1. Introduction
Given that the overarching aim of modern neuroscience is to elucidate the biological bases of our very own minds, it is hardly surprising that in recent years many of the field’s most important advances have been presented to the general public through popular newspapers, magazines, television shows, and websites. This growing media attention has certainly increased people’s awareness and appreciation of brain research, but it has also led to an unwarranted glorification of all things ‘neuro’. One reason for this extreme reaction is that when the key findings get filtered through the prismatic perspectives of journalists, pundits, and other non-experts, their nature often becomes oversimplified and their significance exaggerated. Another reason, though, is that even experts are not always successful at resisting the temptation to overinterpret exciting new discoveries, especially when those discoveries appear to have profound implications, as they sometimes do. Due to both of these factors, a strong backlash is now underway, as exemplified by books such as Paolo Legrenzi and Carlo Umiltà’s Neuromania: on the limits of brain science (2011), Sally Satel and Scott Lilienfeld’s Brainwashed: on the seductive appeal of mindless neuroscience (2013), and Raymond Tallis’s Aping mankind: neuromania, Darwinitis, and the misrepresentation of humanity (2014).
One of the latest installments in this genre, Gregory Hickok’s The myth of mirror neurons: the real neuroscience of communication and cognition (2014), is particularly noteworthy because it focuses on what has been called “the most hyped concept in neuroscience” (Jarrett, Reference Jarrett2012). Mirror neurons were first discovered in 1992 by Giacomo Rizzolatti and his colleagues in Parma, Italy (di Pellegrino, Fadiga, Fogassi, Gallese, & Rizzolatti, 1992; for reviews see Kilner & Lemon, Reference Kilner and Lemon2013; Rizzolatti, Catttaneo, Fabbri-Destro, & Rozzi, Reference Rizzolatti, Cattaneo, Fabbri-Destro and Rozzi2014). Basically, the distinguishing property of these cells is that they discharge not only when an individual performs a specific type of action (e.g., a thumb–forefinger precision grip), but also when that individual sees someone else perform an identical or similar type of action. To be sure, such firing patterns are quite intriguing, since they suggest that action observation is closely related to action execution. During the past few decades, however, mirror neurons have attracted so much attention from both scientists and laypeople that they have been claimed to play critical roles in an ever-expanding array of psychological phenomena, ranging from more or less plausible domains like action understanding, language, and mind-reading, to increasingly far-fetched ones like political attitudes, mass hysteria, and sexual orientation.
Hickok’s primary aim is to expose what he believes to be a number of fundamental problems with mirror neuron theorizing. He doesn’t waste much time with the obviously eccentric interpretations, but instead devotes most of his book to attacking the core hypothesis – associated most prominently with Rizzolatti’s team – that mirror neurons allow people to comprehend each other’s behavior at a deep level by unconsciously simulating the observed movements in their own motor systems. As he indicates in the Preface, during the late 2000s, when everyone seemed to be jumping on the mirror neuron bandwagon, he took it upon himself to become more familiar with the relevant literature and ended up thinking that the conclusions simply weren’t supported by the data. He expressed this opinion as follows in his blog, Talking Brains: “We all agree that mirror neurons are very interesting neural creatures, but the idea that they are the basis for action understanding is darn near incoherent.” Then he wrote a critique called “Eight problems for the mirror neuron theory of action understanding in monkeys and humans”, and it not only became the most downloaded paper from the Journal of Cognitive Neuroscience, but served as the springboard for the book.
My goal here is not to review Hickok’s whole book, but rather to evaluate his major criticisms of the action understanding account of mirror neurons. I first discuss how these issues pertain to the observation of other people’s behavior, and then I consider how they bear on two aspects of language processing – namely, comprehending linguistically represented actions, and perceiving speech. Before delving into the details, however, a few preliminary remarks are in order about my general strategy.
At a critical juncture in Chapter 7 (pp. 180–181), Hickok makes the following point: “‘Understanding’ is a complicated thing with lots of moving parts. You can’t pull out one part and call that the ‘basis of action understanding.’ It doesn’t work for just the motor bits and it doesn’t work for just the sensory bits.” I completely agree (Kemmerer, Gonzalez-Castillo, Talavage, Patterson, & Wiley, Reference Kemmerer, Gonzalez-Castillo, Talavage, Patterson and Wiley2008; Kemmerer, Rudrauf, Manzel, & Tranel, Reference Kemmerer, Rudrauf, Manzel and Tranel2012), and so do many other researchers (e.g., Barsalou, Reference Barsalou2013; Damasio & Meyer, Reference Damasio and Meyer2008; Spunt & Lieberman, Reference Spunt and Lieberman2012; Van Overwalle & Baetens, Reference Van Overwalle and Baetens2009). But Hickok goes on to make another claim: “The motor part, while associated with the action for sure, is such a minute part of the bigger picture as to be virtually useless on its own. At the very most, one could possibly argue that accessing a motor copy of the perceived action could help facilitate or speed access to the broader, nonmotor network that enables understanding.” Similar attempts to minimize the functional contribution of the mirror system to action understanding appear in several other chapters, too, but my sense is that most of these efforts are misguided. In fact, my main concern about Hickok’s book is that in his zeal to undermine the overly strong view that mirror neurons are the sole ‘basis’ of action understanding, he veers too far in the opposite direction, denying that they play any significant role at all in that process. As I demonstrate below, although many problems remain unresolved, a substantial amount of evidence does support the notion that the motor cortices of the human brain make non-trivial contributions to both the perception and the interpretation of other people’s actions, not only when those actions are directly observed, but also when they are conveyed by speech.
2. Observing other people’s actions
At the beginning of Chapter 3, Hickok acknowledges that “it is a virtual given that humans have mirror neurons” (p. 27). But then he spends the rest of the chapter painting a mostly negative picture of the work that has been done during the past twenty years to delineate the mirror system in the human brain. For instance, he cites Grèzes and Decety’s (Reference Grèzes and Decety2001) meta-analysis of the first wave of functional neuroimaging studies (i.e., studies employing either positron emission tomography [PET] or functional magnetic resonance imaging [fMRI]) to justify the statement that “if you looked across many studies you could find evidence for Broca’s area activating during observation in one study and during execution in another, but if you were to plot the precise location of these studies, as a 2001 study did, very little direct overlap was evident in the human homologue of monkey area F5, the core of the mirror system” (pp. 36–37, emphasis in original). However, neither here nor in any other part of the book does Hickok mention that as the field continued to evolve, further meta-analyses were conducted that not only included a larger number of experiments but also employed more sophisticated methods, and all of them did reveal that both action execution and action observation tend to engage the posterior part of Broca’s area as well several other regions, most notably the premotor cortex and anterior intraparietal sulcus (see Figure 1; Van Overwalle & Baetens, Reference Van Overwalle and Baetens2009; Molenberghs, Cunnington, & Mattingley, Reference Molenberghs, Cunnington and Mattingley2012; see also Caspers, Zilles, Laird, & Eikhoff, Reference Caspers, Zilles, Laird and Eickhoff2010).

