INTRODUCTION
Seed dispersal, the movement of seeds away from the parent plant, is critical to the life cycle of most plants (Wang & Smith Reference WANG and SMITH2002). Dispersal processes are necessary for recruitment and thus underpin forest structure and dynamics (Howe Reference HOWE, Bawa and Hadley1990). The Janzen–Connell hypothesis for the maintenance of species diversity is based on the premise that effective seed dispersal reduces distance- and density-dependent mortality in trees (Connell Reference CONNELL, den Boer and Gradwell1971, Janzen Reference JANZEN1970). Moreover, attributes of dispersal effectiveness (Schupp Reference SCHUPP, Fleming and Estrada1993) are integral to other dispersal-assembly hypotheses, such as the recruitment limitation hypothesis (Hurtt & Pacala Reference HURTT and PACALA1995, Seidler & Plotkin Reference SEIDLER and PLOTKIN2006) and the neutral theory of species diversity (Hubbell Reference HUBBELL2001, May et al. Reference MAY, HUTH and WIEGAND2015). While there has been significant progress in understanding patterns and mechanisms of seed-dispersal systems in temperate and tropical systems, there has been little synthesis or evaluation of patterns in seed-dispersal processes along environmental gradients. In particular, the mechanisms driving the functional diversity of fruits and seeds, and disperser effectiveness, in tropical montane forests (TMF) are poorly understood. Evaluating how environmental gradients drive functional diversity is vital for managing global environmental change (McGill et al. Reference MCGILL, ENQUIST, WEIHER and WESTOBY2006).
In this review we define TMF as forest above 1500 m asl, but which may occur as low as 500–800 m asl closer to the coasts (Bruijnzeel & Veneklaas Reference BRUIJNZEEL and VENEKLAAS1998, Hamilton Reference HAMILTON1998, Scatena et al. Reference SCATENA, BRUIJNZEEL, BUBB, DAS, Bruijnzeel, Scatena and Hamilton2010). The parameters that differentiate TMF from TLFs (tropical lowland forests) include lower alpha diversity and dominance of a few tree species (Gentry Reference GENTRY1992), but with higher species turnover (beta diversity; Gentry Reference GENTRY1992, Whittaker & Niering Reference WHITTAKER and NIERING1975). Compared with TLF, TMF trees have small leaves, with poorly developed drip-tips and an absence of buttresses (Bruinjzeel & Veneklaas Reference BRUIJNZEEL and VENEKLAAS1998, Dalling et al. Reference DALLING, HEINEMAN, GONZÁLEZ and OSTERTAG2016, Fahey et al. Reference FAHEY, SHERMAN and TANNER2016, Grubb Reference GRUBB1977). Those parameters of TMF directly relevant to dispersal ecology are summarized in Figure 1, a conceptual model of the effects of altitude on seed-dispersal ecology, and discussed below.
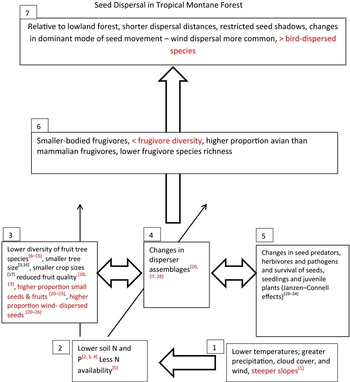
Figure 1. A conceptual diagram of how abiotic changes with elevation select for changes in flora and fauna which impact seed dispersal ecology in TMF. Arrows indicate pathways. [1] Bruijnzeel et al. Reference BRUIJNZEEL, SCATENA and HAMILTON2010, [2] Dalling et al. Reference DALLING, HEINEMAN, GONZÁLEZ and OSTERTAG2016, [3] Grubb (Reference GRUBB1977), [4] Körner (Reference KÖRNER2007), [5] Carbutt et al. Reference CARBUTT, EDWARDS, FYNN and BECKETT2013, [6] Basabose (Reference BASABOSE2002), [7] Dutton et al. (Reference DUTTON, CHAPMAN and MOLTCHANOVA2014), [8] Ganas et al. (Reference GANAS, ROBBINS, NKURUNUNGI, KAPLIN and MCNEILAGE2004), [9] Giraldo et al. (Reference GIRALDO, GÓMEZ-POSADA, MARTÍNEZ and KATTAN2007), [10] Gross-Camp et al. (Reference GROSS-CAMP, MASOZERA and KAPLIN2009), [11] Hamilton (Reference HAMILTON1975), [12] Rothman et al. (Reference ROTHMAN, NKURUNUNGI, SHANNON, BRYER, Grow, Gursky-Doyen and Krzton2014), [13] Schmitt et al. Reference SCHMITT, DENICH, DEMISSEW, FRIIS and BOEHMER2010), [14] Yamagiwa & Basabose (Reference YAMAGIWA and BASABOSE2006), [15] Zhuang et al. (Reference ZHUANG, TIAN, CHEN, LI, LI and LU2012), [16] Bruijnzeel et al. (Reference BRUIJNZEEL, SCATENA and HAMILTON2010), [17] Wright et al. (Reference WRIGHT, ACKERLY, BONGERS, HARMS, IBARRA-MANRIQUEZ, MARTINEZ-RAMOS, MAZER, MULLER-LANDAU, PAZ, PITMAN, POORTER, SILMAN, VRIESENDORP, WEBB, WESTOBY and WRIGHT2007), [18] Marshall et al. (Reference MARSHALL, TOPP-JØRGENSEN, BRINK and FANNING2005), [19] Ramírez et al. (Reference RAMÍREZ, MÓNICA, GALVIS, VARGAS, LÉON, CIFUENTES, STEVENSON, Grow, Gursky-Doyen and Krzton2014), [20] Almeida-Neto et al. (Reference ALMEIDA-NETO, CAMPASSI, GALETTI, JORDANO and OLIVEIRA-FILHO2008), [21] Buitrón-Jurado & Ramírez (Reference BUITRÓN-JURADO and RAMÍREZ2014), [22] Duivenvoorden et al. (Reference DUIVENVOORDEN and CUELLO2012), [23] Friis (Reference FRIIS1992), [24] Grubb & Stevens (Reference GRUBB and STEVENS1985), [25] Tanner (Reference TANNER1982), [26] Vázquez & Givnish (Reference VÁZQUEZ-G. and GIVNISH1998), [27] Hamann & Curio (Reference HAMANN and CURIO1999), [28] Terborgh (Reference TERBORGH1977), [29] Meng et al. (Reference MENG, GAO, CHEN and MARTIN2012), [30] Nyiramana et al. (Reference NYIRAMANA, MENDOZA, KAPLIN and FORGET2011), [31] Xu & Yu Reference XU and YU2014, [32] Wenny (Reference WENNY2000), [33] Boudreau & Lawes (Reference BOUDREAU and LAWES2008), [34] Matthesius et al. (Reference MATTHESIUS, CHAPMAN and KELLY2011).
