Introduction
Second only to being a classic example of symbiosis, lichenized fungi are well known as producers of unique secondary metabolites (see Rankovíc (Reference Ranković2015) and references therein). For the systematist, these compounds take on special significance as taxonomic characters because of their high congruence with morphological variation and their ease of identification, even in old museum specimens (reviews: Culberson Reference Culberson1969a; Brodo Reference Brodo1986; Rogers Reference Rogers1989). Some morphological species, however, comprise multiple, morphologically indistinguishable chemical races, or chemotypes (Culberson Reference Culberson1969a; Culberson & Culberson Reference Culberson and Culberson1970; Culberson Reference Culberson1986). Traditionally, in cases where such chemotypes have their own distinct ecologies or geographical ranges, they are interpreted as sibling species, or as subspecies (review: Lumbsch Reference Lumbsch, Frisvad, Bridge and Arora1998). Sometimes, rather than the presence or absence of individual compounds, the presence or absence of chemosyndromes (Culberson & Culberson Reference Culberson and Culberson1977) has been used to delimit species (review: Elix & Stocker-Wörgötter Reference Elix, Stocker-Wörgötter and Nash2008). In most cases, however, the interpretation of taxonomic rank based on chemotype has traditionally been assigned subjectively, without rigorous evaluation using experimental or quantitative methods.
Phylogenetic analyses of DNA sequence data have provided a more detailed picture of the evolution of chemical variation in lichenized fungi, including the polyketide synthase (PKS) genes that control production of the secondary metabolites themselves (e.g. Muggia et al. Reference Muggia, Grube and Tretiach2008). DePriest (Reference DePriest1994, Reference DePriest1995) was the first to use DNA to evaluate the taxonomic status of chemotypes: using RFLP patterns from small subunit ribosomal DNA (SSU), she demonstrated that, in the Southern Appalachians, only one of the chemotypes of the well-studied Cladonia chlorophaea chemotype complex (Cladonia grayi G. Merr. ex Sandst.) possessed unique patterns that merited recognition at the species level. More recently, analyses of nuclear ribosomal internal transcribed spacer region (ITS) sequences suggest that two other chemotypes, C. merochlorophaea Asahina and C. novochlorophaea (Sipman) Brodo & Ahti, may also be monophyletic (Dolnik et al. Reference Dolnik, Beck and Zarabska2010). In the chemically rich Ramalina americana chemotype complex, LaGreca (Reference LaGreca1999) used ITS sequences to divide the complex into two species: one, R. americana Hale, is largely acid-deficient and occurs in the northern half of the geographical range of the complex; the other, R. culbersoniorum LaGreca, encompasses five chemotypes and occupies the southern half. In another, well-studied chemotype complex in Ramalina, the R. farinacea complex, Stocker-Wörgötter et al. (Reference Stocker-Wörgötter, Elix and Grube2004) found no evidence from ITS sequences for elevating any of the chemotypes to species status. During his work on Lepraria, Lendemer (Reference Lendemer2012) discovered that a chemically variable species he described as L. normandinoides Lendemer & R. C. Harris actually comprises two sympatric sibling species, based on ITS sequence data. The original species was re-circumscribed to include the protocetraric acid and acid-deficient chemotypes, while a segregate species (called L. oxybapha Lendemer) was erected for the fumarprotocetraric chemotype. A phylogenetic investigation of the well-studied, chemically heterogeneous Parmotrema perforatum complex (Widhelm et al. Reference Widhelm, Egan, Bertoletti, Asztalos, Kraichak, Leavitt and Lumbsch2016) concluded that individuals producing an orcinol-type depsidone (alectoronic acid) comprise one phylogenetic lineage, while those producing ß-orcinol depsidones comprise two separate lineages. More recently, a molecular phylogenetic study of the Usnea cornuta complex (Gerlach et al. Reference Gerlach, Toprak, Naciri, Caviró, de Silveira and Clerc2019) found a strong correlation between nine robustly supported lineages and their chemistry; most of them were characterized by only one chemotype. Furthermore, in a ground-breaking investigation of chemical variation in lichens, Spribille et al. (Reference Spribille, Tuovinen, Resl, Vanderpool, Wolinski, Aime, Schneider, Stabentheiner, Toome-Heller and Thor2016) provided evidence that the presence of Cyphobasidium yeasts in the cortex might influence the production of secondary metabolites by demonstrating that the frequency of such yeasts is significantly different in two chemospecies of Bryoria: B. tortuosa (G. Merr.) Brodo & D. Hawksw. (with more vulpinic acid and more yeast) and B. fremontii (Tuck.) Brodo & D. Hawksw. (with less vulpinic acid and less yeast). How the occurrence of yeast on the surfaces of lichens might influence the chemistry of those lichens, however, remains obscure.
One of the most fascinating, and best-documented, examples of chemotypes exhibiting different ecologies is the Ramalina siliquosa complex. These lichens grow in dense mats on maritime, granitic cliffs in western Europe, Iceland and South-East Asia (Lynge Reference Lynge1940; Kashiwadani Reference Kashiwadani1992; Smith et al. Reference Smith, Aptroot, Coppins, Fletcher, Gilbert, James and Wolseley2009; Moon Reference Moon2013; Nimis Reference Nimis2016; Stenroos et al. Reference Stenroos, Velmala, Pykälä and Ahti2016), where they form the major component of vegetation from the high-tide zone up to where vascular plants become dominant (Fig. 1). The Ramalina siliquosa complex consists of seven morphologically similar chemotypes, all with different but broadly overlapping geographical distributions (Culberson et al. Reference Culberson, Culberson and Johnson1977; Hamada Reference Hamada1985; Kashiwadani Reference Kashiwadani1992). Chemical variation in this complex was first discovered by Nylander (Reference Nylander1870), who used spot tests to differentiate two chemotypes. Later, Zopf (Reference Zopf1906) showed that the chemotypes of this complex exhibit different ecologies: on a maritime cliff in Sweden, he observed that one chemotype (salazinic acid) grows towards the bottom of the cliff, closer to the sea, than another (protocetraric acid). Expanding on Zopf's observation, Culberson & Culberson (Reference Culberson and Culberson1967) discovered that on a maritime cliff at Holyhead, Wales, chemotypes of this complex display a bewildering zonation: one chemotype (hypoprotocetraric acid) is found only at the very top; another (stictic acid) occurs exclusively at the very bottom, nearest the water's edge; in between, an additional three chemotypes (salazinic, acid-deficient and norstictic) are arranged in distinct bands according to elevation. A similar pattern was discovered on a cliff in westernmost Portugal (Culberson Reference Culberson1969b) except that, unlike the Welsh locality, the protocetraric chemotype grows at the very top of the cliff; at Holyhead, this chemotype is found only on boulders and stone walls in more sheltered, inland localities. In fact, throughout Europe, the protocetaric and hypoprotocetraric chemotypes are the only ones that occur at substantial distances inland; the others are restricted to the coast. On the Danish island of Bornholm in the Baltic Sea (Søchting Reference Søchting1976), the pattern found was identical to that found at the Swedish locality (the zonation of which is further characterized by Culberson et al. Reference Culberson, Culberson and Johnson1977). On cliffs in north-west Spain and north-west France, Alvarez et al. (Reference Alvarez, Paz-Bermúdez and Sánchez-Biezma2001) and Parrot et al. (Reference Parrot, Jan, Baert, Guyot and Tomasi2013), respectively, reported no less than seven chemotypes but did not discuss their zonation. Of the seven known chemotypes, the one containing 4-O-demethylbarbatic acid is the rarest, its only localities being a cliff in north-west Spain (Culberson et al. Reference Culberson, Culberson and Johnson1977) and cliffs in north-west France (Parrot et al. Reference Parrot, Jan, Baert, Guyot and Tomasi2013). In all these seashore environments, biological zonation is the rule; every species of invertebrate and alga below the high-tide level has its own place in the total community (Lewis Reference Lewis1964; Moore & Seed Reference Moore and Seed1986). In other words, in Europe, the individual chemotypes of these lichens behave, ecologically, like species of other organisms on these cliffs. This compelled Culberson (Reference Culberson1986) to assert that the chemotypes of the R. siliquosa complex are closely related sympatric species, declaring them ‘the best example of sibling species marked phenotypically by natural-product chemistry’.

