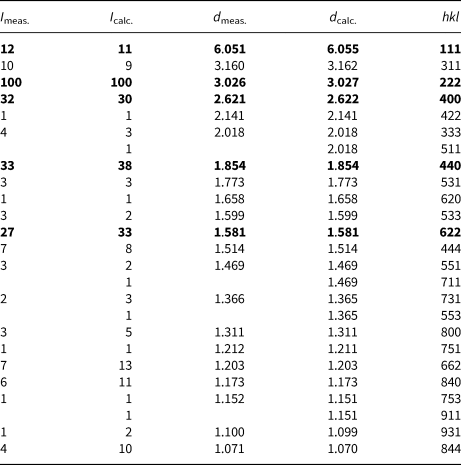
The strongest reflections are in bold.
The structure was solved by the dual space method using the SHELXL-2015 program (Sheldrick, Reference Sheldrick2015) and refined to R 1 = 0.019 on the basis of 104 independent reflections with I ≥ 2σ(I), using SHELXL-2015 and Olex2 programs (Sheldrick, Reference Sheldrick2015; Dolomanov et al., Reference Dolomanov, Bourhis, Gildea, Howard and Puschmann2009). For crystal parameters, data collection information and structure refinement details see Table 4. Fractional atomic coordinates, anisotropic and isotropic displacement parameters are given in Table 5; and selected interatomic bond distances are listed in Table 6. Complete crystallographic data and refinement parameters for oxybismutomicrolite can be retrieved from the crystallographic information files that have been deposited with the Principal Editor of Mineralogical Magazine and are available as Supplementary material (see below).
Table 4. Crystal parameters, data collection and structure refinement details for oxybismutomicrolite.

Table 5. Fractional atomic coordinates, anisotropic and isotropic displacement parameters (Å2) for oxybismutomicrolite.

Table 6. Interatomic bond distances (Å) for oxybismutomicrolite.

The crystal structure of oxybismutomicrolite (Fig. 8) belongs to a classic pyrochlore type with the ideal formula A 2B 2O 6Y (Atencio et al., Reference Atencio, Andrade, Christy, Gieré and Kartashov2010; Britvin et al., Reference Britvin, Siidra, Lotnyk, Krivovichev and Depmeier2010). It can be described as a three-dimensional framework built up of the two types of metal–oxygen (-fluorine) polyhedra (Fig. 8). The [TaO6] octahedra share common corners forming a perforated framework that bears wide channels propagated along [110] (Fig. 8b). The channels are occupied by Bi and its substituents along with O2– and F– ions. Alternatively, the structure can be represented as a pseudo-framework consisting of edge-sharing distorted hexagonal bipyramids [BiX 8] (X = O and F) where the Ta atoms reside in the cavities of the structure (Fig. 8c).

Fig. 8. Crystal structure of oxybismutomicrolite. (a) General view: a framework composed of interpenetrating [TaO6]- (green) and [(Bi,Na)X 8]-based (red) subunits (X = O, F). (b) The Ta-based subunit: a framework of corner-sharing [TaX 6] octahedra bearing wide channels occupied by (Bi, Na) (red) and F (yellow). (c) The Bi-based subunit: a pseudo-framework composed of edge-sharing distorted hexagonal bipyramids [(Bi,Na)X 8] (X = O or F). Ta atoms have been omitted for clarity.
Discussion
Pegmatites of the Malkhan field have a strong LCT signature (enriched in Li, Cs and Ta) in the sense of Černý and Ercit (Reference Černý and Ercit2005). As noted above, one of the specific geochemical features of several pegmatite bodies of the Malkhan field is their enrichment in bismuth which results in the development of unique Bi mineralisation and formation of primary aureoles of its dispersion.