Altitude and topography are two major environmental gradients driving tree species composition in TMF (Homeier et al. Reference HOMEIER, BRECKLE, GÜNTER, ROLLENBECK and LEUSCHNER2010), and key characteristics that show directional change along altitudinal gradients include temperature, precipitation, wind, cloud cover and soil fertility (Figure 1). These characteristics may act synergistically on the distribution of plant traits, mostly through indirect effects via soil properties, microclimate and influence on frugivore diversity and density.
TMF comprise a small subset of tropical forests worldwide and in some regions are under severe threat from agriculture and pastoral farming (Burgess et al. Reference BURGESS, BUTYNSKI, CORDEIRO, DOGGART, FJELDSÅ, HOWELL, KILAHAMA, LOADER, LOVETT, MBILINYI, MENEGON, MOYER, NASHANDA, PERKIN, ROVERO, STANLEY and STUART2007, Cordeiro et al. Reference CORDEIRO, BURGESS, DOVIE, KAPLIN, PLUMPTRE and MARRS2007, Doumenge et al. Reference DOUMENGE, GILMOUR, PÉREZ, BLOCKHUS, Hamilton, Juvik and Scatena1995). Compared with TLFs, they generally harbour lower but nevertheless substantial levels of biodiversity and endemism (Bruijnzeel et al. Reference BRUIJNZEEL, SCATENA and HAMILTON2010, Bussmann Reference BUSSMANN2004, Myers et al. Reference MYERS, MITTERMEIER, MITTERMEIER, FONSECA and KENT2000) and are a source of numerous ecosystem services and functions; they store a significant amount of carbon (Spracklen & Righelato Reference SPRACKLEN and RIGHELATO2014, Yepes et al. Reference YEPES, HERRERA, PHILLIPS, CABRERA, GALINDO, GRANADOS, DUQUE, BARBOSA, OLARTE and CARDONA2015) and have a potentially important, although poorly understood role in hydroclimatic and biogeochemical cycles (Schröter et al. Reference SCHRÖTER, CRAMER, LEEMANS, PRENTICE, ARAÚJO, ARNELL, BONDEAU, BUGMANN, CARTER, GRACIA and ANNE2005).
Given the global importance of TMF, understanding their seed-dispersal ecology is necessary to ensure their persistence and regeneration in the face of increasing anthropogenic disturbance, and to maintain biodiversity and vital ecosystem functions that impact surrounding landscapes.
DISPERSAL ECOLOGY
The seed-dispersal cycle (sensu Wang & Smith Reference WANG and SMITH2002) comprises fruit production, fruit removal, seed dispersal, germination and recruitment. Biotic and abiotic factors influence the patterns of tree species distribution resulting from these processes and the patterns in turn influence community structure and dynamics (Charles-Dominique Reference CHARLES-DOMINIQUE, Alterman, Doyle and Izard1995, Franklin et al. Reference FRANKLIN, JOHANSSON, DEWAR, DIECKMANN, MCMURTRIE, BRÄNNSTRÖM and DYBZINSKI2012).
Dispersal agents include animals (zoochory), gravity through ballistic propulsion (ballistochory), wind (anemochory) and water (hydrochory). In tropical forests a large proportion of tree species have fleshy fruit evolved for seed dispersal by fruit-eating animals (Howe & Smallwood Reference HOWE and SMALLWOOD1982, Jordano Reference JORDANO and Fenner1992), so that plant–animal interactions underpin most dispersal processes. Certain combination sets of diaspore traits, ‘dispersal syndromes’ attract particular frugivore types (Gautier-Hion et al. Reference GAUTIER-HION, DUPLANTIER, QURIS, FEER, SOURD, DECOUX, DUBOST, EMMONS, ERARD, HECKETSWEILER, MOUNGAZI, ROUSSILHON and THIOLLAY1985, Howe & Smallwood Reference HOWE and SMALLWOOD1982, Janson Reference JANSON1992). For example, fruits of plants dispersed by birds tend to be small (<2 cm diameter), with many small seeds, and brightly coloured such as red, orange, purple, or black, while those eaten by primates tend to be larger (>2 cm diameter) harder, brown or green in colour with few large seeds, fibre and sugary pulps (Gautier-Hion et al. Reference GAUTIER-HION, DUPLANTIER, QURIS, FEER, SOURD, DECOUX, DUBOST, EMMONS, ERARD, HECKETSWEILER, MOUNGAZI, ROUSSILHON and THIOLLAY1985). However, dispersal syndromes are not mutually exclusive. In tropical forests, bats, birds, primates and other frugivore taxa may contribute to the dispersal of plant species with different seed-dispersal syndromes (Dowsett-Lemaire Reference DOWSETT-LEMAIRE1985). Nonetheless, diaspore size is probably the single most important determinant of the dominant disperser type (Jordano Reference JORDANO1995, Wheelwright Reference WHEELWRIGHT1985).
The dispersal effectiveness of zoochory in terms of plant fitness varies according to animal morphology, physiology and behaviour (Côrtes & Uriarte Reference CÔRTES and URIARTE2013, Ruxton & Schaefer Reference RUXTON and SCHAEFER2012). Seed dispersal produces a seed shadow, whose shape in endozoochorous (dispersal by passage through an animal's gut) species is determined by frugivore foraging patterns and the distances they move seeds, the habitat into which they disperse seeds, seed treatment (swallowing, regurgitating, spitting) and gut retention time if swallowed (Schupp Reference SCHUPP, Fleming and Estrada1993, Wang & Smith Reference WANG and SMITH2002). All of these processes influence the spatial distribution of dependent plant species (Gross-Camp & Kaplin Reference GROSS-CAMP and KAPLIN2011, Wenny & Levey Reference WENNY and LEVEY1998). Secondary dispersers, like rodents and dung-beetles, further influence dispersal kernels, often shaping the kernel and moving seeds into more suitable microhabitats (Gallegos et al. Reference GALLEGOS, HENSEN and SCHLEUNING2014). Frugivore seed handling and seed deposition patterns, secondary dispersers and the relative importance of anemochory versus zoochory are all influenced by altitude, with the possibility of predictable community-wide differences in plant structure and dynamics. However, as tropical forests vary in composition and structure among regions, depending on factors such as latitude, biogeographic history, anthropogenic history and other site characteristics (Corlett & Primack Reference CORLETT and PRIMACK2011, Culmsee et al. Reference CULMSEE, LEUSCHNER, MOSER and PITOPANG2010, Griffiths & Lawes Reference GRIFFITHS and LAWES2006, Nadkarni & Wheelwright Reference NADKARNI and WHEELWRIGHT2000), it is simplistic to expect altitude to have a unifying influence on dispersal ecology. Nevertheless, some generalizations can be made and we hypothesize that differences in dispersal syndromes and dispersal processes between TMF and TLFs are primarily linked to altitudinal changes in floristic composition, and corresponding variation in vertebrate and invertebrate assemblages. We explore how changes in the environment along the altitudinal gradient from lowland to montane forests select for different diaspore traits and different animal guilds with different behaviours (i.e. dispersal syndromes), which in turn affect dispersal ecology with implications for plant recruitment, community composition and habitat dynamics. It remains unclear to what extent abiotic factors such as temperature and moisture, rather than biotic factors such as frugivore assemblages select for diaspore traits; the two are deeply intertwined and over evolutionary and ecological time, both contribute to varying extents (Correa et al. Reference CORREA, ÁLVAREZ and STEVENSON2015).
