Introduction
The genus Graphis forms the largest element of the lichen family Graphidaceae, a chiefly tropical group of crustose lichens comprising more than 1 200 species, including those previously separated in the family Thelotremataceae (Sipman & Harris Reference Sipman, Harris, Lieth and Werger1989; Kirk et al. Reference Kirk, Cannon, Minter and Stalpers2008; Staiger et al. Reference Staiger, Kalb and Grube2006; Mangold et al. Reference Mangold, Martín, Lücking and Lumbsch2008). The family is characterized by rounded to lirellate or pseudostromatic ascomata with hemiangiocarpous development, non-amyloid, functionally unitunicate asci with apical wall thickenings (‘annelasceous’), ascospores with mostly thick, often amyloid septa and lens-shaped lumina, and a trentepohlioid photobiont in most species (Wirth & Hale, Reference Wirth and Hale1963, Reference Wirth and Hale1978; Henssen & Jahns, Reference Henssen and Jahns1974; Hale Reference Hale1974, Reference Hale1978, Reference Hale1981; Staiger Reference Staiger2002; Staiger et al., Reference Staiger, Kalb and Grube2006; Frisch et al. Reference Frisch, Kalb and Grube2006; Lücking & Rivas Plata Reference Lücking and Rivas Plata2008; Lücking et al. Reference Lücking, Chaves, Sipman, Umaña and Aptroot2008a; Mangold Reference Mangold2008; Mangold et al. Reference Mangold, Martín, Lücking and Lumbsch2008, Reference Mangold, Elix and Lumbsch2009).
Delimitation of genera within the lirellate Graphidaceae was initially based on a combination of morphological and anatomical characters, in particular ascomata morphology and ascospore septation. No less than 50 generic names were established during the 19th century (Staiger Reference Staiger2002). The first universally accepted system of the family was presented by Müller Argoviensis (Reference Müller Argoviensis1880, Reference Müller Argoviensis1882, Reference Müller Argoviensis1887). He distinguished five genera with pseudostromatic ascomata (Glyphis, Gymnographa, Medusulina, Sarcographa, Sarcographina) and divided the remainder, with non-stromatic, lirellate ascomata, into four further genera, based solely on ascospore colour and septation: Graphis (hyaline, transverse), Graphina (hyaline, muriform), Phaeographis (grey-brown, transverse), andPhaeographina (grey-brown, muriform). In doing so, he established three new, conceptually superfluous and formally illegitimate names (Graphina, Phaeographis, Phaeographina), since several earlier names were available for these groups (Lücking et al. Reference Lücking, Kalb, Staiger and McNeill2007). His concept was not accepted by Vainio (Reference Vainio1890, Reference Vainio1921), who united all species of the family into a single genus Graphis, but used subgeneric entities similar to the genera distinguished by Müller Argoviensis. Zahlbruckner (Reference Zahlbruckner, Engler and Prantl1907, Reference Zahlbruckner1923, Reference Zahlbruckner, Engler and Prantl1926) reinstated Müller Argoviensis' system. Several other genera established around the turn of the 19th century by Vainio Reference Vainio(1890), Nylander Reference Nylander(1891), Müller Argoviensis Reference Müller Argoviensis(1891), Clements Reference Clements(1909), Zahlbruckner Reference Zahlbruckner(1923), and Watson Reference Watson(1929), did not receive broad acceptance.
Except for the introduction of Gymnographopsis (Dodge Reference Dodge1966) and Cyclographina (Awasthi & Joshi Reference Awasthi and Joshi1979), the concept of using the ascospore-based genera Graphis, Graphina, Phaeographis, and Phaeographina, the stromatic genera Glyphis, Medusulina, and Sarcographa, and Sarcographina, and the apothecioid genus Gyrostomum, remained virtually unchanged (Wirth & Hale Reference Wirth and Hale1963, Reference Wirth and Hale1978; Nakanishi Reference Nakanishi1966; Awasthi & Singh Reference Awasthi and Singh1975; Patwardhan & Kulkarni Reference Patwardhan and Kulkarni1976, Reference Patwardhan and Kulkarni1977, Reference Patwardhan and Kulkarni1979a–Reference Patwardhan and Kulkarnic; Patwardhan & Nagarkar Reference Patwardhan and Nagarkar1979; Awasthi Reference Awasthi1991; Archer Reference Archer1999, Reference Archer2000, Reference Archer2001a–Reference Archere; Nakanishi et al. Reference Nakanishi, Kashiwadani and Moon2003a). Although workers as early as Redinger (Reference Redinger1933–Reference Redinger1935) stated that this concept was artificial (Wirth & Hale Reference Wirth and Hale1963, Reference Wirth and Hale1978; Harris Reference Harris1990, Reference Harris1995), it was not until about ten years ago that Kalb and collaborators started a thorough generic revision of the family (Staiger & Kalb Reference Staiger and Kalb1999; Kalb & Staiger Reference Kalb and Staiger2000), which culminated in the monographic treatments put forward by Staiger Reference Staiger(2002) and Kalb et al. Reference Kalb, Staiger and Elix(2004). Two further genera have been established recently (Lücking Reference Lücking2007; Lücking et al. Reference Lücking, Chaves, Sipman, Umaña and Aptroot2008a), and as a result, the lirellate Graphidaceae now comprise 24 genera (Staiger Reference Staiger2002; Nakanishi et al. Reference Nakanishi, Kashiwadani and Moon2003b; Archer Reference Archer2003a, Reference Archerb, Reference Archer2005, Reference Archer2006; Makhija & Adawadkar Reference Makhija and Adawadkar2005a, Reference Makhija and Adawadkarb; Makhija et al. Reference Makhija, Dube, Adawadkar and Chitale2005; Adawadkar & Makhija Reference Adawadkar and Makhija2006, Reference Adawadkar and Makhija2007; Lücking et al. Reference Lücking, Chaves, Sipman, Umaña and Aptroot2008a; Lücking & Rivas Plata Reference Lücking and Rivas Plata2008).
The genus Graphis itself forms the dominant element of the family, irrespective of its circumscription. Early concepts of Graphis were rather diffuse, and the ascospore-based genera established by Müller Argoviensis (Reference Müller Argoviensis1880, Reference Müller Argoviensis1882) were the first to be universally accepted. As such, Graphis was defined, until most recently, to include all species with non-stromatic, lirellate ascomata and hyaline, transversely septate ascospores, a highly artificial group. Wirth & Hale Reference Wirth and Hale(1978) noted that species with identical features but different ascospores were placed in different genera, and they called such taxa ‘sporomorphs’.
Recent studies in the Ostropales demonstrate the importance of ascomata anatomy, in particular excipular structures, for taxonomy and systematics at the genus level (Sherwood Reference Sherwood1977; Hale Reference Hale1980, Reference Hale1981; Lücking et al. Reference Lücking, Sérusiaux and Vězda2005; Frisch et al. Reference Frisch, Kalb and Grube2006; Staiger et al. Reference Staiger, Kalb and Grube2006; Mangold et al. Reference Mangold, Martín, Lücking and Lumbsch2008, Reference Mangold, Elix and Lumbsch2009). Accordingly, also in Graphidaceae excipulum structure turned out to be the most important character complex in defining genera, together with thallus and ascoma morphologies, ascospore colour, and chemistry (Staiger Reference Staiger2002; Kalb et al. Reference Kalb, Staiger and Elix2004). Ascospore septation, on the other hand, is variable in most genera, and both transversely and muriform ascospores can be found in closely related species of the same genus, as is the case in most of the Ostropales and also in other groups, such as the Pyrenulales and Trypetheliales (Harris Reference Harris1989; Aptroot et al. Reference Aptroot, Lücking, Sipman, Umaña and Chaves2008). Ascospore pigmentation, however, as well as iodine reaction, appears to be predictive at the genus and subfamily levels, at least in the lirellate Graphidaceae, as shown by molecular studies (Staiger Reference Staiger2002; Kalb et al. Reference Kalb, Staiger and Elix2004; Staiger et al. Reference Staiger, Kalb and Grube2006; Mangold et al. Reference Mangold, Martín, Lücking and Lumbsch2008).
In the sense of Staiger Reference Staiger(2002), Graphis is defined as having lirellate ascomata with well-developed, usually convergent labia and mostly closed disc, partly to fully carbonized excipulum, mostly non-inspersed hymenium, hyaline, transversely septate to muriform amyloid ascospores that react I+ violet-blue, and a usually corticate, mostly white-grey thallus. It comprises chiefly species previously assigned to either Graphis or Graphina sensu Müller Argoviensis. The genus now includes more than 300 species (Lücking et al. Reference Lücking, Archer and Aptroot2009, this volume), even after many taxa have been transferred to Acanthothecis, Anomomorpha, Carbacanthographis, Diorygma, Dyplolabia, Fissurina, Glyphis, and Hemithecium. Graphis sensu Staiger Reference Staiger(2002) appears to be a natural group, although molecular phylogenetic studies indicate a split into two lineages and suggest that Graphis might also include species presently assigned to Hemithecium, for example, with a non-carbonized excipulum (Staiger et al. Reference Staiger, Kalb and Grube2006; Mangold et al. Reference Mangold, Martín, Lücking and Lumbsch2008). The genera that most closely resemble Graphis are Carbacanthographis, Dyplolabia, Platygramme, and Thecographa (Lücking & Rivas Plata Reference Lücking and Rivas Plata2008; Lücking et al. Reference Lücking, Archer and Aptroot2009, this volume).
Table 1. Characters used in the numerical and phylogenetic analyses and their character state definitions and coding plus weights (W)