The average content of Bi in the pegmatites increases with the increase of their productivity in gem tourmalines. Its strong positive correlation with that of Li, Sn, Be, sometimes with F, Cs and Rb suggests that Bi content in the pegmatite melt increases with increasing geochemical fractionation. Tantalum and niobium behave the same way. The transition from non-productive to low-productive and then to highly-productive pegmatite bodies is accompanied by an increase of the content of both Ta and Nb, but Ta content increases to a much higher extent. In the most productive zones of the veins like Solnechnaya, the Ta/Nb ratio is always above 1, sometimes reaching 5–7 (Zagorsky and Peretyazhko, Reference Zagorsky and Peretyazhko1992a). As a result, this vein is characterised by a strong predominance of Ta mineralisation over Ti–Nb leading to the appearance of bismutotantalite, stibiotantalite and various microlite-group minerals.
Variable Bi, Nb and Ta content and Ta/Nb ratio recorded at certain pegmatite bodies or their different textural–paragenetical units mirror the variable degree of the geochemical fractionation of the pegmatite melt from which the mineral association crystallised. The average content of Bi in the Solnechnaya vein is 31 ppm, which is the highest concentration for the Malkhan pegmatite field, in particular, two times higher than the next highest contents in the Zapadnaya-1 and Mechenaya veins (15 ppm for each). Average contents (in ppm) of Li (278), Sn (11), Be (30), Rb (284), Cs (261) and F (933) in the Solnechnaya pegmatite are also among the highest in the Malkhan pegmatite field (all the data are taken from Zagorsky and Peretyazhko, Reference Zagorsky and Peretyazhko1992a).
As the content of Bi in major rock-forming minerals of the pegmatite is low (3 ppm in quartz and 2–3 ppm in micas) to moderate (15 ppm in feldspars) [see Zagorsky and Peretyazhko, Reference Zagorsky and Peretyazhko1992a] and the pegmatite crystallisation propagates from the contact inwards, the intensive solidification of the less evolved pegmatite units resulted in a strong enrichment of Bi as well as in other incompatible elements including B, F, Li, Cs, Rb, Bi, Nb, Ta and Sn in a residual pegmatite melt. Very high and variable B2O3, and H2O contents in melt and fluid inclusions entrapped in quartz from different portions of several pegmatites documents an increasing content of the fluxing agents during the melt evolution and, moreover, formation of coexisting highly-fluxed aluminosilicate melt and hydrosaline fluids (Peretyazhko et al., Reference Peretyazhko, Zagorsky, Smirnov and Mikhailov2004; Thomas et al., Reference Thomas, Davidson and Badanina2012). Such highly fluxed liquids subsequently caused intensive metasomatic replacement of the solidified parts and formation of the albite–lepidolite–elbaite complex with miarolitic cavities (e.g. Zagorsky, Reference Zagorsky2015). Hence, the albite–lepidolite–elbaite complex represents one of the most fractionated parts of the pegmatite body and hosts disseminated accessory Bi-, Nb- and Ta-oxide minerals and the Bi content in elbaite reaches 5000 ppm (Zagorsky and Peretyazhko, Reference Zagorsky and Peretyazhko1992a).
Bismuth-rich microlite-group minerals have been discovered in several pegmatite bodies of the Malkhan field. For example, green ‘microlite’ from the Zapadnaya-1 vein contains up to 11.77 wt.% Bi2O3 (0.27 Bi apfu) and black ‘microlite’ from the Danburitovaya vein is even more Bi-rich, with the Bi2O3 content up to 13.31 wt.% (0.29 Bi apfu) (Zagorsky and Peretyazhko, Reference Zagorsky and Peretyazhko1992a). However, only in the Solnechnaya vein do concentrations of Bi2O3 in ‘microlites’ vary so widely that they can reach the quantities when Bi becomes a species-defining component.
One of the very important features of the chemistry of microlite-group minerals from the Solnechnaya vein is the total absence of OH-groups and H2O in their compositions, which has been confirmed by IR spectroscopy (see Zagorsky and Peretyazhko, Reference Zagorsky and Peretyazhko1992a; our data). This made it possible to assign a particular species name to each of the investigated ‘microlites’ on the basis of electron microprobe (EMP) analyses. Our EMP studies (42 analyses made on 10 different samples) show a high degree of heterovalent isomorphism, when Na+ and, to a lesser extent, Ca2+ are replaced by Bi3+ at the A site with the simultaneous substitution of F– by O2– at the Y site (Figs 9, 10). In the samples studied, Na or Bi are the dominant A cations whereas Ca is almost always third. Consequently, at the Solnechnaya vein we register a continuous solid-solution series between fluornatromicrolite and oxybismutomicrolite, but there are no compositions falling in the field of ‘calciomicrolite’ (Fig. 9).
