Introduction
Species of the reindeer lichen Cladonia subgen. Cladina (Cladoniaceae, Lecanoromycetes) have an important European role as ‘species of community interest’ in Annex V of the EEC Habitat Directive (1992) (Stenroos et al. Reference Stenroos, Hyvönen, Myllys, Thell and Ahti2002). Three of these species, Cladonia arbuscula, C. mitis and C. rangiferina, which are protected in Hungary, are the focus of our study. All three species have various propagation modes (vegetative via thallus fragments, asexual via asexual propagules (conidia) and sexual via ascospores (Crittenden Reference Crittenden2000)). Therefore, reproductive capacity is unlikely to be the limiting factor in their distribution. They are characterized by a fruticose growth form, forming cushions, richly branched podetia, and an evanescent crustose primary thallus (Verseghy Reference Verseghy1994). Less than 0.5% of lichen species worldwide are able to develop closed, carpet-like ground cover including species of the subgenus Cladina of the genus Cladonia as well as the genera Cetraria, Stereocaulon and Alectoria (Crittenden Reference Crittenden2000). Lichen-dominated vegetation covers c. 8% of the world's surface, the largest part of which is found in subarctic and arctic regions (Larson Reference Larson1987). The mat-forming lichens appear in open habitats, where the cover of vascular plants is insignificant. In boreal regions, mat-forming lichens represent 60% of reindeer nutrition in winter, and the damage caused by trampling is considerable throughout the year (Miller Reference Miller1976; Boertje Reference Boertje1990). Mat-forming lichens can grow on top of one another's thalli, the freshly developing thalli often growing over the dead ones. In the north-eastern part of Finland, more than 90% of the total lichen biomass is produced by Cladonia rangiferina and C. mitis (Helle et al. Reference Helle, Aspi and Tarvainen1983). However, in Hungary these species do not form extensive uninterrupted ground cover, but generally occur in patches of c. 10–30 cm2 (exceptionally up to 1 m2) (personal observations). Their sporadic occurrence may be caused by habitat loss due to grazing and trampling by wild animals, vehicle tracks (off-road, motocross and quad bikes), and less frequently by tourism.
The taxonomy of the ‘Cladina’-group was studied in detail by Ahti (Reference Ahti1961) but it remains unclear and continues to be the subject of investigation (Ahti Reference Ahti2000; Ahti & DePriest Reference Ahti and DePriest2001; Carbonero et al. Reference Carbonero, Montai, Woranovicz-Barreira, Gorina and Iacomini2002; Stenroos et al. Reference Stenroos, Hyvönen, Myllys, Thell and Ahti2002; Myllys et al. Reference Myllys, Stenroos, Thell and Ahti2003; Bültmann Reference Bültmann, Bültmann, Fartmann and Hasse2006; Piercey-Normore et al. Reference Piercey-Normore, Ahti and Goward2010; Ahti et al. Reference Ahti, Stenroos and Moberg2013; Athukorala et al. Reference Athukorala, Pino-Bodas, Stenroos, Ahti and Piercey-Normore2016). The greyish Cladonia rangiferina is a species well-delimited from C. mitis and C. arbuscula both morphologically (having more robust branches and tips turning in the same direction) and chemically (containing atranorin and fumarprotocetraric acid). The usnic acid-containing and therefore yellowish C. arbuscula has three subspecies, C. arbuscula (Wallr.) Flot. subsp. arbuscula (with additional psoromic acid), subsp. squarrosa (Wallr.) Ruoss (with fumarprotocetraric acid) and subsp. mitis (Sandst.) Ruoss (with rangiformic acid) (Ruoss Reference Ruoss1987), but only the latter two have been recognized in Hungary (Farkas et al. Reference Farkas, Lőkös, Molnár and Lipnicki2012). Following traditional nomenclature (since it is irrelevant for our study at which rank these differences are handled), the three existing taxa (C. arbuscula subsp. squarrosa, C. arbuscula subsp. mitis and C. rangiferina) are treated at species level as C. arbuscula, C. mitis and C. rangiferina.
Cladonia arbuscula and C. mitis are circumpolar species which occur in boreal-subarctic-subalpine regions; however, C. rangiferina is also a circumpolar species but with an arctic-alpine distribution (cf. Dingová Košuthová & Šibík Reference Dingová Košuthová and Šibík2013). The major distribution of these species is boreal, but they can also be found in alpine and montane zones from Central Europe to the Mediterranean (Myllys et al. Reference Myllys, Stenroos, Thell and Ahti2003; Dingová Košuthová & Šibík Reference Dingová Košuthová and Šibík2013). Their distribution and ecology overlap widely and are seldom investigated with statistical rigour (Ahti Reference Ahti2000; Fabiszewski & Szczepańska Reference Fabiszewski and Szczepańska2010; Landolt et al. Reference Landolt, Bäumler, Erhardt, Hegg, Klötzli, Lämmler, Nobis, Rudmann-Maurer, Schweingruber and Theurillat2010; Dingová Košuthová & Šibík Reference Dingová Košuthová and Šibík2013). Hungarian populations of these three species of Cladonia grow near to the southern edge of their European distribution (GBIF 2020) (Fig. 1) and in the Balaton Uplands they occupy a narrow range as isolated populations. The aim of this study was to compare the environmental and microhabitat characteristics of these species in the populations found in the Balaton Uplands.