* Weights are derived from the homoplasy assessment (see Table 8), the discriminant analysis (see Table 2) and correlation tests (see Tables 3, 5–7).
† Excipulum carbonization was transformed into binary transformation series for phylogenetic analysis since three species have an apically and basally carbonized excipulum (laterally uncarbonized); this was coded as 2·5 in Spearman rank correlation calculations.
This paper attempts to assess the taxonomic value of phenotype characters (morphology, anatomy and chemistry) for the delimitation of species and species groups in Graphis, by means of numerical and cladistic analysis. Naturally, such a study has its limitations compared to molecular analysis. We are currently working on a molecular revision of Graphidaceae, including Graphis, but due to the limited access to fresh material of most species and difficulties in extracting DNA from tropical lichens associated with Trentepohlia, it will take a significant amount of time and effort to assemble a data set that would be even remotely representative of a genus including more than 300 species. In addition, analysis of phenotype characters is useful in two ways. First, it provides an objective approach to analyse the same characters that were used by previous workers but without analytical tools and often with ad-hoc decisions as to which characters are more important than others. Second, the results of such an analysis provide specific hypotheses for a targeted molecular approach; instead of randomly sequencing available material, one can select taxa to test specific hypotheses. The dataset analysed here is the largest ever used in the family, with almost all of the 320 currently accepted species included (Lücking et al. Reference Lücking, Archer and Aptroot2009, this volume).
Material and Methods
Material
Material of Graphis was studied from the following regions and collections: North America (F, FH, LSU, NY), Mexico (MEXU, F: collections by the author from 2001 to 2008), Guatemala (F: collections P. Standley, collections by the author from 2008), Costa Rica (B, CR, INBio, F, USJ: collections from TICOLICHEN project; see Lücking et al. Reference Lücking, Chaves, Sipman, Umaña and Aptroot2008a), Panama (US: collections M. Hale; F: collections by the author from 2006), Caribbean (MSC: collections H. Imshaug), Colombia (B: collections H. J. M. Sipman; F: collections by the author from 2008), Venezuela (B: collections H. J. M. Sipman; US: collections M. López Figueiras; F: collections by the author from 2008), Guyana (B: collections H. J. M. Sipman; F: collections by the author from 1997), French Guiana (B: collections H. J. M. Sipman; F: collections by the author from 1995), Ecuador (F: collections by the author from 2008), Peru (F: collections by the author from 2005 to 2008), Bolivia (F: collections by the author from 2007), Brazil (F, URM: collections M. E. S. Cáceres, collections by the author from 2000 to 2007), Argentina (CTES: collections L. I. Ferraro), Africa (BM, FH: collections C. W. Dodge, B. Fink, M. R. D. Seaward), India, SE Asia, Australia (ABL, CANB, F: collections A. Aptroot, A. Archer, H. T. Lumbsch; F: collections by the author from Thailand and the Philippines from 2007 and 2008). Altogether, several thousand specimens were examined.
Material was identified by comparison with types if possible, in some cases also by reference to well-illustrated literature or comparison with authentic type photographs (Wirth & Hale Reference Wirth and Hale1963, Reference Wirth and Hale1978; Nakanishi Reference Nakanishi1966; Patwardhan & Kulkarni Reference Patwardhan and Kulkarni1976, Reference Patwardhan and Kulkarni1977, Reference Patwardhan and Kulkarni1979a–Reference Patwardhan and Kulkarnic; Patwardhan & Nagarkar Reference Patwardhan and Nagarkar1979; Archer Reference Archer1999, Reference Archer2000, Reference Archer2001a–Reference Archere, Reference Archer2003a, Reference Archerb, Reference Archer2005, Reference Archer2006, Reference Archer2007; Nakanishi et al. Reference Nakanishi, Kashiwadani and Moon2003a, Reference Nakanishi, Kashiwadani and Moonb; Makhija & Adawadkar Reference Makhija and Adawadkar2005a, Reference Makhija and Adawadkarb; Makhija et al. Reference Makhija, Dube, Adawadkar and Chitale2005; Adawadkar & Makhija Reference Adawadkar and Makhija2006, Reference Adawadkar and Makhija2007). More than 600 type specimens were revised, chiefly from the herbaria in B, BM, FH, G, H, M, MSC, S, TUR, UPS, US, and W. For authors and synonymy of the species included in this study, see the world key provided by Lücking et al. (Reference Lücking, Archer and Aptroot2009; this volume).
Morphology, anatomy and chemistry
Material was analyzed at the herbaria mentioned above and, to a large extent, at The Field Museum, using a LEICA MS5 (×6·3–40) dissecting microscope and a ZEISS AXIOSCOPE 2 compound microscope. SIGMA Lugol's solution was used to test for ascospore amyloidity. Secondary substances were analyzed using thin-layer chromatography (TLC), following standard procedures (Culberson & Kristinsson, Reference Culberson and Kristinsson1970; Culberson, Reference Culberson1972; Walker & James Reference Walker and James1980; Lumbsch, Reference Lumbsch, Kranner, Beckett and Varma2002), and solvent C was routinely used for TLC analyses. Type material was not analyzed if reliable annotations of previous TLC analyses were present and no conflicting data existed as to the chemistry of the corresponding taxon.
Where TLC was not available or impossible due to limited material, microchemical reactions were performed under the microscope using a 10% KOH solution (to test for the presence of norstictic, salazinic, or stictic acid) and under the dissecting microscope using Steiner's PD solution (to test for the presence of protocetraric acid). For microchemical reactions, thin hand-cut sections of thallus and lirellae were used and KOH was applied from the side to which the upper surface of the thallus section pointed. A pale to bright yellow efflux on that side that remained yellow (observed over five minutes) without formation of crystals indicated stictic acid, whereas a bright yellow efflux eventually producing red, needle-shaped crystals (immediately or sometimes up to 60 seconds later) indicated norstictic (large crystals) or salazinic acid (small crystals). In some cases, the bark substratum reacts with a yellow efflux to KOH, but the colour is more orange-yellow and only appears on the lower (bark) side of the thallus; with some experience, the characteristic stictic-yellow can be readily recognized. PD was applied on small pieces of thallus from which the cortex was carefully scraped off to expose the medulla; orange-red colour indicated protocetraric acid. In a test of 360 samples using both TLC and microchemical and spot tests, the latter predicted the major compounds present (or nil) accurately in 357 samples (99% accuracy); in one case, KOH gave a false negative on norstictic acid (very low concentration found by TLC), in one case a false positive on stictic acid (apparently the bark substratum had a substance reacting to KOH in the same way as stictic acid), and in a third case, the microchemical test suggested salazinic acid and TLC revealed the presence of three major substances (norstictic, salazinic, and protocetraric acid). It should be noted that microchemical and spot tests can only be used to routinely test for the presence of a known chemosyndrome but cannot replace a detailed chemical analysis including minor and trace substances, as well as unknown chemosyndromes.
Variability was assessed on the individual, species, and character level as follows: each specimen was checked for apparent damage such as necrotic areas, discolorations due to inappropriate drying or degrading secondary substances, feeding traces and abrations, postmature ascomata without hymenia and collapsed ascospores. Only specimens not showing such damage, and in particular recently collected material, were taken into consideration when assessing characters such as colour, pruinosity, surface structure, ascospore pigmentation and amyloidity. For infra-individual variability, on each specimen lirellae of different ages from the thallus periphery to the thallus centre were sectioned to assess variation in lirella morphology and anatomy, such as excipular carbonization and striation, and ascospore size. For infra-specific variability, selected species or species groups were used which were easily characterized by unique features. For example, all specimens belonging to Graphis chrysocarpa and relatives (the only large-spored species with orange pigmented lirellae) were analyzed with respect to emergence, size, and thallus cover of the lirellae, as well as other characters. In Graphis chrysocarpa, it was found that specimens varied to a certain extent with regard to length and branching of the lirellae, as well as the presence of striation, but all specimens invariably had a white-grey thallus, sessile lirellae when mature, a completely carbonized excipulum, a clear hymenium, very large muriform ascospores, and no chemistry except for the presence of anthraquinones. Other species used for this assessment included G. illinata, G. lumbricina, G. mexicana and G. rhizocola, among others. Although species can have a different degree of variation, this assessment suggests that variability is limited and for example no species can include both specimens with completely immersed and sessile lirellae at maturity. Data from an ecological analysis of nearly one hundred Costa Rican species of Graphis (Lücking et al. Reference Lücking, Chaves, Sipman, Umaña and Aptroot2008a) were used to assess possible correlations between environmental parameters and lirellae morphology, in particular lirella emergence and thalline margin. Since for most other collections no precise ecological data were available, a mixed-collections approach was used to assess whether substratum type or microclimatic conditions affect character variation (see below).
Photographs were taken in the laboratory using a Nikon Coolpix 5400 digital camera connected to the Leica dissecting microscope and in the field with Canon Powershot A620, A630, and A650 cameras.
Taxa and characters
A total of 313 accepted species were used as taxonomic units in this study. For each species, 48 characters were observed and scored. This included all characters of potential value for taxonomic purposes, either used in other studies or novel characters. Character states were coded using integer values (Table 1). Ordered multistate characters were either maintained as such for calculating linear correlations or transformed into binary series for the numerical and cladistic analyses (Wiley et al. Reference Wiley, Siegel-Causey, Brooks and Funk1991; Poe & Wiens Reference Poe, Wiens and Wiens2000). Thus, a 1-2-3-4 ordered multistate character was transformed into a binary series as follows: 1 = 1-0-0-0, 2 = 1-1-0-0, 3 = 1-1-1-0, 4 = 1-1-1-1. Unordered multistate characters (e.g., secondary chemistry) were transformed into binary characters (e.g., presence versus absence of a particular substance). Continuous characters were scored as discrete categorical characters by defining lower and upper limits for each category (Table 1). For morphometric data such as lirellae and ascospore length and width, frequency distributions of 1000 original measurements of 100 randomly selected species (10 per species) were constructed and categorical limits were set to reflect closely the shape of the multimodal distributions. For example, ascospore sizes frequently fall into the ranges 30–40 μm, 70–80 μm, and 130–170 μm, with local minima in between, following a logarithmic pattern, and consequently, categorical limits were set to 20–50 μm, 50–100 μm and 100–200 μm.
For each species, only one character state was allowed. For continuous characters transformed into discrete states, in most cases the allowed range for a given state accommodated variation observed in a given species. If variation was detected in a species that would have encompassed more than one state for a given character (excluding ontogenetic variation), the state that was most frequently observed in thalli with mature ascomata was adopted. For example, species may show variation of (mature) lirella emergence encompassing two states, such as immersed to erumpent or prominent to sessile. If most specimens had immersed rather than erumpent (sessile rather than prominent) lirellae when mature, immersed (sessile) was adopted as the state for the species.
Depending on the type of analysis, the dataset was either used in full or partial datasets of 167 selected species and/or 27 selected characters were used for particular analyses (see below).
Statistical analysis
For correlations, non-parametric tests including Spearman rank correlation, Mann-Whitney U test, observed versus expected Chi-Square test, discriminant analysis, and Monte Carlo simulations, were employed. Discriminant analysis was performed on a subset of 167 species and 27 characters. Each of the 27 characters was used to divide the 167 species into groups representing the allowed states of that character (grouping variable), and the remaining 26 characters were used as dependent variables. Discriminant analysis tests how the selected character (grouping variable) explains the distribution of the remaining characters (dependent variables) among the groups of species defined by the states of the selected character (grouping variable). For example, ‘thallus cortex’ as grouping variable divides the species into two groups, one with cortex and the other without (see Table 1). Discrimant analysis tests whether the states of the dependent variable ‘labium pruina’ are distributed in a random or non-random manner among the two groups; if non-random, a correlation between the two characters can be assumed.
To test for potential influence of substratum and microclimatic conditions on thallus and lirella morphology without having such data at hand, the mixed-collections approach was used. One hundred collections where two species were growing side by side were randomly selected and each species was scored for three thallus characters (colour, cortex, surface) and two lirella characters (emergence, thalline margin). For each co-occurring species pair, the difference in character states was observed: if one species had prominent and the other one immersed lirellae, the difference was 2 (see Table 1). Differences over the 100 pairs were summarized in a frequency distribution curve. The whole dataset was then shuffled, i.e. species were randomly paired with other species, and the character state differences were recalculated. This Monte-Carlo approach was repeated 100 times to create a random frequency distribution of the data. The distribution based on the real data was compared to the random distribution and tested for significant difference using a Mann-Whitney U test. If there are substratum or microclimatic constraints on thallus and lirellae morphology, such as a soft substratum causing a thicker thallus with immersed lirellae in a species that usually has a thin thallus with prominent lirellae, one would assume that character state differences between co-occurring species are lower than expected by chance. In other words, if substratum type drives thallus and lirella morphology, it would be less likely to observe two species growing together, one of which having a thin thallus with sessile lirellae lacking a thalline margin and another with a thick thallus with immersed lirellae completely covered by thallus.
Species were ordinated based on the full set of weighted characters (see below) using non-metric multidimensional scaling (NMS) with Euclidean distance measure. NMS was performed with 500 runs with real data and the maximum number of iterations per run set to 250. The number of axes extracted was set to three and the stability criterion to 0·00001.
Statistical and multivariate analyses were performed using STATISTICA 6.0 and PC-ORD 5.03 (McCune & Mefford Reference McCune and Mefford1999; McCune & Grace Reference McCune and Grace2002).
Character weighting
Characters were weighted based on independent assessments of character correlations and homoplasy level. Character correlations were extracted from the discriminant analysis and from Spearman rank correlations and observed versus expected Chi-Square tests between characters. Three types of correlations can be distinguished: 1) autocorrelations that refer to double coding of the same character, 2) functional correlations, and 3) evolutionary correlations by shared ancestry. Autocorrelations by means of double coding of the same character are to be avoided by defining logically independent characters, as done here. Functional correlations include those in which one character will affect another character to a certain extent but not necessarily so. For example, ascospore size is functionally correlated with the number per ascus, but not in all cases do large ascospores occur singly in an ascus; in some species, such as Graphis acharii, an ascus can produce 8 large to very large ascospores. Therefore, such characters have to be coded separately but must be downweighted in numerical and cladistic analyses, as their correlation has little signal in terms of evolutionary relationships. On the other hand, correlations by shared ancestry carry a significant evolutionary signal and can be recognized in the lack of apparent functional relationships. The frequent co-occurrence of pigmented lirellae, a completely carbonized excipulum, and large ascospores does not appear to have any functional component and instead is likely to be explained by shared ancestry. Characters with predominantly non-functional correlations should therefore receive a higher weight in the analysis.
Homoplasy is usually assessed by the distribution and change of character states over a tree. However, weighting characters a posteriori based on homoplasy indices bears the danger of circular argument. Therefore, a novel method to assess homoplasy prior to a cladogram was used here. Total character distances (Manhattan distances) based on all 48 characters were calculated pairwise for each possible taxon pair, resulting in a 313 × 313 = 97969 cell large distance matrix. Subsequently, cladistic distances were calculated for each possible taxon pair but using each character separately, resulting in 48 individual matrices 313 × 313 = 97969 cells in size. All 49 matrices were converted into tabular form and the 48 individual character distance matrices were each multiplied with the overall character matrix. For each multiplied matrix, the weighted average was computed and the overall weighted average was substracted from this value to result in a homoplasy index for each character. A Monte-Carlo simulation was applied to test for significant differences, by randomly resampling 1000 values from the overall distance distribution and each individual character distance distribution (1000 replicates) and comparing the two resulting distributions using mean, standard deviation, and number of observations. For instance, the overall character distance matrix resulted in Manhattan distances 1-15-8-26-17 for five taxon pairs. The individual distance matrix for character ‘lirellae emergence erumpent’ (binary form) resulted in Manhattan distances 0-1-0-1-1 for the same pairs. This means that taxon pairs exhibited differences in this character when overall differences were high (15-17-26) but no differences when overall differences were low (1-8). The resulting homoplasy index in this case would be 0 × 1 + 1 × 15 + 0 × 8 + 1 × 26 + 1 × 17 / (0 + 1 + 0 + 1 + 1) = 19·3 (weighted average for the character) − [(1 + 15 + 8 + 26 + 17) / 5] = 13·4 (overall average for all characters) = 5·9. Highly homoplastic characters, by definition, vary frequently between closely related species (small overall cladistic distance) whereas barely homoplastic characters vary more between distantly related species. Thus, the resulting homoplasy index will be comparatively low for highly homoplastic characters (close to zero or even negative) and comparatively high for barely homoplastic characters. A method to assess the level of homoplasy prior to a phylogeny in molecular sequence data was presented by Dress et al. Reference Dress, Flamm, Fritzsch, Grünewald, Kruspe, Prohaska and Stadler(2008); however, the algorithm used in that method is more complex than the one used here and specifically fits molecular data.
Based on correlation and homoplasy analyses, characters were assigned final weights 1 (low level of non-functional correlation and high level of homoplasy detected), 2 (intermediate level of non-functional correlation and homoplasy), and 4 (high level of non-functional correlation and low level of homoplasy; Table 1).
Cladistic analysis
The partial dataset of 167 species plus one outgroup taxon (either Fissurina dumastii or Diorygma minisporum) and the full dataset of 48 weighted characters (Table 1) was subjected to cladistic analysis using minimum evolution (ME). Searches were done using tree-branching-regrafting (TBR) as branch swapping algorithm, with MulTrees option in effect. Branch support was calculated using Jackknife support with 10% deletion and 1000 Jackknife replicates. Because of the generally high level of homoplasy in morphological (phenotype) versus molecular (genotype) data, bootstrapping or a corresponding Jackknife support with 50% deletion usually do not give usable results; on the other hand, Jackknife support with 10% deletion cannot be directly compared to bootstrapping, as it will give higher values; it is more to be seen as providing a crude estimate of the internal consistency of the data. The two alternative outgroups were selected based on molecular studies (Staiger et al. Reference Staiger, Kalb and Grube2006; Mangold et al. Reference Mangold, Martín, Lücking and Lumbsch2008), with Fissurina as basal lineage in the Graphidaceae and Diorygma as close relative of Graphis. Either outgroup was used alternatively to test for potential differences in tree topology.
Cladistic analysis was performed in PAUP* 4.0b10 (Swofford Reference Swofford2003) and tree drawing in MESQUITE 2.5 (Maddison & Maddison Reference Maddison and Maddison2008).
Results
Thallus morphology
The thallus in Graphis is rather uniform, most species having a white-grey colour caused by abundant calcium oxalate crystals in or above the photobiont layer, a smooth to uneven surface, and a more or less dense, prosoplectenchymatous cortex (Fig. 1A). A few species, such as G. caribica (Fig. 2A), G. endoxantha, G. fournierii and G. olivacea, have an olive thallus reminiscent of other genera (Glyphis, Phaeographis). This colour does not correlate with the absence or presence of a cortex but with the distribution of the calcium oxalate crystals: in most species of Graphis, the crystals form a thick, almost continuous layer with the photobiont cells dispersed in between or underneath the crystal clusters, whereas in species with an olive thallus, the photobiont layer is continuous below the cortex and the crystal clusters are located below the photobiont layer (Fig. 1B). Several species feature a distinctly verrucose thallus, such as G. granulosa (Fig. 2B), caused by more or less regularly dispersed, large accumulations of calcium oxalate crystals. Ecorticate thalli are characteristic of G. glaucescens (Fig. 2C) and G. farinulenta and relatives; in these, the crystal layer with the photobiont cells intermixed is exposed and gives the thallus a farinose appearance. Vegetative dispersal is extremely rare: soredia are found in only one species, G. sorediata, whereas there are three isidiate taxa, G. patwardhanii (Fig. 2D), G. isidiata (Fig. 2E), and G. isidiza. Species that are well-characterized by morphological and anatomical features of their ascomata, such as G. chrysocarpa, G. illinata, G. lumbricina, G. mexicana and G. rhizocola, have rather uniform thalli with a narrow range of variation, indicating that thallus morphology (colour, surface, cortex, vegetative dispersal) is species-specific and of taxonomic importance. Among the sorediate and isidiate species, only G. isidiata has a non-isidiate counterpart (‘species pair’) agreeing in all other characters with G. mexicana (Fig. 2F). Discriminant analysis shows a strong correlation between the characters thallus cortex and labium pruina (Table 2); ecorticate species more frequently have pruinose labia. Otherwise, the thallus does not correlate strongly with any other character.