Very sudden transitions in floristic and faunal composition can occur along altitudinal gradients, independent of environmental gradients and often a consequence of biogeography (Buitrón-Jurado & Ramírez Reference BUITRÓN-JURADO and RAMÍREZ2014, Friis Reference FRIIS1992, Gentry Reference GENTRY, Bullock, Mooney and Medina1995, Grimshaw Reference GRIMSHAW, Huxley, Lock and Cutler1998, Sugden Reference SUGDEN1982, Vázquez & Givnish Reference VÁZQUEZ-G. and GIVNISH1998, Webb et al. Reference WEBB, TRACEY and JESSUP1986, White Reference WHITE1983) and/or climate-disturbance interactions (Martin et al. Reference MARTIN, SHERMAN and FAHEY2007). We briefly discuss the nature of such transitions in TMF, but first we examine directional change to test the hypothesis that altitudinal differences in flora and fauna lead to predictable effects in dispersal ecology along the altitudinal gradient.
CHANGES WITH ALTITUDE
The contribution of each environmental characteristic to changes in plant traits, animal assemblages and microbial communities with increasing altitude (Figure 1) cannot easily be separated. However, the trend for smaller trees, lower diversity of fleshy-fruited tree species and smaller crop size can be directly linked to soil nutrient availability, especially nitrogen (N), which is critical for fruit production (DiManno & Ostertag Reference DIMANNO and OSTERTAG2016). Nitrogen often declines, or become less available to plants (Carbutt et al. Reference CARBUTT, EDWARDS, FYNN and BECKETT2013) with increasing altitude, and this, together with fewer beneficial soil micro-organisms in TMF in general (Dalling et al. Reference DALLING, HEINEMAN, GONZÁLEZ and OSTERTAG2016, Tanner et al. Reference TANNER, VITOUSEK and CUEVAS1998), leads to trade-offs in plant functional traits.
Diaspore traits
Diaspore traits most obviously affecting seed-dispersal ecology include seed or fruit size (variously recorded as length, diameter and/or mass), fruit type (e.g. hard, fleshy, dehiscent) and seed-dispersal mode (e.g. zoochory, anemochory, barochory) (Howe & Smallwood Reference HOWE and SMALLWOOD1982). To investigate trends in these traits with increasing altitude we compiled data comprising diaspore traits for 867 tree species along five montane tropical forest altitudinal gradients over 17 sites in Thailand, the Philippines, Tanzania, Malawi and Nigeria (Figure 2). These data were sourced from the published literature, online databases including Kew Seed Information Database, Africa Tree Database and JSTOR Global Plants, as well as unpublished data from author contributions (Table 1).

Figure 2. Map showing general location of the five montane tropical forest altitudinal gradients in Malawi, Nigeria, the Philippines, Tanzania and Thailand.
Table 1. Main descriptors for each of the tropical forests used in this review. Disturbance is classified based on low (intact, no hunting, heavily protected), moderate (some resources extracted, some hunting, some protection), high (heavily degraded, hunting severe, low protection). 1= Mittermeier et al. (Reference MITTERMEIER, ROBLES-GIL, HOFFMANN, PILGRIM, BROOKS, MITTERMEIER, LAMOREUX and FONSECA2004); 2 = Chapman & White (Reference CHAPMAN and WHITE1970); 3 = Dowsett-Lemaire (Reference DOWSETT-LEMAIRE1985); 4 = Chapman & Chapman (Reference CHAPMAN and CHAPMAN2001); 5 = Hamann et al. (Reference HAMANN, BARBON, CURIO and MADULID1999); 6 = Ingle (Reference INGLE2003); 7 = Kanzaki et al. (Reference KANZAKI, HARA, YAMAKURA, OHKUBO, TAMURA, SRI-NGEMYUANG, SAHUNALU, TEEJUNTUK, BUNYAVEJCHEWIN, Losos and Leigh2004); 8 = Brockelman et al. (Reference BROCKELMAN, NATHALANG and GALE2011); 9 = Lovett (Reference LOVETT1998); 10 = Hamilton & Bensted-Smith (Reference HAMILTON and BENSTED-SMITH1989) and unpublished data.

A lack of data across all diaspore traits and the inconsistent way in which traits have been recorded across studies necessitated a simplified analytical approach. As more data were available on fruits than seeds for most species, we used fruit as analogous to diaspore, given that in many cases it is the fruit that is transported by dispersal agents. Among the fruit traits we evaluated – fruit size (length and diameter), fruit type and dispersal mode – fruit size was analysed as continuous, while fruit type and dispersal mode were converted into ordinal factors as follows: (1) fruit type was an ordinal factor where 0 = dry fruits and 1 = fleshy fruits; and (2) dispersal mode was treated as 0 = abiotically dispersed species and 1 = animal-dispersed species. From the latter, higher values of community weighted means (CWM) for these three fruit traits represent larger, animal-dispersed, fleshy fruits.
To determine the relationship between fruit traits and altitude we used ‘species per site’ and ‘trait per species’ matrices to calculate CWM for each study site, using the ‘matrix.t’ procedure in the ‘SYNCSA’ package for R software. We then checked for collinearity among trait CWM using the function ‘corrplot’ from the R package ‘corrplot’.
Our analysis of five altitudinal gradients found that altitude accounted for 30% of the variation in fruit length, and 20% of the variation in fruit diameter. Fruit length was significant, indicating a decrease in diaspore size with increasing altitude (F1,15 = 7.87; P = 0.013; adjusted R² = 0.30; Figure 3a). Although other traits related to diaspore size tended to decrease with altitude, the relationship was weak (F1,15 = 3.81; P = 0.07; adjusted R² = 0.14; Figure 3b). A community-wide decrease in diaspore size (both fruit and seed) with increasing altitude in tropical forests is well documented, especially in the Neotropics (Figure 1 and references therein). Species with small fleshy fruits are the norm at higher altitudes and are common at lower altitudes, while species with large fleshy fruits tend to be absent at high altitudes (Almeida-Neto et al. Reference ALMEIDA-NETO, CAMPASSI, GALETTI, JORDANO and OLIVEIRA-FILHO2008, Duivenvoorden et al. Reference DUIVENVOORDEN and CUELLO2012, Friis Reference FRIIS1992).

Figure 3. Regression analysis of community weighted means (CWM) for (a) fruit length (cm) and (b) fruit diameter against altitude (m asl) from 867 tree species along five tropical forest altitudinal gradients in Malawi, (M), Nigeria (N), the Philippines (Ph), Tanzania (Tz) and Thailand (Th). M.1 – 1250 m asl, M.2 – 1830 m asl, M.3 – 1980 m asl, M.4 – 2075 m asl, N.1 – 230 m asl, N.2 – 230 m asl, N.3 – 1500 m asl, N.4 – 1830 m asl, N.5 – 2090 m asl, Ph.1 – 1000 m asl, Ph.2 – 1450 m asl, Tz.1 – 240 m asl, Tz.2 – 1025 m asl, Th.1 – 700 m asl, Th.2 – 795 m asl, Th.3 – 1100 m asl, Th.4 – 1700 m asl. These data were sourced from the published literature, online databases including Kew Seed Information Database, Africa Tree Database and JSTOR Global Plants, as well as unpublished data from author contributions.