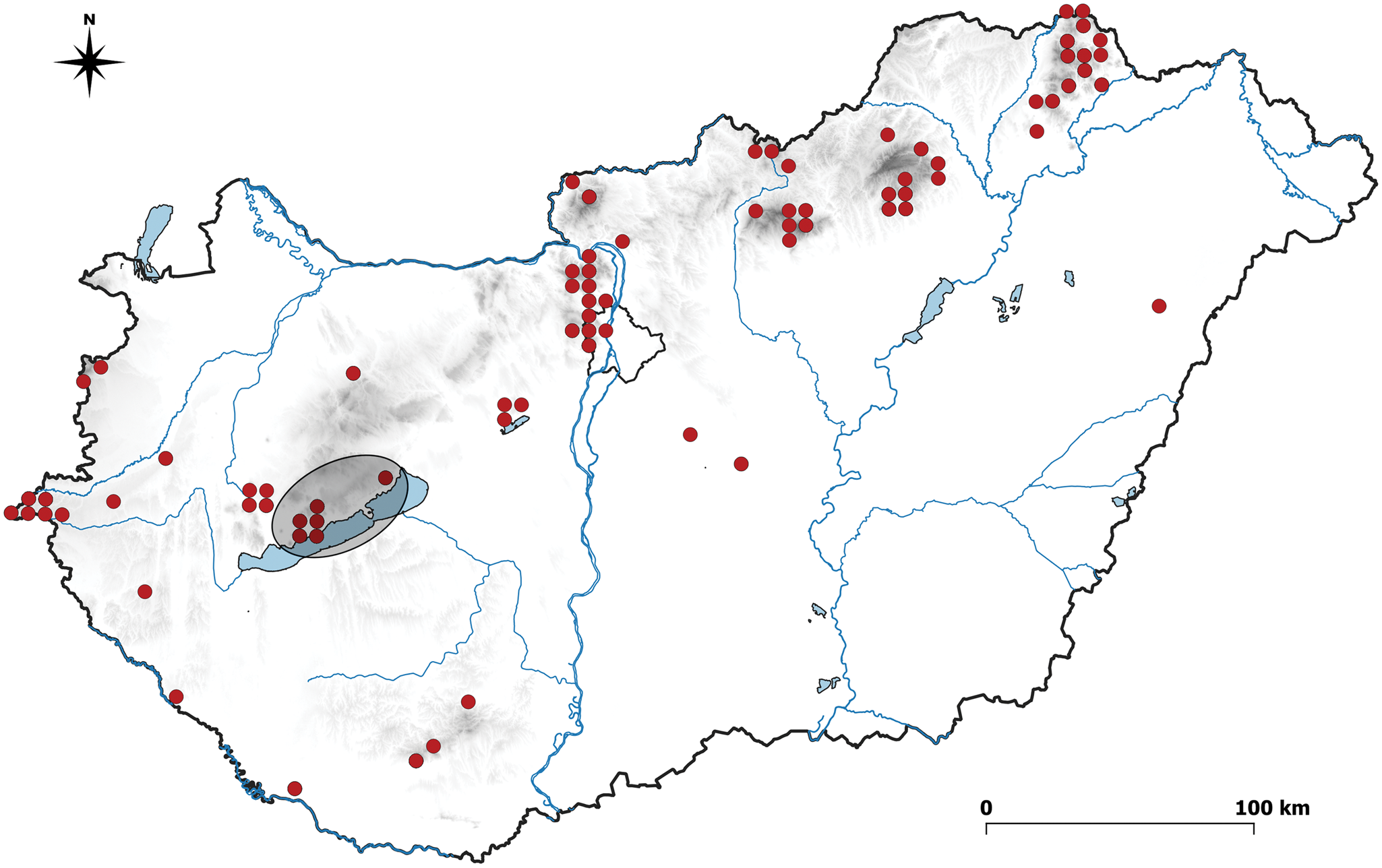
Fig. 1. Distribution of Cladonia subgen. Cladina in Hungary. Circles represent an area of c. 5 × 6 km (a quarter of the grid unit) (Niklfeld Reference Niklfeld1971). The Balaton Uplands are indicated by the ellipsoid highlighted in grey. In colour online.
Material and Methods
Study area
Our study area is situated to the north of Lake Balaton, in the Balaton Uplands region (Fig. 1). Most of the quadrats are located in the Káli-medence basin, which is bordered by basalt mountains (Sátorma-hegy, Öreg-hegy and Fekete-hegy) in the north and red sandstone mountains in the east and south at 150–400 m a.s.l. The west part of the Káli-medence consists of lime-free sandstone conglomerate, and the underlying rock of the centre of the basin is Triassic limestone and dolomite (Futó Reference Futó2005). The climate of this area is moderately cool (annual mean temperature is 10.2–10.5 °C), with a mean annual precipitation of c. 620–640 mm. This area can be characterized by the high proportion of southern, sub-Mediterranean species. Zonal vegetation is dry deciduous forest (closed thermophilous Quercus pubescens forest) mixed with calcareous rocky steppes and Q. pubescens scrub stands. Acidofrequent oak forests grow on Permian red sandstone underlying rock together with acidophilous vascular plant, lichen and bryophyte species (Fig. 2). Tall-sedge beds and marshes remain as fragments alongside Lake Balaton (Dövényi Reference Dövényi2010). The distribution of lichens in the study area is affected by wild animals (grazing and trampling), vehicle tracks (off-road, motocross and quad bike) and less frequently by tourism.

Fig. 2. Examples of the habitats in which Cladonia arbuscula, C. mitis and C. rangiferina were found in the Balaton Uplands, Hungary. A, open acidofrequent oak forest. B, siliceous open rocky grassland. C, patches of Cladonia thalli among bryophytes in open acidofrequent oak forest. In colour online.
Sampling of environmental and species data
A total of 46 quadrats was sampled. The sampling points were selected based on information obtained from the literature and in herbaria (BP, BTM, EGR), as well as new locations found during the study (Fig. 1).
A sampling frame of flexible rope was used for ease of handling on rocky grasslands and wooded cliffs. These individual sampling quadrats (2 × 2 m) were placed where at least one of Cladonia arbuscula, C. mitis or C. rangiferina were present, avoiding tree trunks, but often just under the tree canopy.