Fig. 1. Lichens of the Ramalina siliquosa chemotype complex covering a maritime cliff on the Isle of Skye, Inner Hebrides, Scotland, UK.
In South-East Asia, the chemistry of these lichens is not as thoroughly documented as it is in Europe, but three of the European chemotypes (salazinic, protocetraric and acid-deficient) have been verified in Japan (Culberson Reference Culberson1970; Hamada Reference Hamada1985; Kashiwadani Reference Kashiwadani1992). Unlike in Europe, zonation of chemotypes does not occur in Japan or Korea; individuals of all chemotypes appear to grow side by side (H. Kashiwadani & K. Moon, personal observation), although the concentration of salazinic acid in the salazinic chemotype has been shown to be highly correlated with temperature (Hamada Reference Hamada1981).
The morphology of the Ramalina siliquosa complex is quite variable, but not useful in a reliable way for diagnosing chemistry. In Europe, it has been shown that individuals at the bottom of cliffs (i.e. norstictic, stictic and acid-deficient chemotypes) tend to have melanized bases and pycnidia, and are often unbranched, or, when branched, the branches are primarily from the apex of the lobes; those at the top (i.e. salazinic, hypoprotocetraric and protocetraric chemotypes) tend to be non-melanized and branch primarily from the base of the thallus (Culberson Reference Culberson1967; Søchting Reference Søchting1976; Sheard Reference Sheard1978b). Interestingly, these two broad morphotypes correlate precisely with the two distinct biogenetic pathways proposed for their secondary products (Culberson et al. Reference Culberson, Culberson and Johnson1977; Sheard Reference Sheard1978a). This correlation has been used to justify a two-species taxonomy for the complex in Europe (i.e. R. cuspidata Nyl. and R. siliquosa (Huds.) A. L. Small), which has been followed in recent floras (e.g. Smith et al. Reference Smith, Aptroot, Coppins, Fletcher, Gilbert, James and Wolseley2009; Stenroos et al. Reference Stenroos, Velmala, Pykälä and Ahti2016). In South-East Asia, two morphotypes have also been noted, similar to those in Europe (Kashiwadani Reference Kashiwadani1992). Unlike in Europe, however, no correlation of morphology with chemotype, or heights on cliffs, has been observed (H. Kashiwadani & K. Moon, personal observation).
There have been two previous genetic studies of the Ramalina siliquosa complex. One study (Mattsson & Kärnefelt Reference Mattsson and Kärnefelt1986), utilizing a phenetic analysis of isozymes, showed that on the cliff in Sweden where Zopf (Reference Zopf1906) first observed the zonation of the chemotypes, three groups could be discerned. One of these groups comprised individuals of the norstictic, stictic and acid-deficient chemotypes; the other two contained individuals of the salazinic, protocetraric and acid-deficient chemotypes. A parsimony analysis of this same data set (Mattsson Reference Mattsson1990), however, indicated that each of the chemotypes yielded a different isozyme pattern, and thus should be accepted as distinct taxonomic entities. The other genetic study of the complex, by Culberson et al. (Reference Culberson, Culberson and Johnson1993), utilized chemical analyses of spore progeny from thalli collected on the cliff in Wales that was the site of their very first study of the complex (Culberson & Culberson Reference Culberson and Culberson1967). Of over 300 spores analyzed, 96% matched the maternal thallus, indicating a very high level of reproductive isolation for the chemotypes. Of the six chemotypes detected on that cliff, only the acid-deficient and norstictic chemotypes appeared to interbreed with each other.
There have been multiple phylogenetic studies of the genus Ramalina (Marsh Reference Marsh1996; LaGreca Reference LaGreca1997; LaGreca & Lumbsch Reference LaGreca and Lumbsch2001; Joneson Reference Joneson2003; Stocker-Wörgötter et al. Reference Stocker-Wörgötter, Elix and Grube2004; Sérusiaux et al. Reference Sérusiaux, van den Boom and Ertz2010; Timsina et al. Reference Timsina, Stocker-Wörgötter and Piercey-Normore2012; Pérez-Vargas & Pérez-Ortega Reference Pérez-Vargas and Pérez-Ortega2014; Gasparyan et al. Reference Gasparyan, Sipman and Lücking2017), and of species complexes within Ramalina (Groner & LaGreca Reference Groner and LaGreca1997; LaGreca Reference LaGreca1999; Ohmura et al. Reference Ohmura, Moon and Kashiwadani2008; Hayward et al. Reference Hayward, Blanchon and Lumbsch2014; Gumboski et al. Reference Gumboski, Eliasaro, Scur, Lorenz-Lemke and Borges da Silveira2018). Most of these studies utilized the ribosomal ITS region alone, although some combined ITS with another locus, such as ß-tubulin, the mitochondrial small subunit (mtSSU), or the IGS, SSU or large subunit (LSU) regions of ribosomal DNA. Each of these studies revealed one or more strongly supported, monophyletic species; however, most of the deeper, internal branches in these published phylogenies lack statistical support. To paraphrase Timsina et al. (Reference Timsina, Stocker-Wörgötter and Piercey-Normore2012), it appears that molecular data generated thus far for Ramalina are best used for species diagnosis rather than phylogenetic reconstruction.
Despite being the subject of various investigations for 150 years, the systematics of the Ramalina siliquosa complex remain poorly understood. How many species does the complex actually comprise? Do the Asian populations represent separate species? The current paper addresses these questions by reconstructing a phylogeny for the R. siliquosa complex using nucleotide sequences of four loci from 59 individuals representing six chemotypes across the geographical range of the complex. These results are discussed in the context of a preliminary phylogeny of the genus Ramalina, including 45 additional samples representing 36 taxa. The application of the ‘sibling species’ concept to these and other lichens is also explored.
Material and Methods
Taxon sampling, secondary product identification, and morphological examination
We obtained sequence data from 59 individuals of the Ramalina siliquosa chemotype complex from throughout its geographical range. These samples represent all of the major chemotypes and morphotypes and include 20 samples from South-East Asia (Supplementary Material Table S1, available online). A total of 24 additional samples representing 21 additional species of Ramalina and one species of Niebla, mostly from North America and Europe, were also sequenced. The nomenclatural authorship of all species included in our phylogenetic analyses is provided in Supplementary Material Table S1. All of the common species of Ramalina occurring in North America (Esslinger Reference Esslinger2019) were sampled. For one of these species, R. culbersoniorum, three additional specimens from portions of its geographical range not included by LaGreca (Reference LaGreca1999), including two additional chemotypes not sequenced in that study, were sequenced in order to further understand the circumscription of this chemically diverse species. In addition, 19 ribosomal DNA internal transcribed spacer region (ITS) sequences and two ribosomal intergenic spacer region (IGS) sequences from 15 Ramalina species were retrieved from GenBank to contribute to the analyses (see Supplementary Material Table S1). Niebla homalea was selected as the outgroup because previous studies (Sérusiaux et al. Reference Sérusiaux, van den Boom and Ertz2010; Miadlikowska et al. Reference Miadlikowska, Kauff, Högnabba, Oliver, Molnár, Fraker, Gaya, Hafellner, Hofstetter and Gueidan2014; Gasparyan et al. Reference Gasparyan, Sipman and Lücking2017) strongly suggest that the segregate genus Niebla is both monophyletic and closely related to Ramalina.
Secondary metabolites of all specimens were determined by TLC, using solvents A, B′ and C (Culberson & Ammann Reference Culberson and Ammann1979; Culberson & Johnson Reference Culberson and Johnson1982); spots were visualized using 10% sulphuric acid sprayed over the plates, followed by heating at 110 °C for c. 5–15 min. In addition, we closely examined the morphology of 159 Japanese and Korean specimens of the Ramalina siliquosa complex, deposited in the herbarium of the National Institute of Biological Resources, Korea (NIBR) and herbarium TNS.