For those species for which seed-length data were available, we evaluated seed length across the altitudinal gradients; we grouped the sites into three altitudinal zones, 0–1000 m asl, 1100–1500 m asl and 1600–2100 m asl. Seed length (log-transformed) at the community level also declined with increasing altitude (ANOVA F2,920 = 4.53, P = 0.011), and sites in the two higher altitudinal zones had smaller seeds on average (Mean ± SD; 1100–1500 m = 10.7 ± 11.1 mm; 1600–2100 m = 10.9 ± 11.8 mm; both P < 0.05) than the lowland zone (0–1000 m = 13.0 ± 12.9 mm); seeds from the two higher zones did not differ (P > 0.05). Including sites from higher altitudes (>2500 m), and from a wider geographic area is needed to verify the consistency of this trend in seed size with altitude.
Why does community-wide diaspore size decrease with increasing altitude in tropical forests and to what extent is this trend driven by abiotic or biotic factors? Almedia-Neto et al. (Reference ALMEIDA-NETO, CAMPASSI, GALETTI, JORDANO and OLIVEIRA-FILHO2008) point out that this is a difficult question to answer because seed size is positively correlated with other plant traits such as tree height and leaf size, that are more obviously affected by environmental factors (Wright et al. Reference WRIGHT, ACKERLY, BONGERS, HARMS, IBARRA-MANRIQUEZ, MARTINEZ-RAMOS, MAZER, MULLER-LANDAU, PAZ, PITMAN, POORTER, SILMAN, VRIESENDORP, WEBB, WESTOBY and WRIGHT2007). As tree height in TMF decreases with altitude (Figure 1) so does seed size, commensurate with a described allometric relationship between tree size and fruit size (Grubb Reference GRUBB1998). Likewise leaf size decreases with altitude and the allometric relationship between leaf size and inflorescence size is such that seed size also is predicted to decrease (Midgley & Bond Reference MIDGLEY and BOND1989). Small seed size disadvantages seedlings growing in shady habitats as it provides lower initial energy reserves needed to tolerate shade for longer and to grow faster to access as much light as possible (Leishman & Westoby Reference LEISHMAN and WESTOBY1994). The small seed size in TMFs may be partly due to low levels of available soil nitrogen (Fahey et al. Reference FAHEY, SHERMAN and TANNER2016). While larger seeds should theoretically be at an advantage in nutrient-poor soils (Moles & Westoby Reference MOLES and WESTOBY2004), large seeds require more nutrients to produce than small seeds, and at altitude where these nutrients are limited and trees consequently have relatively small stature, selection for less costly, abundant small seeds may enhance recruitment in TMF. At lower elevations there is selection pressure for large seeds because of higher metabolic costs associated with establishment (Lord et al. Reference LORD, EGAN, CLIFFORD, JURADO, LEISHMAN, WILLIAMS and WESTOBY1997). Numerous small seeds may ensure pioneer species are more competitive in montane forests through greater broadcast dispersal of seeds into gaps and forest edges (Dalling & Hubbell Reference DALLING and HUBBELL2002), especially in Afromontane forests where climatic fluctuations have led to continual recolonization (Grimshaw Reference GRIMSHAW, Huxley, Lock and Cutler1998).
Frugivores may exert selection pressure on fruit size or vice versa where fruit size may determine the structure of the frugivore guild. The direction of selection is difficult to elicit in this instance. Correa et al. (Reference CORREA, ÁLVAREZ and STEVENSON2015), Jordano (Reference JORDANO1995), Lambert & Garber (Reference LAMBERT and GARBER1998) and Lord et al. (Reference LORD, EGAN, CLIFFORD, JURADO, LEISHMAN, WILLIAMS and WESTOBY1997) all argue that frugivores select for diaspore size because gape width and body size limit the size of fruit that a frugivore can swallow (Moermond & Denslow Reference MOERMOND and DENSLOW1985, Wheelwright Reference WHEELWRIGHT1985). Mashers, birds that mandibulate fruits to drop seeds and ingest only fruit pulp or juice (Levey Reference LEVEY, Estrada and Fleming1986), strongly select for small seed size by virtue of their seed treatment, and are common in Neotropical montane forests (Moermond & Denslow Reference MOERMOND and DENSLOW1985). The general trend in TMF is that frugivore body size decreases with increasing altitude (Figures 1, 4, 5). Figure 4 illustrates how body mass of frugivorous birds tends to decrease at higher altitudes (ANOVA F 2,1185 = 3.5, P = 0.03). Specifically, birds from lower altitudes (< 1000 m) were larger on average than those from middle altitudes (1000–2000 m; Tukey HSD, P = 0.033); body mass of birds from higher altitudes (>2000 m) did not differ from those at middle and lower elevations (Ps > 0.05).

Figure 5. Body mass (g) (n = 1206) of fruit-eating birds at the community level at three altitudinal ranges: lower (< 1000 m asl), middle (1000–2000 m asl) and upper (>2000 m asl) in five different regions: Australasia, Africa, Indomalaya, Neotropics and Oceanica. Data from Handbook of the birds of the world (HBW 1992–2013).
A decrease in bird body size is generally associated with smaller gape size (Wheelwright Reference WHEELWRIGHT1985), and this body size–gape size trend was confirmed in TMF (Wenny et al. Reference WENNY, SEKERCIOGLU, CORDEIRO, ROGERS, KELLY, Sekercioglu, Wenny and Whelan2016). However, a comparison of frugivorous birds' body mass across Australia, Africa, Indonesia, the Neotropics and Oceania illustrated that the extent of the general trend toward smaller body size at higher altitudes differs among regions (ANOVA F 4,1181 = 9.2, P < 0.001). Specifically, birds from Africa were smaller on average than those from Australasia (Tukey HSD, P = 0.011), and birds from the Neotropics were smaller on average than those from Australasia (P < 0.001) and Indomalaya (P < 0.009). Avian body mass did not differ significantly for the other possible pairwise comparisons among geographic regions (Ps > 0.05).
Where large-seeded species persist at relatively high altitudes in TMF, they are likely to have specialized and coevolved dispersal syndromes. For example, in high montane forest in the Drakensberg of South Africa the large seeds of Calodendrum capensis may be dispersed by bats and the large Rameron pigeon (Columba arquatrix; Coates Palgrave Reference COATES PALGRAVE2003). Similarly, in Nigerian and Tanzanian TMF, large-seeded tree species such as Carapa and Allanblackia, respectively, have nuts that are eaten and dispersed by Cricetomys rodents (Aliyu et al. Reference ALIYU, ADAMU, MOLTCHANOVA, FORGET and CHAPMAN2014, Seltzer et al. Reference SELTZER, KREMER, NDANGALASI and CORDEIRO2015). In general, however, large-bodied frugivores in TMF are rare and therefore the exception that proves the rule that frugivore size likely selects for small seed size in TMF.
