Introduction
With the exception of few anhydrous vanadates, known to date as anthropogenic phases (Witzke and Ruger, Reference Witzke and Ruger1998), most of the anhydrous vanadate minerals originate from the fumaroles of Izalco volcano, El Salvador (Hughes and Stoiber, Reference Hughes and Stoiber1985) and Tolbachik volcano, Kamchatka Peninsula, Russia (Pekov et al., Reference Pekov, Zubkova, Zelenski, Yapaskurt, Polekhovsky, Fadeeva and Pushcharovsky2013; Vergasova and Filatov, Reference Vergasova and Filatov2016). The former is especially famous for exceptionally vanadium-rich exhalative mineral assemblages. Geochemical environments that led to this type of mineralisation on the fumaroles of both volcanos were discussed by Hughes and Birnie (Reference Hughes and Birnie1980) and Zelensky et al. (Reference Zelenski, Zubkova, Pekov, Boldyreva, Pushcharovsky and Nekrasov2011). None of these works could give a definite answer to explain such V-rich mineralisation. Basaltic scoria on both localities show similar concentrations of V as compared to common Earth's basalts.
Exhalative mineral assemblages from fumaroles of the First and Second scoria cones, Great Fissure Tolbachik eruption 1975–76 are well-known for their exceptional diversity of Cu oxysalt minerals. Yadovitaya fumarole located on top of the Second scoria cone of the Great Fissure Tolbachik eruption is a type locality for 21 mineral species. Fifteen of them contain considerable amounts of Cu2+ (Pekov et al., Reference Pekov, Koshlyakova, Zubkova, Lykova, Britvin, Yapaskurt, Agakhanov, Shchipalkina, Turchkova and Sidorov2018).
Herein we report on the chemical composition, structure and properties of dokuchaevite (Cyrllic: докучаевит), Cu8O2(VO4)3Cl3. The mineral is named in honour of Prof Vasily V. Dokuchaev (Cyrillic: Василий Васильевич Докучаев) (1846–1903). V.V. Dokuchaev is a founder of Soil Science. He was a professor in the Department of Mineralogy and from 1879, lectured on mineralogy and crystallography at St. Petersburg State University. The term ‘dokuchaevite’ has been used as a synonym of delafossite from Dzhezkazgan mining district, Karaganda, Kazakhstan. The usage of the term is not in a wide circulation, thus it should not be confused with the new fumarolic mineral described in this paper. Both the mineral and the mineral name were approved by the Commission on New Minerals, Nomenclature and Classification of the International Mineralogical Association (IMA2018-012, Siidra et al. Reference Siidra, Nazarchuk, Zaitsev, Polekhovsky, Wenzel and Spratt2018b). Type material is deposited at the Mineralogical Museum, St. Petersburg State University, St. Petersburg, Russia (catalogue no. 1/19664).
Occurrence and association
Dokuchaevite occurs as a product of fumarolic activity. It was found in June, 2016 in the Yadovitaya fumarole, Second scoria cone, Northern Breakthrough (North Breach), Great Tolbachik Fissure Eruption, Tolbachik volcano, Kamchatka, Russia. The Second scoria cone is located ~18 km SSW of the active shield volcano Ploskiy Tolbachik (Fedotov and Markhinin, Reference Fedotov and Markhinin1983). Dokuchaevite closely associates with kamchatkite, euchlorine and langbeinite (Fig. 1). Other associated minerals are alumoklyuchevskite, lyonsite, pseudolyonsite, tenorite, hematite and anhydrite (Fig. 1). Dokuchaevite is a fumarolic mineral that is deposited directly from volcanic gas emissions as a sublimate. The temperature of gases at the sampling location was ~300°C. All the recovered samples were packed and isolated when collected to avoid any contact with the external atmosphere.