Fig. 1. Thallus anatomy in species of Graphis. A, G. acharii, section through thallus showing upper cortex, clusters of calcium oxalate crystals, and photobiont cells (arrows); B, G. caribica, section through thallus showing upper cortex, photobiont layer (arrows) and clusters of calcium oxalate crystals. Both images to same scale (images are 200 μm high).

Fig. 2. Thallus morphology in species of Graphis. A, G. caribica, olive-green thallus (dark), next to G. intricata with white-grey thallus; B, G. granulosa, verrucose thallus (covering also the lirellae); C, G. glaucescens, ecorticate, minutely farinose thallus; D, G. patwardhanii, isidiate thallus (arrows) with lirellae; E, G. isidiata, isidiate thallus with round lirellae; F, G. mexicana, non-isidiate thallus with round lirellae. Scales = 1 mm.
Table 2. Discriminante analysis of 27 selected characters. Three asterisks*** denote highly significant discrimination, one asterisk* denotes significant discrimination, using the column character as grouping variable. F and P-level indicate the overall level of discrimination of each character. Note that the matrix is not exactly symmetrical since the behaviour of characters as grouping (columns) or dependent (rows) variables is not necessarily identical. Cells shaded in grey indicate highly significant non-functional correlations between characters whereas framed boxes indicate groups of functionally correlated characters)

† CORT = thallus cortex, EMER = lirella emergence, MARG = lirella thalline margin, THIC = lateral margin thick, SHAR = labium sharply delimited, LENG = lirella length, WIDT = lirella width, RELA = length to width ratio, BRAN = lirella branching, PRUI = labium pruina, PIGM = labium pruina yellow or orange, EXPO = disc exposure, STRI = labium striation, CARB = excipulum carbonization, INSA = hymenium inspersion A, INSB = hymenium inspersion B, NUMB = ascospore number, LENG = ascospore length, WIDT = ascospore width, RELA = length to width ratio, TRAN = septa transversal, LONG = septa longitudinal, NORS = chemistry norstictic, STIC = chemistry stictic, SALA = chemistry salazinic, PROT = chemistry protocetraric.
Lirella morphology
Lirella morphology is composed of five characters with a total of 4 × 5 × 5 × 5 × 2 = 1000 − 1 × 2 × 5 × 5 × 2 (certain combinations are not possible, see below) = 900 possible character state combinations (Table 3): 1) emergence, 2) thalline margin, 3) relative length, 4) degree of branching, and 5) labia striation. Emergence plus thalline margin (1 & 2) are here referred to as lirella type combinations, whereas length and degree of branching (3 & 4) represent lirella shape combinations and all five together (1–5) lirella morph combinations. Of the 900 possible state combinations, only 107 have actually been found in the 313 species included in this study, suggesting that certain constraints strongly limit character state combinations in Graphis lirellae.
Table 3. Character states regarding lirella emergence, thalline margin, relative length, branching, and striation, and the theoretically possible number of free combinations (last row) in the genus Graphis

Lirella emergence has four character states (Figs 3 & 4): immersed (upper part of lirellae ± level with thallus surface; e.g., Graphis immersa), erumpent (± upper half of the lirellae raised above thallus surface and lower half immersed; e.g., G. lineola), prominent (largely raised above thallus surface but basally not constricted; e.g., G. tumidula), and sessile (fully raised above thallus surface and basally constricted; e.g., G. adpressa). More than half of the species feature erumpent and about one third have prominent lirellae (Fig. 5A), suggesting a strong bias towards these two character states, whereas immersed and sessile lirellae are infrequent. A similar bias is observed with respect to thalline margins (Figs 3 & 4), which can be complete and thick (e.g., G. rhizocola), complete but apically thin with the upper part of labia dark grey (e.g., G. argentata), lateral with upper part of labia black (e.g., G. lineola), basal (e.g., G. geraensis), or absent (e.g., G. adpressa). Almost half of the species feature a lateral thalline margin, whereas a complete (thick), complete (thin), basal, or absent thalline margin are each found in less than 10–20% of the taxa (Fig. 5B).

Fig. 3. Variation in the genus Graphis. A, lirella emergence; B, thalline margin; C, excipulum carbonization. (Schematic; from Lücking et al. Reference Lücking2008).

Fig. 4. Types of lirellae in the genus Graphis. A, G. immersa, immersed lirellae with apically complete (thick) thalline margins; B, G. lineola, erumpent lirellae with lateral thalline margins (upper part of labia black); C, G. geraensis, prominent lirellae with basal thalline margins; D, G. adpressa, sessile lirellae lacking thalline margins; E, G. tumidula, prominent lirellae with complete (thick) thalline margins; F, G. argentata, prominent lirellae with complete (thin) thalline margins (upper part of labia dark grey). Scales = 1 mm.
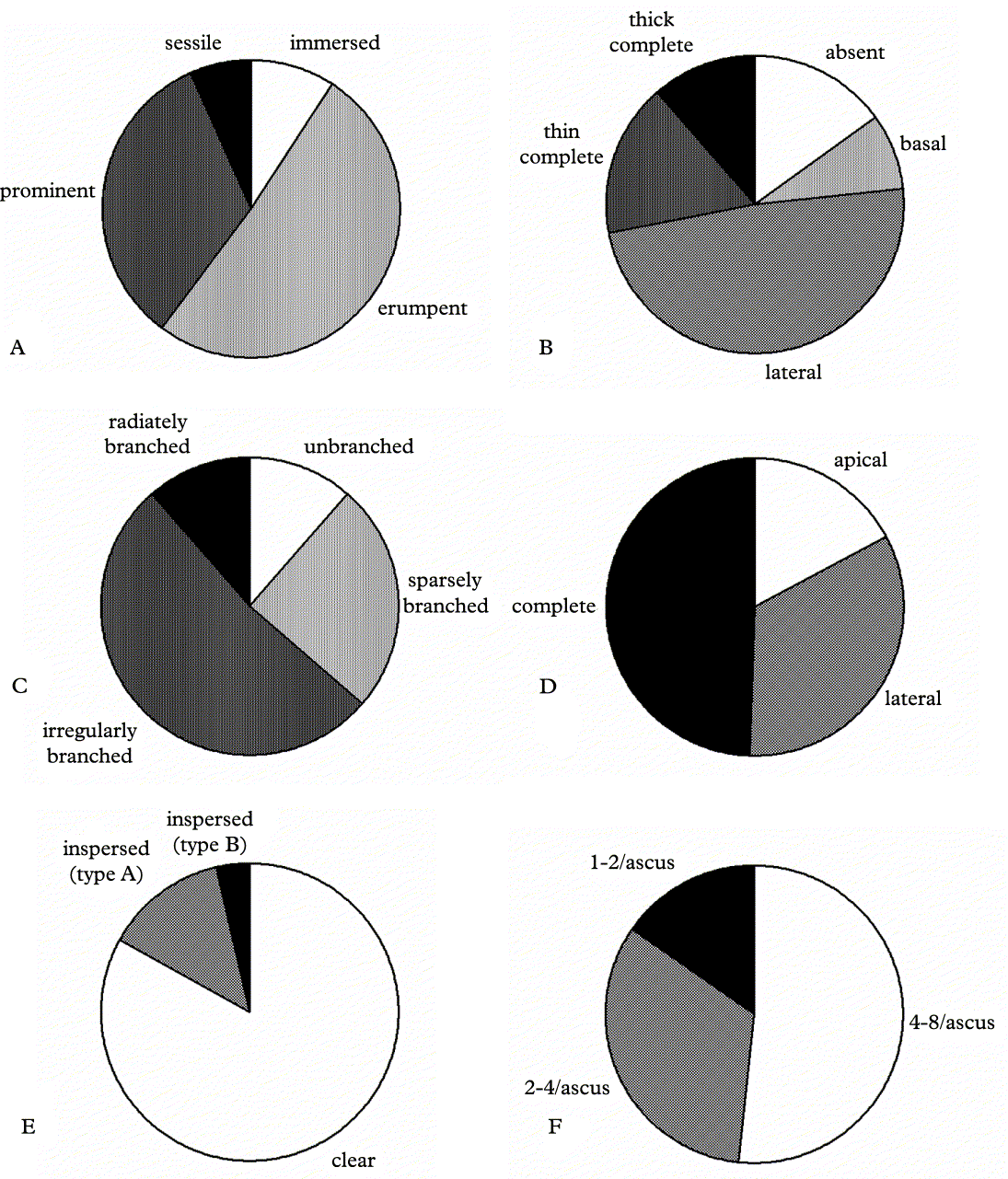
Fig. 5. Proportion of species having different types of lirella emergence (A), thalline margin (B), branching (C), excipulum carbonization (D), hymenium inspersion (E), and ascospores per ascus (F).
Lirellae emergence and thalline margin result in theoretically 4 × 5 = 20 possible combinations, but since immersed lirellae must have at least a lateral thalline margin, two combinations (‘immersed-basal’ and ‘immersed-absent’) are not possible. The remaining 18 combinations are all found in the genus (Fig. 6), in at least one species (sessile-thin in Graphis nudaeformis), with the most frequent combination being ‘erumpent-lateral’ (e.g., G. lineola and G. scripta) found in 101 species (about 30%). A Chi-Square test shows a highly significant deviation of the relative frequency of theobserved combinations from chance (Table 4). Species with ‘immersed-lateral’ (e.g., G. saxiseda), ‘erumpent-lateral’ (e.g., G. lineola), ‘prominent-thin’ (e.g., G. cinerea), ‘prominent-absent’ (e.g., G. striatula), and ‘sessile-absent’ (e.g., G. adpressa) are more frequent than expected by chance, whereas species with ‘erumpent-thick’ (e.g., G. superans), ‘erumpent-absent’ (e.g., G. duplicata), ‘prominent-basal’ (e.g., G. cupei), and ‘sessile-lateral’ (e.g., G. plagiocarpa), are less frequent. However, although one would assume more prominent lirellae to be less frequently covered by a thalline margin, there is no monotonous correlation between lirellae emergence and thalline margin (R Spearman = 0·09, P = 0·119). This can also be deduced from the frequency of certain combinations: in prominent lirellae, both forms with complete (thin) and with absent thalline margin are more frequent than expected by chance, and in erumpent lirellae, both forms with complete (thick) and absent thalline margin are less frequent. Both lirella emergence and thalline margin are highly discriminative against each other and against labium pruina, labium striation, and excipulum carbonization (Table 2).