DNA isolation, PCR amplification and sequencing
For specimens extracted before 2014, total genomic DNA was extracted using the DTAB/CTAB method of Armaleo & Clerc (Reference Armaleo and Clerc1995). For specimens extracted more recently, either the Prepease DNA Isolation Kit (USB, Cleveland, OH, USA; product discontinued) or the DNeasy Plant Mini Kit (Qiagen, Germany) were used, following the plant leaf extraction protocol. The ITS locus (ITS1 + 5.8S + ITS 2; c. 500 bp total) was amplified and sequenced for all samples except four (see Supplementary Material Table S1). Fragments were also amplified from the intergenic spacer region (IGS) of ribosomal DNA (c. 400 bp) and two low-copy, protein-coding markers: the largest subunit of the RNA polymerase II gene (RPB1; c. 830 bp) and the first part of the second-largest subunit of the RNA polymerase II gene (RPB2; c. 800 bp). Sequencing success rates among these three loci were more variable than for ITS (Supplementary Material Table S1). All primers used to amplify and sequence loci used in this study are given in Table 1. For the most part, PCR amplifications prior to 2014 were performed in 50 μl reactions following the method described in LaGreca (Reference LaGreca1999); more recent amplifications were conducted in 25 μl reactions using Ready-To-Go PCR Beads (GE Healthcare, Foster City, CA, USA) following the manufacturer's instructions. The PCR amplifications on the South-East Asian R. siliquosa and R. sinensis samples were performed in 20 μl volumes using AccuPower PCR tubes (Bioneer, Republic of Korea) containing 2 μl of extracted DNA solution, 1 μl each of 10 pmol/μl of each primer (Table 1), and 16 μl of deionized sterile water. PCR products were quantified on 1% agarose gels and stained with ethidium bromide or Dyne LoadingSTAR (DYNE BIO, Republic of Korea). Complementary strands were sequenced from cleaned PCR products using the same primers as for amplifications. Sequencing reactions were performed using BigDye v.3.1 or ABI PRISM 3730XL (Applied Biosystems Inc., Foster City, CA, USA) and run on an ABI automated sequencer according to recommended protocols (Applied Biosystems Inc.).
Table 1. Information for the primers used in this study, including literature references.

Sequence alignment and analysis
Contigs were assembled and edited using Sequencher v.4.10 (Gene Codes Inc., Ann Arbor, MI, USA). Two of the loci sequenced, ITS and IGS, include a number of difficult to align regions, resulting in ambiguous alignments. To address this, we tested two alignment strategies. The first was a traditional alignment using MAFFT v.7 (Katoh & Standley Reference Katoh and Standley2013). For the protein-coding loci (RPB1 and RPB2), we used the G-INS-i alignment algorithm and ‘1PAM/K = 2’ scoring matrix, with an offset value of 0.9, and the remaining parameters were set to default values. For the ribosomal ITS and IGS loci, we used the same parameters with the exception of an offset value set to 0.0 rather than 0.9. The resulting alignment was manually adjusted as necessary. The second approach utilized the GUIDANCE2 server (Sela et al. Reference Sela, Ashkenazy, Katoh and Pupko2015; http://guidance.tau.ac.il/ver2/) to remove regions aligned with low confidence (i.e. ambiguous regions) from the data set. In the GUIDANCE2 alignment, the multiple sequences alignment algorithm was set to MAFFT, implementing the ‘globalpair’ pairwise alignment method. Following the alignment step, GUIDANCE scores were calculated and residues with a GUIDANCE score < 0.90 were masked.
Alignments for each of the four loci (ITS, IGS, RPB1 and RPB2) were analyzed separately with a maximum likelihood (ML) criterion using RAxML v.8.2.10 (Stamatakis Reference Stamatakis2014) as implemented on the CIPRES Science Gateway v.3.3 (Miller et al. Reference Miller, Pfeiffer and Schwartz2010), using Niebla homalea as the outgroup. For each of the four single-locus trees, the ML analyses for the MAFFT versus GUIDANCE alignments did not reveal any conflicts; so, going forward, we used the more conservative GUIDANCE alignments. The single-locus topologies based on these GUIDANCE alignments were all congruent, so a concatenated data set was analyzed. Initial models of DNA sequence evolution for each marker were selected with jModelTest v.0.1 (Posada Reference Posada2008), using the AIC criterion. For all four markers, the GTRCAT option (a General Time Reversible model of nucleotide substitution under a Gamma model of rate heterogeneity) provided the best fit for our data. Additional exploratory analyses of alternative substitution models and partition strategies yielded topologies and nodal support values similar to GTRCAT, so GTRCAT was used for the concatenated data set for all final ML analyses. Additionally, for all ML analyses, the extended majority-rule consensus tree criterion was used. Branch support was estimated using 1000 pseudoreplicates and a non-parametric bootstrap approach.
A Bayesian phylogenetic hypothesis was also inferred from the concatenated GUIDANCE alignment using BEAST v.1.8.3 (Drummond & Rambaut Reference Drummond and Rambaut2007; Heled & Drummond Reference Heled and Drummond2010), also using Niebla homalea as the outgroup. The Yule-Process was implemented as the tree prior (branching model). The data matrix was partitioned by individual loci, implementing the GTR+G+I substitution model for each partition based on the jModelTest results performed prior to the ML analyses. Trees were estimated under both a strict molecular clock and an uncorrelated relaxed lognormal molecular clock (Drummond et al. Reference Drummond, Ho, Phillips and Rambaut2006). For both strict and relaxed lognormal estimates, two independent MCMC runs of 15 million generations were performed, sampling every 1000 steps. Chain mixing and convergence were inspected using the program Tracer v.1.6 (Rambaut & Drummond Reference Rambaut and Drummond2003), considering ESS values > 200 as good indicators. After excluding the first 25% of sampled trees as burn-in, trees from the two independent runs were combined using the program LogCombiner v.1.8.3 (Rambaut & Drummond Reference Rambaut and Drummond2003), and the final MCMC tree was estimated from the combined posterior distribution of trees using TreeAnnotator v.1.8.3 (Rambaut & Drummond Reference Rambaut and Drummond2009). The exclusion criterion of 25% was used because it was well above the point of convergence that was identified by inspecting the average standard deviation of split frequencies value.
Results
A total of 255 new sequences were generated for this study and aligned with 21 sequences downloaded from GenBank. The final, concatenated, four-locus alignment is available online as Supplementary Material File 1, and also from the Dryad Digital Repository: https://doi.org/10.5061/dryad.08kprr4zd. Our combined data set contained 2507 aligned positions, of which 886 were variable within the ingroup. Of these variable characters, 169 occurred in the ITS region, 184 in the IGS, 233 in the RPB2 and 300 in the RPB1. The total proportion of gaps and indeterminable characters in the alignment was 52.77%. The ML tree (Fig. 2) had a final ML optimization likelihood value of −14969.549914. Clades with thickened lines are supported by Maximum Likelihood Bootstrap (MLBS) values ≥ 75%; those denoted by capital letters are discussed in the text. The following taxa and clades have branch lengths much longer than other branches in the ML tree: Ramalina denticulata, R. leiodea, R. ovalis, R. pacifica, R. sayreana, and clade M (= the branch containing R. celastri and R. ovalis). The Bayesian phylogeny (see Supplementary Material Fig. S1, available online) exhibited no significant (MLBS values ≥ 75%) conflicts with the ML tree but was less resolved.

Fig. 2. Phylogenetic relationships within the genus Ramalina based on a maximum likelihood analysis of concatenated ITS, IGS, RPB1 and RPB2 sequences. Bootstrap values ≥ 75% are given above the internodes; these branches are depicted with thickened lines. Clades marked with capital letters are discussed in the text. Clades highlighted in grey are samples from the ingroup, the R. siliquosa complex. For chemotype abbreviations of samples from the R. siliquosa complex, see Table 2. Chemotype numbers for the R. americana and R. culbersoniorum samples follow the numbering systems of Culberson et al. (Reference Culberson, Culberson and Johnson1990) and LaGreca (Reference LaGreca1999). The dashed line indicates the branch connecting the two parts of the tree, which was divided because of space considerations. Scale = nucleotide substitution rate.