Fig. 1. Activation peaks from fMRI studies of the mirror system, plotted according to Talairach y-z coordinates. The posterior superior temporal sulcus (pSTS) is engaged during the visual perception of whole-body motion (green circles) and gaze direction (yellow circles), whereas the premotor cortex (PMC) and anterior intraparietal sulcus (aIPS) – which constitute what are traditionally regarded as the two main components of the mirror system – are recruited during the execution of hand actions (dark blue circles), the imitation of hand actions (light blue circles), the observation of hand actions and, in a few studies, mouth or foot actions (green diamonds), and the understanding of the immediate goals of perceived actions (orange squares). A = left hemisphere; B = right hemisphere; horizontal y axis = anterior–posterior dimension; vertical z axis = superior–inferior dimension (from Van Overwalle & Baetens, Reference Van Overwalle and Baetens2009, p. 576; reproduced with permission).
In Chapters 4 and 8, Hickok advocates the view that mirror neurons do not significantly enhance action understanding per se, but instead play a role in action selection by virtue of participating in sensory-motor mappings (see also Cook, Bird, Catmur, Press, & Heyes, Reference Cook, Bird, Catmur, Press and Heyes2014). To support this purely associative position, he summarizes a study by Catmur, Walsh, and Heyes (Reference Catmur, Walsh and Heyes2007) that used transcranial magnetic stimulation (TMS) to show that it is possible to create ‘counter mirror neurons’. The investigators first demonstrated that, in keeping with previous research, when the subjects watched someone else move either an index finger or a pinky finger, the motor excitability of their own corresponding finger increased. Then they trained the subjects to respond to the sight of an index finger movement by making a pinky finger movement, and to respond to the sight of a pinky finger movement by making an index finger movement. Afterward, they found that when the subjects saw an index finger movement, the motor excitability of their own pinky finger increased, and when they saw a pinky finger movement, the motor excitability of their own index finger increased. Hickok argues that these results challenge the mirror neuron theory of action understanding because “if you can alter the motor response without affecting perception, then the motor response can’t be the basis of the percept” (p. 54). This is not really a valid conclusion, however, because other studies have shown that learning arbitrary visuomotor rules does not eliminate normal mirror responses; on the contrary, normal mirror responses still occur in an early time window (150–250 ms post stimulus onset), whereas trained ‘counter mirror’ responses do not occur until a late time window (300–320 ms post stimulus onset) (Barchiesi & Cattaneo, Reference Barchiesi and Cattaneo2013; Reference Ubaldi, Barchiesi and CattaneoUbaldi, Barchiesi, & Cattaneo, in press). These findings therefore leave open the possibility that the mirror system does contribute to the perception and interpretation of actions, and that possibility has received increasing support from numerous TMS studies (Figure 2) and lesion studies (Figure 3).
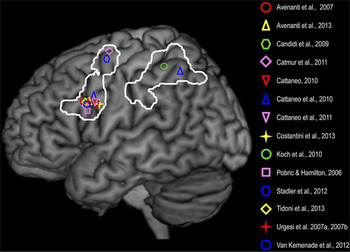
Fig. 2. Frontal and parietal sites where TMS causally affected various aspects of the perception and understanding of observed actions. In the study by Catmur et al. (Reference Catmur, Walsh and Heyes2011), the ventral and dorsal frontal sites were stimulated in the right hemisphere but are represented here on the left hemisphere. The white lines indicate the frontal and parietal nodes of the ‘action observation network’ that is based on Caspers et al.’s (Reference Caspers, Zilles, Laird and Eickhoff2010) meta-analysis of fMRI studies. Complete references to the TMS studies cited here can be found in Avenanti, Candidi, and Urgesi (Reference Avenanti, Candidi and Urgesi2013). (from Avenanti et al., Reference Avenanti, Candidi and Urgesi2013, p. 3; reproduced with permission).
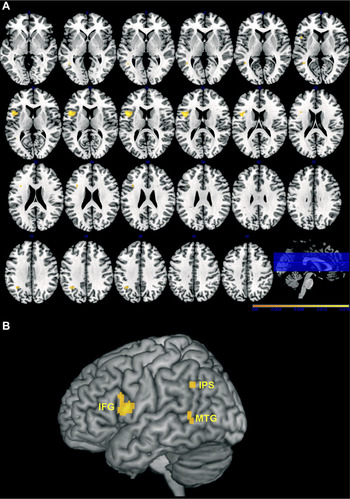
Fig. 3. Clusters of voxels where significant associations were found between lesion sites and disorders involving various aspects of the perception and understanding of observed actions. The results are based on an anatomic likelihood estimation meta-analysis of 11 lesion-symptom mapping studies encompassing 361 brain-damaged patients. (A) Axial slices, where the left hemisphere is on the left. (B) 3D rendering of the Montreal Neurological Institute (MNI) template. Note that deeper regions, especially for the IPS, are projected onto the surface of the template. MTG = middle temporal gyrus; IFG = inferior frontal gyrus; IPS = intraparietal sulcus (from Urgesi et al., Reference Urgesi, Candidi and Avenanti2014, p. 9; reproduced with permission).