Fig. 6. Lirella types and lirella morphs in the genus Graphis. A, G. saxiseda, ‘immersed-lateral’, stellate, entire (coarctata morph); B, G. symplecta, ‘immersed-complete’ (thin), irregular, striate (symplecta morph); C, G. sulphurella, ‘immersed-complete’ (thick), irregular, entire (subserpentina morph); D, G. duplicata, ‘erumpent-absent’, irregular, striate (striatula morph); E, G. proserpens, ‘erumpent-basal’, irregular, striate (striatula morph); F, G. cincta, ‘erumpent-lateral’, sparsely branched, entire (lineola morph); G, G. negrosina, ‘erumpent-complete’ (thin), irregular, entire (negrosina morph); H. G. superans, erumpent-complete (thick), sparsely branched, entire (subserpentina morph); I. G. striatula, ‘prominent-absent’, irregular, striate (striatula morph); J. G. cupei, ‘prominent-basal’, irregular, entire (hossei morph); K. G. flavens, ‘prominent-lateral’, irregular, entire (marginata morph); L. G. cinerea, ‘prominent-complete’ (thin), sparsely branched, striate (acharii morph); M. G. illinata, ‘prominent-complete’ (thick), irregular, entire (illinata morph); N. G. adpressa, ‘sessile-absent’, unbranched, entire (nuda morph); O. G. slendrae, sessile-basal, irregular, entire (hossei morph); P. G. plagiocarpa, ‘sessile-lateral’, unbranched, entire (dussii morph); Q. G. nudaeformis, ‘sessile-complete’ (thin), unbranched, entire (nudaeformis morph); R. G. granulosa, ‘sessile-complete’ (thick), unbranched, striate (granulosa morph).
Table 4. Observed versus expected frequency of lirella type combinations (emergence and thalline margin; see Fig. 5) in the genus Graphis and observed versus expected frequency of entire and striate labia in each type

The degree of lirella emergence and thalline margin does not correlate with environmental parameters except for an altitudinal and seasonality gradient: species with prominent to sessile lirellae are more frequently found at higher altitudes (R Spearman = 0·46, P < 0·001), whereas species with immersed to erumpent lirellae are more common in seasonally dry climates (R Spearman = −0·44, P < 0·001). However, no such correlation is found with thalline margin, as can also be deduced from the fact that high altitude species are dominant among those with ‘prominent-complete thin’ and ‘sessile-absent’ type lirellae. The mixed-collections approach did not result in a significant difference between the observed distribution of lirella type characters and one occurring by chance.
The bulk of species is evenly divided into taxa with short (up to 3 mm), elongate (up to 5 mm), and very long (up to 15 mm, in extreme cases up to 80 mm) lirellae (Fig. 7). Very short lirellae (up to 2 mm) are also common, and some species, such as Graphis mexicana, feature round lirellae with slit-like openings (Fig. 7A). Branching varies greatly among Graphis species (Fig. 7), with more than half of the taxa having irregularly branched lirellae (Fig. 5C). Radiate or stellate branching is found in little more than ten percent of the species (e.g., G. centrifuga, G. dendrogramma, G. stellata). In G. hyphosa, the lirellae form stellate clusters immersed in pseudostromata (Fig. 7F). About ten percent of the species feature unbranched lirellae, whereas 25% have sparsely branched lirellae. Not unexpectedly, there is a very strong positive and highly significant (functional) correlation between relative length of the lirellae (length related to width) and the degree of branching (R Spearman = 0·75, P < 0·001); longer lirellae tend to be more frequently and sometimes even radiately branched. This is also apparent from the discriminant analysis (Table 2). There is no correlation between lirella type (emergence and thalline margin) and lirella shape (relative length and branching). However, when comparing lirella emergence alone with lirella shape (relative length and branching), a highly significant negative correlation is found; the stronger the emergence, the less the relative length (R Spearman = −0·23, P < 0·001) and degree of branching (R Spearman = −0·33, P < 0·001). This correlation cannot be interpreted as functional, since there is no reason to assume that more emergent lirellae should be shorter and less branched.

Fig. 7. Lirella shapes and lirella morphs in the genus Graphis. A, G. mexicana, round and unbranched (globosa morph); B, G. cleistomma, very short and unbranched (cleistomma morph); C, G. handelii, short and sparsely branched, with exposed disc (handelii morph); D, G. glaucescens, elongate and irregularly branched (glaucescens morph); E, G. dendrogramma, very long and radiately branched (dendrogramma morph); F, G. hyphosa, stellate clusters in pseudostromata (hyphosa morph). Scales = 1 mm.
Labia can appear entire (Fig. 4B–E) or striate (Fig. 4F), the latter mostly due to the formation of new hymenia and excipula within old lirellae and hence believed to be an ontogenetic rather than a taxonomic feature. A strictly ontogenetic feature would imply absence of correlation between lirella morphology and striation. However, the frequency of certain combinations of lirella type and striation significantly deviates from chance (Table 4). Thus, entire labia are more frequently found in ‘immersed-lateral’, ‘erumpent-lateral’, ‘prominent thick’, and ‘sessile-absent’ lirellae. Striate labia, on the other hand, are more frequent in ‘erumpent-thin’, ‘erumpent-absent’, ‘prominent-thin’, and ‘prominent-absent’ lirellae. Notably, lirellae with lateral and complete (thick) thalline margin more frequently have entire labia, whereas those with complete (thin) margin more frequently have striate labia; in lirellae lacking a thalline margin, erumpent and prominent lirellae tend towards striate labia whereas sessile lirellae are always entire. No correlation is found when a combination of lirellae emergence, thalline margin, relative length, and branching are compared with labia striation (χ2 = 126·6, P = 0·909). Discriminant analysis shows only a weakly discriminative power for striate labia, with the best correlation found for lirellae emergence (Table 2).
Pruinose labia (Fig. 7D) are found in 32 species of Graphis, roughly ten percent of all taxa. Exposed discs (Fig. 7C) are comparatively rare, being present in 18 species, including the type species, G. scripta. There is a clear concentration of pruinose labia among species with lateral thalline margins, particularly with ‘immersed-lateral’ (10 species) and ‘erumpent-lateral’ lirellae (10 species); this correlation is, however, only marginally significant (χ2 = 48·2, P = 0·067). Similarly, almost all species with exposed discs are found in ‘immersed-lateral’ (three species) and ‘erumpent-lateral’ lirellae (13 species). Due to the overall low number of taxa with exposed discs, this correlation is not significant. In the discriminant analysis, labium pruina and disc exposure show intermediate discriminative power, with correlations with thallus cortex and lirella emergence (Table 2).
There are very few instances in which species can be separated by lacking or having basal thalline margins; on the other hand, certain species or groups of species (e.g., Graphis dussii, G. marginata and G. subserpentina) are characterized by a conspicuously thick, sometimes bulging lateral thalline margins (Fig. 6P). Merging lirella types with an absent and basal thalline margin but separating those with lateral and lateral thick thalline margin results in 19 possible lirella types, which can be combined with lirella shape (relative length and branching) and striation, as well as labium pruina and disc exposure, to 19 × 5 × 2 × 2 × 2 = 760 free combinations of lirella morphs (190 basic lirella morph combinations and 570 corresponding morphs with pruinose labia and/or exposed disc). Yet, the majority of these are not realized in the genus, and there are only 65 commonly found basic lirella morphs, with some additional rare morphs found in one species each (Table 5; see also Figs 6 & 7). Only 22 corresponding morphs have labium pruina and/or exposed discs and these are clearly concentrated among lirella types with an erumpent thalline margin and with entire labium. For instance, the basic deserpens morph (lirella erumpent, with lateral thalline margins, elongate and irregularly branched) has four corresponding morphs with pruinose labia (caesiella), pruinose labia and ecorticate thallus (glaucescens), exposed disc (handelii), and exposed, pruinose disc (scripta).
After assessing variation and continuity, certain morphs were combined under a single name, resulting in 24 commonly found, named basic morphs and eight commonly found, named corresponding morphs with pruinose labia and/or exposed disc (Table 5). Nine morphs (lineola, deserpens, centrifuga, coarctata, subserpentina, dichotoma, symplecta, and consanguinea) include both immersed and erumpent lirellae; three others (nuda, dussii and globosa) encompass prominent and sessile lirellae, and in two cases (hossei and striatula), contiguous variation was observed between erumpent and prominent lirellae. The subserpentina morph includes species with laterally thick to complete thalline margin; this is because in immersed lirellae of this group, the bulging lateral margin often converges above the lirellae to appear complete. Graphis glaucescens is the most common of a handful of species with pruinose labia and ecorticate thallus and usually has entire lirellae, but with striate lirellae often found in the centre of the thallus; in this case, both the entire and striate form were called glaucescens morph.
There are clear constraints regarding variation; morphs that include immersed and erumpent lirellae are only found in lirellae with lateral to complete thalline margins, whereas those with erumpent to prominent lirellae are restricted to lirellae with absent to basal thalline margins. Morphs that encompass prominent to sessile lirellae are found only in rounded to very short and unbranched lirellae (Table 5). In morphs with immersed to erumpent lirellae with lateral thalline margins and entire labia, the lirellae usually exhibit a rather uniform shape on a single thallus, and hence, three morphs are distinguished based on lirella shape: lineola (short and sparsely branched; see Figs 6F & 7C), deserpens (elongate and irregularly branched), and centrifuga (very long and radiately branched; see Fig. 7E). In morphs with erumpent to prominent lirellae with absent or basal thalline margins and striate labia, on the other hand, lirella shape varied greatly on individual thalli and hence all have been merged under the striatula morph (Table 5).
The most common morphs are, in descending order of frequency: striatula morph (27 species), subserpentina morph (27), deserpens morph (20), lineola morph (19), acharii morph (18), hossei morph (18), symplecta morph (15), tenella morph (14), caesiella morph (11), dussii morph (11), illinata morph (11), nuda morph (11), scripta morph (11), centrifuga morph (9), marginata morph (8), coarctata morph (7), negrosina morph (7), chrysocarpa morph (6), rhizocola morph (6), consanguinea morph (5), glaucescens morph (5), dichotoma morph (4), globosa morph (4), and farinulenta morph (3). The most distinctive is the nuda morph, which closely resembles the genus Melaspilea. The hossei morph is similar to Opegrapha species.
Table 5. Lirellae morphs in the genus Graphis based on combinations of lirellae emergence, thalline margin, relative length, branching, striation, labia pruina, and disc exposure. If no clear separation was possible, contiguous morphs were combined into one name

* (e) = disc exposed, (ep) = disc exposed and pruinose, (f) = thalline margin flaking off, (p) = labia pruinose, (pe) = labia pruinose and thallus ecorticate, (pig) = labia pigmented.
Lirella anatomy
Lirellae of Graphis species are anatomically characterized by three principal characters: 1) excipulum crenation, which is externally visible as labia striation (see above), 2) the degree of excipulum carbonization (Fig. 3, 8), and 3) the presence or absence of hymenium inspersion. The excipulum is developed laterally and often also basally below the hypothecium. Species with lateral excipulum are apically to laterally carbonized (with the base of the lateral excipulum ± widely separate), whereas taxa with basally continuous excipulum can have apical, lateral, or complete carbonization, often with intermediate stages. Three species (G. gregmuelleri, G. immersoides, and G. mirabilis) are known with an apically and basally carbonized excipulum, whereas the lateral part is non-carbonized. Almost half of all Graphis species have a completely carbonized excipulum, about one third feature a laterally carbonized excipulum, and in the remaining species the excipulum is apically carbonized (Fig. 5D). There is a highly significant positive, possibly functional correlation between lirellae emergence and the degree of carbonization (R Spearman = 0·39, P < 0·001): species with more emergent lirellae tend to have a basally closed and completely carbonized excipulum. Sessile lirellae are almostexclusively completely carbonized. This suggests vertical growth of the basal excipulum with age, much like the vertical growth of the carbonized hypothecium in the genera Leiorreuma, Sarcographa and Thecaria.

Fig. 8. Excipulum carbonization and hymenium inspersion in species of Graphis. A, G. glaucescens, apically carbonized excipulum; B, G. pavoniana, laterally carbonized excipulum; C, G. gomezii, completely carbonized excipulum with basal part formed by carbonized substratum; D, G. rhizocola, completely carbonized excipulum; E, G. insulana, inspersed hymenium (type A); F, G. argentata, inspersed hymenium (type B); E and F to same scale (images are 100 μm wide).
A similar correlation is seen when comparing lirellae type (emergence and thalline margin) with the degree of carbonization (χ2 = 87·4, P = 0·002). Immersed lirellae with lateral thalline margin have a higher than expected frequency of apical carbonization only (9 out of 21 species), whereas in erumpent lirellae with lateral thalline margin, lateral carbonization is dominant (54 out of 101) and complete carbonization is rare (28 out of 101). The latter is also true of ‘immersed-lateral’ lirellae (4 out of 21). In prominent lirellae with complete (thin) thalline margin, the situation is reversed, complete carbonization being much more frequent than expected (24 out of 28) whereas lateral carbonization is much less frequent (3 out of 28). Overall, the discriminative power of excipulum carbonization is weak, although the correlation with lirellae emergence is obvious (Table 2).
Hymenium inspersion (Fig. 8E & F) comes in two types. In type A inspersion, small oil droplets are regularly lined along the paraphyses, the latter remaining readily visible in hand sections; the oil droplets persist after adding KOH. In type B inspersion, densely arranged, larger and irregular oil droplets completely obstruct the view of the paraphyses but the droplets rapidly dissolve when KOH is added. Both types are different from the inspersion found in the genus Phaeographis and its allies. While the majority of Graphis species lack inspersion, type A inspersion can be found in about 13% of the species, and type B in three percent (Fig. 5E). Type A inspersion is concentrated in ‘erumpent-lateral’ lirellae, with half of the inspersed species associated with this lirellae type. All other lirellae types have none or only a few species with type A inspersion. Due to the large number of species with ‘erumpent-lateral’ lirellae (101), this correlation is not significant. Type B inspersion is almost exclusively found in prominent lirellae with complete (thin) thalline margin; of the 11 species with this type of inspersion, nine are found associated with such lirellae. This correlation is highly significant (χ2 = 77·1, P < 0·001).
Ascospores
The following nine characters can be used to characterize ascospores in the genus Graphis: 1) number per ascus, 2) length, 3) width, 4) length to width ratio, 5) number of transverse septa, 6) number of longitudinal septa per segment, 7) external wall thickness, 8) pigmentation, and 9) endospore development. As expected, the numeric and morphometric ascospore characters show strong reciprocal discriminative power (Table 2) and are highly significantly correlated with each other (Table 6); the larger the ascospores, the smaller their number per ascus, and the longer (wider) the ascospores the higher the number of transverse (longitudinal) septa. However, as the strength of the correlations suggests (R Spearman between 0·26 and 0·82), there is no absolute redundancy in ascospore characters, and a combination of all characters is needed to describe properly ascospore features.
Table 6. Linear correlation (Spearman rank correlation) of ascospore characters in the genus Graphis

Whereas most species have (4–)8 ascospores per ascus, a reduction of the number of ascospores per ascus is commonly observed, with quite a number of species featuring single-spored asci (Fig. 5F). Most species have comparatively small ascospores, being 15–50 × 5–8 μm in size (Fig. 9B–D), although even in species with short ascospores, the ascospores are often rather wide (8–15 μm). Large (to very large) ascospores 100–250 × 15–50 μm in size (Fig. 9I) are found in about one third of all species. The proportion of species with different numbers of transverse septa (Fig. 9A–E) follows a similar pattern. The bulk of taxa lack longitudinal septa; a few species have submuriform ascospores with 0–3 longitudinal septa per segment, whereas a large proportion features truly muriform ascospores with 3–7 longitudinal septa per segment (Fig. 9G–K). A number of species feature a particular ascospore type with longitudinal septa present only in the terminal segment (Fig. 9F).

