Introduction
The last eruption of the Tolbachik volcano (Kamchatka Peninsula, Russian Far East) occurred almost ten years ago. It was termed the 2012–2013 Tolbachik fissure eruption. The extensive post-eruption activity led to the formation of fumarolic fields with structurally and chemically diverse mineralisation. The diversity and originality of this mineralisation is due to a combination of unique conditions and mechanisms: these are high temperatures, atmospheric pressure, oxidising conditions and gas transport of most components. Sulfate and arsenate mineralisations are the most widespread in terms of the number of mineral species. At the moment, more than 350 mineral species have been reliably established and more than 120 new mineral species have been discovered on the Tolbachik volcano (Pekov et al., Reference Pekov, Agakhanov, Zubkova, Koshlyakova, Shchipalkina, Sandalov, Yapaskurt, Turchkova and Sidorov2020a). Five chloro-vanadates have been described on the Tolbachik volcano, including leningradite PbCu3(VO4)2Cl (Vergasova et al., Reference Vergasova, Filatov, Semenova and Anan´ev1990; Siidra et al., Reference Siidra, Krivovichev, Armbruster, Filatov and Pekov2007), averievite Cu6O2(VO4)2Cl2⋅n(K,Cs,Rb)Cl (Starova et al., Reference Starova, Krivovichev, Fundamensky and Filatov1997; Vergasova et al., Reference Vergasova, Starova, Filatov and Anan´ev1998), yaroshevskite Cu9O2(VO4)4Cl2 (Pekov et al., Reference Pekov, Zubkova, Zelenski, Yapaskurt, Polekhovsky, Fadeeva and Pushcharovsky2013), dokuchaevite Cu8O2(VO4)3Cl3 (Siidra et al., Reference Siidra, Nazarchuk, Zaitsev, Polekhovsky, Wenzel and Spratt2019b) and aleutite Cu5O2(AsO4)(VO4)(Cu0.5□0.5)Cl (Siidra et al., Reference Siidra, Nazarchuk, Agakhanov and Polekhovsky2019a). Vanadate mineralisation is represented, for example, by the following minerals: pseudolyonsite Cu3(VO4)2 (Zelenski et al., Reference Zelenski, Zubkova, Pekov, Boldyreva, Pushcharovsky and Nekrasov2011), udinaite NaMg4(VO4)3 (Pekov et al., Reference Pekov, Zubkova, Koshlyakova, Belakovskiy, Vigasina, Agakhanov, Turchkova, Britvin, Sidorov and Pushcharovsky2018b), kainotropite Cu4Fe3+O2(V2O7)(VO4) (Pekov et al., Reference Pekov, Zubkova, Yapaskurt, Polekhovsky, Britvin, Turchkova, Sidorov and Pushcharovsky2020b). Vergasova and Filatov (Reference Vergasova and Filatov2012, Reference Vergasova and Filatov2016), Pekov et al. (Reference Pekov, Koshlyakova, Zubkova, Lykova, Britvin, Yapaskurt, Agakhanov, Shchipalkina, Turchkova and Sidorov2018a, Reference Pekov, Agakhanov, Zubkova, Koshlyakova, Shchipalkina, Sandalov, Yapaskurt, Turchkova and Sidorov2020a) and Vergasova et al. (Reference Vergasova, Filatov, Moskaleva, Nazarova and Shablinskii2022) investigated the fumarolic activity and mineralisation of Tolbachik eruptions.
Herein we describe medvedevite (Russian Cyrillic: медведевит), a new mineral, ideally KMn2+V2O6Cl⋅2H2O, found in 2020 on the Toludskoe lava field, formed during the 2012–2013 Tolbachik fissure eruption, Kamchatka Peninsula, Russia. Medvedevite (symbol Mvv) has been approved by the Commission on New Minerals, Nomenclature and Classification of the International Mineralogical Association (IMA2021-082a, Shablinskii et al., Reference Shablinskii, Avdontseva, Vergasova, Filatov, Avdontseva, Povolotskiy, Moskaleva, Kargopoltsev and Britvin2022). The mineral has been named in honour of the Russian geologist and chemist Robert Alexandrovich Medvedev (1939–2005). He was a member of several geological parties of the Steppe Expedition (Makinsk, Tselinograd Region, Kazakh SSR), and worked on uranium deposits in Northern and Central Kazakhstan. The geological prospecting works resulted in the discovery of the three major ore regions of Kazakhstan with 62 uranium deposits.
Under the leadership of Robert Alexandrovich Medvedev and with his direct participation progressive methods were introduced for the determination of gases in various alloys of rare earth metals. As a qualified specialist with a pronounced research approach, Robert Alexandrovich worked constantly on the improvement of laboratory methods in order to increase their quality and reduce the time for performing analyses. Many of his initiatives formed the basis of rationalisation proposals and scientific and technical work. Thus, Robert Alexandrovich Medvedev created a department for active leaching, desorption and electrolysis in the chemical and metallurgical workshop in order to extract gold from ‘poor’ raw materials and obtain finished products at the Ural Electrolytic Copper Refinery ‘Uralelectromed’.
The type specimen of medvedevite is deposited in the Mineralogical Museum of Saint-Petersburg State University (St. Petersburg, Russia) under catalogue number 1/19900.
Occurrence and association
The holotype material was collected (11.09.2020) on the Toludskoe lava field, which was formed during the 2012–2013 Tolbachik fissure eruption. The mineral was found in the stalactites of a lava cave (Fig. 1). The size of the stalactite was 3 m × 2 m. The stalactite had a basalt core, and the thickness of the mineral deposits was 3 mm. Thin needle transparent bright red crystals of medvedevite were found included in thénardite crystals. Medvedevite occurs in association with thénardite, halite, aphthitalite, leonite, kieserite, eugsterite and syngenite.