Starting with perception, in Chapter 7 Hickok correctly notes that the observation of biological motion patterns recruits the posterior superior temporal sulcus (pSTS; Figure 1), but he neglects to mention that it also depends on the inferior frontal cortex (IFC, including specifically the posterior part of Broca’s area and the ventral premotor cortex). Evidence comes from research showing that when subjects are asked to detect point-light walkers amid noisy backgrounds, not only does their performance improve as the amount of gray matter increases in both the pSTS and the IFC (Gilaie-Dotan, Kanai, Bahrami, Rees, & Saygin, Reference Gilaie-Dotan, Kanai, Bahrami, Rees and Saygin2013), but their performance is also disrupted by TMS (van Kemenade, Muggleton, Walsh, & Saygin, Reference van Kemenade, Muggleton, Walsh and Saygin2012) or structural damage (Saygin, Reference Saygin2007) to either region. In addition, the ability to discriminate between pictures of slightly different actions is impaired by TMS (Urgesi, Calvo-Merino, Haggard, & Aglioti, Reference Urgesi, Calvo-Merino, Haggard and Aglioti2007a; Urgesi, Candidi, Ionta, & Aglioti, Reference Urgesi, Candidi, Ionta and Aglioti2007b; Reference Jacquet and AvenantiJacquet & Avenanti, in press) or structural damage (Moro, Urgesi, Pernigo, Lanteri, Pazzaglia, & Aglioti, Reference Moro, Urgesi, Pernigo, Lanteri, Pazzaglia and Aglioti2008) to the IFC. And when subjects watch an actor lift boxes of different weights, TMS to the IFC interferes with their capacity to estimate the weights of the boxes (Pobric & Hamilton, Reference Pobric and Hamilton2006) and to judge whether the actor is trying to deceive them about those weights (Tidoni, Borgomaneri, di Pellegrino, & Avenanti, Reference Tidoni, Borgomaneri, di Pellegrino and Avenanti2013). Yet another remarkable discovery is that a brain-damaged patient whose lesion affected the left inferior, middle, and superior frontal gyri violated Fitts’s law (Fitts, Reference Fitts1954) during both the execution and the observation of actions (Eskenazi, Grosjean, Humphreys, & Knoblich, Reference Eskenazi, Grosjean, Humphreys and Knoblich2009). This law is perhaps the most well-established principle of motor control, and it basically captures speed–accuracy trade-offs, stating that “the average time it takes to move between two targets is determined by the width of the targets and the distance separating them” (Eskenazi et al., Reference Eskenazi, Grosjean, Humphreys and Knoblich2009, p. 479). Hickok does not discuss any of these findings, but all of them (among others) bolster the idea that the IFC, which is a major component of the mirror system, plays a genuinely causal role in the perception of actions. It may use unconscious motor simulations in a predictive manner to constrain the perceptual processing of observed actions, or it may use them to construct more complete action representations from partial, subtle, or ambiguous visual cues (Kilner, Friston, & Frith, Reference Kilner, Friston and Frith2007; Urgesi, Candidi, & Avenanti, Reference Urgesi, Candidi and Avenanti2014; Wilson & Knoblich, Reference Wilson and Knoblich2005). In this connection, it is also noteworthy that more superior sectors of the premotor and primary motor cortices are engaged in a roughly somatotopic manner during the observation of body-part-specific movements (Buccino et al., Reference Buccino, Binkofski, Fink, Fadiga, Fogassi, Gallese, Seitz, Zilles, Rizzolatti and Freund2001; Filimon, Nelson, Hagler, & Sereno, Reference Filimon, Nelson, Hagler and Sereno2007; Jastorff, Begliomini, Fabbri-Destro, Rizzolatti, & Orban, Reference Jastorff, Begliomini, Fabbri-Destro, Rizzolatti and Orban2010; Sakreida, Schubotz, Wolfensteller, & von Cramon, Reference Sakreida, Schubotz, Wolfensteller and von Cramon2005; Wheaton, Thompson, Syngeniotis, Abbott, & Puce, Reference Wheaton, Thompson, Syngeniotis, Abbott and Puce2004). And while it is less clear whether these regions also facilitate perception, supportive evidence is beginning to accrue (Michael et al., Reference Michael, Sandberg, Skewes, Wolf, Blicher, Overgaard and Frith2014; Serino, De Filippo, Casavecchia, Coccia, Shiffrar, & Ladavas, Reference Serino, De Filippo, Casavecchia, Coccia, Shiffrar and Ladavas2010).
Turning to more interpretive aspects of action observation, in Chapter 7 Hickok summarizes a study by Tranel, Kemmerer, Adolphs, Damasio, and Damasio (Reference Tranel, Kemmerer, Adolphs, Damasio and Damasio2003) in which ninety brain-damaged patients with widely distributed left- or right-hemisphere lesions were given the following two tasks, both of which probe non-verbal knowledge of action concepts: (1) Picture Attribute, in which, for each item, the subject is shown two photographs of actions and must indicate which one satisfies a certain value for a single attribute (e.g., whether swinging on a swingset or sliding down a slide would be more tiring); and (2) Picture Comparison, in which, for each item, the subject is shown three photographs of actions and must indicate which one is different in kind from the other two (e.g., sewing a sock, sewing a button, or threading a needle). The researchers found that twenty-six patients failed one or both tasks, and when they contrasted the lesion sites of these impaired patients against those of the sixty-four unimpaired patients, they discovered that the greatest lesion overlap specific to the impaired patients was in the left inferior frontoparietal cortex and underlying white matter, as well as in the white matter beneath the left pSTS and posterior middle temporal gyrus (pMTG). In discussing these results, Hickok argues that while the involvement of the pSTS/pMTG does constitute evidence that this region subserves critical aspects of action concepts, the involvement of the inferior frontoparietal territory merely reflects task demands, such as having to imagine the dynamic motion properties of the statically portrayed actions, or having to draw inferences about their relationships along various dimensions. This account in terms of task demands is open to criticism, however.
First, in a later study involving seventy-eight patients, Tranel, Manzel, Asp, and Kemmerer (Reference Tranel, Manzel, Asp and Kemmerer2008) used not only static photographs but also dynamic video clips in two parallel action naming tasks that do not require as much inferential processing as the Picture Attribute and Picture Comparison tasks employed in the previous study. Importantly, they still found that impaired performance, regardless of stimulus type, was linked with a pattern of lesion sites very similar to the one reported by Tranel et al. (Reference Tranel, Kemmerer, Adolphs, Damasio and Damasio2003). Second, in another study involving 147 patients, Kemmerer et al. (Reference Kemmerer, Rudrauf, Manzel and Tranel2012) used six tasks: Picture Attribute, Picture Comparison, Static Action Naming, and three tasks requiring verb comprehension – specifically, Word–Picture Matching (in which a single verb must be matched with one of two photographs of actions), Word Attribute (analogous to Picture Attribute, only with verbs as stimuli), and Word Comparison (analogous to Picture Comparison, only with verbs as stimuli). As in the earlier experiments, the deficit-lesion correlations across tasks implicated not only the left pSTS/pMTG, but also, and more consistently, the left IFC. Third, similar results have emerged from several other lesion studies that have explored the neural underpinnings of action concepts (Figure 3). And fourth, there is converging evidence from TMS that the IFC contributes not only to the perception of observed actions (as discussed above), but also to the higher-level conceptual processing of their sequential and goal-directed properties (Cattaneo, Sandrini, & Schwarzbach, Reference Cattaneo, Sandrini and Schwarzbach2010; Clerget, Winderickx, Fadiga, & Olivier, Reference Clerget, Winderickx, Fadiga and Olivier2009; Reference Jacquet and AvenantiJacquet & Avenanti, in press). Taken together, these findings suggest that, contrary to Hickok’s view, the left inferior frontoparietal territory does not simply fulfill general task demands when actions must be recognized and categorized; instead, it appears to contribute to the actual content of the relevant concepts. More precisely, this territory may underlie some of the motor features of action concepts, while the pSTS/pMTG may underlie their visual motion features (Kemmerer et al., Reference Kemmerer, Gonzalez-Castillo, Talavage, Patterson and Wiley2008; Kemmerer, Reference Kemmerer2012, Reference Kemmerer, de Almeida and Manouilidou2014).