In each quadrat, we recorded aspect (north (N), east (E), south (S), west (W), north-east (NE), south-east (SE), south-west (SW), north-west (NW) and hilltop), underlying rock type (red sandstone, gravel, Pannonian sandstone and basalt), habitat type (open acidofrequent oak forests (L4b), closed acidofrequent oak forests (L4a), slope steppes on stony soils (H3a), siliceous open rocky grasslands (G3), open sand steppes (G1), dry Calluna heaths (E5) and wet and mesic pioneer scrub (P2b)) (General National Habitat Classification System, Á-NÉR; MÉTA 2019), elevation (m a.s.l.), total percentage cover of exposed rock, bryophytes, lichens, vascular plants and canopy (estimated as an upwards occlusion of skylight) and the number of species of lichens, bryophytes and vascular plants were recorded. Soil pH and CaCO3 content were measured as follows. Soil samples were collected from five points in each quadrat and mixed in the field. A minimum of 25 g combined soil sample per quadrat was necessary for the analyses. Soil pH measurements were carried out by potentiometry using a Handylab pH 11, according to a recognized protocol (Hungarian Standard: MSZ-08-0206/2:1978) in the laboratory. Gas volumetry was used for the analysis of CaCO3 content using a Scheibler calcimeter at the Institute of Environmental and Earth Sciences, University of Sopron (Hungarian Standard: MSZ-08-0206/2:1978) and was expressed as a percentage. Soil depth (cm) was measured with a pointed iron rod at five points within each quadrat (four corners and the centre), and the mean value of these measurements was calculated. The impact of animals and general small-scale disturbance of quadrats was assigned to a four-point scale: 0 = zero disturbance, 0–5% trampling and grazing; 1 = weak disturbance, 5–25% trampling and grazing; 2 = medium disturbance, 25–50% trampling and grazing; 3 = strong disturbance, above 50% trampling and grazing.
Every 2 × 2 m quadrat was divided into 400 microquadrats (each of 10 × 10 cm), in which the horizontal thallus size (cm2) and the number of well-developed and fragmented thalli of the three Cladonia species were recorded. Thalli (obviously broken) under 1 × 1 cm in size as well as some juvenile thalli were considered to be fragmented.
The nomenclature of vascular plants follows Király (Reference Király2009) and The Plant List (2013; http://www.theplantlist.org/); lichen species are named according to CABI (2020), and names of bryophyte species are based on Hill et al. (Reference Hill, Bell, Bruggeman-Nannenga, Brugués, Cano, Enroth, Flatberg, Frahm, Gallego and Garilleti2006) and Grolle & Long (Reference Grolle and Long2000). Small samples of the Cladonia species were collected from each 10 × 10 cm microquadrat, where at least one species was sampled and identified on the basis of secondary substances determined by HPTLC analysis in solvent system C according to Arup et al. (Reference Arup, Ekman, Lindblom and Mattsson1993). Several specimens of the three species collected from the study area are deposited in the Bakony Museum of the Hungarian Natural History Museum (BTM). Bryophyte, vascular plant and other lichen species were collected from quadrats for the purpose of identification.
Distribution maps were prepared using QGIS (QGIS.org 2020). An adaptation of the Central European grid system (Niklfeld Reference Niklfeld1971; Borhidi Reference Borhidi1984) was used to identify the collection sites of herbarium specimens (BP, BTM, EGR).
Data processing
Univariate statistical and multivariate methods were used for data analyses. Box and whisker plots and bar graphs were used to visualize the differences between Cladonia mitis, C. arbuscula and C. rangiferina. As 14 of the 46 quadrats contained two or three of these species, these data were not accessible to more complex statistical analyses.
Canopy cover, total number and cover of species (vascular plants, bryophytes and lichens), pH, elevation, soil depth and CaCO3 content were subjected to Mann-Whitney tests to compare quadrats with one Cladonia species with those without that particular Cladonia species (e.g. 15 quadrats of C. arbuscula were compared with 31 quadrats without C. arbuscula) (Zar Reference Zar1999). For categorical variables (underlying rock type, aspect, vegetation type and disturbance), the Fisher exact test was used to compare quadrats with and without the investigated Cladonia species.
Two Principal Component Analyses (PCA) were calculated, one for species composition data and the other using environmental variables (bryophyte, lichen, vascular plant, canopy and exposed rock percentage cover as well as soil pH and CaCO3 content). Species that occurred in fewer than five quadrats were excluded from the PCA analyses and therefore only 46 species of the 154 vascular plants, lichens and bryophytes recorded were used in the analyses. Descriptive statistics (mean, max., min.) were calculated using Statistica 13.6 (StatSoft 2019). The univariate statistics (Mann-Whitney test, Fisher exact test) and multivariate analyses were calculated using R version 3.6.1 (R Core Team 2019). Box and whisker plots and bar graphs were produce using the R packages vegan v. 2.5–6, ggplot2 v. 3.2.1, cowplot v. 1.0.0 and ade4 v. 1.7–13.
Results
Only a limited number of isolated populations of the protected Cladonia subgen. Cladina species (15 of C. arbuscula, 36 of C. mitis and 13 of C. rangiferina) were found in the Balaton Uplands region, mostly on hilltops with acidic underlying rock (Fig. 3). All former localities of the three Cladonia species in the Balaton Uplands were checked during fieldwork taking place between 2016 and 2019; 11 of the 20 previously described populations were successfully confirmed, with the addition of 23 new records. Detailed distribution data were published in Sinigla et al. (Reference Sinigla, Lőkös and Farkas2019).