Fig. 1. Lava cave of the Toludskoe lava field (2020). Photo by A.A. Kargopoltsev.
General appearance and physical properties
Medvedevite occurs as red well-formed prismatic or fine acicular crystals up to 0.15 mm long (Figs 2, 3). The streak is red and the lustre is vitreous. Cleavage is perfect {010}. The calculated density based on the empirical formula and powder unit-cell parameters obtained from the powder X-ray diffraction (XRD) data is 2.69 g/cm3.
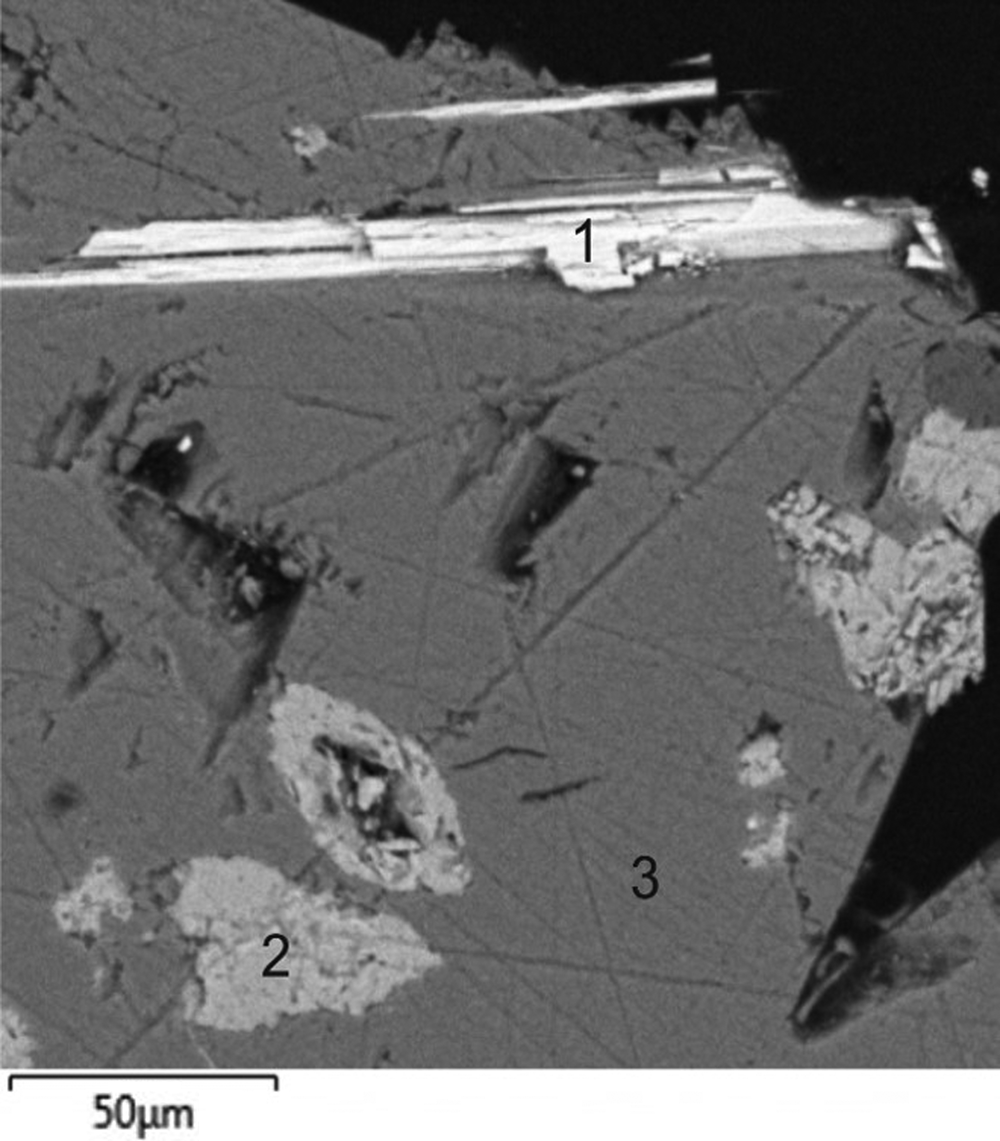
Fig. 2. Crystals of medvedevite (1) with aphthitalite (2) and thénardite (3). Scanning electron microscopy image, specimen #6.