On the other hand, I think Hickok is right to highlight a number of neuropsychological conditions in which action production is disrupted but action understanding is apparently preserved. For example, in Chapter 4 he points out that even though some patients with limb apraxia display corresponding execution and comprehension impairments for actions involving tools, others have deficits that are restricted to execution (e.g., Halsband, Schmitt, Weyers, Binkofski, Grützner, & Freund, Reference Halsband, Schmitt, Weyers, Binkofski, Grützner and Freund2001; Negri, Rumiati, Zadini, Ukmar, Mahon, & Caramazza, Reference Negri, Rumiati, Zadini, Ukmar, Mahon and Caramazza2007; Pazzaglia, Smania, Corato, & Aglioti, Reference Pazzaglia, Smania, Corato and Aglioti2008). He also indicates in Chapter 4 that individuals with Moebius syndrome are quite good at recognizing facial expressions of emotion despite being congenitally incapable of moving the muscles that generate such expressions (Bogart & Matsumoto, Reference Bogart and Matsumoto2010). And in Chapter 6 he notes that while people who suffer from amyotrophic lateral sclerosis or cerebral palsy gradually lose much of their own motor control, they seem to retain the ability to fathom other people’s behavior, as illustrated by famous figures like the physicist Stephen Hawking and the novelist Christopher Nolan.
Hickok correctly argues that these sorts of dissociations between impaired action production and spared action understanding challenge the hypothesis that in order to make sense of other people’s movements it is absolutely necessary to simulate them in one’s own motor system. This does not entail, however, that motor simulation never adds anything meaningful to action understanding. After all, if that were the case, it would be extremely difficult, if not impossible, to account for all of the TMS and lesion data described above. Moreover, it would leave unexplained a variety of interesting results involving expertise effects.
In particular, several studies have shown that in the domains of dance (Calvo-Merino, Grèzes, Glaser, Passingham, & Haggard, Reference Calvo-Merino, Grèzes, Glaser, Passingham and Haggard2006; Cross, Hamilton, Kraemer, Kelley, & Grafton, Reference Cross, Hamilton, Kraemer, Kelley and Grafton2009a; Cross, Kraemer, De, Hamilton, Kelley, & Grafton, Reference Cross, Kraemer, De, Hamilton, Kelley and Grafton2009b) and basketball (Aglioti, Cesari, Romani, & Urgesi, Reference Aglioti, Cesari, Romani and Urgesi2008), during action observation the mirror system is modulated significantly more by motor expertise than visual expertise. In addition, a recent experiment involving soccer demonstrated that the ability to predict the outcomes of penalty kicks is disrupted by TMS to the dorsal premotor cortex in skilled players but not in novices, thereby suggesting that highly refined motor knowledge in a specific domain can causally influence one’s perceptual discrimination of actions in that domain (Reference Makris and UrgesiMakris & Urgesi, in press). Hickok addresses the topic of expertise effects in Chapter 7, but he does not consider any of the studies cited here. Instead, he proposes, in keeping with his purely associative view of the mirror system, that even though one’s motor cortex may resonate to the sight of specialized actions that one is able to perform, such responses “are not the cause of the richer understanding while watching, they are a consequence of understanding the scenario more richly, which often boils down to having more sensory experience” (p. 155, emphasis in original). It is not clear, however, how this approach can accommodate the findings just mentioned (see also Casile & Giese, Reference Casile and Giese2006).
A final issue that warrants discussion is whether the mirror system contributes to understanding the intentions that motivate other people’s actions. In Chapter 4, Hickok summarizes a frequently cited single-cell recording study by Rizzolatti’s team in which macaque monkeys both executed and observed two types of kinematically similar but intentionally different actions: first, reaching and grasping objects with the intention of placing them in a container; and second, reaching and grasping objects with the intention of eating them (Fogassi, Ferrari, Gesierich, Rozzi, Chersi, & Rizzolatti, Reference Fogassi, Ferrari, Gesierich, Rozzi, Chersi and Rizzolatti2005). The researchers found that some cells in the parietal lobe had firing patterns that appeared to distinguish between the specific intentions of the movements, regardless of whether they were executed or observed, and before the target objects were contacted by the hand. Hickok rightly points out, however, that the experimental design had a confound, because the presence/absence of the container served as a contextual cue regarding the agent’s ultimate aim, with presence signaling the intention to place the objects in the container, and absence signaling the intention to eat them. Thus, in response to the question “How did the monkey’s neurons predict the goal?”, Hickok answers “It was the nonmotoric context, not the observed movement” (p. 69, emphasis in original).