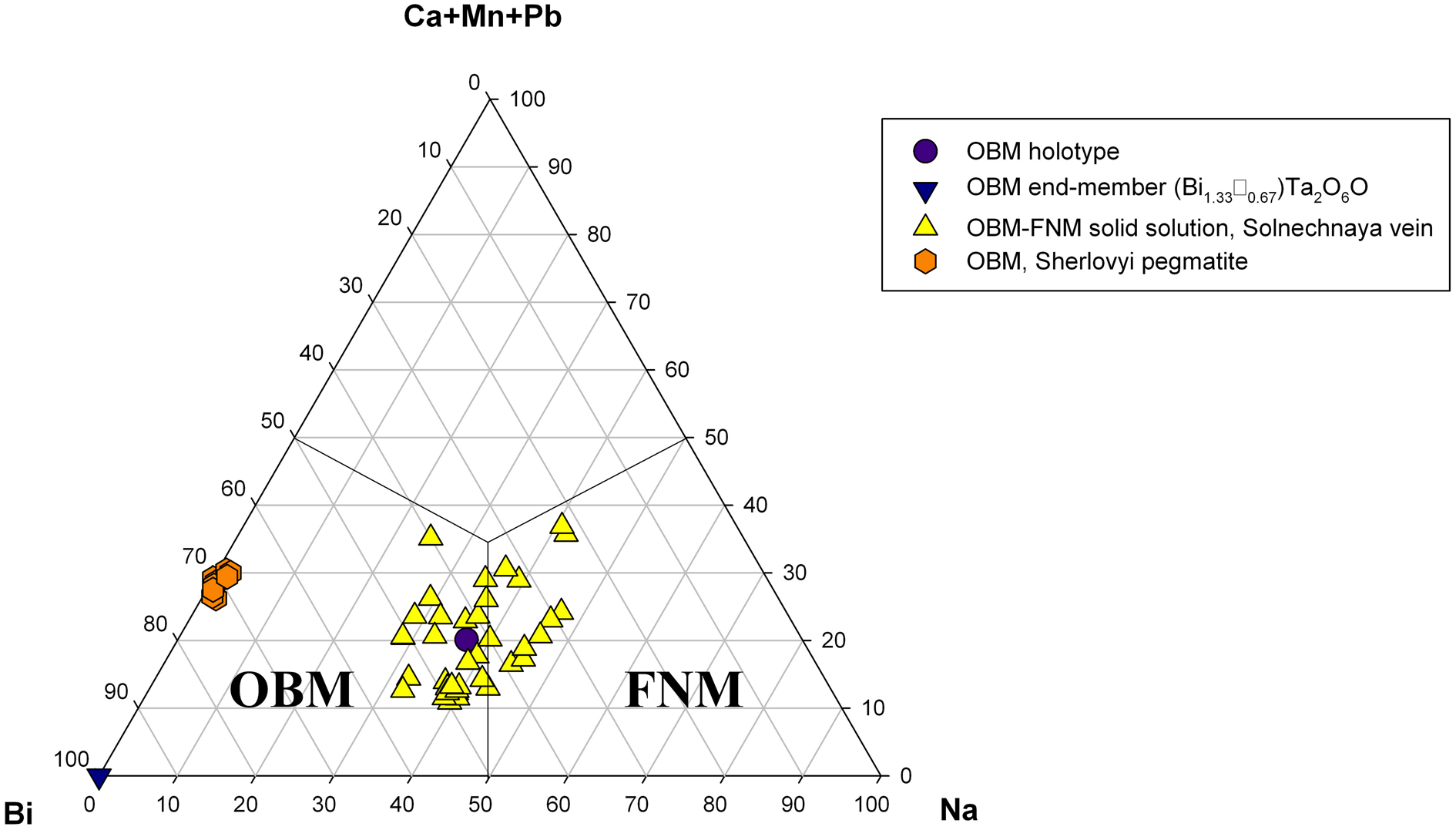
Fig. 9. Ternary plot showing the ratios of major cations at the A site in the microlite-group minerals from the Solnechnaya vein, the Malkhan pegmatite field and the Sherlovyi pegmatite, Southern Urals. OBM = oxybismutomicrolite and FNM = fluornatromicrolite.
