Introduction
Cerberiopsis candelabra (common name, Candelabre; Apocynaceae) is a monocarpic (= semelparous, reproducing once before dying) tree, endemic to rain forests of New Caledonia in the southwest Pacific. It is large, commonly c. 30 m high, and potentially long-lived, with ages often exceeding 100 y. Flowering is commonly synchronous, with many trees of a population flowering together (Veillon Reference Veillon1971), often coincident across many populations (Read et al. Reference Read, Sanson, Jaffré and Burd2006). This flowering behaviour, relatively unusual in a large, long-lived tree (Foster Reference Foster1977, Thomas Reference Thomas, Meinzer and Dawson2011), may negatively impact population demography and persistence. In particular, abundance may decline if seed release from a mass-flowering event (i.e. a masting event) does not coincide with conditions suited to seedling establishment.
Previously we hypothesised that effectively continuous regeneration might occur through pulses of seedling establishment and recruitment following episodes of mass flowering and parental death (Read et al. Reference Read, Sanson, Burd and Jaffré2008). However, no evidence was found of the reverse-J curves of tree frequency versus size that are expected in continuously regenerating populations (Hett & Loucks Reference Hett and Loucks1976, Ogden Reference Ogden1985), even in older populations that have experienced multiple flowering events (Read et al. Reference Read, Sanson, Burd and Jaffré2008). Instead, size structures were usually unimodal, suggesting one or more establishment events followed by recruitment failure and predicting population decline (Read et al. Reference Read, Sanson, Burd and Jaffré2008). However, size distributions are not always good predictors of population trends (Condit et al. Reference Condit, Sukumar, Hubbell and Foster1998, Feeley et al. Reference Feeley, Davies, Noor, Kassim and Tan2007, Ogden Reference Ogden1985), and population persistence can be difficult to assess without long-term monitoring of dynamics and growth (Condit et al. Reference Condit, Sukumar, Hubbell and Foster1998, Newbery et al. Reference Newbery, van der Burgt, Worbes and Chuyong2013).
Demographic patterns and persistence are potentially influenced by the controls of flowering onset (Jacquemyn et al. Reference Jacquemyn, Brys and Jongejans2010). Some monocarpic perennials, including some bamboos (Janzen Reference Janzen1976), are ‘periodical’, that is, flowering occurs at a fixed time after germination of more than 2 y and is nearly completely synchronous (Kakashima et al. Reference Kakishima, Yoshimura, Murata and Murata2011, Reference Kakishima, Liang, Ito, Yang, Lu, Okuyama, Hasebe, Murata and Yoshimura2019). Other monocarpic perennials have non-periodical flowering, with or without flowering synchrony. In long-lived species, flowering onset could potentially affect population dynamics and long-term persistence if its likelihood is higher at particular plant sizes or ages, especially if synchronicity is high. For example, in a closed forest, flowering of small trees is likely to lead to recruitment only if the species is shade-tolerant, or if flowering is synchronised with conditions that open the canopy i.e. large-scale disturbances.
As for some other long-lived monocarps, including some with mass flowering (Foster Reference Foster1977), C. candelabra is not a rare species. Hence, its flowering strategy has been successful in past environments. Notably, it occurs in a climate that is relatively equable other than a 2–3-mo dry season of variable severity. Observations across recent flowering events suggest several factors that should enhance population persistence despite the risks of a once-only flowering strategy (Read et al. Reference Read, Sanson, Jaffré and Burd2006, Reference Read, Sanson, Burd and Jaffré2008). First, flowering occurs over a wide range of tree size, and probably age (Read et al. Reference Read, Sanson, Jaffré and Burd2006), which may allow greater responsiveness to environmental flowering cues. Furthermore, the large size of many flowering trees potentially increases fecundity as well as the gap size created by parental death, facilitating recruitment of the shade-intolerant offspring, but also increases the risk of dying before flowering due to the long delay (Read et al. Reference Read, Sanson, Burd and Jaffré2008). In addition, gregariousness (i.e. commonly occurring in groups) combined with synchronous flowering, and coincidence of flowering with disturbances such as cyclones or droughts (stochastically or deterministically), can further increase gap size and thereby recruitment (Read et al. Reference Read, Sanson, Burd and Jaffré2008). Finally, flowering is not completely synchronous within or across populations (Read et al. Reference Read, Sanson, Jaffré and Burd2006, Veillon Reference Veillon1971), allowing multiple regeneration opportunities within populations and regions, a potentially important safety factor.