Fig. 3. (a) Red acicular crystal of medvedevite; (b) crystals of medvedevite in thénardite [specimen #6].
The mineral is optically biaxial (+), with α =1.782(2), β = 1.786(2), γ = 1.792(2) and 2V(calc) = 41° (λ = 589 nm). Orientation: Y = b. Pleochroism is weak, with the following absorption scheme: Z (dark red) > X (bright red). The Gladstone–Dale compatibility index (Mandarino, Reference Mandarino1981) based on the ideal formula and unit-cell parameters obtained from single crystal XRD data is calculated as 1–(K P/K C) = 0.004 (superior).
Chemical composition
The chemical composition of medvedevite was studied using a TESCAN “Vega3” electron microprobe equipped with an Oxford Instruments X-max 50 silicon drift energy-dispersive spectroscopy system, operated at 20 kV and 760 pA, with a beam size of 220 nm. Analytical results are given in Table 1. The data processing was done using Aztec software and an X-MAX-80 mm2 detector. The empirical formula calculated on the basis of 13+ positive charge units for the anhydrous part and 2H2O is (K1.02Na0.03)Σ1.05Mn0.95(V1.92S0.05Si0.04)Σ2.01O6.02Cl0.96⋅2H2O. The simplified formula is (K,Na)Mn(V,S,Si)2O6Cl⋅2H2O. The ideal formula is KMn2+V5+2O6Cl⋅2H2O, which requires K2O 12.96, MnO 19.52, V2O5 50.05, H2O 9.91, Cl 9.76, –O = Cl –2.20, total 100 wt.%.
Table 1. Chemical data (in wt %) for medvedevite.

* Calculated for 2H2O in the empirical formula
S.D. – standard deviation
Powder X-ray diffraction
The powder XRD data were collected using a Rigaku R-AXIS RAPID II diffractometer (Gandolfi mode with CoKα) and handled using the domestic software (Britvin et al., Reference Britvin, Dolivo-Dobrovolsky and Krzhizhanovskaya2017). Medvedevite is monoclinic, P21/c, a = 7.1831(5), b = 10.1121(9), c = 12.737(1) Å, β = 106.16(3)°, V = 888.6(4) Å3 and Z = 4. The measured and calculated powder diffraction patterns are given in Table 2.
Table 2. Powder X-ray diffraction data (d in Å) for medvedevite.