Fig. 3. Distribution of the three Cladonia species examined in this study and the types of underlying rock with which they are associated in the Balaton Uplands, Hungary. Triangle = C. arbuscula, circle = C. mitis, star = C. rangiferina. Out of 20 previously described populations, 11 were confirmed, 9 were not found and 23 new records were established. In colour online.
Altogether 154 species (42 lichens, 97 vascular plants, 15 bryophytes) were recorded in the 46 quadrats (see Supplementary Material Table S1, available online). The abundance of the three Cladonia species in the quadrats was as follows: 5 quadrats with only C. arbuscula, 22 with only C. mitis, 4 with only C. rangiferina, 5 with C. arbuscula and C. mitis, 5 with C. mitis and C. rangiferina, and 4 with all three Cladonia species. Table 1 summarises the range of environmental variables associated with each of the three species of Cladonia.
Table 1. A summary of the range, mean and standard deviation of the environmental variables measured in association with three species of rare and protected lichens, Cladonia arbuscula, C. mitis and C. rangiferina, found in the Balaton Uplands of Hungary. n = number of quadrats in which each species was recorded. See ‘Material and Methods’ for estimation of disturbance.
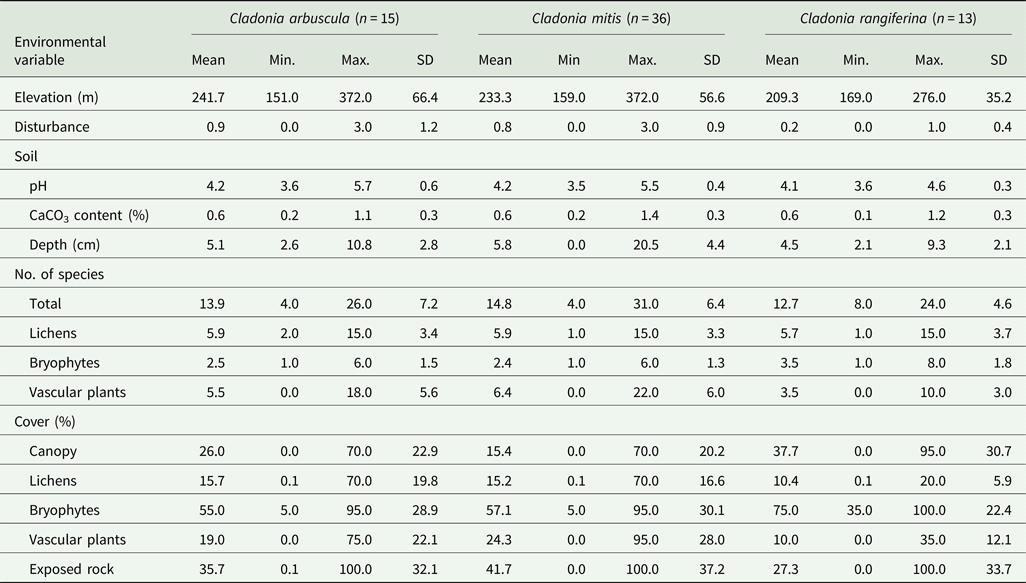
The PCA of species percentage cover separated the quadrats of these three Cladonia species moderately well (Fig. 4). The C. mitis plots can be found in the entire ordination space, and those of C. arbuscula and C. rangiferina are scattered. In the case of C. rangiferina, the quadrats (black symbols) are more-or-less concentrated in the upper part of the ordination diagram, which emphasizes its narrower ecological range. The species occurring together with the Cladonia species are concentrated around the 0.0 point, and are mainly the three bryophyte species, Dicranum scoparium, Hypnum cupressiforme and Polytrichum piliferum which separate the quadrats. The point-concentrations can be detected near to the three most frequent bryophyte species, but these are independent from the occurrence of Cladonia species. However, C. rangiferina rarely occurs together with P. piliferum, but is associated mainly with places dominated by D. scoparium and H. cupressiforme.

Fig. 4. Principal Component Analysis based on species composition data. Analysis was performed on a Hellinger transformed dataset, and species with a minimum of five occurrences in quadrats were included excluding the three Cladonia species under investigation. Encircling lines: thin = quadrats containing C. arbuscula; dashed = quadrats containing C. mitis; thick = quadrats containing C. rangiferina. Presence in quadrats is designated as follows: grey squares = C. arbuscula alone; open circles = C. mitis alone; triangles = C. rangiferina alone; grey closed circles = both C. arbuscula and C. mitis present; black closed circles = both C. mitis and C. rangiferina present; asterisk = all three Cladonia species present. Vascular plants, lichens and bryophytes are listed in Supplementary Material Table S1 (available online). POLFOR = Polytrichum formosum, CLAFUR = Cladonia furcata, VISVUL = Viscaria vulgaris, AGRCAP = Agrostis capillaris, CANVIT = Candelariella vitellina, ANTODO = Anthoxanthum odoratum, CERPUR = Ceratodon purpureus, CALVUL = Calluna vulgaris, POLPIL = Polytrichum piliferum, DICSCO = Dicranum scoparium, HYPCUP = Hypnum cupressiforme. In colour online.
The PCA of environmental variables (Fig. 5) showed a more explicit separation between Cladonia species. Cladonia mitis (circle and star symbols) sampled in the Balaton Uplands can be found in the whole ordination space. Places with a deeper soil layer or with more vascular plants seem to be suitable for C. mitis, and quadrats which contain C. rangiferina are located in relatively delimited places, emphasizing that C. rangiferina has a somewhat narrower ecological range than the other two species. Cladonia rangiferina grows in places where the canopy and bryophyte cover are greater, there is less vascular plant and lichen cover, the soil is thinner, and the pH is lower. The quadrats with C. arbuscula did not form a dense group, although they are concentrated in the narrowest part of the ordination diagram. Cladonia arbuscula grows mainly in rocky places with few vascular plants and a higher cover of bryophytes or lichens.