The controls of flowering in C. candelabra are unknown, but flowering onset generally requires a state of physiological or resource competence, regulated endogenously, plus environmental cues (Bergonzi & Albani Reference Bergonzi and Albani2011, Thomas Reference Thomas2013). Environmental cues may promote population persistence if they improve reproductive efficiency by synchronising flowering, and more so if they tie the timing of reproduction to environmental conditions that enhance seedling recruitment (Kelly et al. Reference Kelly, Geldenhuis, James, Holland, Plank, Brockie, Cowan, Harper, Lee, Maitland, Mark, Mills, Wilson and Byrom2013, Pearse et al. Reference Pearse, Koenig and Kelly2016). In mass-flowering polycarps, flowering is typically entrained by a broad environmental cue, usually weather-related (Kelly et al. Reference Kelly, Geldenhuis, James, Holland, Plank, Brockie, Cowan, Harper, Lee, Maitland, Mark, Mills, Wilson and Byrom2013, Pearse et al. Reference Pearse, Koenig and Kelly2016).
Given the wide size range of flowering trees and overlap in size with non-flowering trees, flowering competence in C. candelabra seems to be influenced by factors other than size alone, such as age or growth rate (Burd et al. Reference Burd, Read, Sanson and Jaffré2006; Read et al. Reference Read, Sanson, Burd and Jaffré2008). For example, slow growth can increase the likelihood of death via competition (Brienen & Zuidema Reference Brienen and Zuidema2006, Needham et al. Reference Needham, Chambers, Fisher, Knox and Koven2020, Stephenson et al. Reference Stephenson, van Mantgem, Bunn, Bruner, Harmon, O’Connell, Urban and Franklin2011), and consequently the advantage of early flowering. However, growth trajectory may have a more complex effect through interactions with other traits such as plant size (Burd et al. Reference Burd, Read, Sanson and Jaffré2006, Young Reference Young1985). The balance between current and expected future reproduction is key to understanding the evolution of flowering strategies in monocarpic perennials (Kuss et al. Reference Kuss, Rees, Ægisdóttir, Ellner and Stöcklin2008). Taking into account the dependence of mortality on growth, and the scaling of flower abundance with plant size, the model of Burd et al. (Reference Burd, Read, Sanson and Jaffré2006) predicts a wide range of optimal sizes for flowering in C. candelabra. These predictions are consistent with the wide size range of flowering trees (Read et al. Reference Read, Sanson, Jaffré and Burd2006).
Here, we seek a better understanding of population persistence in C. candelabra, focussing on regeneration dynamics and the factors controlling flowering onset. We investigate (1) whether age structures, based on age estimates from tree rings, confirm population decline, and (2) whether the timing of tree establishment or climate records indicate a potential environmental cue for flowering, for example cyclones or droughts. In addition, we use age estimates and measurements of growth rate over a 7–14-y period to explore the influence of age and growth trajectory on flowering. We focus on a flowering event in 2017, the only major event since 2003 to our knowledge, asking (3) whether flowering is more strongly associated with tree age or growth trajectory than tree size; in particular, does optimal size for flowering depend on an individual plant’s growth trajectory, as predicted by Burd et al. (Reference Burd, Read, Sanson and Jaffré2006)? We investigate two predictions derived from the models of Burd et al. (Reference Burd, Read, Sanson and Jaffré2006): (a) slow growers should flower at a narrow range of small size, fast growers should flower at a narrow range of large size, and plants with intermediate growth rates should flower across a wide size range from small to large; and (b) non-flowering trees that are larger than flowering ones should also have higher growth rates. These patterns are robust qualitative predictions from the several growth functions and range of parameter values considered by Burd et al. (Reference Burd, Read, Sanson and Jaffré2006). Both predictions arise because size and growth rate account for a tree’s immediate reproductive prospects as well as its likely future fecundity if flowering is delayed, but with a risk of mortality before the next flowering event.
Methods
This study focuses on Cerberiopsis candelabra Vieill. var. candelabra (henceforth referred to as C. candelabra), which occurs in the central mountain ranges and southern massif of the main island of New Caledonia. C. candelabra var. vexillaria (Däniker) Boiteau, a small tree with a restricted distribution and known from only two collections (Boiteau & Allorge Reference Boiteau and Allorge1981), is not considered here. We report on 14 of the 18 study sites established in 2004 (Read et al. Reference Read, Sanson, Jaffré and Burd2006), plus a site established in 2008–2009 (Pic Mouirange; Table 1, Figure 1). Mean annual rainfall of the study sites is c. 1700–3000 mm (Météo France de Nouvelle-Calédonie: rainfall stations or from isohyet maps), with an annual mean maximum temperature of 25.7°C (WorldClim v. 1.4 (Hijmans et al. Reference Hijmans, Cameron, Parra, Jones and Jarvis2005); 30 arc seconds resolution with DIVA-GIS v. 7 (Hijmans et al. Reference Hijmans, Guarino and Mathur2012)). All sites are on ultramafic (serpentine) soils, which cover a third of the island (Isnard et al. Reference Isnard, L’Huillier, Rigault and Jaffre2016), with low concentrations of P, K and Ca, and high levels of potentially toxic and bioavailable metals, including Ni (Read et al. Reference Read, Sanson, Burd and Jaffré2008). The Tontouta sites lie northwest of other sites (Figure 1) and differ by their river-edge location.