Fig. 1. Dark-red prismatic crystals of dokuchaevite associated with kamchatkite (yellow–green), euchlorine (emerald-green) and langbeinite (white).
Physical properties
Dokuchaevite occurs on the crusts (Fig. 1) of various copper sulfate exhalative minerals (such as kamchatkite and euchlorine) as individual prismatic crystals up to 30 μm × 30 μm × 150 μm (but usually from 5 to 20 μm in size). Dokuchaevite is dark red, with a reddish black streak and close to adamantine lustre. It is brittle with no cleavage observed. Parting was not observed, and its fracture is uneven. The density could not be measured due to lack of sufficient material. The calculated density using the empirical formula derived from the analytical data given in Table 1 is 4.142 g cm–3.
Table 1. Reflectance values for dokuchaevite (SiC standard in air).

The values required by the Commission on Ore Mineralogy are given in bold.
Dokuchaevite has a Vickers Hardness Number (VHN20) of 55 kg mm–2 (n = 5, range 54–56 kg mm–2), which corresponds to a Mohs’ hardness of ~2.
Despite the transparency of dokuchaevite, its optical properties had to be investigated in reflected light, because of the high values of refractive indices typical of vanadates and arsenates. Reflectance measurements were made using a SiC standard in air in the range 400–700 nm (Table 1). In reflected light, dokuchaevite is grey with a yellowish tint. It is non-pleochroic, with abundant yellow–red internal reflections and a weak bireflectance. The measured anisotropy is ΔR 589 = 0.85%.
Dokuchaevite is soluble in warm H2O.
Chemical composition
Three crystals (34 × 9, 60 × 13 and 65 × 10 μm) of dokuchaevite were mounted in epoxy resin and polished with oil suspension. The mineral has been studied and analysed by both energy- and wavelength-dispersive spectrometry (EDS and WDS respectively).
Preliminary EDS analyses were obtained using a Hitachi S-3400N scanning electron microscope equipped with an Oxford Instruments X-Max 20 Energy Dispersive Spectrometer (installed in St. Petersburg State University). The electron beam accelerating voltage was 20 kV and the current 2.0 nA; both focused and defocused beam (1 to 3 μm spot size) were used and the X-ray acquisition time was 30 s. The following standards were used: Сu (Cu), Zn (Zn), FeS2 (Fe), V (V), InAs (As), CaSO4 (S), Mo (Mo), SiO2 (Si), InP (P) and NaCl (Cl). However, there is a strong overlap for Mo (Lα 2.293) and S (Kα 2.307) lines in the ED spectrum.
The WDS analyses were obtained using a JEOL 8900 Superprobe (Universität Tübingen) and Cameca SX 100 (Natural History Museum, London), the accelerating voltage was 20 kV, beam current 15 nA and probe diameter 1 μm. The ZAF method was used for data raw correction. Standard and analytical data are given in Table 2. Note, that Cl was analysed using two different standards – tugtupite and NaCl.
Table 2. Analytical data (wt.%) for dokuchaevite.

S.D. – standard deviation
The empirical formula calculated on the basis of 17 anions per formula unit is (Cu7.72Zn0.06Fe0.05)Σ7.83(V2.20As0.61Mo0.10S0.06P0.03Si0.03)Σ3.03O13.96Cl3.04. The simplified formula is Cu8O2(VO4)3Cl3. Ideally dokuchaevite contains CuO = 64.18 wt.%, V2O5 = 27.52 wt.%, Cl = 10.73 wt.%, –O = Cl2 = 2.42 wt.%, total = 100.00 wt.%.
X-ray crystallography
Experiment
Powder X-ray diffraction data were collected using a Rigaku R-AXIS Rapid II single-crystal diffractometer equipped with cylindrical image plate detector using Debye-Scherrer geometry (CoKα radiation, d = 127.4 mm). Data (in Å) are given in Table 3. Unit-cell parameters refined from the powder data processed using osc2xrd software (Britvin et al. Reference Britvin, Dolivo-Dobrovolsky and Krzhizhanovskaya2017) are as follows: triclinic, space group P $\bar{1}$, a = 6.3335(4) Å, b = 8.2088(5) Å, c = 15.556(1) Å, α = 90.509(6)°, β = 97.239(7)°, γ = 90.911(6)°, V = 802.20(7) Å3 and Z = 2.
Table 3. Powder X-ray diffraction data (d in Å) for dokuchaevite.