The seven strongest lines are shown in bold.
For the calculated pattern, only reflections with intensities ≥1 are given.
*The values of d calc are calculated on the basis of unit cell parameters refined by powder XRD data.
Single-crystal X-ray diffraction
A single crystal of medvedevite was studied using a Rigaku XtaLab Synergy-S diffractometer equipped with a high-speed direct-action detector HyPix–6000HE. The study was carried out by means of microfocus monochromatic MoKα radiation (λ = 0.71073 Å) with frame widths 1.0° in ω and 180 s counting time for each frame. The data were interpolated into the CrysAlisPro software (2015) for further processing. An absorption correction was introduced using the SCALE3 ABSPACK algorithm. The crystal structure was solved by direct methods and refined by least-square techniques in the monoclinic space group P21/c to R 1 = 0.029 (wR 2 = 0.077) for 2152 unique observed reflections with I ≥ 2σ(I) using the SHELX program package (Sheldrick, Reference Sheldrick2015) within the Olex2 shell (Dolomanov et al., Reference Dolomanov, Bourhis, Gildea, Howard and Puschmann2009). The hydrogen atoms of O8 were placed in calculated positions and refined using a ‘riding’ model and were included with U iso(H) set to 1.5 U eq(O) and an O–H bond length restrained to 0.92 Å. The hydrogen atoms of O7 were located from the inspection of difference-Fourier maps using the DFIX command. Crystallographic data and refinement parameters, atomic coordinates and isotropic displacement parameters, atomic anisotropic displacement parameters and selected bond lengths are summarised in Tables 3–5. The crystallographic information file has been deposited with the Principal Editor of Mineralogical Magazine and is available as Supplementary material (see below).
Table 3. Crystallographic data and refinement parameters for medvedevite.

Table 4. Atomic coordinates and displacement parameters (Å2) for medvedevite.

Table 5. Selected bond lengths (Å) for medvedevite.
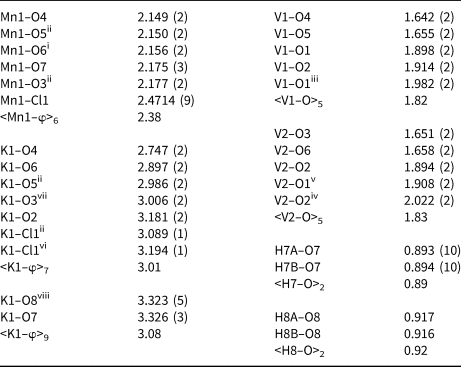
Crystal structure
The crystal structure of medvedevite belongs to a new structure type. The concept of ‘structural unit’ and ‘interstitial complex’ (Hawthorne, Reference Hawthorne1983; Schindler et al., Reference Schindler, Hawthorne and Baur2000a, Reference Schindler, Hawthorne and Baur2000b) could be applied to crystal structure of medvedevite. The structural units in medvedevite are based on the high bond-valence V5+O5 polyhedra which share edges and link into [V2O6] chains elongated along the a axis (Figs 4, 5). The interstitial complexes consist of Mn2+, K+ cations and H2O groups and occupy the interstices between structural units.

Fig. 4. Crystal structure of medvedevite: (a) the cb section; (b) the ac section. The unit cell is outlined.