More recent research, though, supports the view that, at least in some situations, both the frontal and the parietal components of the human mirror system are sensitive to subtle kinematic features that convey information about the intentions behind observed actions (e.g., Becchio, Cavallo, Begliomini, Sartori, Feltrin, & Castiello, Reference Becchio, Cavallo, Begliomini, Sartori, Feltrin and Castiello2012; Lestou, Pollick, & Kourtzi, Reference Lestou, Pollick and Kourtzi2008; Vingerhoets, Honoré, Vandekerckhove, Nys, Vandemaele, & Achten, Reference Vingerhoets, Honoré, Vandekerckhove, Nys, Vandemaele and Achten2010; for a review see Reference Ansuini, Cavallo, Bertone and BecchioAnsuini, Cavallo, Bertone, & Bechio, in press). For example, on each trial of Becchio et al.’s (Reference Becchio, Cavallo, Begliomini, Sartori, Feltrin and Castiello2012) fMRI study, subjects first saw an object-grasping movement that had either a social intent (to compete or cooperate) or a non-social intent (to act independently), and then they judged whether a static photograph depicted a continuation of the observed action. Despite the lack of contextual cues, the observation of movements with social intent engaged the IFC and the supramarginal gyrus to a greater degree than the observation of movements with non-social intent. What’s more, relative to perceived non-social grasping, perceived social grasping recruited two components of the so-called mentalizing system – specifically, the dorsomedial prefrontal cortex and the temporoparietal junction (the other components of this network are the precuneus and the anterolateral temporal cortex). This is especially interesting because the mentalizing system has been associated with a wide range of tasks that involve explicitly, deliberately determining ‘why’ actions are performed (Schurtz, Radua, Aichhorn, Richlan, & Perner, Reference Schurz, Radua, Aichhorn, Richlan and Perner2014; Van Overwalle, Reference Van Overwalle2009). In contrast, even though the mirror system seems to be at least somewhat involved in decoding intentions, it has been linked more strongly with representing ‘how’ actions are performed (Spunt & Lieberman, Reference Spunt and Lieberman2012; Van Overwalle & Baetens, Reference Van Overwalle and Baetens2009). Although Hickok does talk briefly about the mentalizing system in Chapter 9, it is somewhat surprising that he does not directly compare the different ways in which this system and the mirror system contribute to action understanding.
3. Comprehending linguistically represented actions
I suggested above that the motor components of action concepts may be represented, in part, in motor-related frontal and parietal regions. During the past few years, this issue has received a great deal of attention because it bears directly on the neural substrates of the meanings of action verbs and sentences. But even though the literature on this topic is now fairly large, the fundamental issue remains quite controversial (for reviews and perspectives see Kemmerer & Gonzalez-Castillo, Reference Kemmerer and Gonzalez-Castillo2010; Kemmerer, Reference Kemmerer2012, Reference Kemmerer, de Almeida and Manouilidou2014, in press; Papeo, Pascual-Leone, & Caramazza, Reference Papeo, Pascual-Leone and Caramazza2013; Pulvermüller, Reference Pulvermüller2005, Reference Pulvermüller2013; Rueschemeyer & Bekkering, Reference Rueschemeyer, Bekkering, Coello and Bartolo2013; Taylor & Zwaan, Reference Taylor and Zwaan2009; Tomasino & Rumiati, Reference Tomasino and Rumiati2013). In Chapters 6 and 7, Hickok maintains that the motor features of action verbs and sentences do not depend on the precentral motor cortices, but I hope to show that many of his arguments can be challenged.
One of Hickok’s lines of attack proceeds as follows (pp. 135–136, emphasis in original): “The movements themselves, as they are coded in motor cortex, are semantically ambiguous and therefore meaningless. The very same motor program that results in lifting a pitcher and pouring liquid into a cup could mean POUR or FILL depending on whether we conceptualize the act from the perspective of the pitcher or the cup … The meanings simply aren’t in the movements, and the closer you get to motor codes for specific movements, such as primary motor cortex, the farther removed from meaning you get.”
First of all, it is certainly true that the same objective scenario can be described in different ways, but Hickok seems to be unaware of the fact that I myself have investigated how this phenomenon might be related to the mirror system (Kemmerer & Gonzalez-Castillo, Reference Kemmerer and Gonzalez-Castillo2010; see also Kemmerer, Reference Kemmerer and Arbib2006). The complete analysis is rather complex, but the short story goes roughly like this. Pour and fill are actually the names of two different subclasses of the large class of English ‘putting’ verbs (Levin, Reference Levin1993). Pour verbs (e.g., dribble, drip, drop, dump, spill) share an event structure template that can be broadly characterized as ‘X causes Y to go to Z in some manner’, whereas fill verbs (e.g., decorate, drench, encrust, saturate, shroud) share an event structure template that can be broadly characterized as ‘X causes Z to change state by means of adding Y’. It is because of these contrasting templates that the two subclasses of verbs occur in contrasting argument structure constructions: pour water into the cup versus *fill water into the cup, and *pour the cup with water vs. fill the cup with water. The two templates vary with respect to which entity is treated as the main ‘target’ of the action – the thing that changes location, or the thing that changes state – and these semantic differences regarding the relative prominence of the participants in the hierarchical goal structures of the templates correspond isomorphically to syntactic differences regarding the relative prominence of the noun phrases in the hierarchical grammatical structures of the constructions. For present purposes, what matters most is that the relevant template-level motor features of verb meaning capture high-level, differentially construable aspects of action representation that involve the goals and intentions of agents, and there is some evidence they depend on the left IFC, most notably the posterior part of Broca’s area (for details see Kemmerer & Gonzalez-Castillo, Reference Kemmerer and Gonzalez-Castillo2010; see also Kemmerer, Reference Kemmerer2012).
Another important point has to do not so much with the semantic features that are shared by all the members of a particular (sub)class of verbs, but instead with the semantic features that are unique to individual verbs. Many action verbs do not elaborate the motor components of their meanings in very much detail. For example, after surveying approximately 1,900 English action verbs involving the thematic role commonly known as ‘instrument’, Koenig, Mauner, Bienvenue, and Conklin (2008, p. 175) conclude that “verbs that require or allow instruments constrain the end states of the situations they describe more than they constrain the agent’s initial activity”. It is also the case, however, that many action verbs do specify categories of bodily movements that are defined rather narrowly (e.g., squint, nibble, pinch, kneel, tiptoe; for data on cross-linguistic diversity, see Filipovic, Reference Filipovic2007; Levinson & Wilkins, Reference Levinson and Wilkins2006; Malt, Gennari, Imai, Ameel, Tsuda, & Majid, Reference Malt, Gennari, Imai, Ameel, Tsuda and Majid2008; Slobin, Reference Slobin, Niemeier and Dirven2000, Reference Slobin, Hickmann and Robert2006). The question, then, is whether the motor components of such semantically idiosyncratic verbs rely on the precentral motor cortices. Based on the passage from Hickok that I quoted above, it would appear that he is skeptical. Yet that passage seems to conflict with an earlier one in the same chapter, where he acknowledges that “sensory and motor systems perform abstract, complex computations” (p. 128, my emphasis). It is also noteworthy that although Hickok focuses mainly on the primary motor cortex throughout his discussion of action concepts (pp. 131, 136, 137, 159, 174), the majority of experimental studies have concentrated more on the premotor cortex, which is widely believed to support a greater degree of schematization.