The strongest lines are given in bold.
A dark red transparent crystal fragment of dokuchaevite was mounted on a thin glass fibre for X-ray diffraction analysis using a Bruker APEX II DUO X-ray diffractometer with a micro-focus X-ray tube operated with MoKα radiation at 50 kV and 40 mA. The data were integrated and corrected for absorption using a multi-scan type model implemented in the Bruker programs APEX and SADABS (Bruker-AXS, 2014). More than a hemisphere of X-ray diffraction data was collected. The refinement in the P $\bar{1}$ space group converged to R 1 = 0.057 (Table 4). The final model included anisotropic displacement parameters for all atoms. The final atomic coordinates, anisotropic displacement parameters and bond-valence sums are given in Table 5. All bond-valence parameters were taken from Brese and O'Keeffe (Reference Brese and O'Keeffe1991). All of the bond-valence sums are in good agreement with the expected oxidation states for all atoms. Lists of observed and calculated structure factors have been deposited with the Principal Editor of Mineralogical Magazine and are available as Supplementary material (see below).
Table 4. Crystallographic data and refinement parameters for dokuchaevite.
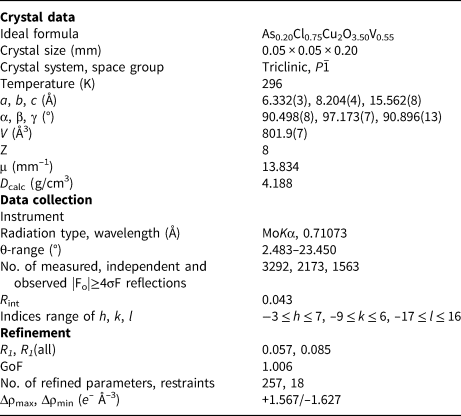
Table 5. Bond-valence sums (BVS), coordinates and isotropic and anisotropic displacement parameters (Å2) of atoms in dokuchaevite.

*V0.75(2)As0.25(2); **V0.84(2)As0.16(2); ***V0.59(2)As0.41(2). Bond-valence sums for mixed T sites were calculated using parameters for V5+–O bonds.
Cation coordination
The crystal structure of dokuchaevite contains eight symmetrically independent Cu sites (Fig. 3a, Table 6) with different coordination environments. All of the copper sites, except for Cu5, demonstrate mixed-ligand coordination. All of the Cu–Cl bonds <3.3 Å were taken into consideration. Cu3, Cu4 and Cu8 have similar distorted square pyramidal [(4O)+Cl] mixed-ligand coordination, common for copper oxychloride minerals and synthetic compounds. The Cu2 atom has also five-fold but [(2O)+(2O + Cl)] coordination. CuO3Cl2 polyhedra with [(3O + Cl)+Cl] and [(2O)+(O + 2Cl)] coordination environments are observed for Cu1 and Cu6 atoms, respectively. The Cu7 atom has exceptionally rare planar square [3O + Cl] mixed-ligand coordination, which has been described previously only in ilinskite NaCu5O2(SeO3)2Cl3 (Krivovichev et al., Reference Krivovichev, Filatov and Vergasova2013a). Cu5 has a strongly distorted [(3O) + 2O] trigonal bipyramid formed exclusively by O atoms. Interestingly, none of the copper sites in dokuchaevite demonstrate Jahn–Teller distorted octahedral coordination environments typical for Cu2+ cations.
Table 6. Selected interatomic distances (Å) in dokuchaevite.