Fig. 5. Principal Component Analysis based on quantitative environmental variables. Encircling lines: thin = quadrats containing C. arbuscula; dashed = quadrats containing C. mitis; thick = quadrats containing C. rangiferina. Presence in quadrats is designated as follows: grey squares = C. arbuscula alone; open circles = C. mitis alone; triangles = C. rangiferina alone; grey closed circles = both C. arbuscula and C. mitis present; black closed circles = both C. mitis and C. rangiferina present; asterisk = all three Cladonia species present. For % cover, BRY = bryophyte, CANOPY = canopy, LYC = lichen, ROCK = exposed rock, VASC = vascular plants. For soil, CaCO3 = CaCO3 content, SOIL = soil depth and pH = pH. In colour online.
Although there were no significant differences between occupied and unoccupied quadrats for underlying rock type, vegetation type and disturbance and only a significant difference for C. mitis for aspect (Table 2), there are a number of interesting observations to be made. All three species grow on red sandstone; more than 75% of the quadrats were sampled on this underlying rock type. Cladonia rangiferina can also be found on gravel, C. arbuscula on gravel and basalt, and C. mitis on all four underlying rock types (Fig. 6A). The aspect of the sampling points showed some differences between species but most occurred in hilltop positions (Fig. 6B). In addition, they were found most frequently in closed acidofrequent oak forests. Cladonia rangiferina was found in the fewest vegetation types, C. mitis grew in the most diverse range of habitats (Fig. 6C) and all species were found mostly in undisturbed or slightly disturbed places (Fig. 6D). Cladonia rangiferina was the most sensitive to disturbance, with more than 75% of samples growing in undisturbed locations, and it was absent from medium or strongly disturbed plots. Both C. mitis and C. arbuscula can occur in more disturbed habitats. In undisturbed habitats, greater numbers of well-developed Cladonia thalli can be found but with increasing disturbance, the number of, and area covered by well-developed and fragmented thalli of C. mitis tend to decline (Fig. 7).
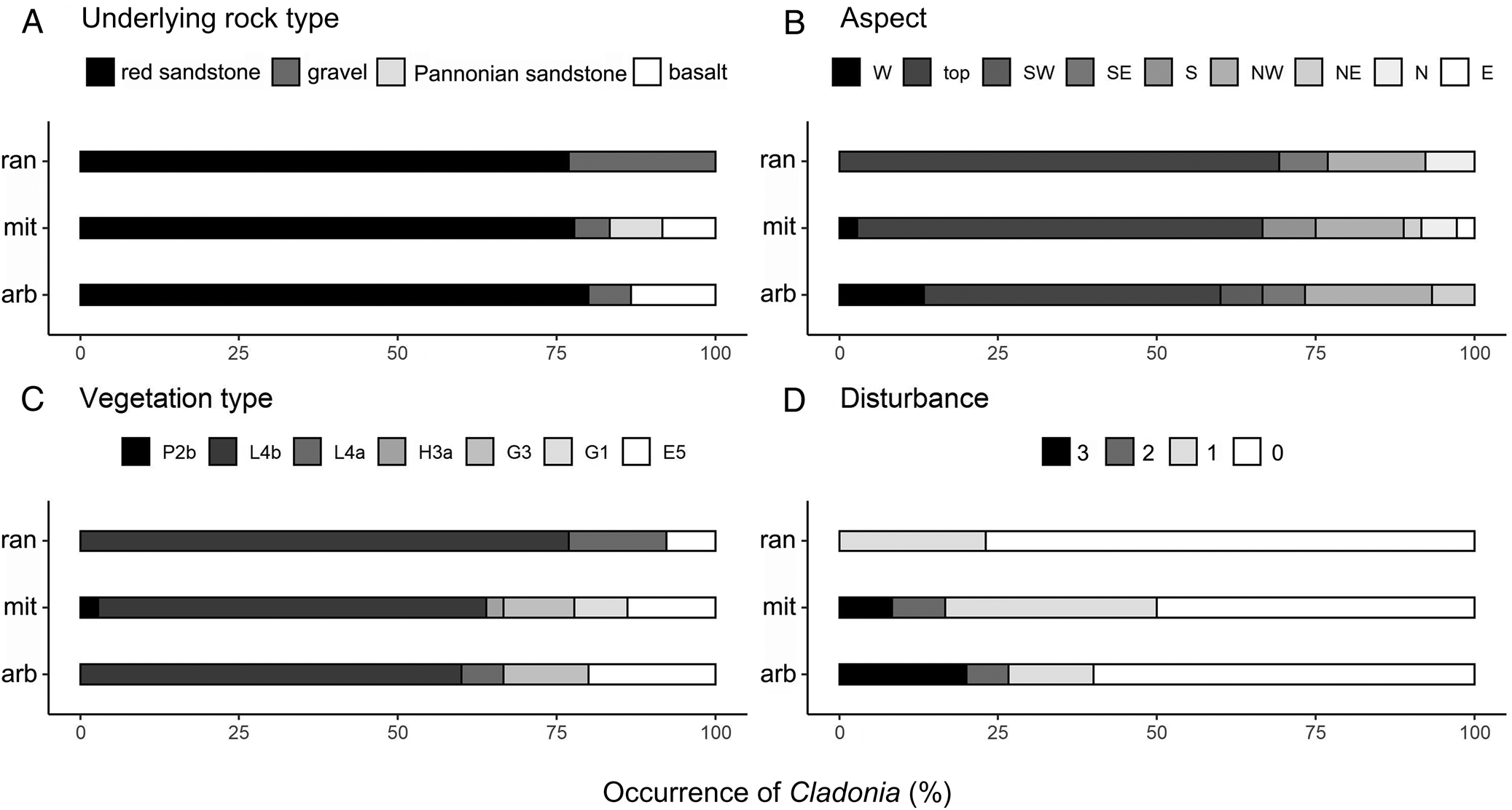
Fig. 6. A comparison of the % occurrence of three protected species of Cladonia in 46 quadrats sampled in the Balaton Uplands of Hungary. A, underlying rock. B, aspect. C, vegetation type. D, disturbance. arb = C. arbuscula, mit = C. mitis, ran = C. rangiferina. For the measurement of these variables see ‘Material and Methods’.