Another well-documented trend is an increase in the relative proportion of anemochorous species with altitude (Figure 1). For example, Grubb & Stevens (Reference GRUBB and STEVENS1985) found that anemochorous species in New Guinea forests increased from 7% (lower montane) to 13% (upper montane) to 20% (subalpine). Vázquez & Givnish (Reference VÁZQUEZ-G. and GIVNISH1998) report similar increases from 1500–2500 m asl in Mexico, and Tanner (Reference TANNER1982) reported a higher proportion of anemochorous diaspores in montane versus lowland forest in Jamaica. Friis (Reference FRIIS1992), found evidence for increased anemochorous tree species with altitude in Ethiopian forests, but not as strongly as the broader taxonomic studies.
The increasing proportion of anemochorous species along altitudinal gradients in tropical montane forests may in part be a phylogenetic constraint (Jordano Reference JORDANO1995); temperate floristic elements have a higher representation in TMF than in TLFs, and many of these are herbaceous or shrubby and anemochorous (Buitrón-Jurado & Ramírez Reference BUITRÓN-JURADO and RAMÍREZ2014, Gentry Reference GENTRY, Bullock, Mooney and Medina1995, White Reference WHITE1983). Vascular epiphytes, many of which, but by no means all, are orchids with tiny seeds, increase in abundance with altitude in cloud forests (Grubb Reference GRUBB1977, Murray et al. Reference MURRAY, KINSMAN, BRONSTEIN, Nadkarni and Wheelwright2000, Vázquez & Givnish Reference VÁZQUEZ-G. and GIVNISH1998). However, it is a reasonable assumption that this may also reflect adaptation to windier conditions in TMF. Buitrón-Jurado & Ramírez (Reference BUITRÓN-JURADO and RAMÍREZ2014) tested for phylogenetic independence of anemochory, and concluded that the greater phylogenetic overdispersion in upper relative to lower montane forests in the Andes was evidence for anemochory being adaptive at higher altitudes.
In our analyses CWM fruit type changed with increasing altitude with fleshy fruit species increasing relative to non-fleshy species (F1,15 = 2.64, P = 0.125, Figure 6). A similar trend is observed in South-East Asia where anemochorous/barochorous dipterocarp species decrease in frequency with increasing altitude and are ‘replaced’ by ornithochorous Lauraceae (Padmawathe et al. Reference PADMAWATHE, QURESHI and RAWAT2004). This suggests that the increase in anemochory reported above is not universal, or that it is more obvious at the upper elevational limits of TMF (above ~ 2200 m asl) or under different biogeographic pressures.

Figure 6. Regression analysis of community weighted means (CWM) for fruit type (dry fruits = 0, fleshy fruits =1) against altitude (m asl) from 867 tree species along five tropical forest altitudinal gradients in Malawi (M), Nigeria (N), the Philippines (Ph), Tanzania (Tz) and Thailand (Th). M.1 – 1250 m asl, M.2 – 1830 m asl, M.3 – 1980 m asl, M.4 – 2075 m asl, N.1 – 230 m asl, N.2 – 230 m asl, N.3 – 1500 m asl, N.4 – 1830 m asl, N.5 – 2090 m asl, Ph.1 – 1000 m asl, Ph.2 – 1450 m asl, Tz.1 – 240 m asl, Tz.2 – 1025 m asl, Th.1 – 700 m asl, Th.2 – 795 m asl, Th.3 – 1100 m asl, Th.4 – 1700 m asl. These data were sourced from the published literature, online databases including Kew Seed Information Database, Africa Tree Database and JSTOR Global Plants, as well as unpublished data from author contributions.
Faunal composition
Faunal assemblage structure and body size change along altitudinal gradients in response to reduced ecosystem productivity – diets become less diverse and of lower quality and quantity (Figure 1), and the relative abundance and diversity of small and large mammals (Heaney et al. Reference HEANEY, HEIDEMAN, RICKART, UTZURRUM and KLOMPEN1989, Ingle Reference INGLE2003, Steinmetz et al. Reference STEINMETZ, CHUTIPONG, SEUATURIEN and CHIRNGSAARD2008) and birds (Dehling et al. Reference DEHLING, FRITZ, TÖPFER, PÄCKERT, ESTLER, BÖHNING-GAESE and SCHLEUNING2014, Ferger et al. Reference FERGER, SCHLEUNING, HEMP, HOWELL and BÖHNING-GAESE2014, Terborgh Reference TERBORGH1977) shifts towards smaller-bodied species (Rodríguez et al. Reference RODRÍGUEZ, OLALLA-TÁRRAGA and HAWKINS2008). Large-mammal assemblages are less species rich and have lower densities with increasing altitude. Elephants and tapirs drop out of TMF with altitude (Steinmetz et al. Reference STEINMETZ, CHUTIPONG, SEUATURIEN and CHIRNGSAARD2008), there are fewer primate species at upper elevations in African (Goodman & Rasolonandrasana Reference GOODMAN and RASOLONANDRASANA2001, Marshall et al. Reference MARSHALL, TOPP-JØRGENSEN, BRINK and FANNING2005), Asian (Caldecott Reference CALDECOTT1980, Grow et al. Reference GROW, GURSKY and DUMA2013) and South American TMF (Almeida-Neto et al. Reference ALMEIDA-NETO, CAMPASSI, GALETTI, JORDANO and OLIVEIRA-FILHO2008, Geise et al. Reference GEISE, PEREIRA, BOSSI and BERGALLO2004, Shanee Reference SHANEE2009, Reference SHANEE, Defler and Stevenson2014), and fewer fruit bats in TMF in the Philippines (Hamann & Curio Reference HAMANN and CURIO1999), Peruvian Andes (Patterson et al. Reference PATTERSON, PACHECO and SOLARI1996) and oceanic Africa (Goodman & Rasolonandrasana Reference GOODMAN and RASOLONANDRASANA2001). Primates (Grow et al. Reference GROW, GURSKY and DUMA2013), fruit bats and birds (Figure 4) are all generally smaller bodied at high altitudes. Rodents also decline in species richness with increasing altitude, with major changes occurring at ecotones between lowland and montane forest (Willig & Presley Reference WILLIG and PRESLEY2016). However, small-mammal densities tend to peak everywhere in mid-elevation forest (Heaney Reference HEANEY2001, McCain Reference MCCAIN2004, Moreira et al. Reference MOREIRA, MANDUCA, GONÇALVES, MORAIS, PEREIRA, LESSA and DERGAM2009), the mid-domain effect (Colwell & Lees Reference COLWELL and LEES2000). In addition, at higher altitudes primate species switch diets from a predominantly fruit diet to a more mixed diet (Fourrier Reference FOURRIER2013, Gross-Camp et al. Reference GROSS-CAMP, MASOZERA and KAPLIN2009, Ramírez et al. Reference RAMÍREZ, MÓNICA, GALVIS, VARGAS, LÉON, CIFUENTES, STEVENSON, Grow, Gursky-Doyen and Krzton2014, Twinomugisha et al. Reference TWINOMUGISHA, CHAPMAN, LAWES, WORMAN, DANISH, Newton-Fisher, Notman, Reynolds and Paterson2006, Yamagiwa & Basabose Reference YAMAGIWA and BASABOSE2006) so that overall, at higher altitudes ornithochory (avian frugivory) is prominent and far less seed is removed by primates (Gonzales & Stevenson Reference GONZALEZ, STEVENSON, Potoki and Krasinski2009).