Fig. 9. Ascospore types in the genus Graphis. A, Graphis rhizocola (note the shortly tailed ends); B, Graphis ovata (ascospores to the right in KOH, note the change in lumina and wall structure); C, Graphis adpressa (note the thick outer wall and oval lumina); D, Graphis elegans (note the thick outer wall and oval lumina; ascospores to the right in KOH, note the change in lumina and wall structure); E, Graphis pittieri (ascospores to the right in KOH, note the change in lumina and wall structure); F, Graphis vestitoides (note the terminally muriform septation and apical gelatinous cap); G, Graphis ruiziana (note the rounded lumina); H, Graphis subruiziana (note the rounded lumina); I, Graphis myrtacea (note the reduced endospore and almost angular lumina); K, Graphis insulana (note the lack of endospore and angular lumina, as well as the inspersed hymenium). Scales = 10 μm.
While the majority of Graphis species have rather thin-walled, hyaline ascospores with distinct endospore and lens-shaped to rounded lumina, a few taxa deviate from this pattern: G. elegans and G. adpressa feature uncharacteristically thick-walled ascospores (Fig. 9C & D), whereas genuinely grey-brown (not overmature as sometimes found in G. chrysocarpa and G. phaeospora) ascospores are characteristic of G. mucronata and G. pittieri (Fig. 9E). Certain species with distinctly muriform ascospores, such as G. insulana and allies, have the endospore more or less reduced, and the ascospores appear almost euseptate. In other species with large, muriform ascospores, such as G. illinata and G. mexicana and allies, the endospore is ± well-developed.
Ascospore characters are weakly but highly significantly correlated with lirella type (Table 7). Species with more emergent (prominent, sessile) lirellae and those with a better developed thalline margin tend to have larger ascospores with more numerous septa. Regarding lirella emergence, this correlation appears to be functional, since larger ascospores need more space (hymenium height) to develop.
Table 7. Linear correlation (Spearman rank correlation) between lirella type and ascospore characters

Secondary chemistry
Secondary chemistry is comparatively simple in the genus Graphis; more than half of the species (162) lack secondary substances. By far the most common substances are norstictic and stictic acid and their satellites (connorstictic and constictic acid), together occurring in about one third of the taxa (Fig. 10). Other major but rare substances include salazinic, hypostictic, protocetraric, and hirtifructic acid, as well as lichexanthone. In 24 species, such as G. capillacea, G. litoralis and G. subasahinae, two or three major substances occur together, usually a combination of norstictic, stictic, salazinic, and/or protocetraric acid. Anthraquinone and other pigments are found in eleven species: tetrahydroxyanthraquinone-1,3,6,8 and satellite substances in G. chromothecia, G. chrysocarpa, G. inversa, G. lutea and G. subchrysocarpa, isohypocrelline in G. hypocrellina (basal excipulum) and G. persicina (hymenium), and unidentified yellow pigments in G. firferi, G. flavoaltamirensis and G. flavominiata. Species with erumpent lirellae with lateral thalline margins have a high frequency of secondary substances (61 out of 101 species), whereas those with prominent lirellae with complete (thin) thalline margins have a comparatively low frequency; however, overall this pattern is not significant. There is a remarkable concentration of species with secondary substances among those with lirellae featuring a lateral thalline margin (88 out of 152 or 58%), whereas those with complete (thin) thalline margins have secondary substances much less frequently (17 out of 53 or 32%); again, this difference is not significant. There is a weak and marginally significant positive correlation between the presence of secondary substances and the presence of labial pruina (R Spearman = 0·10, P < 0·070). This is also apparent in the discriminant analysis which otherwise indicates low discriminative power for secondary chemistry in terms of species groupings (Table 2).

Fig. 10. Proportions of species with chemical substances in the genus Graphis.
Homoplasy assessment
The phylogeny-independent homoplasy assessment suggests that the following characters and character states have a high level of homoplasy: thallus cortex (absent), lirella branching, labia pruinosity, disc exposure, labia striation, excipulum carbonization, hymenium inspersion (type A), and secondary chemistry, with the exception of the presence of anthraquinones (Table 8). Intermediate levels of homoplasy are found in the majority of ascospore characters, although some have a high (ascospores of intermediate size) and others, such as large ascospores with many septa, have a low degree of homoplasy. Generally low homoplasy was detected in lirella emergence, thalline margin (except basal), lirella length and width, and hymenium inspersion (type B). In these, deviation from a (homoplastic) chance distribution among taxa was significant, suggesting strong phylogenetic signal.
Table 8. Phylogeny-independent homoplasy assessment of the character states used in the phylogenetic analysis (complete binary transformation) in the genus Graphis

Mean = mean overall phylogenetic taxon distance at which a character differs in a given taxon pair; N = number of pairwise comparisons in which character differs in a given taxon pair; Index = difference from overall mean (homoplasy index); P-level = significance level from Monte-Carlo simulation; Weight = degree of deviation from mean.
Cladistic analysis
The cladistic minimum evolution (ME) analysis of 167 selected taxa of the genus Graphis plus one outgroup taxon, based on 48 phenotype characters, shows a rather well-resolved topology (Figs 11 & 12). Of the 48 characters, 42 are parsimony-informative. Backbone support and support for individual branches are mostly low to non-existent. This is due to the high level of homoplasy in the data (CI = 0·126, HI = 0·874), but also to the high number of taxa compared to the low number of characters available. Maximum parsimony (MP; not shown) resulted in a similar topology but with a lesser degree of monophyletic resolution at the base.
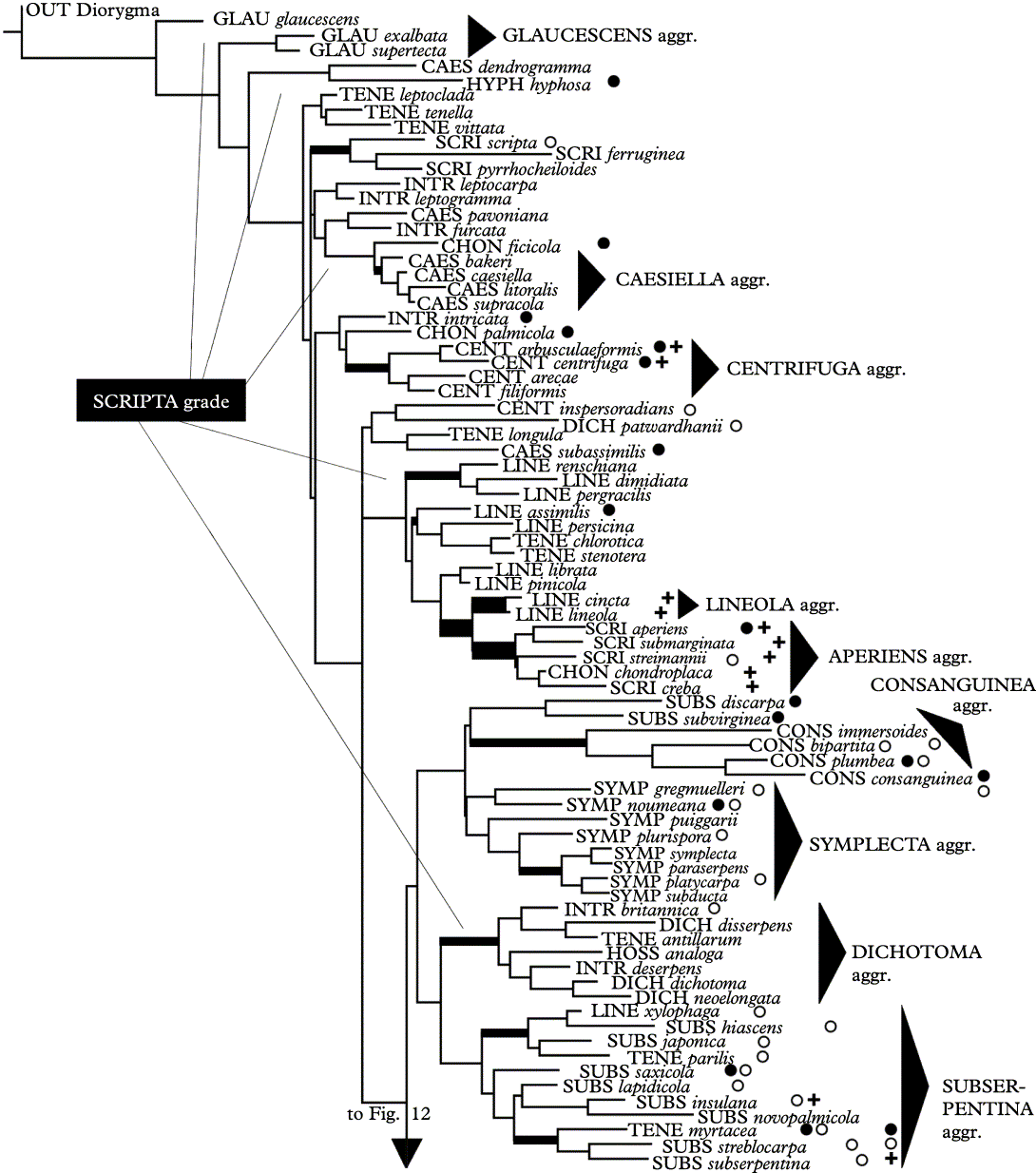
Fig. 11. First partition of shortest tree of 167 Graphis species plus outgroup Diorygma minisporum (48 characters) found using the ME criterion (heuristic search with 1000 replicates). Tree length = 823·885, CI = 0·126, HI = 0·874, number of parsimony-informative characters = 42. Thick branches indicate > 50% and very thick branches > 75% Jackknife support. •, species with completely carbonized excipulum; ○, species with medium-sized to large ascospores (> 50 μm); +, species with inspersed hymenium (+ = type A, ++ = type B).