Table 1. Summary of study sites. The data comprise the site location, total stand basal area (trees ≥ 20 cm DBH) and percentage contribution by Cerberiopsis candelabra (trees ≥ 20 cm DBH, unless otherwise indicated) before and after the 2003 flowering event, and the canopy height (modified after Read et al. Reference Read, Sanson, Burd and Jaffré2008). Canopy height is the average of 5-10 measurements of the main canopy, with the average height of the five tallest C. candelabra trees in brackets. All sites were monitored from 2004, except Pic Mouirange which was established in 2008-2009. Pic Mouirange, Col de Mouirange and Forêt Nord 2 were not monitored in 2018, but age estimates of C. candelabra and Agathis lanceolata are included from these sites. nf, no flowering was recorded on the site; na, data not available.

a Plot size in 2004-2007. Some plots were extended in area in 2007-2018 to include more live trees.
b Calculated using trees of ≥10 cm DBH because of the generally small tree size.

Figure 1. Map of New Caledonia showing study site locations. The enlarged section of the south of the main island shows the peridotite massif in grey. Study sites (triangles) are as follows: 1, Tontouta sites in the Tontouta River valley; 2, west to east, Mouirange, Pic Mouirange, Col de Mouirange, Col de Deux Tétons, Bois du Sud; 3, Col de N’Go sites; 4, north to south, Aerodrome 2, Pic du Grand Kaori, Forêt Stephane, Forêt Nord 1, Forêt Nord 2; 5, Kuebini.
Population age structures and age differences of flowering versus non-flowering trees were investigated by analysis of growth rings in trunk increment cores. In 2007, 14C dating was undertaken to determine whether growth rings of C. candelabra were laid down annually and could be used to determine tree age. Increment cores were taken from a trunk of C. candelabra from two sites (Mouirange and Aerodrome 2) and from a trunk of Agathis lanceolata Lindl. ex Warb. (Araucariaceae) at Mouirange where it grew with C. candelabra. A. lanceolata commonly has annual rings and a long lifespan (Read & Jaffré Reference Read and Jaffré2013, Vincent et al. Reference Vincent, Genthon, Stievenard, Nasi and Masson-Delmotte2007) and so can potentially provide a longer site chronology and thereby an insight into conditions under which C. candelabra established. Two wood samples were taken from each of the three increment cores, estimated by ring counts to date at 1958 and 1971. Alpha cellulose extracted from these samples (Hua et al. Reference Hua, Barbetti, Zoppi, Fink, Watanasak and Jacobsen2004) was dated by radiocarbon using the STAR AMS Facility at the Australian Nuclear Science and Technology Organisation (Hua et al. Reference Hua, Jacobsen, Zoppi, Lawson, Williams, Smith and McGann2001, Fink et al. Reference Fink, Hotchkis, Hua, Jacobsen, Smith, Zoppi, Child, Mifsud, van der Gaast, Williams and Williams2004). The radiocarbon results were converted to calendar ages using the bomb radiocarbon (14C) data for the Southern Hemisphere zone 1–2 (Hua et al. Reference Hua, Barbetti and Rakowski2013) and CALIBomb program (Reimer & Reimer Reference Reimer and Reimer2004).
Since rings appeared to be close to annual in these samples (see Results), trunk increment cores were collected in 2007 (with a small number collected in 2005) from most or all live C. candelabra saplings and trees ≥1.3 m high at five permanent plots (Aerodrome 2, Bois du Sud, Mouirange, Tontouta 2 and Tontouta 3). Full stem sections (cookies) were cut in the smallest trees. In 2008, DBH (diameter at breast height, 1.3 m above ground) was measured at the new site, Pic Mouirange, in all C. candelabra individuals ≥1.3 m high in two parallel 50 × 5 m transects, 20 m apart. Increment cores were collected from the nearest C. candelabra tree at 4 m intervals along the transects (n = 30). Cores were also taken from 2–3 of the largest and smallest trees (total of 4–6 cores) at all other sites except Pic du Grand Kaori to estimate the age range of C. candelabra trees. Cores were extracted using a 40-cm SUUNTO (Vantaa, Finland) increment borer (5 mm diameter) at c. 1–1.3 m above the base of the tree. Generally, two cores were extracted per tree (sometimes only one, through the full diameter), with the second core extracted perpendicular to, and 2–5 cm above, the first core. Cores were mounted in supports, sanded to 1200–1500 grit, and digitally scanned, usually at 2400 dpi.