Fig. 5. Divanadate V2O6 chain in the crystal structure of medvedevite with edge-shared VO5 polyhedra (structural unit).
Each of the two independent VO5 polyhedra includes two short vanadyl (1.642–1.658 Å) and three long equatorial (1.894–2.022 Å) bonds producing 2+3 coordination. The O atoms that participate the vanadyl bonds are in cis configuration and connected only with one V atom. The O atoms that participate in the equatorial bonds are connected with three V atoms and involved in the forming of chains via edges. The Mn1 site is coordinated octahedrally by four O atoms of vanadium polyhedra (<Mn1–O> 2.149–2.177 Å), one chlorine (<Mn1–Cl1> 2.471 Å) and one O atom of the H2O molecule (<Mn–O7> 2.175 Å). The K1 site is surrounded by five oxygen atoms (<K1–O> 2.747–3.181 Å) and two chlorine atoms (<K1–Cl1> 3.089–3.194 Å).
Raman spectroscopy
The Raman spectrum was measured with a Horiba Jobin-Yvon LabRam HR800 spectrometer with a spectral resolution of 3 cm–1. The CW He–Ne laser with a wavelength of 632.8 nm and a power of 10 mW was used to excite the scattering. The laser beam was focused on the sample surface with 50× objective lens. The scattering light was collected with the same objective, and the spectrum was recorded twice within 60 seconds.
The Raman spectrum of medvedevite is shown in Fig. 6. The observed Raman bands and their assignments (according to Baran et al., Reference Baran, Cabello and Nord1987; Yao et al., Reference Yao, Ren and Liu2018) for medvedevite KMn2+V5+2O6Cl⋅2H2O are given in the Table 6. The bands around 475 cm–1 and 837 cm–1 were assigned to symmetric and antisymmetric stretching vibrations V–O–V, respectively. Baran et al. (Reference Baran, Cabello and Nord1987) note that similar stretching vibrations are observed at similar frequencies for V2O5. Raman bands below 400 cm−1 are related to VO2 rocking, twisting and chain deformation vibrations (Yao et al., Reference Yao, Ren and Liu2018). Thus, the Raman spectroscopy data agree with the XRD data, and the main contribution to the vibrational spectra is made by vanadium polyhedra linked into chains through a bridging oxygen.

Fig. 6. Raman spectrum of medvedevite.
Table 6. Assignment of the Raman and FTIR bands for medvedevite KMn2+V5+2O6Cl⋅2H2O.

str – stretch, as – antisymmetric stretch, s – symmetric stretch
FTIR spectroscopy
The FTIR spectrum was measured in transmission mode (crystal on a KBr plate) on a Bruker Hyperion 2000 IR microscope connected to a Bruker Vertex 70 FTIR spectrometer. The spectrum with a resolution of 4 cm–1 was recorded by an InGaAs detector cooled with liquid nitrogen.
The FTIR spectrum of medvedevite is shown in Fig. 7. The IR spectrum clearly identifies the water that is part of the studied mineral by the O–H stretching vibrations, which correspond to the bands in the region of 3250, 3410 and 3570 cm–1. Moreover, the bending vibrations of molecular water show bands in the region of 1660 and 1600 cm–1. The position of the bands of these vibrations is similar to the vibrations in metarossite (Frost et al., Reference Frost, Erickson and Weier2004) (Table 6).

Fig. 7. FTIR spectrum of medvedevite.
Discussion
Medvedevite KMn2+V5+2O6Cl⋅2H2O is structurally related to delrioite Sr(VO3)(H2O)4 (Thompson and Sherwood, Reference Thompson and Sherwood1959), metarossite Ca(VO3)2(H2O)2 (Kelsey and Barnes, Reference Kelsey and Barnes1960), rossite Ca(VO3)(H2O)4 (Ahmed and Barnes, Reference Ahmed and Barnes1963), metadelrioite CaSr(V2O6)(OH)2 (Smith, Reference Smith1970), munirite NaVO3(H2O)2 (Butt and Mahmood, Reference Butt and Mahmood1983), metamunirite NaVO3 (Kato and Takayama, Reference Kato and Takayama1984), dickthomssenite MgV2O6(H2O)7 (Hughes et al., Reference Hughes, Cureton, Marty, Gault, Gunter, Campana, Rakovan, Sommer and Brueseke2001), ansermetite MnV2O6(H2O)4 (Brugger et al., Reference Brugger, Berlepsch, Meisser and Armbruster2003) and calciodelrioite Ca(VO3)(H2O)4 (Kampf et al., Reference Kampf, Marty, Nash, Plasil, Kasatkin and Skoda2012). The crystal structure of these minerals contain the same structural unit – divanadate V2O6 chains with edge-shared VO5 polyhedra. According to (Schindler et al., Reference Schindler, Hawthorne and Baur2000a, Reference Schindler, Hawthorne and Baur2000b) Lewis basicities of this structural unit can be vary from 0.14 to 0.22 valence units (vu). The calculation of Lewis basicity for the structural unit and Lewis acidity for the interstitial complex in the medvedevite crystal structure lets us to determine the role of the H2O groups, stability of the minerals and possible response to dehydration of the medvedevite crystal structure.
The bond-valence calculations were performed using empirical parameters taken from Brown and Altermatt (Reference Brown and Altermatt1985) (Table 7). The bond-valence calculations and values of the bond lengths can confirm the valence of Mn (e.g. Levi and Aurbach, Reference Levi and Aurbach2014). According to Levi and Aurbach (Reference Levi and Aurbach2014), the average bond length for MnO6 is 2.18–2.23 Å for Mn2+, 2.02–2.08 Å for Mn3+, 1.89–1.92 Å for Mn4+. The average bond length of the MnO5Cl polyhedra in the crystal structure of medvedevite is 2.21 Å. Therefore, the valence of Mn in the medvedevite is 2+ according to analysis of bond-valence and bond lengths data. If we calculate the bond valence sums (BVS) for MnO5Cl polyhedra in the crystal structure of medvedevite using r 0 parameter for Mn2+ (Brown and Altermatt, Reference Brown and Altermatt1985), the BVS is 2.23 vu. And if we calculate BVS for MnO5Cl polyhedra in the crystal structure of medvedevite using r0 parameters for Mn3+ (Brown and Altermatt, Reference Brown and Altermatt1985), the BVS is decreased (2.09 vu). Therefore, the valence of Mn is 2+.
Table 7. Bond-valence analysis (vu = valence units) for medvedevite.