At the outset of this study, the ITS region was amplified using the primers BMB-CR and SLG-1 (Table 1), which anneal further towards the 5′ end of the rDNA SSU than does the primer ITS-1F, our preferred 5′ primer in recent years. Use of BMB-CR and SLG-1 caused PCR amplification of multiple PCR products, including both algal rDNA (c. 1250 bp) and fungal rDNA; in addition, in certain samples, two different sizes of fragments of fungal rDNA were amplified (c. 750 bp vs c. 900–1000 bp). Depending on the sample, sequencing of the larger fragment revealed the presence of Group I introns at either position 1512 or 1516 of the 18S rDNA (using the system of Gargas et al. (Reference Gargas, DePriest and Taylor1995)). Ramalina celastri, R. complanata, R. montagnei, R. paludosa and R. willeyi contained the 1512 intron, while R. americana, R. culbersoniorum, R. roesleri and R. sinensis contained the 1516 intron. None of the samples possessed introns at both positions. A BLAST search (Altschul et al. Reference Altschul, Madden, Schäffer, Zhang, Zhang, Miller and Lipman1997) using the position 1512 intron of R. paludosa as a query yielded multiple similar rDNA SSU sequences, the most similar (93% similarity) from Anthracothecium nanum (Zahlbr.) R. C. Harris (GenBank # KT232207), followed by R. complanata (92%; GenBank # FJ356152). Another BLAST search using the position 1516 intron from a R. culbersoniorum sample as a query yielded many similar rDNA SSU sequences, all from other Ramalina species; the most similar (95%) was from R. complanata (GenBank # HQ650720). We have deposited the two intron sequences used in our BLAST searches in GenBank (position 1512: GenBank # MN906756, 1516: GenBank # MN906757). Group I introns from both of these positions are commonly found in various lineages of lichenized fungi (e.g. DePriest & Been Reference DePriest and Been1992; Bhattacharya et al. Reference Bhattacharya, Lutzoni, Reeb, Simon, Nason and Fernandez2000; Gutiérrez et al. Reference Gutiérrez, Blanco, Divakar, Lumbsch and Crespo2007). Additional details of all the introns found in the present study, including their inferred folded secondary structures and alignment among different taxa, are given in LaGreca (Reference LaGreca1997).
The ML tree (Fig. 2) shows the 59 ingroup samples forming three well-supported clades (A, C and G), none of which are direct phylogenetic sisters. Most of the backbone branches of the reconstructed tree have low support (MLBS < 75%), except for one strongly supported clade (H) comprising all Ramalina species included in this study that produce medullary depsides and depsidones. By contrast, a number of strongly supported (MLBS > 75%), more derived groups (clades B, D, E, F and I–N) can be discerned. One of these is a clade (B) pairing R. roesleri with one of the clades of ingroup samples (C) as sister species. Another strongly supported clade comprises all south-eastern USA coastal plain and tropical Ramalina species (clade J). This includes a well-supported clade comprising R. montagnei, R. peruviana, R. stenospora and R. usnea (clade I). All geographically and chemically disparate specimens of Ramalina culbersoniorum form a strongly supported clade with other specimens of that species (E). Specimens of R. farinacea and R. subfarinacea group with strong support in clade F. Clade K includes R. fraxinea as sister to a clade (L) comprising R. menziesii and a fertile/sorediate species pair, R. leptocarpha and R. subleptocarpha. Finally, there is a robust clade pairing R. celastri with R. ovalis (M), and another strongly supported clade (N) comprising multiple specimens of R. sinensis together with two specimens of R. unifolia. Neither K, M nor N are robustly placed within the global phylogeny presented.
Discussion
The Ramalina siliquosa complex
Our ML analysis (Fig. 2) provides strong evidence that the European members of the Ramalina siliquosa complex comprise two distinct evolutionary lineages (‘phylogenetic species’ sensu Mishler (Reference Mishler and Brandon1996)), supporting a two-species classification (Table 2): R. siliquosa (Huds.) A. L. Sm. s. str. (clade C; 96% MLBS), including the hypoprotocetraric, protocetraric and salazinic chemotypes and R. cuspidata Nyl. (clade G; 99% MLBS), including the norstictic and stictic chemotypes. These are the oldest available names for these two taxa (for thorough taxonomic reviews see Laundon Reference Laundon1966; Sheard & James Reference Sheard and James1976; Sheard Reference Sheard1978a). The grouping of our samples into these two clades supports the two hypothetical biogenetic pathways proposed for their secondary products by previous workers (Culberson et al. Reference Culberson, Culberson and Johnson1977; Sheard Reference Sheard1978a). Interestingly, acid-deficient samples fall into both lineages. This result agrees with Sheard (Reference Sheard1978a), who observed that some individuals of the acid-deficient chemotype possess the typical morphology (i.e. terete branches with pigmented bases and pycnidia) of R. cuspidata, but others key out to R. siliquosa s. str. (i.e. flatter branches with non-pigmented bases and pycnidia). This also makes sense given that lichen secondary products can feasibly be caused by regulatory repression of the involved PKS pathways. For example, differential silencing of the atranorin versus norstictic acid PKS pathways in the cortex versus the medulla of Parmotrema hypotropum (Nyl.) Hale has been proposed (Armaleo et al. Reference Armaleo, Zhang and Cheung2008), and large differences in PKS gene expression have been demonstrated between two single-spore isolates from the same lichen, Cladonia grayi (Armaleo et al. Reference Armaleo, Sun and Culberson2011).
Table 2. Two competing taxonomies for the Ramalina siliquosa chemotype complex.

The occurrence of the Ramalina siliquosa complex in South-East Asia has been well known since Nylander's time (Nylander Reference Nylander1890), with three chemotypes (acid-deficient, protocetraric and salazinic) that mirror those found in Europe (Culberson Reference Culberson1970). One additional chemotype, producing divaricatic acid as its major medullary product, is reported here as new; it differs from the others in producing an orcinol-type para-depside (protocetraric and salazinic acids are both ß-orcinol depsidones). Unlike in Europe, the chemotypes in South-East Asia do not display zonation on cliffs (H. Kashiwadani & K. Moon, personal observation). South-East Asian material has been identified until now as R. siliquosa but is slightly different morphologically. Based on our ML analysis of 20 R. siliquosa specimens from Japan and Korea (Fig. 2, clade A; 96% MLBS), the South-East Asian populations belong to a separate species which we originally thought was new to science. A review of the literature, however, revealed a little-known variety of R. scopulorum (Ach.) Ach., var. semicuspidata Räsänen (Räsänen Reference Räsänen1940), which was elevated to species level by Sheard (Reference Sheard1978a) in a short footnote (op. cit., p. 936). Due to its obscurity, as well as an incomplete description and an absence of photographs in the original protologue, we provide a thorough account of this species in the ‘Taxonomic Conclusions’ section at the end of this paper.
Additional records of the Ramalina siliquosa complex from North America, South America and Africa exist in both the literature and herbaria (Howe Reference Howe1913; Fink Reference Fink1935; Calvelo & Liberatore Reference Calvelo and Liberatore2002; Gumboski et al. Reference Gumboski, Eliasaro, Scur, Lorenz-Lemke and Borges da Silveira2018; CNALH 2019). Most of these records are clearly misidentifications but others warrant further inspection; they may represent separate species, like R. semicuspidata. Furthermore, in Europe, the R. siliquosa complex occurs to the south in Portugal, Spain and Italy (Culberson et al. Reference Culberson, Culberson and Johnson1977; Nimis Reference Nimis2016), countries not included in the present study. Future investigations of this species complex in southern Europe might reveal other species, and provide answers to other questions, such as the taxonomic status of the rare 4-O-demethylbarbatic chemotype (‘Ramalina zopfii’, Table 2).
Relationships among other Ramalina species
In order to both identify a sister group for the Ramalina siliquosa complex and provide a preliminary phylogeny for the genus, 45 Ramalina samples representing 36 other taxa, mainly from North America and Europe, were added to our four-locus data set. As in previous studies (e.g. Pérez-Vargas & Pérez-Ortega Reference Pérez-Vargas and Pérez-Ortega2014; Gasparyan et al. Reference Gasparyan, Sipman and Lücking2017; references therein), the relationships among many of the Ramalina taxa included in our broader phylogeny remain unresolved (Fig. 2). This lack of resolution indicates that the evolutionary history of the genus may be too complex to be adequately captured by a dichotomously branching phylogeny based on only a few loci. A number of clades, however, were reconstructed with MLBS values ≥ 75%. For example, clade J (85% MLBS) includes all south-eastern United States coastal plain and tropical species. Although there are no obvious morphological characters uniting these species, all of them (except perhaps R. sayreana) occur on the coastal plain of the south-eastern United States or in humid, tropical habitats. There is little meaningful resolution within this clade, except for one monophyletic group (clade I; 99% MLBS) that includes only species with fusiform spores. This supports Howe's (Reference Howe1912) proposed section Fusisporae, comprising all species of Ramalina with fusiform spores.