Continuing with this theme, numerous fMRI studies have shown that tasks such as reading action verbs (e.g., Hauk, Johnsrude, & Pulvermüller, Reference Hauk, Johnsrude and Pulvermüller2004), hearing action verbs (e.g., Raposo, Moss, Stamatakis, & Tyler, Reference Raposo, Moss, Stamatakis and Tyler2009), making semantic similarity judgments about action verbs (e.g., Kemmerer et al., Reference Kemmerer, Gonzalez-Castillo, Talavage, Patterson and Wiley2008), and distinguishing action verbs from non-words (e.g., de Grauwe, Willems, Rueschemeyer, Lemhöfer, & Schriefers, 2014) tend to evoke more or less somatotopically organized responses in premotor and/or primary motor areas. In considering such findings, Hickok expresses several concerns.
One is that when the verb-induced activation peaks from multiple studies are plotted in relation to cytoarchitectonically defined premotor and primary motor regions, many of them fall beyond the boundaries of both areas (de Zubicaray, Archiuli, & McMahon, 2013). This worry is mitigated, however, by the fact that the premotor and primary motor regions have also been defined on the basis of functional neuroimaging data (Mayka, Corcos, Leurgans, & Vaillancourt, Reference Mayka, Corcos, Leurgans and Vaillancourt2006), and when numerous verb-induced activation peaks are plotted on these maps instead, most if not all of them fall inside the boundaries of the two areas (see Figure 4; Kemmerer & Gonzalez-Castillo, Reference Kemmerer and Gonzalez-Castillo2010; Kemmerer et al., Reference Kemmerer, Rudrauf, Manzel and Tranel2012). Additional research is needed to determine which approach to defining the regions is more appropriate. But it is noteworthy that – completely apart from the definitional debate, and perhaps of greater importance – several studies have used functional localizer scans to verify that some of the motor areas that are engaged when subjects process body-part-specific action verbs are also engaged when they execute correspondingly body-part-specific movements (e.g., Desai, Binder, Conant, & Seidenberg, Reference Desai, Binder, Conant and Seidenberg2010; Hauk et al., Reference Hauk, Johnsrude and Pulvermüller2004; Moody-Triantis, Humphreys, & Gennari, Reference Moody-Triantis, Humphreys and Gennari2014; Raposo et al., Reference Raposo, Moss, Stamatakis and Tyler2009).

Fig. 4. Activation peaks in left primary motor and premotor cortices reported by some of the fMRI studies that have investigated the neural substrates of the motor features of verbs and sentences encoding leg/foot-related actions (green circles), arm/hand-related actions (light blue circles), and face/mouth-related actions (red circles). Activations are plotted on an inflated brain with boundaries for the primary motor cortex (dark blue) and premotor cortex (yellow) based on Mayka et al.’s (Reference Mayka, Corcos, Leurgans and Vaillancourt2006) Human Motor Area Template. Complete references to the fMRI studies from which activation data were drawn can be found in Kemmerer et al. (Reference Kemmerer, Rudrauf, Manzel and Tranel2012) (from Kemmerer et al., Reference Kemmerer, Rudrauf, Manzel and Tranel2012, p. 843; reproduced with permission).
Another concern of Hickok’s is that, according to a recent meta-analysis by Watson, Cardillo, Ianni, and Chatterjee (Reference Watson, Cardillo, Ianni and Chatterjee2013), some fMRI studies have found that action verbs do not elicit any significant responses whatsoever in the precentral motor cortices. But while this may appear to undermine the hypothesis that the motor features of verb meanings are represented in the precentral motor cortices, it does so only if one assumes that those features must always be accessed when action verbs are processed. A close look at the literature on this topic reveals a considerable amount of variability. On the one hand, several studies employing either electrophysiology or magnetoencephalography have found that verbs for leg/foot actions, arm/hand actions, and face/mouth actions not only trigger the expected topographic response patterns in the precentral motor cortices, but do so extremely quickly – in some cases within approximately 200 ms post stimulus onset (Dalla Volta, Fabbri-Destro, & Gentilucci, Reference Dalla Volta, Fabbri-Destro and Gentilucci2014; Hauk & Pulvermüller, Reference Hauk and Pulvermüller2004; Klepp et al., Reference Klepp, Weissler, Niccolai, Terhalle, Geisler, Schnitzler and Biermann-Ruben2014; Pulvermüller, Shtyrov, & Illoniemi, Reference Pulvermüller, Shtyrov and Illoniemi2005b), and in other cases even faster, as soon as 80 ms after the words can be uniquely identified (Shtyrov, Butorina, Nikolaeva, & Stroganova, Reference Shtyrov, Butorina, Nikolaeva and Stroganova2014). But while these findings suggest that action verbs engage the precentral motor cortices automatically, a growing number of other results support the alternative view that this process is strongly influenced by both task and context. Here are just two examples. In a TMS study, Papeo, Vallesi, Isja, and Rumiati (Reference Papeo, Vallesi, Isaja and Rumiati2009) found that although the level of activity in hand-related primary motor cortex was enhanced when participants were instructed to think about the motor features of hand-related verbs, it was not enhanced when participants were instructed to count the number of syllables in the very same words. And in an fMRI study, Raposo et al. (Reference Raposo, Moss, Stamatakis and Tyler2009) found that although somatotopically mapped motor areas were engaged when participants heard action verbs in isolation (e.g., kick) and in literal phrases (e.g., kick the ball), they were not engaged when participants heard such verbs in idiomatic phrases (e.g., kick the bucket) (for similar results see Aziz-Zadeh, Wilson, Rizzolatti, & Iacoboni, Reference Aziz-Zadeh, Wilson, Rizzolatti and Iacoboni2006, Desai, Conant, Binder, Park, & Seidenberg, Reference Desai, Conant, Binder, Park and Seidenberg2013, and Schuil, Smits, & Zwaan, Reference Schuil, Smits and Zwaan2013, but for contrary results see Boulenger, Hauk, & Pulvermüller, Reference Boulenger, Hauk and Pulvermüller2009). These studies, among others (Aravena et al., Reference Aravena, Courson, Frak, Cheylus, Paulignan, Deprez and Nazir2014; Aravena et al., Reference Aravena, Delevoye-Turrell, Deprez, Cheylus, Paulignan, Frak and Nazir2012; Mirabella, Iaconelli, Spadacenta, Federico, & Gallese, Reference Mirabella, Iaconelli, Spadacenta, Federico and Gallese2012; Papeo, Rumiati, Cecchetto, & Tomasino, Reference Papeo, Rumiati, Cecchetto and Tomasino2012; Sato, Mengarelli, Riggio, Gallese, & Buccino, Reference Sato, Mengarelli, Riggio, Gallese and Buccino2008), suggest that verb-induced motor activation is not a rigid, inflexible affair, but is instead sensitive to attentional and situational factors that are only beginning to be understood. It is essential to realize, however, that the mere fact that there is some variability regarding when and how the motor features of verb meanings are retrieved does not imply that those features are not really long-term components of the concepts, or that they are not really subserved by the precentral motor cortices. After all, as Reference Lebois, Wilson-Mendenhall and BarsalouLebois, Wilson-Mendenhall, and Barsalou (in press) recently demonstrated, variability in conceptual grounding effects – i.e., in behavioral and neural markers of the retrieval of modality-specific representations during conceptual processing – is far more common than often assumed, and even the most entrenched features of concepts are not always activated in an immediate, automatic manner (see also Binder & Desai, Reference Binder and Desai2011; Willems & Casasanto, Reference Willems and Casasanto2011; Willems & Fracken, Reference Willems and Francken2012; Zwaan, Reference Zwaan2014).