The increase in relative frequency of frugivorous birds versus mammals with increasing altitude (Figure 1) may in part be due to the generally smaller body size (and therefore nutritional needs) of birds but also because the temporal fruit availability in TMF leads to downslope migration of avian frugivores in times of fruit scarcity (Cox Reference COX2010, Leighton & Leighton Reference LEIGHTON, LEIGHTON, Sutton, Whitmore and Chadwick1983, Loiselle & Blake Reference LOISELLE and BLAKE1991, Wright Reference WRIGHT, Dew and Boubli2005). This more general finding contrasts with Kimura et al. (Reference KIMURA, YUMOTO and KIKUZAWA2001), who report more constant fruit production in montane forests, relative to lowland forests of Mt. Kinabalu, Borneo. This may contribute to explanations for the greater relative abundance of birds year-round in this locale. However untangling cause and effect is complicated as an increase in the relative proportion of birds versus mammals at higher elevations may select for smaller, fleshy fruit (Duivenvoorden & Cuello Reference DUIVENVOORDEN and CUELLO2012, Lord et al. Reference LORD, EGAN, CLIFFORD, JURADO, LEISHMAN, WILLIAMS and WESTOBY1997).
DISPERSAL ECOLOGY AND COMMUNITY STRUCTURE AND DYNAMICS
Here we review how dispersal ecology influences the community ecology of TMF. The paucity of information on this aspect evokes the need for further research.
Shorter dispersal distances and restricted seed shadows
While dispersal mode is not a strong predictor of dispersal distance in TLF (Clark et al. Reference CLARK, POULSEN, BOLKER, CONNOR and PARKER2005, Muller-Landau et al. Reference MULLER-LANDAU, WRIGHT, CALDERÓN, CONDIT and HUBBELL2008), large-bodied frugivores do tend to disperse seeds farther away from the parent tree than small-bodied ones (Jordano et al. Reference JORDANO, GARCIA, GODOY and GARCIA-CASTANO2007), and small passerine birds mostly disperse seeds only relatively short distances (Breitbach et al. Reference BREITBACH, BÖHNING-GAESE, LAUBE and SCHLEUNING2012, Jordano et al. Reference JORDANO, GARCIA, GODOY and GARCIA-CASTANO2007, Murray Reference MURRAY1988). Therefore, the distance seeds are dispersed should theoretically decline at the community level with increasing altitude in TMF. We found no published evidence for differences in movement range of primates between TMF and TLF, corroborating the findings of Chapman & Russo (Reference CHAPMAN, RUSSO, Campbell, Fuentes, MacKinnon, Panger and Bearder2007). However, the general finding that more of the available endozoochorous seeds are dispersed by passerine birds than primates in TMF points to relatively more seeds experiencing shorter gut passage times and shorter dispersal distances in TMF than TLFs. This hypothesis needs testing.
Dispersal
Several factors suggest that seed dispersal patterns should differ in a predictable way between TMF and TLF. Dispersal distances vary with frugivore species, so that fewer seed dispersing species in TMF should result in a narrower distribution of dispersal distances. The increase in smaller-bodied avian frugivores may arguably lead to more dispersal close to the parent tree in TMF relative to TLF. For reasons of propulsion and diaspore type, anemochorous diaspores tend to have shorter average dispersal distances than zoochorous ones (Augspurger Reference AUGSPURGER1986, Greene & Johnson Reference GREENE and JOHNSON1989, Nathan Reference NATHAN2006); given the relatively high proportion of anemochory in TMF this should also result in more dispersal closer to the parent tree in TMFs than LTFs (Figure 1).
Steeper slopes in TMF than TLF may cause more seeds to be washed downhill, away from the parent tree. Again, this aspect of TMF dispersal ecology has not received any attention – the only empirical investigation we found on clumping in TMF was from Ngel Nyaki forest in Nigeria where, at 1600 m asl, Matthesius (Reference MATTHESIUS2006) found that two tree species groups tested had a greater number of conspecific nearest neighbours than would be expected to occur by chance alone.
Post-dispersal seed fate
Predation/dispersal balance. Very little has been published on the predation/dispersal balance in TMF despite the dependence of many tropical forest trees on secondary dispersal (Aliyu et al. Reference ALIYU, ADAMU, MOLTCHANOVA, FORGET and CHAPMAN2014). Post-dispersal seed fate strongly affects forest regeneration (Wright Reference WRIGHT2003). Seed and seedling predators can act as selective filters to recruitment and establishment. Seed predators include mammals, birds and invertebrates but sometimes these predators inadvertently act as dispersers by moving but not immediately consuming seeds. This is particularly significant in the case of large nutrient-rich seeds which are sometimes secondarily dispersed by rodents through seed caching or scatterhoarding. The fate of cached/buried seeds will depend in part on rodent densities and overall fruit abundance. When overall fruit abundance is low, the ratio of predation to scatterhoarding or caching increases (Forget Reference FORGET1996) so that the potential for secondary dispersal declines. As overall fruit abundance is lower in TMF than in TLF and small-mammal densities increase up to mid-altitude forests, we predict higher predation/dispersal ratios in TMF than in TLFs. Meng et al. (Reference MENG, GAO, CHEN and MARTIN2012) found indirect evidence to support this hypothesis – higher densities of rodents in montane than lowland forest in southern China increased seed predation of experimental wild banana seeds. Even stronger support comes from Cannon (Reference CANNON2001). Cannon (Reference CANNON2001) found that some Asian Lithocarpus species, that typically have a well defended acorn, had a more extensive cupule than others and that these well defended species were more common in montane than lowland forest. The latter suggests greater selection for protection from predators at higher elevation (but see Chen et al. Reference CHEN, CANNON and CONKLIN-BRITTAN2012, Payne et al. Reference PAYNE, FRANCIS and PHILLIPS1985). Seed predation can be greater at higher altitudes, for example, an experiment using Carapa procera seeds in a Rwandan montane forest above 2000 m asl resulted in almost 100% predation, mainly by the African pouched rat (Cricetomys spp.) (Nyiramana et al. Reference NYIRAMANA, MENDOZA, KAPLIN and FORGET2011). In contrast, a similar experiment in Nigerian TMF at 1600 m asl found the dispersal probabilities for Carapa oreophylla seeds by Cricetomys spp. were close to those recorded from lowland Neotropical Carapa guianensis (Aliyu et al. Reference ALIYU, ADAMU, MOLTCHANOVA, FORGET and CHAPMAN2014). However, in a lean fruiting year at the same site almost 100% C. oreophylla seeds were predated (Chapman & Godwin unpubl. data). We hypothesize that higher predation rates of dispersed seeds in TMF lead to greater dispersal and recruitment limitation at higher altitudes than in TLFs.