Clade E (98% MLBS) indicates that a broader circumscription is needed for Ramalina culbersoniorum, a chemically rich species segregated from R. americana (which, unlike R. culbersoniorum, is almost always acid-deficient) on the basis of an ITS phylogeny (LaGreca Reference LaGreca1999). Subsequent phylogenetic studies of Ramalina (Stocker-Wörgötter et al. Reference Stocker-Wörgötter, Elix and Grube2004; Timsina et al. Reference Timsina, Stocker-Wörgötter and Piercey-Normore2012; Pérez-Vargas & Pérez-Ortega Reference Pérez-Vargas and Pérez-Ortega2014; Gasparyan et al. Reference Gasparyan, Sipman and Lücking2017) have all indicated that R. culbersoniorum is a robustly supported, monophyletic species. The present study expands the sampling by LaGreca (Reference LaGreca1999) of R. culbersoniorum with additional loci, one specimen from Florida and one each from two Midwestern states, Missouri and Arkansas. The Florida specimen (LaGreca 558, DUKE) represents the divaricatic/sekikaic chemotype (chemotype ‘8’ sensu Culberson et al. Reference Culberson, Culberson and Johnson1990), which is the most southern chemotype and the only chemotype known at the time that was not sequenced by LaGreca (Reference LaGreca1999). It clearly falls with the other R. culbersoniorum samples in our tree. The Missouri specimen (Ladd 19804, FH) is a lecanoric/evernic individual (chemotype ‘5’ sensu Culberson et al. Reference Culberson, Culberson and Johnson1990) that also falls within R. culbersoniorum. The Arkansas specimen (Ladd 21904, NY) is acid-deficient, and on that basis we expected it to be placed within R. americana; however, this sample also groups with R. culbersoniorum. Based on these results, we confirm that R. culbersoniorum occurs in the Midwest; however, we must also expand the circumscription of R. culbersoniorum to include an acid-deficient chemotype.
Clade F (81% MLBS) indicates that Ramalina farinacea and R. subfarinacea are closely related, corroborating ideas put forward by numerous lichenologists (Culberson Reference Culberson1966; Hawksworth Reference Hawksworth1968; Krog & James Reference Krog and James1977). In our analysis, samples of R. subfarinacea form a monophyletic group within a paraphyletic R. farinacea, the latter group including samples from both North America and Europe. More intensive sampling of this widespread species complex will be required to properly distinguish R. subfarinacea from R. farinacea. Notably, one of the R. farinacea samples sequenced in our study (LaGreca 514, DUKE) contains variolaric acid only. Variolaric acid is found in some chemotypes of R. farinacea in Europe (Zedda Reference Zedda2002), where it always co-occurs with other medullary substances (usually protocetraric acid). However, no other medullary substances could be detected in the variolaric acid-containing specimen we included in our analysis. This specimen, from Newfoundland, Canada, represents the first record of variolaric acid in a North American specimen of R. farinacea (cf. Bowler & Rundel Reference Bowler and Rundel1978), and also represents a new chemotype for this species.
Clade H (98% MLBS) is remarkable because it contains all of the Ramalina species in our data set that produce depsides and depsidones in the medulla. This supports the suggestion of Stocker-Wörgötter et al. (Reference Stocker-Wörgötter, Elix and Grube2004) and Timsina et al. (Reference Timsina, Stocker-Wörgötter and Piercey-Normore2012) that Ramalina species containing medullary products are derived. By contrast, all Ramalina species that lack these medullary products are found in clades K, L, M and N. Furthermore, interestingly, compared to all but one of the species (R. usnea) in Clade H, the species in these four clades (K, L, M and N) all produce large, horsey, strap-shaped thalli. It has been shown that environmental stress limits the growth of lichen thalli but it also seems to induce the production of secondary metabolites; in such situations, accumulated carbohydrates may be shifted to other pathways to produce secondary metabolites that are not essential for growth (Culberson & Armaleo Reference Culberson and Armaleo1992; Stocker-Wörgötter Reference Stocker-Wörgötter2001). In other words, slower mycelial growth resulting from inadequate nutrients may be linked to the production of secondary metabolites (Bu'Lock Reference Bu'Lock1961; Fox & Howlett Reference Fox and Howlett2008), which is related to the carbon-nutrient balance hypothesis (Bryant et al. Reference Bryant, Chapin and Klein1983). This was the explanation put forward by Timsina et al. (Reference Timsina, Sorensen, Weihrauch and Piercey-Normore2013) to explain the negative relationship they observed between culture diameter and the amounts of secondary metabolites produced by cultures of R. dilacerata, and by Hyvärinen et al. (Reference Hyvärinen, Walter and Koopmann2002) to explain nutrient content in Cladonia stellaris (Opiz) Pouzar & Vězda relative to herbivory. In other words, if cell growth and secondary metabolism are indeed competing processes (Bu'Lock Reference Bu'Lock1961), then the inability of the species in clades K, L, M and N to produce medullary depsides and depsidones might allow those species to spend more energy on growth, resulting in larger thalli.
Clade L (100% MLBS) includes three species endemic to the west coast of North America: Ramalina leptocarpha, R. menziesii and R. subleptocarpha. Ramalina menziesii, a pendulous Ramalina with holes in its thallus, is sister to a clade containing the other two species, both of which are strap-shaped and without holes. Ramalina leptocarpha and R. subleptocarpha are a classic lichen ‘species pair’ (Rundel & Bowler Reference Rundel and Bowler1976; Tehler Reference Tehler1982), the former being exclusively sexual and the latter reproducing only by soredia. Species pairs have been a popular subject for molecular phylogenetic studies of lichens at the species level (e.g. Lohtander et al. Reference Lohtander, Källersjö and Tehler1998; Myllys et al. Reference Myllys, Lohtander and Tehler2001; Buschbom & Mueller Reference Buschbom and Mueller2006), with most concluding they are merely populations of the same species (but see Widhelm et al. (Reference Widhelm, Egan, Bertoletti, Asztalos, Kraichak, Leavitt and Lumbsch2016) and Grewe et al. (Reference Grewe, Lagostina, Wu, Printzen and Lumbsch2018)). More extensive sampling will be needed to adequately address whether this is the case here. Clade M (100% MLBS) supports the results of Hayward et al. (Reference Hayward, Blanchon and Lumbsch2014) that R. ovalis is a distinct species from the morphologically similar but more broad-ranging R. celastri, which is sister to it. Equally interesting, however, is how the New Zealand specimen of R. celastri pairs with the Texas specimen of R. celastri in a well-supported clade (100% MLBS). This indicates that R. celastri may be a nearly cosmopolitan lichen species, being reported from North America, South America, Africa and Australasia; recent studies (e.g. Leavitt et al. Reference Leavitt, Divakar, Ohmura, Wang, Esslinger and Lumbsch2015, Reference Leavitt, Westberg, Nelsen, Elix, Timdal, Sohrabi, St. Clair, Williams, Wedin and Lumbsch2018) have demonstrated that widespread lichen species such as this might be more common than previously thought. Clade N (100% MLBS) pairs R. sinensis, a widespread, strap-shaped Ramalina known from South-East Asia and western and northern North America, with R. unifolia, a North American endemic species (Thomson Reference Thomson1990) known from Minnesota, Wisconsin and the Dakotas. In our analysis, R. sinensis is paraphyletic to a strongly supported (98% MLBS), monophyletic R. unifolia. The two species are strikingly similar, each bearing wide, flat lobes with broad, ecorticate areas on the lower surface. Additional sampling is required to assess the delimitation of R. unifolia from R. sinensis.