Fig. 12. Second partition of shortest tree of 167 Graphis species plus outgroup Diorygma minisporum (48 characters). For details and explanation of symbols see Fig. 11.
Several paraphyletic grades and monophyletic clades can be distinguished which largely correspond to lirellae types or lirellae morphs. When using Diorygma minisporum as outgroup, the first, basal partition of the tree is composed of a paraphyletic grade (scripta grade) that includes all species with (immersed to) erumpent lirellae and lateral (to complete) thalline margins (Fig. 11). Species with glaucescens morph lirellae (and thallus) fall at the base of the tree (glaucescens aggregate), which is consistent with their close similarity to Diorygma species. Species with a corticate thallus and mostly erumpent lirellae with lateral thalline margins (lineola, deserpens, centrifuga, caesiella, tenella, and dichotoma morphs) fill the backbone of the basal partition, but the individual morphs do not form clear monophyletic entities, except for a small caesiella and a small centrifuga aggregate and a more or less clean, paraphyletic grade with lineola morph lirellae. Several species with an inspersed hymenium (type A) cluster together in a small lineola aggregate (disc concealed) and an aperiens aggregate (disc exposed). Notably, morphs with striate lirellae (tenella and dichotoma) are dispersed and do not cluster together.
Nested within this large grade are three further groups (Fig. 11): the consanguinea aggregate (lirellae immersed-erumpent with apically thick complete thalline margins), the symplecta aggregate (lirellae immersed-erumpent with apically thin complete thalline margins), and the subserpentina aggregate (lirellae immersed-erumpent with laterally thick to complete thalline margins). The first two are very similar morphologically (separated chiefly by the apically thick versus thin complete margin) but the consanguinea aggregate is clearly separated by a long, weakly supported branch. The subserpentina aggregate differs from species with deserpens morph lirellae chiefly in the thick thalline margin and tendency to produce large ascospores. When using Fissurina dumastii as outgroup (not shown), the consanguinea and symplecta aggregates form two monophyletic clades at the base of the tree, whereas the remaining species of this partition form a large, monophyletic clade, with the subserpentina aggregate being sister to a clade including most of the other species (scripta clade). It therefore appears that the consanguinea, symplecta and subserpentina aggregates are distinctive within this partition and their nested or separate placement depends on outgroup selection.
Most of the species in this basal scripta grade have small ascospores, but large-spored species are found in the consanguinea, symplecta, and subserpentina aggregates (Fig. 11). Most species have a laterally (or apically) carbonized excipulum, and species with a completely carbonized excipulum are dispersed within this grade and do not show any tendency to cluster together. Except for the lineola-aperiens aggregates, species with inspersed hymenium are scattered over the cladogram.
Independent of whether using Diorygma minisporum or Fissurina dumastii as outgroup, the second, terminal partition of the cladogram is identical in both cases and composed of two large clades (Fig. 12). The first clade (striatula clade) includes species with hossei and striatula morph lirellae, i.e. erumpent to prominent lirellae lacking or with basal thalline margin, being either entire (hossei morph) or striate (striatula morph); both morphs intermingle. Nested within this clade are three distinctive aggregates: the chrysocarpa aggregate, with pigmented epithecium and large to very large ascospores, the chromothecia aggregate, with pigmented lirellae, exposed discs, and small ascospores, and the nuda aggregate, with very short, unbranched, prominent to sessile, entire lirellae (nuda-morph).
The second clade includes two clades (Fig. 12). The first clade is formed chiefly by the farinulenta and the marginata aggregate. Both have prominent lirellae with thick lateral thalline margins, with pruinose labia and ecorticate thalli in the farinulenta aggregate and with non-pruinose, sharply delimited labia and corticate thalli in the marginata aggregate. The second clade (acharii clade) includes six main lirellae morphs: the rhizocola and acharii morphs, with prominent lirellae with apically thin complete thalline margins and entire (rhizocola) or striate (acharii) lirellae; the illinata morph, with prominent lirellae with apically thick complete thalline margins and entire lirellae, the cleistomma and globosa morphs, with very short to rounded lirellae, and the dussii morph, with prominent to sessile, very short and unbranched lirellae with thick lateral thalline margins. Species representing the first three morphs more or less intermingle, whereas the last three morphs cluster together in a monophyletic clade, more or less separating the dussii from the cleistomma / globosa morphs (Fig. 12).
Most species in this second partition of the tree have a completely carbonized excipulum and produce medium-sized to large ascospores, but there is no clear tendency for species to cluster together based on excipulum carbonization and/or ascospore size, except for those already held together by lirellae morphs (Fig. 12). Species with an inspersed hymenium are scattered over the cladogram and do not cluster together.
Overall, the tree topology shows a dominant structure based on lirella type, i.e. emergence and thalline margin and, to a lesser degree, lirella shape, i.e. relative length and branching. Excipulum carbonization and striation do not have a phylogenetic signal except when correlated with lirella type. Ascospore size exhibits certain tendencies to cluster species with otherwise similar lirellae, but this is only apparent in the subserpentina aggregate as compared to species with lineola or deserpens morph lirellae. Several other aggregates show clusters of closely related species ranging from small to large ascospores, such as the symplecta, nuda, and marginata aggregates.
Species ordination
Ordination of species by means of non-metric multidimensional scaling (NMS) using Euclidean distance measure is largely consistent with the cladistic analysis (Fig. 13). Species of the scripta grade cluster near the lower left centre of the diagram, with species of the subserpentina aggregate largely separated along the first axis towards the right and those of the glaucescens aggregate along the second axis towards the lower part of the diagram. The striatula clade is recovered in the upper left part of the diagram, with the chrysocarpa, chromothecia, and nuda aggregates in close proximity. The acharii clade is located in the lower right part of the diagram, with the globosa aggregate to the right. Four species with illinata morph lirellae cluster separately (illinata aggregate). The symplecta and the consanguinea aggregates are well separated from each other, supporting their recognition as distinct entities, and the former appears intermediate between the scripta grade and the acharii clade. The dussii aggregate, which is nested within the acharii clade in the cladistic analysis, takes a more isolated position in the species ordination, with no clear closest relative.
Using the cladistic analysis and the species ordination, some taxa with unique lirella morphs (‘unassigned’) can be related to larger clades and aggregates. Graphis granulocarpa strongly resembles a species of the G. nuda group, but differs in its apically carbonized excipulum and striate labia; the species is resolved as sister to the nuda aggregate (Figs 11 & 13). Graphis oryzaecarpa agrees with species of the dussii aggregate in lirella shape and anatomy, but has a unique white cover on top of the labia; still it clusters with the dussii aggregate (Figs 12 & 13). The position of G. asterizans agrees with its similarity with species of the farinulenta and marginata aggregate, from which it differs by its striate labia (Figs 12 & 13). Graphis lumbricina is a unique species which has a thin thalline margin usually flaking off and exposing the jet-black, strongly striate labia; it is most similar to species of the acharii clade and falls within that clade in the analysis (Figs 12 & 13). The similar G. elegans is a member of the striatula clade. Graphis hyphosa is also a unique species with pseudostromatic lirellae, but its other characters place it within the scripta grade, a view supported by the cladistic analysis and the species ordination (Figs 11 & 13).
Species groups in the genus Graphis
Based on the cladistic analysis and the species ordination, the following groups can be tentatively distinguished within the genus Graphis.
Graphis scripta group
Lirellae typically immersed to erumpent and with lateral thalline margins; labia mostly entire (lineola morph) but in some species striate (tenella morph); excipulum (apically to) laterally (to completely) carbonized; labia often pruinose (caesiella morph); disc sometimes exposed (handelii morph) or exposed and pruinose (scripta morph); hymenium often inspersed (type A); ascospores small to medium-sized; many species with secondary substances. The group, which largely corresponds to ‘Eugraphis’, contains c. 90 species. Nested within this group are:
centrifuga aggregate—lirellae very long and radiately branched; labia entire, non-pruinose;
lineola aggregate—labia entire, non-pruinose; hymenium inspersed;
caesiella aggregate—labia pruinose;
aperiens aggregate—disc exposed; hymenium inspersed;
glaucescens aggregate—thallus ecorticate; labia mostly striate, pruinose.
Graphis subserpentina group
Lirellae typically immersed to erumpent and with lateral (to complete) thalline margins; labia mostly entire; excipulum (apically to) laterally (to completely) carbonized; hymenium often inspersed (type A); ascospores medium-sized to large, if muriform often with reduced endospore; many species with secondary substances. The group contains c. 20 species. This group is close to the scripta group and chiefly separated by the robust lirellae and usually large ascospores, but it might well be nested within the latter.
Graphis dussii group
Lirellae typically erumpent to prominent and with thick lateral thalline margins, very short and unbranched; labia entire; excipulum laterally to completely carbonized; ascospores small to large; many species with secondary substances. The group contains c. 10 species. Contrary to the cladistic analysis, this group is here kept separate from the acharii group, since it takes a more isolated position in the species ordination and agrees in many tendencies (thalline margin, chemistry) more with the scripta and subserpentina groups.
Graphis marginata group
Lirellae prominent and with thick lateral thalline margins; labia mostly entire; excipulum (laterally to) completely carbonized; labia either jet-black and sharply delimited from the thalline margin or pruinose (then thallus ecorticate); ascospores small to large; many species with secondary substances. The group contains c. 15 species. Nested within this group are:
Graphis farinulenta aggregate—labia pruinose; ascospores small to medium-sized; few species with secondary substances; thallus usually ecorticate.
Graphis marginata aggregate—labia jet-black, sharply delimited from the thalline margin; ascospores small to large; many species with secondary substances; thallus corticate.
Graphis symplecta group
Lirellae typically immersed to erumpent and with complete (thin to thick) thalline margin; labia striate; excipulum apically to completely carbonized; ascospores small to large; few species with secondary substances. The group contains c. 15 species. Similar to species of the acharii group and chiefly differing by the immersed to erumpent lirellae. Nested within this group are:
Graphis symplecta aggregate—lirellae with apically thin complete margin.
Graphis consanguinea aggregate—lirellae with apically thick complete margin.
Graphis acharii group
Lirellae typically prominent to sessile and with (lateral to) complete thalline margins, sometimes very short (cleistomma morph) to round (globosa morph); labia entire (illinata and rhizocola morphs) or striate (acharii morphs); excipulum (apically to) laterally to completely carbonized; hymenium often inspersed (type B); ascospores medium-sized to large; few species with secondary substances. The group contains c. 60 species.
Graphis striatula group
Lirellae typically (erumpent to) prominent (to sessile) and lacking a distinct thalline margin (sometimes basally present); labia mostly striate (striatula morph), more rarely entire (hossei morph); excipulum apically to completely carbonized, in species with laterally carbonized excipulum often basally converging; ascospores small to large; few species with secondary substances but anthraquinones common. The group contains c. 60 species. Nested within this group are:
chromothecia aggregate—labia entire, with pigmented epithecium; ascospores small.
chrysocarpa aggregate—labia entire to striate, with pigmented pruina; ascospores large.
Graphis nuda group
Lirellae (prominent to) sessile and lacking a distinct thalline margin, very short and unbranched (Melaspilea-like); labia mostly entire (nuda morph), rarely striate (granulocarpa morph); excipulum completely carbonized; ascospores small to large; very few species with secondary substances. The group contains c. 10 species. This group may be nested within the striatula group but is kept separate due to its distinctive features.
About 280 of the 320 species of Graphis currently accepted can be assigned to one of the above groups; the remaining species are either intermediate or represent further, distinctive but small groups whose relationships with the above groups need to be studied further.
The concept of ‘morphs’ in the genus Graphis
Species of Graphis that share the same morphology but differ in anatomical or chemical characters can be described as ‘morphs’, emending the term ‘sporomorph’ coined by Wirth & Hale Reference Wirth and Hale(1978). Principally five types of morphs can be distinguished.
Labiomorphs
Species that agree in all characters (thallus and lirella morphology, excipulum carbonization, hymenium inspersion, ascospore septation, size and number, and secondary chemistry) but differ in having entire versus striate labia; such morphs are considered as developmental stages of the same species.
Excipulomorphs
Species that agree in all characters (thallus and lirella morphology, labium striation, hymenium inspersion, ascospore septation, size and number, and secondary chemistry) but differ in the degree of excipulum carbonization.
Inspersomorphs
Species that agree in all characters (thallus and lirella morphology, labium striation, excipulum carbonization, ascospore septation, size and number, and secondary chemistry) but differ in the absence or presence of hymenium inspersion.
Sporomorphs
Species that agree in all characters (thallus and lirella morphology, labium striation, excipulum carbonization, hymenium inspersion, and secondary chemistry) but differ in ascospore septation, size, and number.
Chemomorphs
Species that agree in all characters (thallus and lirella morphology, labium striation, excipulum carbonization, ascospore septation, size and number, and hymenium inspersion) but differ in their secondary chemistry.
An analysis of the 313 species recognized here revealed different patterns regarding the proportions among the five types of morphs. Since about 35% of the species exhibit striate labia, one would expect about the same proportion of labiomorphs if labium striation would be an entirely ontogenetic feature. Yet, occurrences of labiomorphs are comparatively rare, with only 16% of the species having such morphs. If all other features are exactly the same, these were considered as belonging to the same species, for example, Graphis argentata, G. chrysocarpa and G. bulacana (entire) as synonyms of G. glaucescens (striate), G. subcinerea as a synonym of G. phaeospora, and G. subserpens as a synonym of G. cinerea (see Lücking et al. Reference Lücking, Archer and Aptroot2009, this volume).
Excipulomorphs are found in little more than one fifth of the species. Examples include Graphis crebra (lateral) versus G. aperiens (complete), G. duplicata (lateral) versus G. dupaxana (complete), G. arecae (apical) versus G. imersella (lateral) versus G. descissa (complete), G. subtenella (apical) versus G. tenella (lateral) versus G. persulcata (complete), or G. trichospora (apical) versus G. verminosa (lateral) versus G. leptospora (complete). The proportion of excipulomorphs is much lower than one would expect if this where an infraspecifically variable feature. However, in about 5% of the species, the distinction between an apically and laterally or laterally and completely carbonized excipulum is not clearcut and further studies might reveal that taxa currently separated are actually conspecific.
About 15% of the taxa have an inspersed hymenium and 13% of the species have inspersomorphs, which suggests that almost all of these have an inspersomorph with a clear hymenium. However, many species with an inspersed hymenium do not have a counterpart with a clear hymenium, supporting the notion that hymenium inspersion is a species-specific feature. Inspersomorphs are found in the Graphis acharii group, for example, G. acharii (clear) versus G. argentata (inspersed), in the G. striatula group, for example, G. duplicata (clear) versus G. duplicatoinspersa (inspersed), and in the G. scripta group, for example, G. cincta versus G. librata.
Sporomorphs are extremely common and are found in almost half of the species studied here. The best example is the aforementioned G. nuda group, but also the G. cinerea aggregate, including species with acharii type lirellae and type B hymenium inspersion.
One third of the species have at least one chemomorph, but often more than one. A good example is the G. caesiella aggregate, consisting of at least four species with similar morphology but different chemistry: G. bakeri (salazinic), G. caesiella (norstictic), G. dendrogramma (stictic), and G. supracola (protocetraric). Another example is the series G. striatula (nil), G. haleana (lichexanthone), and G. hypocrellina (isohypocrelline).
Several species, especially in the Graphis scripta group, have an exposed disc. To assess the taxonomic value of this character, instances of ‘discomorphs’ have been considered. Of 12 species with exposed discs, only five have a potential discomorph with concealed disc (Table 9). The low number of species with exposed disc also implies that the bulk of species with concealed disc to not have a discomorph with exposed disc. This pattern strongly supports disc exposure as a species-specific feature.
Table 9. Species of Graphis with exposed discs and their potential ‘discomorphs’ with concealed discs