Density-dependent mortality (DDM). Light, herbivory and pathogens exert strong density-dependent effects on seed and seedling survival and can affect plant species diversity in tropical forests. While a reasonable hypothesis is that DDM is less prevalent in TMF relative to TLF because TMF are less light-limited, have fewer herbivores and pathogens and are colder, more nutrient-poor environments (Xu & Yu Reference XU and YU2014), this needs testing. For example, Comita et al. (Reference COMITA, MULLER-LANDAU, AGUILAR and HUBBELL2010) found that common species are not as susceptible to DDM as rare species, a finding which supports our hypothesis because TMF tree diversity is often dominated by a few species, especially at higher-elevations (Martin et al. Reference MARTIN, FAHEY and SHERMAN2011). In contrast, Zhu et al. (Reference ZHU, WOODALL, MONTEIRO and CLARK2015) found that DDM was stronger for common than rare species. Lambers et al. (Reference LAMBERS, CLARK and BECKAGE2002) found that temperate forests have equally strong DDM as tropical forests. Studies within TMF also present equivocal findings. For example, Wenny (Reference WENNY2000) found no evidence of distance dependency in either seeds or seedlings of Ocotea endresiana in Costa Rican montane forest. In a Nigerian montane forest, only the seedling survival of Pouteria altissima, one of six species examined, displayed strong distance and density effects to herbivory (Matthesius et al. Reference MATTHESIUS, CHAPMAN and KELLY2011). In a ballistochorous species Philenoptera sutherlandii, Boudreau & Lawes (Reference BOUDREAU and LAWES2008) found no evidence for either density- or distance-dependent seedling survival (i.e. typically dense seedling populations in the canopy shade of parent trees) in an Afrotemperate forest. They found a hump-shaped dispersal kernel with predator satiation at high seedling densities near the parent tree.
A strong test of whether DDM is more important in TMF than TLF is to compare low and high elevation sites – only one field study has done this (Xu &Yu Reference XU and YU2014). In submontane China, Xu & Yu (Reference XU and YU2014) found lower seedling mortality due to soil-borne pathogens and less DDM among conspecific seedlings at high-elevation than low-elevation sites. Clearly, the extent to which seed dispersal accounts for differences between TMF and TLF in community structure and dynamics remains uncertain. Our proposition is that TMF communities may have better community resilience to disturbance, because of their small, light-adapted seeds, tolerance of low soil fertility and their dependence on small rather than large frugivores for dispersal.
DISPERSAL SYNDROMES CHANGE WITH ISOLATION AND BIOGEOGRAPHIC HISTORY
Biogeographic constraints rather than environmental gradients can influence dispersal ecology in TMF. For example, the dominance of anemochory in some oceanic island TMFs is enforced by their isolation e.g. Hawaii (Carlquist Reference CARLQUIST1966), Pacific cloud forest (Merlin & Juvik Reference MERLIN, JUVIK, Hamilton, Juvik and Scatena1995) and Galapagos (Kitayama & Itow Reference KITAYAMA and ITOW1999). In addition, due to constraints on dispersal from mainland sources, isolated islands often lack sufficient frugivores for seed dispersal and plants have thus evolved other dispersal strategies (Wheelwright & Orians Reference WHEELWRIGHT and ORIANS1982). Biogeographic constraints also apply to continental islands; for example, zoochorous oaks, which dominate TMFs throughout much of Central America (Kappelle et al. Reference KAPPELLE, CLEEF and CHAVERRI1992) are absent from the TMF of the Greater Antilles (Asprey & Robbins Reference ASPREY and ROBBINS1953, Martin et al. Reference MARTIN, SHERMAN and FAHEY2007), presumably because oak seeds have not been able to reach these islands. Moreover, other than rodents and bats, Greater Antillean forests also lack most mainland mammalian dispersers present in Central America.
EFFECTS ON DISPERSAL ECOLOGY DUE TO LOSS OF FRUGIVORES
Tropical montane forests have historically been and continue to be altered by agriculture and the harvesting of the natural resources they contain. Demand for agriculture has converted vast areas into fragmented landscapes, while resource extraction, which includes logging for valuable timber and hunting for animals, has altered forest habitats. As an example of forest loss, between 1981 and 1990, a total of 2.5 million ha of TMF was destroyed at an estimated rate of 1.1% annually (FAO 1993). Unsustainable hunting has also taken its toll, such as in the cases of Kilum-Ijim Forest in Cameroon and the Taraba State forests in Eastern Nigeria where the elephant has been extirpated and a number of other large, seed-dispersing mammal species have been severely reduced in abundance (Chapman et al. Reference CHAPMAN, OLSEN and TRUMM2004, Maisels et al. Reference MAISELS, KEMING, KEMEI and TOH2001). The consequent defaunation of TMFs may cause a trophic cascade affecting other frugivores, altering the seed-dispersal capacity, as well as the structure and composition of TMFs.
Forest loss, fragmentation and hunting in TMFs have predictable negative effects on frugivorous animals. Large, fruit-eating vertebrates often decline with increasing fragmentation in Neotropical (Harcourt et al. Reference HARCOURT, COPPETO and PARKS2002, Renjifo Reference RENJIFO1999), African (Harcourt et al. Reference HARCOURT, COPPETO and PARKS2002, Newmark Reference NEWMARK1991) and Asian TMF (Harcourt et al. Reference HARCOURT, COPPETO and PARKS2002, Pattanavibool & Dearden Reference PATTANAVIBOOL and DEARDEN2002). This guild of large-bodied species is also targeted for food by hunters, and include African elephant in central Africa (Maisels et al. Reference MAISELS, KEMING, KEMEI and TOH2001), chimpanzee in the mountains of Nigeria (Knight et al. Reference KNIGHT, CHAPMAN and HALE2015), elephant, bushpig and primates in eastern Africa (Topp-Jørgensen et al. Reference TOPP-JØRGENSEN, NIELSEN, MARSHALL and PEDERSEN2009), primates in Yunnan, China (Jiang et al. Reference JIANG, LUO, ZHAO, LI and LIU2006), Asian elephant in northern Thailand (Pattanavibool & Dearden Reference PATTANAVIBOOL and DEARDEN2002), bushpig in the Philippines (Scheffers et al. Reference SCHEFFERS, CORLETT, DIESMOS and LAURANCE2012) and primates and birds in the Andes (Shanee Reference SHANEE2012). Among other factors, large body size and low densities in a landscape with limited food resources predispose large frugivorous vertebrate species to local extinction or declines in abundance in response to these pressures. In some cases, these pressures may act in synergy to deplete species. For example, in northern Thailand, the loss of the Asian elephant and large frugivorous birds and mammals in Mae Tuen Wildlife Sanctuary was attributed to the combined effects of forest fragmentation and hunting pressure (Pattanavibool & Dearden Reference PATTANAVIBOOL and DEARDEN2002). In contrast to more predictable losses of larger vertebrates, smaller-bodied frugivores are hunted less (but see Scheffers et al. Reference SCHEFFERS, CORLETT, DIESMOS and LAURANCE2012). In fragmented landscapes, frugivorous passerines in the understorey appear less sensitive to fragmentation (Newmark Reference NEWMARK1991). Defaunation, whether by hunting, fragmentation or habitat loss, produces predictably impoverished and small-bodied faunal assemblages (Canale et al. Reference CANALE, PERES, GUIDORIZZI, GATTO and KIERULFF2012, Dirzo et al. Reference DIRZO, YOUNG, GALETTI, CEBALLOS, ISAAC and COLLEN2014).