The ‘sibling species’ concept in lichens
Whereas cryptic species are defined as morphologically identical (or nearly identical) species (reviews: Mayr Reference Mayr1970; Futuyma & Kirkpatrick Reference Futuyma and Kirkpatrick2017; Struck et al. Reference Struck, Feder, Bendiksby, Birkeland, Cerca, Gusarov, Kistenich, Larsson, Liow and Nowak2017), sibling species can be thought of as a special subset of cryptic species that are each other's closest relatives (Steyskal Reference Steyskal1972; Bickford et al. Reference Bickford, Lohman, Sodhi, Ng, Meier, Winker, Ingram and Das2007). The term ‘sibling species’ is widely used among zoologists and entomologists (e.g. Rohland et al. Reference Rohland, Reich, Mallick, Meyer, Green, Georgiadis, Roca and Hofreiter2010; Lee & Lin Reference Lee and Lin2012) but the concept has also been used by botanists (e.g. Grant Reference Grant1981; Prata et al. Reference Prata, Sass, Rodrigues, Domingos, Specht, Damasco, Ribas, Fine and Vicentini2018). Culberson (Reference Culberson1986) argued for sympatric sibling speciation in a ‘sharply telescoped environment’ as the evolutionary mechanism producing the seven European sibling chemospecies he recognized in the Ramalina siliquosa complex (Table 2). The present study has demonstrated, however, that only two species exist in Europe (R. cuspidata and R. siliquosa) and, furthermore, they are not sibling species. Neither the majority-rule (Fig. 2) nor the single-gene trees (not shown) support a sister relationship for R. cuspidata and R. siliquosa s. str. In fact, the majority-rule tree indicates that the epiphytic, fistulose species R. roesleri is sister to R. siliquosa (80% MLBS) and that this pair (clade B; 80% MLBS), in turn, is sister to two other fistulose species, R. almquistii and R. dilacerata (clade D; 75% MLBS). Although cryptic, the species that comprise the R. siliquosa complex are not sibling species.
Many similar studies have recently demonstrated cryptic species in lichens (e.g. Singh et al. Reference Singh, Dal Grande, Divakar, Otte, Leavitt, Szczepanska, Crespo, Rico, Aptroot and Cáceres2015; Del-Prado et al. Reference Del-Prado, Divakar, Lumbsch and Crespo2016) but, as in the case of the R. siliquosa complex, these species-level lineages are not always sibling species. For example, in a study of the widespread lichen Parmelina quercina (Willd.) Hale, a single, nominal species was revealed to be four separate species, each with a distinct biogeographical distribution, three of which were closely related (Argüello et al. Reference Argüello, Del-Prado, Cubas and Crespo2007). However, the fourth species within the P. quercina complex, P. elixia Argüello & A. Crespo, was subsequently found to belong to a distinct, distantly related evolutionary lineage, Austroparmelia (Crespo et al. Reference Crespo, Ferencova, Pérez-Ortega, Elix and Divakar2010). Similar patterns of cryptic, species-level lineages not forming sibling species include examples in the brown parmelioids (Leavitt et al. Reference Leavitt, Esslinger, Divakar, Crespo and Lumbsch2016), Xanthoparmelia (Hodkinson & Lendemer Reference Hodkinson and Lendemer2011), Parmelia (Divakar et al. Reference Divakar, Leavitt, Molina, Del-Prado, Lumbsch and Crespo2015), Porina (Baloch & Grube Reference Baloch and Grube2009) and others.
The range of evolutionary processes that have been associated with diversification of morphologically cryptic species are numerous, from morphological stasis (Nevo Reference Nevo2001) to convergence (Grube & Kantvilas Reference Grube and Kantvilas2006) to novel symbiotic interactions (Schneider et al. Reference Schneider, Resl and Spribille2016). In the Xanthoparmelia pulla group, rare intercontinental dispersal, followed by diversification, resulted in multiple cryptic species (de Paz et al. Reference de Paz, Cubas, Crespo, Elix and Lumbsch2012). Similarly, in the morphologically variable Leptogium furfuraceum-L. pseudofurfuraceum complex, transoceanic dispersal produced four geographically disjunct phylogenetic lineages (Otálora et al. Reference Otálora, Martínez, Aragón and Molina2010). In these examples, there is strong evidence for allopatric sibling speciation, driven by geographical isolation. A similar scenario might explain why the South-East Asian species revealed in the present study, Ramalina semicuspidata, is morphologically similar to the European R. siliquosa complex; however, phylogenetic evidence for an intercontinental dispersal event is lacking (Fig. 2). By contrast, sympatric speciation, the process by which sibling species co-occur in the same habitats, is reportedly much less common than allopatric speciation (Futuyma & Kirkpatrick Reference Futuyma and Kirkpatrick2017). This is because morphologically similar, co-occurring sister species cannot coexist over time: one either gets outcompeted or adapts to a different ecological niche (Zeigler Reference Zeigler2014).
Therefore, contrary to ideas put forward by Culberson (Reference Culberson1986), the Ramalina siliquosa complex is not an example of sympatric sibling speciation but rather an example of parallel, or perhaps convergent, evolution (a possibility hinted at by Culberson et al. (Reference Culberson, Culberson and Johnson1993)). Another supposed example of sympatric sibling speciation in lichens discussed by Culberson (Reference Culberson1986) was also recently debunked: the Parmotrema perforatum complex. In two classic papers about this complex, Culberson (Reference Culberson1973) and Culberson & Culberson (Reference Culberson and Culberson1973) proposed six sympatric sibling species, each characterized by different combinations of chemistry and reproductive mode (sexual vs asexual). Using a combination of phylogenetic analysis and multi-species coalescent species delimitation methods, Widhelm et al. (Reference Widhelm, Egan, Bertoletti, Asztalos, Kraichak, Leavitt and Lumbsch2016) found that although all their apotheciate samples sorted into three separate, well-supported clades, the relationships among these clades did not correlate with the similarity of their secondary chemistries. Furthermore, no correlation was found between their reconstructed phylogeny and the reproductive mode of their samples. Although a test of monophyly for the P. perforatum complex awaits a more comprehensive analysis at the genus level, Widhelm et al. (Reference Widhelm, Egan, Bertoletti, Asztalos, Kraichak, Leavitt and Lumbsch2016) reduced the complex from six putative sibling species to four and, furthermore, demonstrated that the Culbersons’ traditional sibling species and chemospecies are not supported by phylogenetic analyses.
If the chemotypes of Ramalina cuspidata and R. siliquosa are not sibling species, then what is responsible for their remarkable vertical zonation? In the sublittoral and littoral zones below where these lichens grow, multiple ecological factors influence patterns of zonation, including tides, wave action, type of rock, steepness of the topography, salinity and herbivory/predation (e.g. Underwood & Jernakoff Reference Underwood and Jernakoff1984; Farrell Reference Farrell1991; Sarver & Foltz Reference Sarver and Foltz1993; Chu et al. Reference Chu, Seaward and Hodgkiss2000). On rocky coasts, a steep salt fall gradient may exist, as was demonstrated in Portugal using R. canariensis J. Steiner as a biomonitor (Figueira et al. Reference Figueira, Pacheco, Sousa and Catarino1999a, Reference Figueira, Pacheco, Sousa, Catarino, Brebbia, Jacobson and Powerb). Perhaps the chemotypes that occur lower down these maritime cliffs have a higher tolerance for salt than those above them; moderate salt tolerance was, in fact, demonstrated in one isolate of the R. siliquosa complex by Yamamoto et al. (Reference Yamamoto, Takahagi, Sato, Kinoshita, Nakashima and Yoshimura2001), who grew fungal cultures on media of varying concentrations of NaCl. An alternative, or perhaps complementary, explanation is that different algal photobionts of these lichen fungi possess different levels of salt tolerance, much like the photobionts of some Lepraria spp. possess different levels of tolerance to rain exposure (Peksa & Škaloud Reference Peksa and Škaloud2011). In other words, the algae may actually be driving the chemotype zonation. Yet another factor that could be causing the zonation of chemotypes on these cliffs is mite herbivory. Studies of one cliff in Bornholm revealed that R. siliquosa is grazed more heavily by orbatid mites than R. cuspidata (Gjelstrup & Søchting Reference Gjelstrup and Søchting1979). Future investigations of these lichens, pairing ecological sampling methods with modern, phylogenomic approaches, might uncover the mechanisms underlying their zonation.