The knowledge of the coordination numbers of cation and anions in the structural unit lets us calculate the Lewis basicity of the structural unit (Hawthorne, Reference Hawthorne1985; Schindler et al., Reference Schindler, Hawthorne and Baur2000a, Hawthorne and Schindler, Reference Hawthorne and Schindler2008; Hawthorne, Reference Hawthorne2015). In the structural unit of medvedevite, the average coordination number of O is 3.2. Therefore, there are 19 bonds in the crystal structure that involve O atoms of the structural unit. Within the structural unit, there are 10 bonds in the VO5 polyhedra. The number of additional bonds required from outside the structural unit is 9. Thus, the Lewis basicity of a structural unit is 2/9 = 0.22 vu.
The interstitial complex in the medvedevite crystal structure is [[7]K[6]Mn2+(H2O)d(H2O)e [4]Cl]. The Lewis acidity of the interstitial complex can be defined as its charge divided by the number of bonds to the structural unit: (a + 2b + 3c – f)/[(am + bn + cl) + d – f (q – 1)], where a = number of monovalent cations, b = number of divalent cations, c = number of trivalent cations, d = transformer H2O groups, e = non-transformer H2O groups, f = monovalent anions, m, n, l and q = coordination numbers. According to Hawthorne and Schindler, Reference Hawthorne and Schindler2008, transformer H2O groups are “H2O groups in which the O atoms accept only one bond from a cation (inclusive of hydrogen bonds)”. The Lewis acidity of the interstitial complex in medvedevite is 0.18 vu. The values of Lewis basicity of the structural unit and Lewis acidity of the interstitial complex agree reasonably well according to the valence-matching principle (Brown, Reference Brown, O'Keeffe and Navrotsky1981) and medvedevite is a stable structure.
Let us consider the hypothetical dehydration of the medvedevite. In the dehydration of rossite to metarossite, an equatorial bond V–O in rossite transforms to a vanadyl bond in metarossite. This change balances the bond-valence requirement that is caused during dehydration by the decreasing of the number of transformer H2O groups. In the crystal structure of medvedevite, there are two H2O groups: transformer and isolated (practically non-bonded). Dehydration of the isolated H2O group will not disturb the stability of the medvedevite crystal structure according to the valence-matching principle. Dehydration of the transform H2O group will lead to instability in the structure, due to the fact that the structure cannot respond by transformation of equatorial to vanadyl bonds because there cannot be more than two vanadyl bonds per V5+ atom (Schindler et al., Reference Schindler, Hawthorne and Baur2000a).
Supplementary material
To view supplementary material for this article, please visit https://doi.org/10.1180/mgm.2022.38
Acknowledgements
This work was supported financially by the Russian Science Foundation (RSF) [grant no. 21-77-00069] and the Ministry of Science and Higher Education of the Russian Federation within the scientific tasks of the Institute of Silicate Chemistry (Russian Academy of Sciences) [project number 0081-2022-0002]. Technical support by the SPbSU X-ray Diffraction Centre is gratefully acknowledged.
Competing interests
The authors declare none