Fig. 7. The effect of disturbance on the number and area covered by both well-developed and fragmented thalli of three Cladonia species in the Balaton Uplands of Hungary. Cladonia arbuscula = dark grey, C. mitis = mid grey, C. rangiferina = light grey. For the disturbance scale see ‘Material and Methods’. Upper and lower lines of the boxes represent the upper and lower quartiles and the middle line indicates the median. The ends of the whiskers indicate the maximum and minimum values, dots are the outliers.
Table 2. A comparison of the differences in environmental variables between occupied and unoccupied quadrats for three species of Cladonia; C. arbuscula, C. mitis and C. rangiferina. A Mann-Whitney test was used for canopy cover, pH, elevation, soil CaCO3 content and depth, total number and cover of vascular plants, bryophytes and lichens, and the Fisher exact test for underlying rock type, aspect, vegetation type and disturbance. See ‘Material and Method’ for values attributed to these variables. *** = P < 0.001, ** = P < 0.01, * = P < 0.05, ns = not significant.

There was no significant difference between occupied and unoccupied quadrats in terms of pH, elevation, soil depth or CaCO3 content (Table 2). However, C. rangiferina occurred at lower mean elevations than either C. arbuscula or C. mitis. (Supplementary Material Fig. S1, available online; Table 1). In addition, quadrats containing C. rangiferina had a slightly lower mean pH value than the other two species. (Supplementary Material Fig. S1; Tables 1 & 2). Quadrats containing C. mitis had a wider range of soil depth, 0 cm to 20.5 cm, than those containing C. arbuscula or C. rangiferina, 2.1 cm and 10.8 cm, although the mean values for soil depth were similar.
The numbers of lichen, bryophyte and vascular plant species varied little between occupied and unoccupied quadrats for all three species except for quadrats containing C. rangiferina, where there was a significant difference between quadrats both for bryophyte and vascular plant numbers, with remarkably fewer species in the occupied quadrats (Supplementary Material Fig. S2, available online; Tables 1 & 2). In addition, there was a significant difference in the number of lichen species for C. mitis, and the number of lichen-containing microquadrats for C. arbuscula between occupied and unoccupied quadrats (Supplementary Material Fig. S2; Tables 1 & 2).
There were no significant differences in the cover of lichens, vascular plants and exposed rock between occupied and unoccupied quadrats for all three species (Supplementary Material Fig. S3, available online; Table 2). However, there was a significant difference in bryophyte cover for C. rangiferina with it being slightly higher in the occupied quadrats (Supplementary Material Fig. S3). Only canopy cover showed a significant difference between occupied and unoccupied quadrats for all three species of Cladonia (Table 2): C. rangiferina grows beneath higher canopy cover (mean = 38%) than C. arbuscula (mean = 26%) and C. mitis (mean = 15%). Quadrats of C. arbuscula and C. mitis showed the same minimum (0%) and maximum (70%) values, but those of C. rangiferina were higher at 95%.
The number of fragmented and well-developed thalli as well as the area covered by fragmented and well-developed thalli, were significantly different between occupied and unoccupied quadrats for C. arbuscula and were consistently lower than for the other two species (Supplementary Material Fig. S4, available online; Table 2).
Among the saxicolous lichen species in the study area, Candelariella vitellina (Hoffm.) Müll. Arg., Circinaria caesiocinerea (Nyl. ex Malbr.) A. Nordin et al., Protoparmeliopsis muralis (Schreb.) M. Choisy, Rhizocarpon distinctum Th. Fr., Xanthoparmelia conspersa (Ehrh. ex Ach.) Hale, X. pulla (Ach.) O. Blanco et al. and X. stenophylla (Ach.) Ahti & D. Hawksw. occurred together with these Cladonia species the most frequently, and the most common accompanying terricolous lichen species were C. chlorophaea (Flörke ex Sommerf.) Spreng., C. foliacea (Huds.) Willd., C. furcata (Huds.) Schrad., C. gracilis (L.) Willd., C. macilenta Hoffm., C. rangiformis Hoffm. and C. uncialis (L.) Weber ex F.H. Wigg. Vascular plants which were recorded in 10 or more quadrats were Anthoxanthum odoratum, Calluna vulgaris, Deschampsia flexuosa, Hieracium bauhinii, Jasione montana, Luzula campestris, Polygonatum officinale and Rumex acetosella, and the most frequent bryophytes were Dicranum scoparium, Hypnum cupressiforme, Polytrichum piliferum and P. formosum. Two protected plants, Carex fritschii and Leucobryum glaucum, were also recorded in the quadrats (Supplementary Material Table S1).