Discussion
With the redefinition of Graphis and other genera in the Graphidaceae (Staiger & Kalb Reference Staiger and Kalb1999; Kalb & Staiger Reference Kalb and Staiger2000; Staiger Reference Staiger2002; Kalb et al. Reference Kalb, Staiger and Elix2004; Archer Reference Archer2006; Staiger et al. Reference Staiger, Kalb and Grube2006; Lücking & Rivas Plata Reference Lücking and Rivas Plata2008), the characters used to delimit species have changed considerably compared to previous treatments. Until most recently, Graphis comprised species with hyaline, transversely septate ascospores, including many taxa now assigned to Carbacanthographis, Diorygma, Dyplolabia, Fissurina, Hemithecium, and Thalloloma (Staiger Reference Staiger2002; Kalb et al. Reference Kalb, Staiger and Elix2004). Taxonomic treatments of Graphis sensu Müller Argoviensis and Zahlbruckner (Wirth & Hale Reference Wirth and Hale1963, Reference Wirth and Hale1978; Nakanishi Reference Nakanishi1966; Patwardhan & Kulkarni Reference Patwardhan and Kulkarni1976, Reference Patwardhan and Kulkarni1977, Reference Patwardhan and Kulkarni1979a–Reference Patwardhan and Kulkarnic; Patwardhan & Nagarkar Reference Patwardhan and Nagarkar1979; Archer Reference Archer1999, Reference Archer2000, Reference Archer2001a–Reference Archere; Nakanishi et al. Reference Nakanishi, Kashiwadani and Moon2003a) thus included gross morphological characters that are now used to separate genera, whereas subtle morpho-anatomical characters were not usually considered. Species were rarely compared across different ascospore genera. The habit of comparing taxa with similar chemistry, particularly in previously separated Thelotremataceae (Wirth & Hale Reference Wirth and Hale1963, Reference Wirth and Hale1978; Hale Reference Hale1974, Reference Hale1978, Reference Hale1981), rather than those with similar morphology and anatomy, also obscured the importance of morpho-anatomical characters for species-level taxonomy. The present study is the first attempt to critically assess the taxonomic value of phenotypic characters (morphology, anatomy, chemistry) found in the genus Graphis. The statistical and cladistic analyses show that most characters are useful for delimiting species and even larger, potentially natural, groups.
One of the most important findings of this study is the taxonomic importance of lirella type, that is, its emergence and thalline margin. Few previous authors have paid attention to this character complex, and often species were considered as morphologically variable (e.g., Wirth & Hale Reference Wirth and Hale1963, Reference Wirth and Hale1978). Among all characters, lirellae type showed the strongest non-functional correlations with characters such as excipulum carbonization, hymenium inspersion, ascospores, and chemistry. Non-functional implies that correlations appear to be due to common ancestry, a strong argument supporting their taxonomic value at species and species group level. This notion is supported by the phylogeny-independent homoplasy analysis. Both the cladistic analysis and the species ordination resolved several species groups held together by lirella types. This may come as a surprise, since morphological characters are usually considered highly homoplastic, but molecular phylogenetic studies in Graphidaceae as a whole (including Thelotremataceae), as well as other groups, demonstrate that natural species groups are characterized by a rather uniform morphology of thallus and apothecia (Staiger et al. Reference Staiger, Kalb and Grube2006; Mangold et al. Reference Mangold, Martín, Lücking and Lumbsch2008). In a recent molecular study of species delimitation in the genus Thelotrema, Lumbsch et al. (Reference Lumbsch, Mangold, Martín and Elix2008a) found that closely related species share the same thallus and apothecial morphotype but differ in ascospores and/or secondary chemistry, for example, in the Thelotrema monosporum and the T. lepadinum complex, a strong support for the recognition of ‘sporomorphs’ and ‘chemomorphs’ as different species. Thus, contrary to previous views, specimens that do agree in anatomical, ascospore and chemical characters, but not in morphology, not only do not represent the same species, but may not even be closely related. Of course, molecular studies in Graphis are required to test these results further.
Excipulum striation has been much discussed regarding its taxonomic value. In earlier works, forms with striate labia were invariable considered distinct species, a concept that was continued until recently. Staiger Reference Staiger(2002) discussed the functional importance of labium striation and concluded that in most cases, striation is due to the repeated formation of new hymenia and excipula within the same lirella, pushing aside old hymenia and excipula and thus creating an often strongly layered lateral excipulum. This can be observed very clearly in species such as Graphis elegans and G. lumbricina, but is probably not the case in all taxa, since in some the striation is shallow and restricted to the uppermost part of the otherwise rather thick and continuous lateral excipulum. From these considerations it follows that excipulum striation is primarily an ontogenetic feature and cannot be used as a taxonomic character per se; that is, if there is no other discriminating character, forms with entire and striate labia are to be considered as representing different ontogenetic stages of the same species, those with entire labia representing the first generation lirellae. This can readily be observed in species such as G. chrysocarpa. However, this does not mean that labium striation is of no taxonomic importance. On the contrary, the strong non-functional correlations found with several other characters suggest that labium striation behaves differently in different species groups; while it is absent or rare in some groups (e.g., the G. nuda, G. scripta, and G. subserpentina groups), it is the rule in others, such as the G. striatula and the G. acharii groups. Apparently, in certain groups no visible hymenial regeneration occurs, whereas in others, labia become striate almost from the beginning of their development.
The taxonomic value of the presence of a pruina is controversially discussed in the literature, and its function is unknown. In many cases, especially when produced on the thallus surface, it correlates with other characters and characterizes taxa at different levels from species to genus. In some instances, a pruina formed on the apothecial surface is the only character separating species, and then its taxonomic value is doubtful; however, it is usually accepted to separate species if the difference between pruinose and non-pruinose forms is distinctive, such as in the case of the foliicolous lichens Calopadia foliicola versus C. phyllogena or Tapellaria nana versus T. epiphylla (Lücking Reference Lücking2008). In Graphis, a pruina can be formed either on the upper part of the labium or on the disc surface in species which have the disc exposed. In many cases, the presence of a pruina correlates with the presence of secondary substances; examples are G. caesiella (norstictic acid) and G. dendrogramma (stictic acid), which are both characterized by a thick pruina on the upper surface of the labia. However, there are also taxa with thick labial pruina that lack lichen substances, such as G. glaucescens. Taxa with pruinose labia or disc only rarely have a ‘pruinomorph’, that is a species that agrees in all characters except lacking a pruina. Examples are G. dendrogramma (labia pruinose) versus G. immersella (labia non-pruinose), G. seminuda (labia pruinose) versus G. flavens (labia non-pruinose), and G. crebra (disc pruinose) versus G. handelii (disc non-pruinose). The only common extratropical species, G. scripta, is circumscribed as mostly having but sometimes lacking a pruina, and so far it is the only case where the presence or absence of a pruina is considered an infraspecific variation. Obviously, in particular cases the taxonomic value of a pruina has to be assessed with great care, but it might also be worth while to examine the case of G. scripta, whether or not more than one taxon is involved here.
Excipulum carbonization is another character that has been emphasized with respect to its value for species and species group delimitation (Wirth & Hale Reference Wirth and Hale1963; Reference Wirth and Hale1978; Staiger Reference Staiger2002). Wirth & Hale Reference Wirth and Hale(1978) separated species with a laterally carbonized and basally open excipulum as ‘Eugraphis’ from the rest of the genus. However, the present study shows that this distinction holds only partially; while certain species groups are characterized by a tendency to have either a laterally (G. subserpentina and G. scripta groups) or completely carbonized excipulum (G. nuda and G. acharii groups), almost all groups feature the entire range of variation, with apparently closely related species separated only by apically, laterally, or completely carbonized excipula. This variation is particularly obvious in species of the G. duplicata and G. subserpentina groups. It follows that excipulum carbonization, while useful to delimit species, cannot be used to characterize species groups as a whole. Even at the species level, this character is sometimes difficult to apply, as in some species (particularly in the G. duplicata group), the basal excipulum is often thin in the centre but the lateral, carbonized excipulum converges basally. Further studies are required here to assess infraspecific variation of this character. The rather thick basal excipula in some species suggest a similar mechanism as found in the genera Leiorreuma, Sarcographa, and Thecaria (Staiger Reference Staiger2002), in which the carbonized ‘hypothecium’ gradually becomes thicker with age. Since there is no clearcut distinction between the basal ‘hypothecium’ and excipulum, this mechanism may cause certain Graphis species (especially in the G. duplicata group) with initially laterally carbonized excipulum to eventually develop a basally closed, rather thick and completely carbonized excipulum with age.
Hymenium inspersion characterizes a number of genera within the lirellate Graphidaceae (Staiger Reference Staiger2002; Lücking & Rivas Plata Reference Lücking and Rivas Plata2008). Members of the Phaeographis clade (with grey-brown ascospores; see Staiger et al. Reference Staiger, Kalb and Grube2006; Mangold et al. Reference Mangold, Martín, Lücking and Lumbsch2008) usually have a strongly inspersed hymenium (Leiorreuma, Pallidogramme, Phaeographis, Platygramme, Sarcographa, Thecaria, Thecographa), with the exception of the Phaeographis intricans group. The remaining genera usually have a non-inspersed hymenium, except Anomomorpha (all species inspersed) and a few species in other genera. Until most recently (Staiger Reference Staiger2002), hymenium inspersion has rarely been used as a taxonomic character in Graphis. This is surprising since it is obviously a constant character in many other genera and does not disappear with age. Strictly speaking, hymenium inspersion is a chemical character, and secondary chemistry has been extensively applied to delimit species in Graphidaceae (including Thelotremataceae), especially by Hale and co-workers (Wirth & Hale Reference Wirth and Hale1963, Reference Wirth and Hale1978; Hale Reference Hale1974, Reference Hale1978, Reference Hale1981). In the present study, a distinct correlation was found between the occurrence of hymenium inspersion and other characters that denote groups of species. Also, there are two different types of inspersion restricted to different species groups. Inspersion of type A occurs frequently in the G. scripta and G. subserpentina groups, which mainly differ in the size of their ascospores but otherwise are held together by their usually immersed-erumpent lirellae with lateral to complete (thick) thalline margins, usually entire labia, a variably but often laterally carbonized excipulum, and the frequent occurrence of secondary substances. Inspersion of type B is practically restricted to the G. acharii group, which includes species with more or less prominent lirellae with complete (thin) thalline margins, a tendency to form striate labia and a completely carbonized excipulum, and frequent absence of secondary substances. In both cases, there are species that are distinguished only by the absence or presence of hymenium inspersion (‘inspersomorphs’). These are kept separate since the results of this study do not suggest that inspersion is a character of infraspecific variation; otherwise it would be found more frequently with no apparent taxonomic contraints.
Staiger Reference Staiger(2002) mentions paraphyses as a character to distinguish two groups in Graphis: the G. carassensis group (here called G. illinata aggregate), with rather thin paraphyses, and the remaining species, with thicker, more conglutinate paraphyses. The present study could not confirm these differences, although a tendency towards thinner paraphyses is seen in species with large ascospores. However, such species are found in several different groups, and also the molecular data available so far do not support this character as of systematic importance. A good example is the G. nuda group, in which a continuous transition from species with small ascospores and thicker paraphyses (G. nuda, G. virescens) to the ones with large ascospores and thin paraphyses (G. ruiziana, G. subruiziana) can be observed.
Ascospores are confirmed as one of the most important character complexes to distinguish closely related species. Increasing evidence from a wide range of lichenized and non-lichenized Ascomycota shows that series of closely related species often differ only in the number, size, and septation of their ascospores (Staiger Reference Staiger2002; Herrera-Campos et al. Reference Herrera-Campos, Huhndorf and Lücking2005; Frisch et al. Reference Frisch, Kalb and Grube2006; Lumbsch et al. Reference Lumbsch, Mangold, Martín and Elix2008a; Lücking Reference Lücking2008). In Graphidaceae, this was already recognized by Wirth & Hale Reference Wirth and Hale(1978) and they coined the term ‘sporomorphs’ for such species. However, this phenomenon is commonly found in unrelated families such as Lecanoraceae and Pilocarpaceae (Lecanorales), Physciaceae and Teloschistaceae (Teloschistales), Asterothyriaceae and Gomphillaceae (Ostropales), Pyrenulaceae (Pyrenulales), Verrucariaceae (Verrucariales), Pyrenotrichaceae (Chaetothyriales) and many others. An outstanding example in the genus Graphis is again the G. nuda group, in which a continuous transition from small to large and transversely septate to muriform ascospores is observed. The discussion in such cases is obviously not whether sporomorphs represent different species (the hardly acceptable alternative would be to unite all ascospore types into one taxon), but where to draw the limits between one species and another, especially if there is a large range in variation. The overall distribution of ascospore size frequencies suggests that there are three ‘preferred’ sizes: small (20–50 μm), medium-sized (50–100 μm), and large (100–200 μm). Indeed, most species delimited otherwise by morpho-anatomical and chemical features, such as G. chrysocarpa, G. discarpa, G. firferi, G. inversa, G. lumbricina, G. mexicana and G. seminuda, show that their ascospores fall within the range of variation delimited by these three size classes, and exceptions are comparatively rare. Also, the different species groups show a certain tendency to produce either small to medium-sized or medium-sized to large ascospores, while closely related species with either small or large ascospores are rare. The problem in using ascospore size to delimit species is observational rather than conceptual; in many cases, reported large size ranges are due to the fact that immature ascospores are included in the variation, which is obviously incorrect. Immature ascospores are usually easily discerned by the lack of endospore development and the amorphous nature of their cell contents and must be excluded from ascospore measurements.