Images were imported into WinDENDRO Version 6.4a (Regent Instruments Inc., Quebec, Canada) or CooRecorder 9.0.1 (Cybis Electronik & Data AB, Saltsjöbaden, Sweden) and growth rings were counted. Cross-dating was not possible in many trees (see Results), so estimates are based on raw ring counts, averaged per tree from up to 4 radii. Where cores did not contain pith, estimates of rings to centre were made by the software based on the geometry of innermost rings contained in the core. After discarding cores in which rings were not sufficiently distinct for counting, or where a significant number of rings were missing due to rot, cores and cookies from 264 C. candelabra trees were analysed. Age at coring height was estimated from monitoring growth of tagged seedlings that established below a relatively open canopy at Forêt Nord 2 after the 2003 cyclone and mass-flowering event (Read et al. Reference Read, Sanson, Burd and Jaffré2008): these took 6 y on average to reach 1.1 m in height. Therefore, 6 y was added to ring counts to provide an estimate of age.
Our search for a climate cue that may trigger mass flowering in C. candelabra populations focuses mainly on interannual rainfall variation, given the association of El Niño-intensified dry seasons with mass flowering in some polycarpic rainforest trees (Ashton et al. Reference Ashton, Givnish and Appanah1988, Henkel & Mayor Reference Henkel and Mayor2019). In New Caledonia, effects of El Niño Southern Oscillation (ENSO) events on rainfall are strongest from mid-August to March (Moron et al. Reference Moron, Barbero and Robertson2016). Flowering in C. candelabra is typically from July to December, overlapping the peak flowering period in New Caledonia (Veillon Reference Veillon1971). However, the timing of initiation and development of flower primordia is unknown. We examine annual rainfall, dry season rainfall (September to November), rainfall of the wettest months (January to March), dry plus wet season rainfall (September to March) and annual mean maximum temperature from 1960 to 2019, based on monthly temperature and rainfall data for Yaté Mairie (22.155ºS,166.938ºE, 25 m asl, Figure 1; Metéo France). In the summer wet season, weather depressions can intensify into cyclones. Cyclones were recorded early in the year of the 1996 and 2003 flowering events, so we also searched for cyclone activity in the south or centre of the main island (http://www.meteo.nc/nouvelle-caledonie/cyclone/climatologie-des-cyclones), specifically noting the years in which “tropical cyclones” (defined as 10 min sustained winds ≥ 118 km h−1: http://www.meteo.fr/temps/domtom/La_Reunion/charte/pics/Alertes/tableau-alerte-cyclonique.html#tableaudangercyclone) occurred.
In order to investigate size and growth rates of flowering versus non-flowering trees, DBH was measured in all individuals of C. candelabra ≥ 1.3 m high, noting whether trees were alive or had flowered and died, every 1–2 y from 2004/2005 (391 trees across all sites) to 2011. Trees were re-surveyed in September-October 2018, c. 9–14 mo after the flowering event of 2017 and c. 2–6 mo after death of flowering trees. Some study sites were extended in size in 2007–2018 to increase numbers of live trees for monitoring. DBH was used as the measure of tree size and growth rate since it was more accurately measured than height. DBH and height (measured by clinometer) were strongly correlated (R = 0.868, P < 0.001, n = 169, data log-transformed) in trees at five sites (CNG-A, CDT, Mouirange, Tontouta 2 and Tontouta 3) in 2005. Growth rate, G, was calculated as annual size increment over 7–14 y (2004–2018) (King et al. Reference King, Davies, Supardi and Noor2006): G = (Xf – X0)/T, where Xf is the final DBH, X0 is the initial DBH, and T is the time interval in years. Since the rate of incremental increase can be influenced by initial size, we also calculated relative growth rate (RGR): RGR = loge(Xf/X0)/T, following Wright et al. (Reference Wright, Kitajima, Kraft, Reich, Wright, Bunker, Condit, Dalling, Davies, Díaz, Engelbrecht, Harms, Hubbell, Marks, Ruiz-Jaen, Salvador and Zanne2010). For dead trees, we assessed whether death had occurred in the 2017 flowering event or earlier. Earlier flowering was indicated by earlier surveys or by absence of fine inflorescence branchlets and greater decay of bark and wood. Densities and heights of seedlings (defined here as plants <1.3 m high) were monitored at all sites. Given their generally localized dispersion (Read et al. Reference Read, Sanson, Burd and Jaffré2008, Veillon Reference Veillon1971), seedlings were surveyed only below trees that had flowered, usually in a semicircle of 5 m radius around the base of 10 randomly selected trees.
The relationship between growth trajectory and tree size at flowering was assessed by graphical depiction of the growth rate – DBH association at the Tontouta sites. These study sites were chosen because of substantial numbers of flowering trees in 2017 and a range of small to moderately large trees (DBH of < 5 cm to 40 cm) sufficient to detect any size-related patterns.