Taxonomic Conclusions
Ramalina semicuspidata (Räsänen) Sheard
Canadian Journal of Botany 56, 936 (1978).—Ramalina scopulorum Ach. var. semicuspidata Räsänen, Journal of Japanese Botany 16, 87 (1940); type: Japan, Kyushu, Prov. Ohsumi, 22 August 1913, Yasuda 370 (TUR—holotype; TNS—isotype) [TLC: usnic and salazinic acids].
Diagnosis. Morphologically close to Ramalina cuspidata and R. siliquosa, differing mainly by the presence of pseudocyphellae and the rare production of scattered soredia which are initiated by isidia-like protuberances.

Fig. 3. Isotype of Ramalina semicuspidata (A. Yasuda 370, TNS). A, specimen label; B, intermixed thalli. Scale = mm. In colour online.
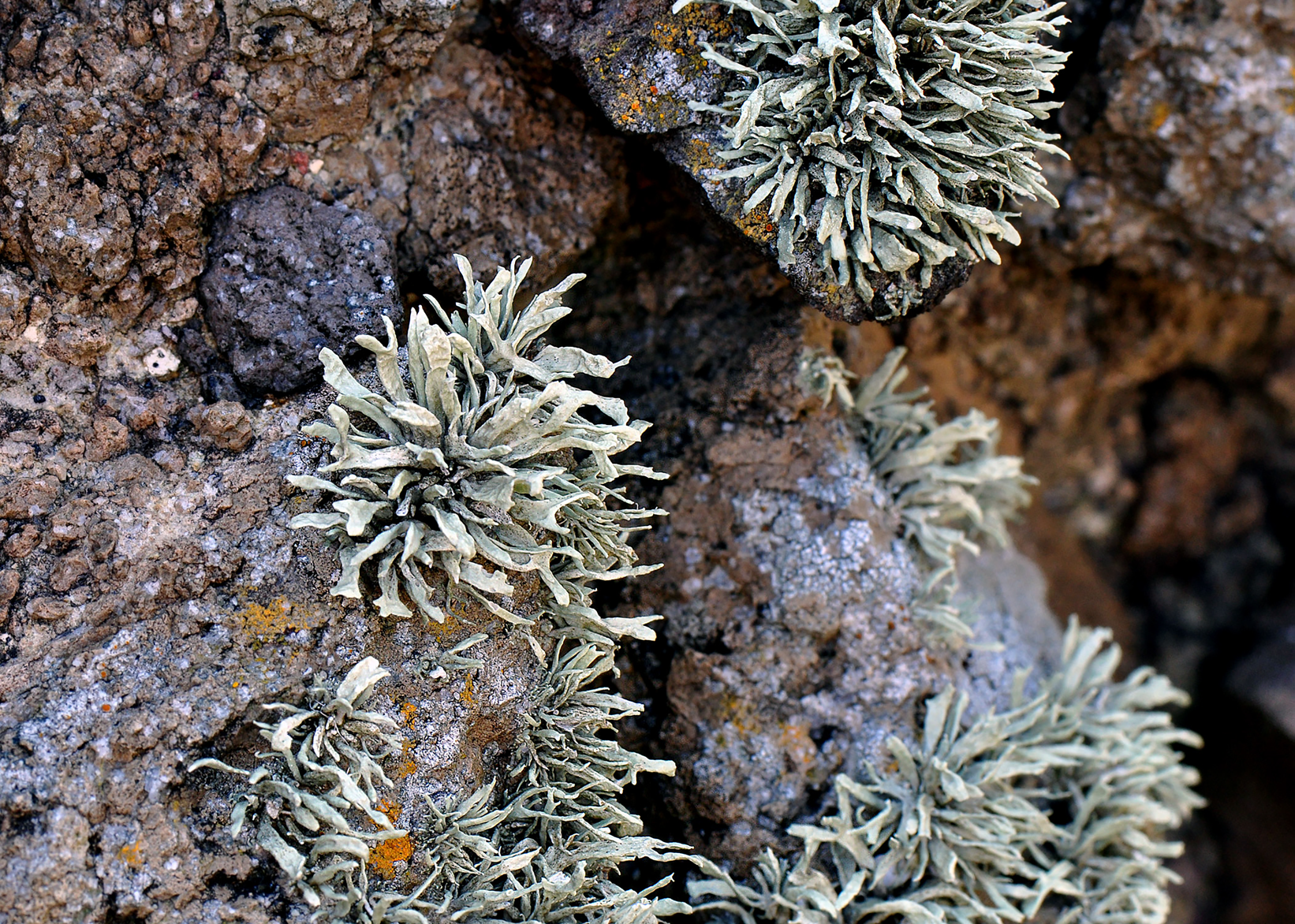
Fig. 4. Habit of Ramalina semicuspidata in Japan (Kashiwadani 51488, TNS).
Thallus saxicolous, erect or rarely subpendulous, caespitose, 1.5–3(–8) cm long, growing from a common holdfast. Surface pale yellow-green (reddish brown in herbaria), holdfast unpigmented to rarely blackened. Branches solid, simple or sparingly branched, 1–3(–5) mm wide, dorsiventral or terete, dorsiventral branches flattened or more or less slightly canaliculate, matt or subnitid, surface smooth or uneven, irregularly ridged by protruded pycnidia, rarely foveolate. Pseudocyphellae ellipsoid or orbicular, often with a slit or tiny cracks near the centre, laminal or marginal, sparse or rarely very conspicuous, especially towards the base. Soredia rare; when present, initiated as isidiate protuberances, and not arranged in soralia but instead scattered marginally or subterminally on the main branches. Thallus 300–800(–1000) μm thick; cortex indistinct, c. 10 μm thick; chondroid tissue continuous or dissected by pseudocyphellae, often penetrating into the medulla, clearly to moderately cracked, 50–180(–300) μm thick.
Apothecia common, subterminal, submarginal or laminal (lateral on terete branches); disc flat, becoming convex with age; thalline exciple entire, pseudocyphellate; hymenium 60–65 μm high; hypothecium 30–40 μm thick; proper exciple 50–100 μm thick; chondroid tissue of thalloid exciple conspicuous, often connected with exciple; ascospores hyaline, broadly ellipsoid, 2-celled, with or without additional septa, 10–12 × 4–5 μm.
Pycnidia common, unpigmented.
Secondary chemistry
Four chemotypes (races) are known: 1) usnic and salazinic acids; 2) usnic and divaricatic acids (previously unreported for this taxon); 3) usnic and protocetraric acids; 4) usnic acid only (acid-deficient).
Ecology and distribution
This lichen grows on non-calcareous, maritime rocks in Japan and Korea.
The protocetraric chemotype of Ramalina semicuspidata is rare, being known from only a handful of Japanese samples (Culberson Reference Culberson1970; Hamada Reference Hamada1985; Kashiwadani Reference Kashiwadani1992). Unfortunately, no fresh material of the protocetraric chemotype was available for DNA extraction but the known specimens are morphologically indistinguishable from all other individuals included in this study, so they are provisionally included within R. semicuspidata. The divaricatic acid race (reported here as new), the salazinic acid race, and the acid-deficient races, by contrast, are commonly found in both Japan and Korea.