Discussion
This study supports the conclusion that Cladonia rangiferina is a good indicator species of its natural habitats in Hungary due to its narrow distribution area and its narrower ecological tolerances. Cladonia mitis and C. arbuscula have wider ranges of occurrence than C. rangiferina in Hungary. In succession and recolonization studies by previous authors, it has also been shown that among reindeer lichens, C. mitis is the first colonizer, then C. arbuscula and finally C. rangiferina (cf. Kershaw Reference Kershaw1977; Ahti & Oksanen Reference Ahti and Oksanen1990; Roturier et al. Reference Roturier, Bäcklund, Sundén and Bergsten2007). Furthermore, C. arbuscula and C. mitis occur in similar habitats, but C. arbuscula usually exists within a narrower range of environmental variables than C. mitis. Due to their greater genetic variability, C. arbuscula and C. mitis might have an adaptive advantage over C. rangiferina (Athukorala et al. Reference Athukorala, Doering and Piercey-Normore2015). Although our studied lichens originate from different locations, our results justify differences between these species in respect of their characteristic habitat features. It should also be noted that in terms of the world distribution of these species (GBIF 2020), the populations in our study area grow near to the southern edge of their European distribution and at a relatively low range of elevation (150–400 m).
Cladonia rangiferina occurs in the cooler submontane to cooler mountain areas (value 3 altitudinal belt) (Dingová Košuthová & Šibík Reference Dingová Košuthová and Šibík2013) where it is found in undisturbed (Fig. 6) and closed habitats (Supplementary Material Fig. S3, available online). It prefers a canopy cover > 30% (Supplementary Material Fig. S3) which is higher than both C. arbuscula and C. mitis, although this difference is not significant (cf. Dingová Košuthová & Šibík Reference Dingová Košuthová and Šibík2013). Based on our study, C. rangiferina grows in closed acidofrequent oak forest with a maximum 95% canopy cover. In heavily trampled and grazed areas (disturbance value 3), Cladonia thalli were frequently fragmented and large, well-developed thalli were absent. Cladonia rangiferina was also different from the other species in this respect, since it was not found in areas with medium to strong disturbance (disturbance value between 2 and 3). Thalli of C. rangiferina had the largest area covered by well-developed thalli among the three Cladonia species (Supplementary Material Fig. S4, available online); this relates to its greater ability to increase its cover when the lichen volume increases during succession, which also promotes its reproductive capacity and the successful recovery of its stands at disturbed sites (cf. Kiss Reference Kiss1985; Oksanen Reference Oksanen1986; Webb Reference Webb1998). The slightly more acidic pH values of soils where C. rangiferina grows is difficult to compare with the scattered values found in the literature (e.g. pH 3.9–7.2 in Bohemia (Rypáček Reference Rypáček1936), pH 2.6–5.7 in Germany (Mattick Reference Mattick1932)); our data show a narrower range for this species (pH 3.6–4.6). Some of the significant relationships between soil characteristics and secondary metabolites revealed by Zraik et al. (Reference Zraik, Booth and Piercey-Normore2018) in Manitoba (Canada) include correlations of atranorin with pH, organic matter and sand content, fumarprotocetraric acid with organic matter and sand content, and usnic acid with pH and organic matter. According to these results, C. rangiferina, by producing atranorin, should occur on less acidic soil, while we found it in relatively more acidic habitats (pH 3.6–4.6) compared to the other two investigated species. This contradiction is explained if we consider that the investigated range of pH was 5.0–7.0 in Canada and 3.5–5.7 in our study, and also compare solar and UV radiation data which are much more important drivers than soil pH for the production of cortical pigments (e.g. Solhaug et al. Reference Solhaug, Gauslaa, Nybakken and Bilger2003; Nybakken et al. Reference Nybakken, Solhaug, Bilger and Gauslaa2004; Nybakken & Julkunen-Tiitto Reference Nybakken and Julkunen-Tiitto2006; McEvoy et al. Reference McEvoy, Solhaug and Gauslaa2007; Nguyen et al. Reference Nguyen, Chollet-Krugler, Gouault and Tomasi2013). The mean bryophyte cover of C. rangiferina quadrats showed the highest value of 75%, though these quadrats contained only two or three moss species (e.g. Dicranum scoparium, Hypnum cupressiforme) (Supplementary Material Figs S2 & S3).
Cladonia arbuscula and C. mitis are circumpolar species in boreal-subarctic-subalpine regions that prefer similar habitat and abiotic factors. According to Dingová Košuthová & Šibík (Reference Dingová Košuthová and Šibík2013), C. arbuscula and C. mitis occur in submontane to colline areas (value 4 altitudinal belt) in the Western Carpathians.
The quantity of light (cf. canopy cover) is a key environmental factor determining the distribution of Cladonia species (Dingová Košuthová et al. Reference Dingová Košuthová, Svitková, Pišút, Senko and Valachovic2013). This is supported by our study, since most Cladonia records occur in areas under a canopy cover below 40% (Supplementary Material Fig. S3). Of the variables studied, only canopy cover showed significant differences between occupied and unoccupied quadrats in all three Cladonia species (Supplementary Material Fig. S3; Fig. 5). The wider tolerance of C. mitis was indicated by its occurrence on the most diverse underlying rocks and its predominance on exposed hilltops (Fig. 6); this can also be explained by the nutrient-poor conditions due to leaching of soils and the fact that the litter layer is carried away by the wind (Dingová Košuthová et al. Reference Dingová Košuthová, Svitková, Pišút, Senko and Valachovic2013). Occurrences of the three Cladonia species are sporadic in underlying rock habitats on acidic underlying rock, where the soil layer is shallow and the vascular plant cover is below 50%. According to Dingová Košuthová & Šibík (Reference Dingová Košuthová and Šibík2013), four of the species (C. chlorophaea, C. gracilis, C. macilenta and C. uncialis) we found that were associated with the investigated species are adapted to extremely acidic soils. The pH values of soil under C. mitis are again of a somewhat narrower range (pH 3.5–5.5) than in other countries (e.g. pH 2.5–6.0 in Japan (Glime & Iwatsuki Reference Glime and Iwatsuki1990)), but slightly wider than those for C. rangiferina. The usnic acid-containing C. arbuscula and C. mitis also grow in more acidic sites (pH 3.6–5.7 and pH 3.5–5.5, respectively) in Hungary than those measured in Canada by Zraik et al. (Reference Zraik, Booth and Piercey-Normore2018). According to Heinken (Reference Heinken1999), moderate trampling can enhance the local dispersal of vegetative propagules of terricolous lichens. Both fragmented and well-developed thalli of C. arbuscula could be found at sites with weak trampling and grazing (disturbance value 1).