The revised definition of Graphis as a genus containing both transversely septate and muriform ascospores recognizes that the historical concept of ascospore genera does not hold. Indeed, it is frequently found that closely related species differ by transversely septate versus muriform ascospores, particularly in the G. acharii and G. nuda groups. Submuriform ascospores, in which only part of the segments have longitudinal septa, are very rare in the genus. On the other hand, quite a number of species feature terminally muriform (‘biocellate’) ascospores. Following Staiger Reference Staiger(2002), this is accepted here as a species-specific feature, since it cannot be explained by the ontogenetic transformation of initially unicellular into regularly muriform ascospores. Also here, the problem is more observational, since ascospores that remain in the ascus are usually compacted towards the tips and then the terminally muriform septation is difficult to observe and the specimens are erroneously believed to have transversely septate ascospores.
A character that needs to be studied in more detail is the nature of the external ascospore wall in Graphis species. Conspicuously thick-walled ascospores are known from Graphis elegans which, at some point, was even separated in its own genus, Aulacographa. However, similar ascospore walls have also been found in other species, such as G. adpressa (Lücking et al. Reference Lücking, Chaves, Sipman, Umaña and Aptroot2008a). Their KOH reaction suggests that these thick walls are almost comparable to the halo found in species of other genera (e.g., Fissurina), although they are slightly different in nature, being constricted at the septa in G. elegans. Further studies are needed to assess the nature of this character and its variation but it is certainly not sufficient to warrant generic status. This is also supported by the great similarity between G. elegans and G. lumbricina, the latter being morphologically identical but having large ascospores with rather normal walls. Another character rarely found in Graphis is pigmented ascospores. In some species with usually hyaline ascospores, such as G. chrysocarpa and G. phaeospora, old ascospores may become greyish-brown, but they are always mixed with mature, hyaline ascospores, and hence no specific differentiation is drawn in these cases. In two cases, however, G. mucronata and G. pittieri, the material available shows uniformly grey-brown ascospores which look mature and healthy. They are not completely out of line with other Graphis species, since similar variation is known from other genera (e.g., Platythecium; Staiger Reference Staiger2002) and is even the rule in many thelotremoid genera (Frisch et al. Reference Frisch, Kalb and Grube2006). In all other characters, the two species agree completely with the bulk of Graphis species.
Contrary to the thelotremoid Graphidaceae, especially Ocellularia and allies, the lirellate Graphidaceae are comparatively poor in lichen substances. If substances are present, they represent depsidones (norstictic, stictic, salazinic, protocetraric and psoromic acids and relatives), rarely other substance classes such as depsides (lecanoric acid), xanthones (lichexanthone), and anthraquinones (tetrahydroanthraquinone, isohypocrelline). Several genera exhibit more or less the entire variation of secondary substances (Acanthothecis, Carbacanthographis, Diorygma, Graphis, Phaeographis, Platythecium); others are characterized by a relatively uniform or slightly varying (Anomomorpha, Fissurina, Hemithecium, Leiorreuma, Platygramme, Sarcographa, Thalloloma, Thecaria) or unique chemistry (Dyplolabia), or lichen substances are completely absent (Glyphis). Different approaches have been used in the Graphidaceae and the now included Thelotremataceae with regard to the importance of chemistry for taxonomic purposes. While Hale and co-workers (Wirth & Hale Reference Wirth and Hale1963, Reference Wirth and Hale1978; Hale Reference Hale1974, Reference Hale1978, Reference Hale1981) often considered species with identical chemistry, rather than similar morphology, as closely related, assuming a certain level of systematic importance for chemical features, Harris (Reference Harris1990, Reference Harris1995) united chemically different forms as chemotypes into a single species, such as in the case of G. caesiella and its allies. Both approaches do not necessarily reflect natural groups and the usefulness of secondary chemistry for taxonomic and systematic purposes is still controversially discussed (Feuerer & Hawksworth 2007).
Hawksworth Reference Hawksworth, Brown, Hawksworth and Bailey(1976) suggested that if morphological, ecological or distributional differences can be correlated with the chemical variants, these should be recognized at the species level. However, in cases where one substance is replaced by one or more closely related substances and there are no morphological, ecological or geographical patterns correlated with chemistry, he suggests recognizing the chemical variants as the same taxon. Lumbsch Reference Lumbsch(1998) proposed recognizing chemical variants as separate species only when morphological differences can be correlated with chemistry. However, it is inconceivable that chemical characters are, a priori, treated differently than other morphological or anatomical features. There is no evidence that secondary chemistry should be conceptually, more or less variable within a species or taxonomic group than ascospore features; yet, the use of ascospore features to separate otherwise identical species is widely accepted. If chemical differences need support by other data to qualify for taxonomic recognition, then why would one use other data, such as differences in ascospore septa, without the support of chemical differences? All these characters are phenotypic in nature and undergo the same phenomena of infraspecific allelic variation or ontogenetic or environmental modification. It should also be noted that certain characters that are chemical in nature, such as ascospore pigmentation, excipulum carbonization, hymenium inspersion, or the presence of pigments, are much more readily accepted as species-specific characters than ‘invisible’ secondary substances.
Molecular studies testing the usefulness of secondary chemistry for taxonomic purposes at the species level are rare but in most cases indicate that chemical differences are species- or lineage-specific. For cortical substances, this has been shown for the pair Parmeliopsis ambigua and P. hyperopta(Tehler & Källersjö Reference Tehler and Källersjö2001). Regarding medullary substances, McDonald et al. Reference McDonald, Miądlikowska and Lutzoni(2003) showed that Sticta ‘weigelii’ s. lat. in the Rocky Mountains represents several different species well-distinguished by their morphology and/or chemistry. Even with variable sustances such as terpenoids in the genus Peltigera, certain specific patterns are detectable (Miądlikowska & Lutzoni Reference Miądlikowska and Lutzoni2000). Stocker-Wörgötter et al. Reference Stocker-Wörgötter, Elix and Grube(2004) showed strong correlation between chemotypes and phylogenetic lineages in the Ramalina farinacea complex. In species of the Heterodermia obscurata group, Lücking et al. (Reference Lücking, Del Prado, Lumbsch, Will-Wolf, Aptroot, Sipman, Umaña and Chaves2008b) found that medullary chemistry and pigment formation correlated well with phylogenetic lineages. Haematomma accolens and H. flexuosum differ chemically in two minor accessory substances but these differences correlate with ITS sequence data (Lumbsch et al. Reference Lumbsch, Nelsen and Lücking2008b). Perhaps the most interesting case is that of Cladonia mitis, which was found to be monophyletic, but nested within the chemically deviating C. arbuscula (Myllys et al. Reference Myllys, Stenroos, Thell and Ahti2003), a convincing example for what has been shown to exist in nature: paraphyletic species (Crisp & Chandler Reference Crisp and Chandler1996; Wiley & Mayden Reference Wiley, Mayden, Wheeler and Meier2000; Funk & Omland Reference Funk and Omland2003; Zander Reference Zander2007). Thus, even from these few studies there appears to be support to separate at least certain chemical variants at the species level.
In the absence of molecular evidence in Graphis, a strict concept is followed here to separate species even if the only difference is secondary chemistry, although infraspecific variation in the occurrence of accessory substances is accepted. This concept is supported by several findings. In the present study, certain correlation patterns were found showing that chemical variation is not equal across the genus: the occurrence of substances is concentrated among the G. scripta and G. subserpentina groups, whereas the G. acharii, G. striatula and G. nuda groups mostly lack substances. This makes infraspecific variation as response to environmental conditions unlikely. In addition, species of Graphis otherwise well-characterized by morpho-anatomical features usually have a very uniform chemistry, such as G. chrysocarpa, G. dendrogramma, G. firferi, G. inversa and G. lumbricina. On the other hand, except for the concentration of secondary substances in certain species groups, there is no evidence that occurrence of a particular substance denotes any level of phylogenetic relationships. This study shows that species that produce the same lichen substance are usually not closely related; for example, the taxa that have the rare substances salazinic or protocetraric acid belong to five different species groups, and the four taxa producing lichexanthone represent three different species groups. The common substances norstictic and stictic acid are found in almost all groups.
When discussing taxonomic relationships in the Graphidaceae based on ascospore genera, Wirth & Hale Reference Wirth and Hale(1978), as other workers before them, noticed the great morphological similarity of certain species placed in different genera because of transverse versus muriform ascospores. They called such species ‘sporomorphs’, but did not draw any formal conclusions about the relationships of such species. The first to recognize that such sporomorphs (without actually using the term) represent closely related species that should be placed in the same genus was Santesson Reference Santesson(1952). For several genera, he discussed the close relationship between species with transversely septate or muriform ascospores, for example, Bacidia gabrielis and Lopadium flammeum, now united in the genus Loflammia, or Bacidia micrommata and Lopadium newtonianum, now united in the genus Eugeniella (Lücking Reference Lücking2008). The genus Tapellaria sensu Santesson was the first genus to unite species with very different ascospore septation, size and number, and, although questioned until recently (Hafellner Reference Hafellner1984), the concept of ‘sporomorphs’ as representing evolutionary series of closely related species is now a widely accepted phenomenon, particularly common in the Ostropomycetidae. While this concept was so far formally restricted to variation in ascospores, it can be easily expanded to encompass any character that is independent of the gross morphology of a given taxon. For example, many lichens occur in morphologically and anatomically identical forms with different secondary chemistry (e.g., Heterodermia japonica versus H. reagens). The findings of this study allow extension of the concept of ‘sporomorphs’ in Graphis, where one can also distinguish‘labiomorphs’ (entire versus striate), ‘excipulomorphs’ (apically versus laterally versus completely carbonized), ‘inspersomorphs’ (clear versus inspersed), and ‘chemomorphs’ (different secondary substances absent or present). In the absence of contrary evidence, such morphs should be separated at the species level, although previous treatmentshave been inconsistent with respect to this phenomenon. While labiomorphs, excipulomorphs, and sporomorphs were almost exclusively recognized as different species, the importance of inspersomorphs has only recently been realized (Staiger Reference Staiger2002). Also, as already mentioned, most authors separated chemomorphs in Graphidaceae at the species level (Wirth & Hale Reference Wirth and Hale1963, Reference Wirth and Hale1978; Archer Reference Archer1999, Reference Archer2000, Reference Archer2001a–Reference Archere, Reference Archer2003a, Reference Archerb, Reference Archer2005, Reference Archer2006), while others lumped them under a single species (Harris Reference Harris1990, Reference Harris1995). As a result of the present study, all morphs are considered different species unless contradicted by independent, molecular data, with the exception of labiomorphs, which are considered developmental stages of the same taxon (if all other characters are identical).
Staiger Reference Staiger(2002) distinguished three major groups within the genus Graphis, mainly based on excipulum structure: the G. scripta group (‘Eugraphis’), with species having entire labia and a laterally carbonized, basally open excipulum; the G. serpens group, with species having entire labia, a completely carbonized excipulum, usually thin paraphyses and often very large ascospores; and the G. acharii group, with species having striate labia often covered by thallus tissue, a completely carbonized excipulum, and usually large ascospores. Perhaps the most important finding of the present study is the strong evidence that potentially natural groups within Graphis are not only larger in number and more diverse but also that characters such as striation and excipular carbonization cannot be used to separate these groups. The most important character complex to separate groups appears to be lirella morphology, and within almost all groups, a certain variation in labium striation and excipulum carbonization can be found. Molecular data are now required to test these findings; the few available sequences of less than ten species thus far provide unsatisfactory evidence with regard to the systematics and taxonomy of Graphis (Staiger et al. Reference Staiger, Kalb and Grube2006; Mangold et al. Reference Mangold, Martín, Lücking and Lumbsch2008). The revised concept of species and species group delimitation in Graphis presented here provides ample opportunities for targeted molecular approaches to test specific hypotheses.
Conclusions
This study shows that critical analysis of phenotype data (morphology, anatomy, chemistry) in Graphis provides substantial evidence for taxonomic species delimitation and systematic classification in the genus. The characters used in this study were not different from those applied by previous workers; yet, the taxonomic and systematic conclusions based on numerical and cladistic techniques are substantially different in their overall approach to the genus. Presumably natural groups are apparently held together by lirella morphology, rather than labium striation, excipulum carbonization, or secondary chemistry. Hymenium inspersion and secondary chemistry are important characters to separate species and also show differential variation in different lineages. The morph concept can be used to characterize evolutionary series of closely related species that might reflect radiation events, such as exemplified by the G. nuda group (sporomorphs) or the G. caesiella aggregate (chemomorphs). While molecular data are required to test these findings, it is obvious that a rigorous numerical and cladistic analysis of available phenotype data in a given taxon, group, or lineage, provides a much more accurate working hypothesis than ad hoc evaluation of such characters. It is therefore recommended to perform phenotype-based statistical and cladistic analyses of any given group before designing and executing a molecular phylogenetic study, since a more robust hypothetical framework is then available that allows for a targeted molecular approach.
This study was made possible by three grants provided by the United States National Science Foundation (NSF): “TICOLICHEN” (DEB 0206125; PI Lücking); “Phylogeny and Taxonomy of Ostropalean Fungi” (DEB 0516116; PI Lumbsch, Co-PI Lücking); and “Neotropical Epiphytic Microlichens” (DEB 0715660; PI Lücking). Curators of the herbaria in B, BM, FH, G, H, M, NY, S, TUR, UPS, US, and W provided access to valuable type material and other significant collections. The author is indepted to Bettina Staiger, Klaus Kalb, Alan Archer, Harrie Sipman, André Aptroot, and Richard Harris for fruitful (and endless) discussions on the taxonomy and nomenclature of Graphidaceae. This study would not have been possible without the groundwork laid by Bettina Staiger and Klaus Kalb, Alan Archer, Minoru Nakanishi, and the late Mason Hale Jr. and his student, Michael Wirth. Richard Harris, James Lendemer and an anonymous reviewer provided valuable comments that helped to improve this manuscript considerably.