Selected specimens examined
Race 1, usnic and salazinic acids. Japan: Hokkaido: Prov. Nemuro, Cape Nosappu, Kurokawa 65711, Lich. Rar. Crit. Exs. no. 679 (TNS, US). Honshu: Prov. Izu (Shizuoka Pref.), Kamo-gun, Minamiizu-cho, Irozaki Harbour, Kashiwadani 51488 (TNS); Shimoda-city, Cape Tsumekizaki, Kashiwadani 51491 & 51493 (CPU, NIBR, TNS); ibid., Tanaka s. n. (hb. Kashiwadani 51690, NIBR, TNS); Kamo-gun, Hamazaki-mura (Shimoda-city), Suzaki, Kurokawa s. n., Lich. Jap. Exs. no. 290 (TNS, US); Prov. Shima (Mie Pref.), Shima-gun. Daiwô-zaki, Murai s. n., Lich. Jap. Exs. no. 145 (TNS, US); Shimoda-city, Suzaki, Lich. Min. Cogn. Exs. no. 321, Shibuichi 8382 & Yoshida (DUKE, FH, TNS, US); Prov. Kii (Wakayama Pref.), Cape Kajino-zaki, Nishi-Muro-gun, Watari s. n. (DUKE). Kyushu: Prov. Bungo (Ohita Pref.), Ohita-city, Saganoseki-cho, Sekizaki, Umezu 4-1 (hb. Kashiwadani 51505, NIBR, TNS); Kita-amabe-gun, Saganoseki-cho, Sekizaki, Matsumoto & Iwashina s. n., Lich. Min. Cogn. Exs. no. 43 (TNS, US); Prov. Higo (Kumamoto Pref.), Amakusa-gun, c. 1.4 km NNE of Cape Shikizaki, Tomioka, Moon 16034 & Kashiwadani (NIBR); ibid., Kashiwadani 51830, Takeshita & Moon (NIBR, TNS); Prov. Tsushima (Nagasaki Pref.), Tsushima-city, Kamitsushima-machi, south end of Mogihama swimming beach, Kashiwadani 51626 & Moon (NIBR, TNS). Shikoku: Prov. Awa (Kagawa Pref.), Shodo-gun, Shodo-shima Island, Lich. Min. Cogn. Exs. no. 170, Moon 3516 (DUKE, FH, TNS, US).—Korea: Incheon: Jabong-do Island, Moon 13197 (CPU, NIBR, TNS); Mo-do Island, Modo Port, Moon 15287 & Kashiwadani (NIBR, TNS). Prov. Gangwon-do: Yangyang-gun, Hajodae, Kashiwadani 51529 (NIBR, TNS). Prov. Gyungsangbuk-do: Pohang-shi, Nam-gu, near the Daedongbae Elementary branch school, Moon 13430 & Kashiwadani (NIBR). Prov. Jeollanam-do: Goheung-gun, Mondol beach, Moon 13712 (NIBR, TNS). Prov. Jeju: Jeju-shi, Yongduam Rock, Moon 15676 & Kashiwadani (NIBR, TNS).
Race 2, usnic and divaricatic acids. Japan: Kyushu: Prov. Tsushima (Nagasaki Pref.), Tsushima-city, Kamitsushima-machi, Ajiro, Kashiwadani 51634 & Moon (NIBR, TNS); ibid., Kashiwadani 51638 & Moon (NIBR, TNS).—Korea: Incheon: Shi-do Island, Sugi Beach, Moon 15291 & Kashiwadani (NIBR, TNS); Jabong-do Island, Moon 13195 (NIBR, TNS). Prov. Gangwon-do: Yangyang-gun, Sol Beach, Kashiwadani 51538 (NIBR, TNS). Prov. Gyungsangnam-do: Tongyoung-shi, Sanyang-eup, Shinjeon-ri, Moon 13377 (NIBR). Prov. Jeju: Jeju-shi, Yongduam Rock, Moon 15675 & Kashiwadani (NIBR, TNS); Seogwipo-shi, Cape Seopjikoji, Moon 15589, Kashiwadani & Ahn (NIBR, TNS).
Race 3, usnic and protocetraric acids. Japan: Honshu: Prov. Rikuzen (Miyagi Pref.), Oga-gun, Senjojiki, Sasaki 8224 (TNS); Prov. Shimofusa (Chiba Pref.), Unakami-gun, Cape Inobu, Imazeki s. n. (TNS); Prov. Izu (Shizuoka Pref.), Kamo-gun, N of Yahatano, Kurokawa 70981 & 70983 (TNS); S of Itho, Yahatano, Hasgudate, Sasaki s. n. (DUKE, TNS) (Kashiwadani Reference Kashiwadani1992).
Race 4, usnic acid only. Japan: Honshu: Prov. Sagami (Shizuoka Pref.), Mitsuiwa, Cape Manazuru, Hisauchi 7 (TNS); Prov. Izu (Shizuoka Pref.), Kamo-gun, Tsumezaki, Shibuichi 4461 (TNS); ibid., Kurokawa 701009 (TNS); Prov. Noto (Ishikawa Pref.), Wajima-city, Aramiko-jima, Satomi s. n. (TNS) (Kashiwadani Reference Kashiwadani1992); Prov. Owari (Aichi Pref.), Chita Peninsula, Cape Hazu, Takahashi 327 (TNS). Kyushu: Prov. Hizen (Nagasaki Pref.), Faurie s. n. (KYO, TNS).—Korea: Incheon: Jabong-do Island, Moon 13198 (NIBR, TNS); Jabong-do Island, around Meolgot, Moon 13489 & Kashiwadani (NIBR). Prov. Gangwon-do: Yangyang-gun, Hajodae, Kashiwadani 51532 (NIBR, TNS). Prov. Gyungsangbuk-do: Gyungju-shi, Gampo-up, Ohryu Beach, Moon 13460 (CPU, NIBR). Prov. Jeju: Jeju-shi, U-do Island, Dolkanee, Moon 15597, Kashiwadani & Ahn (NIBR, TNS); Pukcheju-gun (= Jeju-shi), Hado-ri, Lich. Min. Cogn. Exs. no. 216, Kashiwadani 43860 & Moon (DUKE, FH, TNS, US). Prov. Jeollabuk-do: Gunsan-si, Munyeo-do Island, Moon 13191 (NIBR). Prov. Jeollanam-do: Goheung-gun, Geogeum-do Island, Shinchon-ri, Moon 13914 (CPU, NIBR); Jindo-gun, Setbae shelter, Moon 15236 & Ahn (NIBR).
Acknowledgements
This paper originated as Chapter 3 of the senior author's Ph.D. dissertation at Duke University, expanding into its present form over the intervening 23 years at four other botanical institutions: FH, BM, CUP and F. The researchers, staff and students of all five of these institutions are warmly thanked for their support, encouragement and helpful discussions over the years. We are especially grateful to the following people for their assistance: Ted Ahti, Daniele Armaleo, Michele Cilia, Chicita Culberson, William Culberson, Jerrold Davis, Robert Dirig, Stefan Ekman, Karen Hansen, Kathie Hodge, Anita ‘Rusty’ Johnson, Mary McKellar, Molly McMullen and Donald Pfister. We also thank Frances Anderson, Shelly Benson, Ernie Brodo, Doug Goldman, Trevor Goward, Urs Groner, Starri Heiðmarsson, Laurel Kaminsky, Doug Ladd, Clayton Newberry, Frederick Seavey, Larry St. Clair, Jiro Tanaka, Kazuko Umezu and Alison Withey for generously providing fresh material for DNA extraction. In addition, we are grateful to Chorong Ahn, Andrea Gargas, René Larsen and Shunji Takeshita for their assistance in the field. Steve Russell and Holger Thüs (both BM), and Michaela Schmull (FH), are thanked for shipping the senior author's DNA extractions and frozen specimens to Chicago. Suzanne Joneson (Waukesha/Milwaukee) graciously allowed us to use DNA extracts of R. almquistii and R. dilacerata from her master's thesis work. John Sullivan (Ithaca) gave invaluable advice and assistance with our initial phylogenetic analyses. Duke University's Lutzoni Lab (François Lutzoni, Nic Magain, Ian Medeiros, Carlos Pardo De la Hoz and Jolanta Miadlikowska) made constructive suggestions regarding data analysis, as well as comments on the manuscript. Leena Myllys (H) kindly allowed the senior author to examine the Nylander type of R. cuspidata. Keith Babuszczak, Greg Beirise and Daniel Lopez provided invaluable logistical support for the senior author. The British Lichen Society is warmly thanked for subsidizing the senior author's trip to Helsinki, where this research was first presented, and also for hosting field meetings in places where the R. siliquosa complex grows in profusion. This research was supported by an A.W. Mellon Foundation grant for graduate training in plant systematics to the Duke University Department of Botany. It was also partly supported by scientific research project numbers NIBR 01039 (2012–2014) and 01105 (2015–2016) from the Republic of Korea government to KHM. A generous grant to the senior author from the Field Museum's Visiting Scholar Fund provided the funds necessary to complete this project.
Supplementary Material
To view Supplementary Material for this article, please visit https://doi.org/10.1017/S0024282920000110.