There are three symmetrically independent tetrahedral T sites occupied predominantly by V5+ in dokuchaevite. In addition, all of the T sites contain a considerable amount of As5+ (Table 5). However, the T–O bond lengths and angles fall within the range typically observed in vanadate structures. The refined V/As ratio is in good agreement with the microprobe data, taking into account zonation of the dokuchaevite crystals (Fig. 2) and presence of other cations (Mo, S, Si or P) in the tetrahedral sites.

Fig. 2. Back-scatter electron image of (a) the zoned dokuchaevite crystal and (b) false colour X-ray element distribution map (V = blue and As = green).
The oxygen atoms O2–O13 are each bonded strongly in TO4 tetrahedra. The O1 and O14 sites belong to additional oxygen atoms (Krivovichev et al., Reference Krivovichev, Mentré, Siidra, Colmont and Filatov2013b) being coordinated tetrahedrally by four Cu atoms each (Fig. 3b). The O1 atom is coordinated tetrahedrally by Cu1, Cu3, Cu4 and Cu5 atoms thus forming an O1Cu4 oxocentred tetrahedron. Similarly, the O2 atom is central in the O2Cu4 tetrahedron with Cu2, Cu3, Cu4 and Cu7 in the vertices. The average <O1–Cu> and <O2–Cu> bond lengths in dokuchaevite are 1.926 and 1.922 Å, respectively. Both values are in good agreement with the typical <O–Cu> bond length in oxocentred OCu4 tetrahedra (Filatov et al. Reference Filatov, Semenova and Vergasova1992).

Fig. 3. (a) Cu2+ cation coordination environments; (b) general projection of [O2Cu6]8+ chains in dokuchaevite; and (c) Cu8-centred dimeric units.
Description of the crystal structure
In the structure of dokuchaevite, OCu4 tetrahedra share common corners thus forming [O2Cu6]8+ single chains shown in Fig. 3b. Each of the OCu4 tetrahedra is bidentate. The topology of the [O2Cu6]8+ chain in dokuchaevite is very similar to the [Si2O6]4– silicate chain in the minerals of the pyroxene group (Liebau, Reference Liebau1985), where each SiO4 tetrahedron is replaced by an oxocentred OCu4 tetrahedron. [O2Cu6]8+ chains in dokuchaevite are well-known in Cu2+ oxysalts and can be described as fragments of kagome-nets (Kovrugin et al., Reference Kovrugin, Colmont, Siidra, Mentré, Al-Shuray, Gurzhiy and Krivovichev2015; Siidra et al., Reference Siidra, Kozin, Depmeier, Kayukov and Kovrugin2018a). Note, Cu6 and Cu8 do not form Cu–O bonds with additional oxygen atoms, and thus are not part of the oxocentred tetrahedra. Cu6 polyhedra are isolated one from each other, whereas Cu8-centred polyhedra form dimers depicted in Fig. 3c. Cu6- and Cu8-centred polyhedra provide the three-dimensional integrity of the [O2Cu6]8+ chains into the copper-oxide framework. TO4 mixed tetrahedral groups are located within the cavities of the framework (Fig. 4a). Thus the structural formula of dokuchaevite can be written as Cu2[Cu6O2](VO4)3Cl3.