Finally, Hickok echoes Mahon and Caramazza (Reference Mahon and Caramazza2008) in arguing that even when action verbs do engage the precentral motor cortices, this may not be functionally relevant to comprehension. To support such a position, he refers once again to individuals who have reduced motor capacities but are nevertheless able to use action verbs. For instance, he points out that Stephen Hawking “has severe motor dysfunction, yet understands verbs well enough to function at a very high intellectual level” (p. 138). In keeping with this view, a few recent neuropsychological studies have found that, indeed, disturbances involving the precentral motor cortices do not always affect the comprehension of action verbs (Arévalo, Baldo, & Dronkers, Reference Arévalo, Baldo and Dronkers2012; Kemmerer, Miller, MacPherson, Huber, & Tranel, Reference Kemmerer, Miller, MacPherson, Huber and Tranel2013). It is possible, however, that in all of these cases relatively good performance could be achieved by relying mainly on various non-motor aspects of verb meanings, such as their visual motion properties, which are most likely represented in the left pSTS/pMTG (see the previous section, as well as Kemmerer, 2014). Such a strategy may not always be sufficient, though. As indicated above, when Kemmerer et al. (Reference Kemmerer, Rudrauf, Manzel and Tranel2012) administered three different verb comprehension tasks to 147 brain-damaged patients with widely distributed left- or right-hemisphere lesions, they found that deficits were linked not only with lesions in the left pSTS/pMTG, but also, and more consistently, with lesions in the left IFC. Moreover, across all three tasks, deficits were also related to damage in putatively hand-related sectors of the precentral motor cortices. These latter results dovetail nicely with several TMS studies which have shown that by briefly perturbing hand-related motor regions, one can, depending on the details of the protocol, either facilitate (Pulvermüller, Hauk, Nikulin, & Ilmoniemi, Reference Pulvermüller, Hauk, Nikulin and Ilmoniemi2005a) or disrupt (Gerfo, Oliveri, Torriero, Salerno, Koch, & Caltagirone, Reference Gerfo, Oliveri, Torriero, Salerno, Koch and Caltagirone2008; Kuipers, van Koningsbruggen, & Thierry, Reference Kuipers, van Koningsbruggen and Thierry2013; Repetto, Columbo, Cipresso, & Riva, Reference Repetto, Colombo, Cipresso and Riva2013) the processing of hand-related action verbs. Of course, no experiment is perfect, and both Papeo et al. (Reference Papeo, Pascual-Leone and Caramazza2013) and Hickok rightly point out that some of these TMS studies have limitations. Collectively, however, they bolster the hypothesis that, contrary to Hickok’s position, the precentral motor cortices play genuinely causal roles in the comprehension of linguistically expressed actions (for additional data involving expertise effects, see Beilock, Lyons, Mattarella-Micke, Nusbaum, & Small, Reference Beilock, Lyons, Mattarella-Micke, Nusbaum and Small2008; Locatelli, Gatti, & Tettamanti, Reference Locatelli, Gatti and Tettamanti2012; Lyons, Mattarella-Micke, Cieslak, Nusbaum, & Small, Reference Lyons, Mattarella-Micke, Cieslak, Nusbaum and Small2010).
4. Perceiving speech
In Chapter 5, Hickok focuses on the controversial question of whether the articulatory system contributes not only to speech production but also to speech perception. He discusses this issue in depth because, in his opinion, “the speech domain has become the ultimate test case for the viability of the action understanding interpretation of mirror neurons” (p. 91). Although I do not believe that this particular domain really has such a special status, I nevertheless think that Chapter 5 is one of the best in the book, largely because Hickok is intimately familiar with the material and hence is on very solid ground, having devoted much of his career to investigating, from both empirical and theoretical perspectives, the neural underpinnings of speech processing (Hickok & Poeppel, Reference Hickok and Poeppel2007; Hickok, Houde, & Rong, Reference Hickok, Houde and Rong2011; Hickok, Reference Hickok2012, Reference Hickok2014a, Reference Hickok2014b).
He begins by recounting the rise and fall of the motor theory of speech perception during the 30-year period between the late 1950s and late 1980s. In short, this theory maintains that we do not recognize speech sounds according to their acoustic patterns, but rather according to their articulatory patterns. Hickok deftly describes how this bold proposal gradually gained support in psycholinguistics but then lost favor when it was forced to confront a series of challenging findings, such as that both prelingual infants and non-lingual chinchillas are able to perceive speech sounds like /ta/ and /da/ in a categorical manner, despite being unable to produce them. By the early 1990s, the motor theory no longer had many proponents. It was quickly resurrected, however, after the discovery of mirror neurons, and ever since then a steady stream of brain mapping studies has been exploring the extent to which speech perception is subserved not only by superior temporal areas for auditory processing, but also by frontal motor areas for articulatory control. At first, Hickok admits that some of these studies “are impressive and in isolation appear to provide strong support for the motor theory of speech perception” (p. 91). But then he proceeds to expose a number of mostly methodological shortcomings of several studies, and also claims that a handful of other experiments “are open to a similar kind of critique: they use partially ambiguous stimuli, fail to account for response bias, and use artificial tasks that don’t necessarily tap into processes that are used under normal, everyday speech listening conditions” (p. 101). To reinforce his position that frontal motor areas are not causally involved in ordinary speech perception, he goes on to review several lines of neuropsychological research – including some fascinating work from his own lab – showing that although disturbances of articulatory control can interfere with phoneme discrimination and identification tasks that require close attention to the speech sounds themselves, they do not impair the ability to perform spoken word comprehension tasks, like matching the word bear with a picture of a bear, even when the array of distractors includes pictures of objects with similar-sounding names (e.g., a pear). Based on such data, as well as on the older results from infants and chinchillas, Hickok concludes that “when you take the motor speech system out of the picture the ability to perceive speech sounds remains unaffected. The motor theory of speech perception is (still) wrong” (p. 109).