Understanding the cascading effects of defaunation is paramount in TMF. A growing body of evidence has linked the loss or reduced densities of large frugivores in modified landscapes with a decrease in seed dispersal of large-seeded species (Kurten Reference KURTEN2013, Markl et al. Reference MARKL, SCHLEUNING, FORGET, JORDANO, LAMBERT, TRAVESET, WRIGHT and BÖHNING-GAESE2012). In a meta-analysis of 35 studies, Markl et al. (Reference MARKL, SCHLEUNING, FORGET, JORDANO, LAMBERT, TRAVESET, WRIGHT and BÖHNING-GAESE2012) demonstrated that hunting and selective logging were the most important drivers of reduced seed dispersal, especially for large-seeded species. Seed dispersal in fragmented forests, however, did not show the same result, and this is likely because either effective dispersers are unaffected by fragmentation (Cramer et al. Reference CRAMER, MESQUITA and WILLIAMSON2007, Graham et al. Reference GRAHAM, MARTÍNEZ-LEYVA and CRUZ-PAREDES2002) or, as with one Kenyan montane forest, potentially replaced by matrix species such that seed dispersal increases (Farwig et al. Reference FARWIG, BÖHNING-GAESE and BLEHER2006). The latter has also been observed in southern African montane forests (Lawes et al. Reference LAWES, JOUBERT, GRIFFITHS, BOUDREAU and CHAPMAN2007, Wethered & Lawes Reference WETHERED and LAWES2003). In another review of 42 studies, Kurten (Reference KURTEN2013) further confirmed the finding that large-seeded plant species are affected by reduced dispersal rates when frugivores are depleted. However, comparatively smaller-seeded species are often not affected by defaunation (Cramer et al. Reference CRAMER, MESQUITA and WILLIAMSON2007), and this suggests that other plant traits need to be considered when studying seed dispersal mutualisms in disturbed tropical forests.
All of the few studies conducted in TMF that evaluate the effects of habitat modification on frugivores and seed dispersal have found a reduction in primary dispersal. Cordeiro & Howe (Reference CORDEIRO and HOWE2003), Cordeiro et al. (Reference CORDEIRO, NDANGALASI, MCENTEE and HOWE2009) and Lehouck et al. (Reference LEHOUCK, SPANHOVE, COLSON, ADRINGA-DAVIS, CORDEIRO and LENS2009) have demonstrated a reduction in numbers of primary avian dispersers in response to fragmentation and habitat disturbance within fragments respectively, while Cochrane (Reference COCHRANE2003) attributed the extirpation of the African elephant to habitat loss and persecution. One of the indirect consequences of reduced dispersal is an increase in seedling densities under parents, which through density-dependent mortality effects, reduces overall recruitment (Cordeiro et al. Reference CORDEIRO, NDANGALASI, MCENTEE and HOWE2009, see also Galetti et al. Reference GALETTI, DONATTI, PIRES, GUIMARAES and JORDANO2006). Consequently, defaunated forests tend to support fewer tree species (Kurten Reference KURTEN2013). Loss of larger frugivores can sometimes favour smaller-sized species, which increase in density, e.g. primates in lowland Peru (Nuñez-Iturri & Howe Reference NUÑEZ-ITURRI and HOWE2007). Smaller-seeded species, some of which are early successional, usually possess fruits dispersed by large assemblages of vertebrates and may persist in human-dominated landscapes (Laurance et al. Reference LAURANCE, NASCIMENTO, LAURANCE, ANDRADE, FEARNSIDE, RIBEIRO and CAPRETZ2006). These species may be advantaged by the shrinking population of rarer species and increase their dominance in the tree communities at both local and regional scales (Tabarelli et al. Reference TABARELLI, PERES and MELO2012). In TMF lacking seed dispersers, an important issue is whether or not failed recruitment (Cordeiro et al. Reference CORDEIRO, NDANGALASI, MCENTEE and HOWE2009, Lehouck et al. Reference LEHOUCK, SPANHOVE, COLSON, ADRINGA-DAVIS, CORDEIRO and LENS2009) or increased seed dispersal (Farwig et al. Reference FARWIG, BÖHNING-GAESE and BLEHER2006), as deduced from single-species studies conducted in disturbed forests, offer insights into potential community-wide effects. Lessons learned mostly from TLF studies on defaunation and the effects on seed dispersal and recruitment processes (Corlett Reference CORLETT2007, Effiom et al. Reference EFFIOM, NUÑEZ-ITURRI, SMITH, OTTOSSON and OLSSON2013, Reference EFFIOM, BIRKHOFER, SMITH and OLSSON2014; Nuñez-Iturri & Howe Reference NUÑEZ-ITURRI and HOWE2007, Terborgh et al. Reference TERBORGH, NUÑEZ-ITURRI, PITMAN, VALVERDE, ALVAREZ, SWAMY, PRINGLE and PAINE2008), suggest that forest trees and other plant communities will shift toward less species-rich communities dominated by a few species.
FUTURE RESEARCH
This review of the seed-dispersal ecology of TMFs highlights the need for more research to manage and conserve these important ecosystems. Data are available from only a select few African and Neotropical montane forests, and there is barely any information from Asian TMFs. Some fundamental trends, such as why diaspore size decreases above ~1000–1500 m asl in the tropics, are not understood. Further evaluation of plant dispersal ecology along altitudinal gradients and our ability to manage TMFs will benefit from consideration of changes in diaspore size of the same species (plasticity of phenotypic response) with altitude. In addition, network analyses that examine the structure and stability of frugivore–plant food webs need to be constructed to understand how these networks function and to identify vulnerable links. The influences on seed fate and recruitment rates from seeds in TMF is another area that needs more attention. Most studies remove seeds and place them in optimal conditions to determine germination rates, but few follow seed fate in the field to determine recruitment rates, and even fewer are long-term studies. While it is assumed that more seed dispersal translates into more recruitment and the maintenance of plant diversity in forest ecosystems, the fact is that this assumption has seldom been challenged. Is seed dispersal on its own sufficient to ensure the persistence of TMF? Or, are the subtle interactions between seed dispersers, seed type and the maintenance of suitable microsites in properly functioning TMF ecosystems more important? For example, in one TLF at Barro-Colorado, Panama, Muller-Landau et al. (Reference MULLER-LANDAU, WRIGHT, CALDERÓN, CONDIT and HUBBELL2008) found that seed mass and tree height each contributed more to longer seed dispersal distance than did the dispersal syndrome. No such tests have been carried out in TMF, but could greatly improve our understanding of the factors that contribute most to TMF dynamics. Is seed dispersal a more deterministic and important process than we have previously appreciated?
ACKNOWLEDGEMENTS
The authors are grateful for valuable suggestions from Peter Bellingham, Colin Chapman and Patrick Martin, as well as anonymous reviewers.