Fig. 4. General projection of the crystal structure of (a) dokuchaevite and (b) yaroshevskite (OCu4 = red; VO4 = blue) along the a axis.
Discussion
Dokuchaevite is a new structure type and a new member of the structural family of minerals and synthetic compounds that are based upon (OCu4)6+ oxocentred tetrahedral groups (Krivovichev et al., Reference Krivovichev, Mentré, Siidra, Colmont and Filatov2013). No synthetic copper vanadate-chlorides without additional cations and anions are known to date. Dokuchaevite is structurally and compositionally related to yaroshevskite Cu3[Cu6O2][VO4]4Cl2 (Pekov et al., Reference Pekov, Zubkova, Zelenski, Yapaskurt, Polekhovsky, Fadeeva and Pushcharovsky2013) (Table 7) with the crystal structure (Fig. 4b) also based on the similar [O2Cu6]8+ chains. However yaroshevskite is characterised by lower Cl content and higher Cu amount per formula unit. a and b unit-cell parameters are also similar in dokuchaevite and yaroshevskite (Table 7), whereas the c parameter is enlarged significantly in dokuchaevite. An increase of Cl content in dokuchaevite is responsible for the enlargement of the c parameter from 9.1726(16) Å in yaroshevskite to 15.562(8) Å. Notably Cu2+ coordination environments show considerable diversity in dokuchaevite. This is in strong contrast to yaroshevskite, where most of the Cu sites (except for Cu2) are coordinated exclusively by oxygen atoms. The space between the [O2Cu6]8+ chains in yaroshevskite (Fig. 5a) is filled by Cu2-centred dimeric units similar to those in dokuchaevite (Fig. 3c). In dokuchaevite two possible fillings are possible. One is represented by the Cu-centred polyhedra similar to yaroshevskite, whereas another one contains Cl atoms only (Fig. 5b). The arrangements of the vanadate tetrahedra are very different in both minerals (Fig. 5c,d).

Fig. 5. General projection of the crystal structure of (a) yaroshevskite and (b) dokuchaevite (OCu4 = red) along the b axis. VO4 tetrahedra are omitted for clarity. Cu8-centred dimeric units in dokuchaevite are labelled. Increase of the c parameter value is highlighted. (c, d) The arrangement of VO4 tetrahedra in the structure of yaroshevskite and dokuchaevite, respectively.
Table 7. Crystal chemical data for dokuchaevite and yaroshevskite.

Other copper vanadate–chloride minerals known to date with additional cations include: leningradite PbCu3(VO4)2Cl2 (Siidra et al., Reference Siidra, Krivovichev, Armbruster, Filatov and Pekov2007), averievite Cu5O2(VO4)·nMClx (M = Cu, Cs, Rb or K) (Krivovichev et al., Reference Krivovichev, Filatov and Vergasova2015) and aleutite [Cu5O2](AsO4)(VO4)·(Cu0.5□0.5)Cl (Siidra et al., Reference Siidra, Nazarchuk, Agakhanov and Polekhovsky2019). Coparsite Cu4O2((As,V)O4)Cl (Starova et al., Reference Starova, Krivovichev and Filatov1998) contains, similarly to dokuchaevite, mixed tetrahedral sites but with the slight predominance of As5+ over V5+. Notably, all of these minerals originate from the fumarolic environments of the Second scoria cone, of the Great Tolbachik Fissure Eruption. Crystal structures of coparsite and averievite are also based on the oxocentred units formed by OCu4 tetrahedra. Copper oxyvanadates with ‘additional’ O atoms are common, not only in the fumaroles of the Scoria cones of Tolbachik volcano, but are also characteristic for the fumaroles of the Izalco volcano, El Salvador (e.g. stoiberite, Cu5O2(VO4)2 (Birnie and Hughes, Reference Birnie and Hughes1979) and fingerite, Cu11O2(VO4)6 (Finger, Reference Finger1985).
The crystal structure of dokuchaevite demonstrates the remarkable diversity of Cu2+ mixed-ligand coordination environments. The very complex copper-oxide substructure in dokuchaevite suggests interesting magnetic properties worthy of study for its synthetic analogue.
Supplementary material
To view supplementary material for this article, please visit https://doi.org/10.1180/mgm.2019.41
Acknowledgements
We are grateful to Nikita Chukanov, Peter Leverett and anonymous reviewer for valuable comments. This work was supported financially by the Russian Foundation for Basic Research, grant no. 19-05-00413. Technical support by the SPbSU X-ray Diffraction and Geomodel Resource Centres is gratefully acknowledged.