The most important environmental factors based on our investigation
In this study of the effect of environmental factors on the composition of these important cryptogams, the following are of particular interest: elevation and light availability (cf. canopy cover/PAR) for C. rangiferina, soil pH for C. mitis and elevation for C. arbuscula. These appear to be the most important factors among our results, but only canopy cover proved to significantly affect their distribution (cf. Tilk et al. Reference Tilk, Ots and Tullus2018). The occurrence of the three studied Cladonia species requiring an acidic substratum is naturally restricted to the rare acidic sites of the study area (cf. ‘Material and Methods’). The data on soil CaCO3 content and pH generally show opposing trends (increasing the basic or acidic nature of the substratum); in general, our acidic soil samples still contained a low percentage of CaCO3. The highest maximum value for soil CaCO3 content (1.4%) was found at C. mitis sites. The Hungarian lowland areas have a mainly basic character (4.7% CaCO3; Várallyay Reference Várallyay, Szujkóné-Lacza and Kováts1993) and therefore the studied species occur only exceptionally there (Lőkös & Verseghy Reference Lőkös, Verseghy, Lőkös and Rajczy2001; Aszalósné-Balogh et al. Reference Aszalósné-Balogh, Matus, Lőkös, Varga and Farkas2020).
Nature conservation
Species with a restricted distribution range and low abundance are the most vulnerable to extinction (Johansson Reference Johansson2006). The maintenance of threatened and protected lichens depends on good management practices based on their distribution and habitat preferences. A total of 418 lichen species are listed in the first and only Hungarian red list of lichens (Lőkös & Tóth Reference Lőkös, Tóth, Tóth and Horváth1997), and currently 17 species are protected by Hungarian National Law (83/2013 (IX.25) VM decree).
In the Balaton Uplands, the three Cladonia species investigated here are endangered because of their low abundance and narrow distribution range, and owing to vehicle (off-road, motocross), human and game trampling. Today, human disturbance has a significant impact worldwide in addition to natural disturbance (Burton et al. Reference Burton, Bergeron, Bogdanski, Juday, Kuuluvainen, McAfee, Ogden, Teplyakov, Alfaro, Francis, Mery, Katlia, Galloway, Alfaro, Kanninen, Lobovkov and Varjo2010). Generally, logging is considered to have a negative effect on terricolous lichens due to the changed light conditions and increased exposure of underlying mineral soils (Lesica & Shelley Reference Lesica and Shelley1992; Frisvoll & Presto Reference Frisvoll and Presto1997; Miège et al. Reference Miège, Goward, Waterhouse and Armleder2001; Waterhouse et al. Reference Waterhouse, Armleder and Nemec2011). However, Roturier et al. (Reference Roturier, Bäcklund, Sundén and Bergsten2007) have the opposite opinion: after logging, the lichen cover is significantly higher on the raw mineral soil surface due to improved insolation; this view is supported by our present results. In the vast majority of cases, the selected sampling sites were located on mountain tops, in small abandoned red sandstone quarries. Cladonia subgen. Cladina species are bound to rough soil surfaces, where acidic underlying rock is found on or near the soil surface. Lichen thalli are often flipped over, destroyed and buried due to the mechanical effect of trampling. Using methods similar to those applied by Roturier et al. (Reference Roturier, Bäcklund, Sundén and Bergsten2007), artificial dispersal of fragmented thalli of these three Cladonia species could be suggested in the Balaton Uplands in the future. Furthermore, excluding game from the Cladonia habitats is possibly an additional solution to protect these populations.
Conclusions
In several aspects the comparison of habitat features did not result in significant differences, but smaller differences were found in the requirements of C. arbuscula and C. mitis. The amount of light (cf. canopy cover) is a key environmental factor for the distribution of Cladonia subgen. Cladina species. Cladonia rangiferina was found to be more sensitive to disturbance than the other species, and also its distribution range is narrower. Its low ecological tolerance therefore makes it a good indicator species of natural habitats in Hungary. Our findings contribute to ecological and conservation biological analyses of similar habitats in nearby geographical regions, and also to wide-scale prediction studies.
The current results regarding the distribution and ecological requirements of these three Natura 2000 species of Cladonia represent a useful contribution towards further studies for establishing effective conservation methods. Creating exclusion zones to all forms of trampling and other disturbance both for present and possible future colonization sites could be followed by artificial dispersal of Cladonia thalli.
Acknowledgements
We thank the Institute of Environmental and Earth Sciences, University of Sopron for carrying out the CaCO3 content measurements of the soil samples. The map in Fig. 3 followed the database AGROTOPO (by the Institute for Soil Sciences and Agricultural Chemistry, Centre for Agricultural Research). We are grateful to Dr Arne Thell for his comments and to Professor Mark Seaward for advice and revision of the English text, and also to our anonymous reviewers for their careful work and valuable suggestions. The work is supported by the National Research Development and Innovation Fund NKFI K 124341.
Author ORCIDs
Mónika Sinigla, 0000-0003-4366-9292; Erzsébet Szurdoki, 0000-0003-4595-9090; László Lőkös, 0000-0001-5301-6426; Dénes Bartha, 0000-0001-7106-7614; István Galambos, 0000-0002-3877-850X; András Bidló, 0000-0002-7601-0710; Edit Farkas, 0000-0002-5245-1079.
Supplementary Material
To view Supplementary Material for this article, please visit https://doi.org/10.1017/S0024282921000165.