Data analysis
Previously, we quantified the shape of C. candelabra size structures by fitting a Weibull probability density function to DBH distributions at study sites with ≥ 40 trees (Read et al. Reference Read, Sanson, Burd and Jaffré2008). This allowed us to test the prediction of continuous regeneration: a strong positive skew (the shape index c ≤ 1) is predicted for populations regenerating by repeated frequent episodes of seedling recruitment (Bailey & Dell Reference Bailey and Dell1973, Johnson & Kotz Reference Johnson and Kotz1970). The previous analysis, using DBH distributions recorded in 2004–2005, included trees that died prior to 2007–2008 when most increment cores were collected. In order to compare population size structures with age structures for the six study sites that were intensively cored, Weibull probability densities were re-calculated using 2007–2008 DBH distributions of only cored live trees.
In contrast to non-flowering trees, flowering trees were unlikely to have increased radial stem growth in the 12 mo prior to measurement, due to massive investment in reproduction, followed by death. Therefore, DBH of trees that flowered in 2017 was adjusted by adding the average annual growth increment to the measured DBH. These adjusted values were used to calculate growth rates. Nevertheless, DBH and growth rate might be underestimated in flowering trees if stem shrinkage due to tree death occurred prior to measurement (e.g. Pastur et al. Reference Pastur, Lencinas, Cellini and Mundo2007).
Pearson correlations were used to test associations among tree age, size and growth rate. T-tests were used to determine whether flowering and non-flowering trees differed in size, age and growth rate for four sites where there were at least five trees in both flowering categories. All trees alive in 2017 (flowering and non-flowering) were included in analyses of size and growth rates across sites, not only those for which ages from ring counts were available. Eight trees that flowered in 2018 were included in the flowering category. Quantitative symmetric Kulczynski (QSK) dissimilarity (Cha Reference Cha2007) was used to determine overlap in size, age and growth rate between flowering and non-flowering trees. Analysis of covariance (ANCOVA) was used to test for an effect of growth rate on flowering independent of tree size at the Tontouta sites. The fitdistrplus package (Delignette-Muller & Dutang Reference Delignette-Muller and Dutang2015) in R (R Core Team 2016) was used to fit Weibull density functions to DBH data. SYSTAT v. 13 was used for all other analyses, with a critical level of statistical significance of 5%.
Results
Of the 391 trees ≥1.3 m high that were alive and tagged in 2004–2005, 296 (76%) were alive prior to the 2017 flowering event and 51 flowered in 2017, leaving 245 (63%) alive in 2018 (Table 2). Across the extended study sites, 64 of the 385 trees flowered in 2017, representing 0–44% of live trees per site (Table 2). In late 2017–early 2018, 17 trees were killed by wildfire at CNG-A and CDT, and four trees at CNG-A flowered in 2018 after fire damage to the trunk. Eight additional trees were flowering in five study sites at the time of the 2018 survey. No flowering was recorded at the Bois du Sud site from 2003 to 2018, and no evidence of past flowering was seen at Pic Mouirange in 2008–2009 (this site was not monitored in 2018). Most dead plants ≥5 cm DBH recorded on our study sites in 2003–2018 had flowered: of the 557 dead trees recorded across all study sites (data from this study and from sites reported in Read et al. Reference Read, Sanson, Jaffré and Burd2006), 85% flowered, 5% died without flowering (crushed by a falling tree or burnt), with 10% uncertain due to long intervals between observations.
Table 2. Fate of Cerberiopsis candelabra trees alive in 2005 and monitored until 2018 in 12 study sites. The first column of data shows the number of trees alive in 2005 (all individuals ≥1 cm DBH), followed by this number expressed as a percentage of trees alive prior to the 2003 flowering event in brackets. Columns 2-4 are calculated as a percentage of trees alive in 2005. The number of flowering trees expressed as a percentage of all live trees on the extended sites prior to the 2017 flowering event is given in brackets in the third data column. A few small individuals were cut down for ring counts during the observation period and were not included in these figures
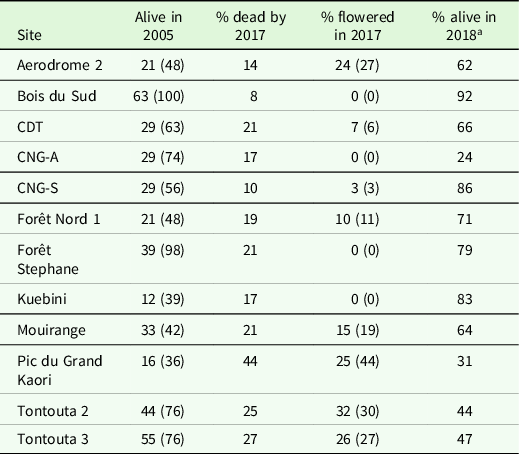
a At the time of surveying in September-October.