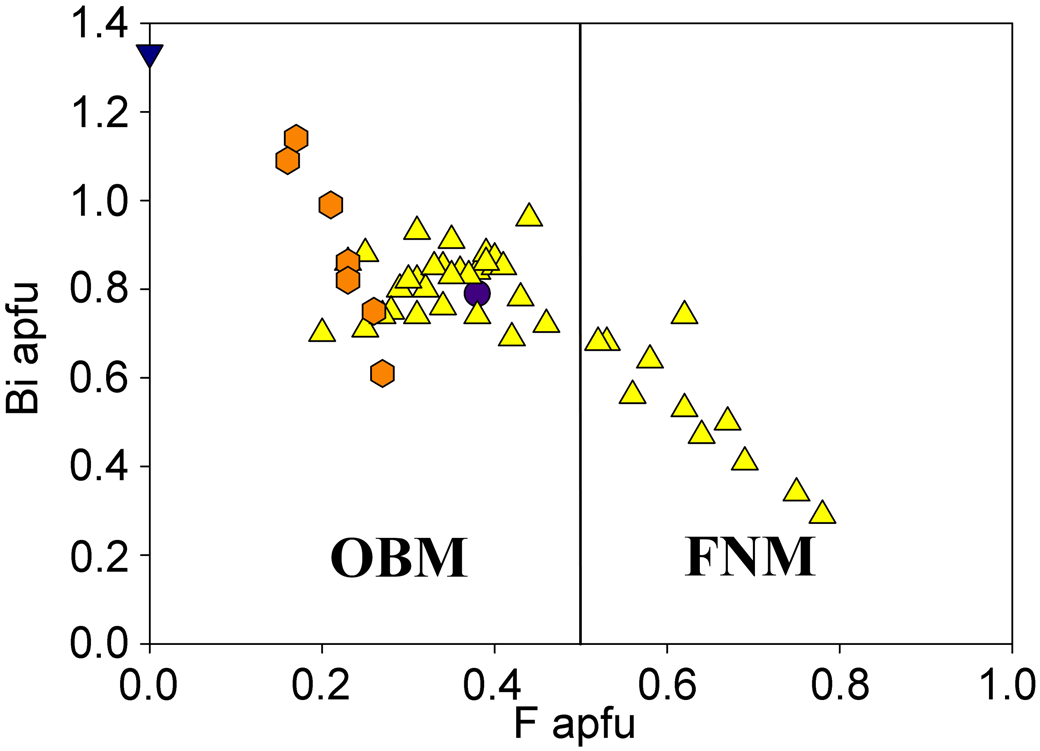
Fig. 10. Bi versus F in the microlite-group minerals from the Solnechnaya vein, the Malkhan pegmatite field, and the Sherlovyi pegmatite, S. Urals. Legends and abbreviations are the same as in Fig. 9.
As a rule, when F content falls below 0.5 apfu, Bi dominates over Na at the A site which corresponds to the transition from fluornatromicrolite to oxybismutomicrolite (Fig. 10).
Nevertheless, during our multiple EMP analyses we recorded two exotic compositions violating this rule:


The first of these compositions has both Na > Bi at the A site and O > F at the Y site. The nomenclature of Atencio et al. (Reference Atencio, Andrade, Christy, Gieré and Kartashov2010) would attribute this mineral to oxynatromicrolite. The second composition has Bi dominance at the A site but F > O at the Y site and, therefore, should be assigned to ‘fluorbismutomicrolite’, potentially a new mineral. As noted above, Zarorsky and Peretyazhko (Reference Zagorsky and Peretyazhko1992a) also reported one analysis corresponding to ‘fluorbismutomicrolite’ (they named it ‘bismutobetafite’), but we must admit that such analyses are unique and rather represent an exception from the whole compositional trend, making it extremely unlikely that a homogenous grain of this species suitable for its description as a new mineral could be found among the ‘microlites’ in the Solnechnaya vein.