To be sure, Hickok makes a powerful case that the motor theory of speech perception places too much weight on the articulatory component and too little weight on the auditory component. I am not entirely convinced, however, that he is right to downplay the importance of the articulatory component as much as he does. For one thing, it may not be such a serious problem that the vast majority of brain mapping studies which have found frontal motor areas to be involved in speech perception have used tasks that force subjects to explicitly detect subtle phonemic contrasts (besides the studies cited by Hickok, see Reference Bartoli, D’Ausilio, Berry, Badino, Bever and FadigaBartoli, D’Ausilio, Berry, Badino, Bever, & Fadiga, in press; Callan, Callan, & Jones, Reference Callan, Callan and Jones2014; Du, Buchsbaum, Grady, & Alain, Reference Du, Buchsbaum, Grady and Alain2014; Liebenthal, Sabri, Beardsley, Mangalathu-Arumana, & Desai, Reference Liebenthal, Sabri, Beardsley, Mangalathu-Arumana and Desai2013; Rogers, Möttönen, Boyles, & Watkins, Reference Rogers, Möttönen, Boyles and Watkins2014; Sehm et al., Reference Sehm, Schnitzler, Obleser, Groba, Ragert, Villringer and Obrig2013). This is because there are actually many situations in daily life when one hears utterances with moderate to low intelligibility – for instance, when listening to a talker in a loud environment, or when listening to a talker who has a heavy accent or a speech impediment – and it certainly seems worthwhile to know that in such circumstances one’s own articulatory system is recruited to facilitate perception, perhaps by using motorically based internal models in a top-down manner to compensate for degraded bottom-up sensory representations (Davis & Johnsrude, Reference Davis and Johnsrude2007; also, for evidence that the engagement of articulatory cortical areas during demanding laboratory-based speech discrimination tasks cannot be explained by response bias, see Reference Smalle, Rogers and MöttönenSmalle, Rogers, & Möttönen, in press).
Furthermore, two recent studies indicate that even when people do not need to perform effortful tasks in response to speech sounds, frontal motor regions are engaged in ways that causally influence perception. In the first study, Möttönen, Dutton, and Watkins (Reference Möttönen, Dutton and Watkins2013) exploited the well-established fact that, completely independent of attention and task demands, occasional changes in sound sequences trigger an electrophysiological effect called the mismatch negativity (MMN), which is generated in the auditory cortex 100–200 ms after stimulus onset (Näätänen, Tervaniemi, Sussman, Paavilainen, & Winkler, Reference Näätänen, Tervaniemi, Sussman, Paavilainen and Winkler2001). Each experiment began by applying a 15-minute train of TMS pulses to either the lip representation or the hand representation in the left primary motor cortex, thereby reducing the excitability of the targeted representation for at least 15 minutes after the end of stimulation. Next, subjects watched a silent film, and while they were focusing their attention on the movie, they heard sound sequences consisting of frequent /da/ syllables and infrequent /ba/ and /ga/ syllables. The main finding was that TMS-induced disruption of the motor lip representation, but not of the motor hand representation, significantly decreased the MMNs elicited by both of the infrequent syllables. This supports the view that the articulatory system normally contributes to the automatic discrimination of speech sounds. It is noteworthy, however, that disruption of the motor lip representation impaired the processing of not only the lip-related /ba/ syllables, but also the non-lip-related /ga/ syllables, suggesting a non-specific effect.
In the second study, Möttönen, van de Ven, and Watkins (Reference Möttönen, van de Ven and Watkins2014) explored this issue further by combining TMS with magnetoencephalography. The subjects heard two sequences of speech sounds – one consisting of alternating /ba/ and /da/ syllables, and another consisting of alternating /da/ and /ga/ syllables – in two different conditions: one in which they were instructed to attend to a silent film, and another in which they saw the same silent film but were instructed to attend to the speech sounds. Once again, when the speech stimuli were ignored, TMS-induced disruption of the motor lip representation interfered with perception in a general manner, modulating the bilateral auditory cortex responses to both the /ba/–/da/ sequence and the /da/–/ga/ sequence, starting around 170 ms after sound onset. On the other hand, when the speech stimuli were attended, TMS-induced disruption of the motor lip representation interfered with perception in an articulator-specific manner, with significantly greater left-lateralized modulation of the /ba/–/da/ sequence than the /da/–/ga/ sequence, starting as early as 60–100 ms after sound onset.
Taken together, the results of these two studies provide compelling evidence that, contrary to Hickok’s view, even when people don’t concentrate directly on the speech signals that they’re hearing, their articulatory system contributes to their perception. Moreover, when people do focus on those sounds, the top-down influence of frontal motor areas is both highly refined and extremely rapid (see also Lametti, Rochet-Capellan, Neufeld, Shiller, & Ostroy, Reference Lametti, Rochet-Capellan, Neufeld, Shiller and Ostroy2014).
5. Conclusion
I applaud Hickok for cutting through all the hype surrounding mirror neurons. However, I think he has gone too far in his critique, to the extent that he has thrown the proverbial baby out with the bathwater. As I have shown here, a growing literature strongly suggests that motor regions in the frontal and parietal lobes not only subserve the execution of actions, but also play genuinely functional roles in the perception and understanding of actions, regardless of whether they are directly observed or linguistically represented. In addition, recent research indicates that although the articulatory system is involved primarily in speech production, it enhances speech perception too, even in the absence of explicit attention to the auditory stimuli. Of course, many questions remain unanswered, but they will most likely be worked out in the coming years, since the field of mirror neuron research is actually much healthier than Hickok seems to realize.