Associations among age, size and growth rate in C. candelabra
14C dating indicated that tree rings were very close to annual in the samples analysed: 0–3 rings were missing in C. candelabra (two trees at two sites) and 2–3 rings were missing in A. lanceolata (one tree) over c. 40 years (Appendix). Hence, ring counts were used to estimate age. However, for the trees cored in 2007–2008, within-tree cross-dating was limited and between-tree cross-dating was not possible, due to a combination of eccentric growth around stems and missing and false rings. This, plus probable variation in age to reach coring height among trees, limits accuracy of age determination. For samples from the intensively cored sites, ring counts varied within an individual tree by an average of 0 rings at Pic Mouirange (average ring count = 40), 2 rings at Tontouta 2 (average ring count = 51) and Tontouta 3 (average ring count = 55), 3 rings at Mouirange (average ring count = 104), 4 rings at Bois du Sud (average ring count = 44) and 9 rings at Aerodrome 2 (average ring count = 108).
Cored/sectioned saplings and trees of C. candelabra were 2–63 cm DBH with 13–146 rings in 2007–2008. Taking into account the estimated age to reach coring height (6 y), they established from c. 1855 to 1988 (Figure 2). The youngest tree cored (c. 19 y old) was dying after flowering in 2007 and is only included in analyses of age versus DBH. Tree age correlated strongly with DBH across all cored trees (both variables log-transformed: R = 0.793, P < 0.001; n = 265) (Figure 3). However, the correlation varied among sites: of the six sites where at least 25 trees were cored, DBH and age were not correlated at two sites (Mouirange: R = 0.042, P = 0.831; Tontouta 2: R = 0.155, P = 0.277), with moderate to strong correlations at other sites (R = 0.578–0.793, P < 0.001–0.003) (Figure 3). Nevertheless, there was often a sizable DBH range for trees of similar age. For example, at Tontouta 3, trees estimated to be 60–65 y old were 6–22 cm DBH (Figure 3). The highest estimated tree ages across plots were 152 y and 155 y in two flowering trees at Col de Mouirange and Aerodrome 2, of 38 cm and 60 cm DBH respectively. DBH was strongly positively correlated with G across all trees at the 12 sites (R = 0.531, P < 0.001; n = 385), but not with RGR (R = 0.052, P = 0.307; n = 385), suggesting the latter is a better size-independent measure of growth rate for this dataset.

Figure 2. Approximate timing of establishment of cored trees of Cerberiopsis candelabra across all study sites. For clarity of illustration, tree establishment is grouped together into 3-y classes. The black arrows indicate known tropical cyclones (10 min sustained winds ≥ 118 km h−1) in the region of the south or centre of the main island. Grey arrows indicate historic cyclone records that do not indicate sustained wind speeds. The dotted arrows indicate known flowering events in the south of the main island (Veillon Reference Veillon1971).

Figure 3. The size-age relationship of trees in forests of Cerberiopsis candelabra (log10-scale used for both axes). Tree DBH and age are given for 2007, except for Pic Mouirange which was cored and measured in 2008. A core from a 2-cm DBH tree was included in the dataset for Tontouta 3, but the tree was dying after flowering and is not included in later analyses.
Do age structures of C. candelabra indicate population decline?
Weibull density functions fitted to the size structures of C. candelabra ≥1.3 m high at six sites at the time cores were collected (2007–2008) showed no evidence of continuous regeneration, with Weibull c-values significantly >1 (Figure 4). Age structures at the same sites were unimodal, confirming this interpretation. Establishment of these trees occurred over c. 28–81 y (Figure 4) (c. 15–81 y across all study sites), followed by a substantial recruitment gap. The youngest trees ranged from c. 29 y (Bois du Sud) to 85 y (Mouirange) on sites that were intensively cored (Figure 4). Given the seedling category (< 1.3 m high, < c. 6 y old) is not included in this calculation, the age gap (recruitment gap) is c. 23–79 y. At sites where fewer trees were cored but included the smallest individuals in 2007, the recruitment gap was estimated as c. 40–67 y. Adjusting ages of surviving trees to 2018 and taking into account a small number of new recruits into the tree category on three sites in 2018, the recruitment gap across intensively cored sites was c. 36 y (Bois du Sud) to 90 y (Mouirange).