An interesting matter of fact is that the isomorphic mechanism in the ‘microlites’ from the Zapadnaya-1 and Danburitovaya veins differs from the substitution scheme A(Na,Ca) + YF → ABi + YO noticed for the Solnechnaya vein. The compensation of the excessive positive charge due to the presence of Bi3+ at the A site is realised not in the anionic part, but at the B site where Ta5+ and Nb5+ are largely substituted by Sn4+ and Ti4+. The total atomic Sn4++ Ti4+ content in these ‘microlites’ is 2.5–3 times higher than in ‘microlites’ from the Solnechnaya vein, while O2– never dominates over F– at the Y site. Thus, the potential discovery of oxybismutomicrolite in these veins seems to be improbable.
Recently, a mineral corresponding in chemical composition to oxybismutomicrolite has been discovered by us in another Russian locality: Scherlovyi pegmatite near Taiginka Village, Kyshtym District, Chelyabinsk Oblast, Southern Urals (Kasatkin, Reference Kasatkin2019). It occurs as numerous dark-brown grains with resinous lustre, up to 2 mm across, embedded in granitic pegmatite. Associated minerals are beryl, bismuth, bismutocolumbite, cheralite, fluorapatite, fluor-schorl, gahnite, hercynite, magnetite, manganocolumbite, monazite-(Ce), quartz, rutile, spessartine, xenotime-(Y) and zircon. All chemical analyses (7) of oxybismutomicrolite from the Scherlovyi pegmatite are plotted in Figs 9 and 10. The average composition is (electron microprobe, wt.%): Na2O 0.04, K2O 0.04, CaO 0.80, MnO 2.61, BaO 0.16, PbO 1.20, Sb2O3 0.37, Bi2O3 32.35, ThO2 0.85, UO2 2.83, TiO2 8.22, SnO2 2.34, Nb2O5 4.38, Ta2O5 31.75, WO3 0.59, F 0.65, –O = F –0.27, total 88.91. The empirical formula on the basis of 2 cations at the B site is: (Bi0.94Mn0.25Ca0.10U4+0.07Pb0.04Sb3+0.02Th0.02Na0.01K0.01Ba0.01)Σ1.47(Ta0.97Ti0.69Nb0.22Sn0.10W0.02)Σ2.00O6.53F0.23. The low total and deficit of cations at the A site show the defectness of this ‘microlite’ and the presence of some quantities of non-structural absorbed water which may be the result of metamict state of the mineral due to substantial contents of UO2 and ThO2. This suggestion is confirmed by X-ray diffraction analysis: at room temperature the mineral is totally amorphous. Nevertheless, even with the absence of structural data and incompleteness of our chemical analyses (lack of information on measured H2O), it is obvious that the electroneutrality of the above empirical formula can be achieved only if O2– dominates at the Y site. Therefore, this mineral should be assigned to oxybismutomicrolite. As can be seen, it is richer in Bi and poorer in F than oxybismutomicrolite from the Solnechaya vein (Fig. 10). In addition, it is depleted in Na and Ca but contains essential Mn. Here again, our EMP analyses show a high degree of isomorphism, but its scheme is quite different: it occurs at the B site where Ta5+ is widely substituted by Ti4+. As a result, numerous compositions with Ti4+ + Sn4+ > Ta5+ + Nb5+ and Ti4+ > Sn4+ have been recorded which should be assigned to a potentially new species ‘oxybismutobetafite’. The decrease of the positive charge in this case is compensated by the growth of Bi content at the A site (up to 1.25 apfu) and respective increase of the analytical total (up to 99.58 wt.%) and occupancy of the A site (up to 1.78 apfu). Unfortunately, even the grains with the highest total and occupancy are completely X-ray amorphous which hampers the investigation and validation of ‘oxybismutobetafite’ from the Scherlovyi pegmatite as new mineral species.