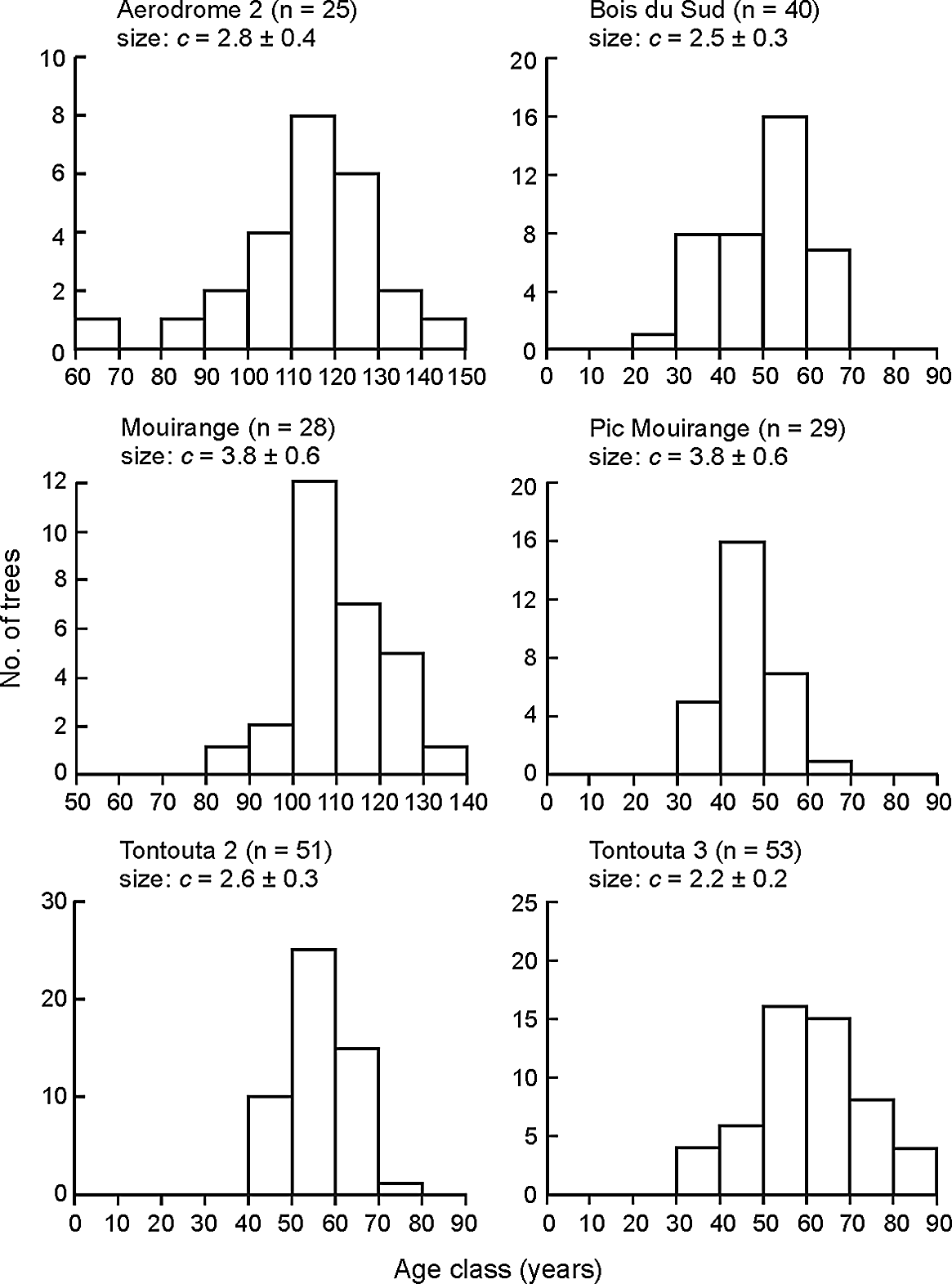
Figure 4. Population age structures of Cerberiopsis candelabra at six study sites in 2007–2008, at the time of collection of increment cores. These sites have ≥ 25 trees ≥ 1.3 m high. The shape parameter c (± SD) is given from fitting Weibull functions to the population size structures. A small number of cores were collected in 2005, for which the age was adjusted to 2007, and their DBH in 2007 used.
High seedling mortalities were common across sites. In 2004, c. 8–12 mo after fruiting from the 2003 flowering event, densities of new seedlings ranged from less than 20 per 100 m2 (8 of the 11 sites with flowering in 2003) to 326 per 100 m2 at CNG-S, dropping to near zero on all monitored sites by 2018 (Figure 5a). We were unable to access two sites (Forêt Nord 2 and St Raphael) in 2018 that had moderate to high seedling density in 2004 (Figure 5a) associated with high tree mortality and an open canopy. At CNG-A, older seedlings, probably from the 1996 flowering event, were monitored: density in 2004 was 750 seedlings per 100 m2, dropping to 10% of this number by 2018 (Figure 5a), with these tightly clustered in the gaps created by dead parents but showing no net height gain in the last 7 y (Figure 5b). In 2018, heights of new seedlings from the 2017 flowering event were similar to those recorded in 2004 from the 2003 flowering event (Figure 5b), but densities were considerably higher at the Tontouta sites, CDT and CNG-A (Figure 5a) than in 2004 (< 20 per 100 m2).