Site-total-charge (STC) approach
Since the publication of Bosi et al. (Reference Bosi, Hatert, Hålenius, Pasero, Miyawaki and Mills2019a) which establishes new rules for the use of the dominant-valency rule to complex mineral compositions, there is an alternative way to express the A constituents in oxybismutomicrolite:



Since the atomic arrangement (BiNa) is the most abundant one, the end-member formula of oxybismutomicrolite should be (BiNa)Ta2O6O. However, in this case, the authors of this paper have to follow the nomenclature rules based on Atencio et al. (Reference Atencio, Andrade, Christy, Gieré and Kartashov2010), which give the formula [(Bi3+,#)2]Σ4+Ta2O6O where # = subordinate substituents, such as Na+, Ca2+ and □ (vacancy).
Generally speaking, the different approaches to writing the end-member formulae are due to some contradictions between the current nomenclature of pyrochlore-supergroup minerals (Atencio et al., Reference Atencio, Andrade, Christy, Gieré and Kartashov2010) and the STC rules described in the Bosi et al. (Reference Bosi, Hatert, Hålenius, Pasero, Miyawaki and Mills2019a,Reference Bosi, Biagioni and Obertib).
For pyrochlore-supergroup minerals, this is likely to cause an issue with the naming of minerals and the proliferation of species if the STC approach is taken. The end-member formula of the mineral from the Scherlovyi pegmatite (described above) calculated according to Bosi et al. (Reference Bosi, Hatert, Hålenius, Pasero, Miyawaki and Mills2019a,Reference Bosi, Biagioni and Obertib) would be (Bi1.33□0.67)Ta2O6O and therefore not the same as for the type specimen from the Malkhan pegmatite field. Consequently, these two occurrences are different according to Bosi et al. (Reference Bosi, Hatert, Hålenius, Pasero, Miyawaki and Mills2019a,Reference Bosi, Biagioni and Obertib), while they both belong to oxybismutomicrolite according to Atencio et al. (Reference Atencio, Andrade, Christy, Gieré and Kartashov2010). The use of the STC approach would also change the end-member formulae of several other members of the pyrochlore-supergroup described earlier in accordance with Atencio et al. (Reference Atencio, Andrade, Christy, Gieré and Kartashov2010). For example, hydrokenomicrolite, ideally □2Ta2[O4(OH)2](H2O) (Andrade et al., Reference Andrade, Atencio, Chukanov and Ellena2013b), would contain essential Ba: (□Ba)Ta2O6(H2O) even if it contains only 0.33 apfu Ba. Oxynatromicrolite, (Na,Ca,U)2Ta2O6(O,F) (Fan et al., Reference Fan, Ge, Li, Yu and Shen2016) would completely loose its ‘oxy-’ prefix and become fluorine-dominant mineral: (Na5/3U⅓)Ta2O6F.
The above examples show that the new approach elaborated by Bosi et al. (Reference Bosi, Hatert, Hålenius, Pasero, Miyawaki and Mills2019a,Reference Bosi, Biagioni and Obertib) and accepted by the IMA−CNMNC might need to be applied to the pyrochlore-supergroup minerals with great care and that the nomenclature of pyrochlore-supergroup minerals needs to be re-examined in light of these STC changes.
Supplementary material
To view supplementary material for this article, please visit: https://doi.org/10.1180/mgm.2020.25
Acknowledgements
This work was partly carried out in accordance with the state task, state registration No. 0089-2019-0013 for NVC. The research was supported by grant GAČR 19-05198S for RŠ. The manuscript benefited from the constructive reviews of Peter Leverett and three anonymous referees, as well as the Associate Editor Ferdinando Bosi. We are grateful to Chairman Ritsuro Miyawaki and voting members of IMA−CNMNC for their recommendations on the idealised formula of the new mineral. The Principal Editor Stuart J. Mills and Igor V. Pekov are thanked for the discussion and constructive comments. Daniel Atencio, Vladimir Yu. Karpenko and Ricardo Scholz are acknowledged for their help in finding several literature sources.