Figure 5. Changes in seedling density (a) and height (b) of Cerberiopsis candelabra from 2004 (c. 8–12 mo after fruiting: Read et al. Reference Read, Sanson, Burd and Jaffré2008) to 2018. Sites are only shown if seedling density > 20 per 100 m2 below trees that had flowered and died. Forêt Nord 2 (FN2) was not monitored in 2018. St Raphael (St R) is shown in (a) for comparison but was not monitored after 2004. For CNG-A, data are shown for older seedlings that were on site prior to the flowering event of 2003 (open points). Density and height of seedlings resulting from the 2017 flowering event (measured in 2018, c. 8–12 mo after fruiting) are shown by grey-filled points. CDT, Col des Deux Tétons; CNG, Col de N’Go; M, Mouirange; T2 and T3, Tontouta sites.
Ring counts in 2007 suggest that large trees of A. lanceolata were c. 140 y, 173–244 y and 164–184 y at Mouirange, Col de Mouirange and Forêt Nord 2, respectively, not adjusted by age at coring height. Taking into account the slower growth of juvenile A. lanceolata than C. candelabra (Read et al. Reference Read, McCoy, Jaffré, Sanson and Logan2017), these trees pre-date the largest living co-occurring C. candelabra trees by decades (maximum recorded age = 123–133 y, 133–152 y and 123–124 y, respectively).
Evidence for a proximate flowering cue from tree ages and climate records
Age determination of C. candelabra and A. lanceolata was insufficiently accurate to detect potential environmental flowering cues based on year of establishment of C. candelabra individuals. The pattern of ‘apparent’ tree establishment in Figure 2 necessarily includes only trees that survived until 2007–2008, with patterns of survival potentially obscuring those of establishment. We witnessed flowering in the region of Col de Mouirange and Pic du Grand Kaori in 1996, and evidence on study sites suggests it was widespread in the south of the island. Flowering was also widespread in the south in 2003 and 2017. Veillon (Reference Veillon1971) reported localised mass flowering in 1963–1969 in the south, in particular at Touaourou (close to Yaté) in 1969, and at Tontouta-Kalouéhola in 1967. One or more tropical cyclones were recorded in 1969, 1996, 2003 and 2017, c. 4–6 mo prior to the start of flowering (Figure 6). However, no cyclone was associated with the Tontouta-Kalouéhola event in 1967 (Figure 6). There was no clear association of flowering events with climate records for the same year (e.g. wet season rainfall) or the previous year (e.g. rainfall of the previous dry season) (Figure 6). For example, dry season rainfalls were low for the year prior to the 1996 and 2003 flowering events (191 mm and 226 mm), but moderate to high prior to other flowering events (272–416 mm) (Figure 6b). No pattern was associated with annual mean maximum temperature (Figure 6), or with differentials from the year prior to flowering, either for wet season (January–March) rainfall or monthly maximum temperatures (S1).

Figure 6. Climate variables from 1960 to 2019 for Yaté Mairie, with timing of cyclones and reported mass-flowering events in southern New Caledonia. Dashed lines with unfilled points indicate known widespread mass-flowering events (1996, 2003 and 2017) in the south of the main island, and localised mass flowering at Touaourou (close to Yaté) in 1969 and at Tontouta-Kalouéhola (grey dashed line) in 1967. Other flowering events may have occurred before 1996 that are not shown here. Arrows indicate tropical cyclones (10 min sustained winds ≥ 118 km h−1) impacting the south or centre of the main island. Data presented are total annual rainfall (a), dry season rainfall, September-November (b), wet season rainfall, January-March (c), dry season rainfall of the previous year plus wet season rainfall of the current year (d) and annual mean maximum temperature (available only from 1968) (e). Note, rainfall at Tontouta is typically lower than at Yaté (Maitrepierre Reference Maitrepierre, Bonvallot, Gay and Habert2012). Meteorological data were kindly provided by Meteo-France.
Do tree size, age and growth trajectory influence the incidence of flowering?
Flowering in C. candelabra is non-periodical: trees that flowered in 2017 were 5–60 cm DBH and 48–155 y old, compared with 4–69 cm DBH and 46–145 y old in non-flowering trees. Size, age and RGR showed moderate to high overlap between flowering and non-flowering trees on all sites, with QSK similarity values of 0.44–0.86 (Table 3). There was no significant difference in tree size, age or RGR between flowering and non-flowering trees except at Tontouta 2, where flowering trees were 1.3 times larger on average than non-flowering trees (Table 3). DBH and RGR were positively correlated in trees ≥5 cm DBH (R = 0.476, P < 0.001, n = 105) at the combined Tontouta sites. Using DBH as a covariate, RGR was lower in flowering trees (adjusted mean RGR = 0.150 ± 0.013 mm cm-1 y-1, n = 38) than non-flowering trees (adjusted mean RGR = 0.214 ± 0.010 mm cm-1 y-1, n = 67) (F = 14.7, P < 0.001). However, the difference in terms of absolute growth rate is small (0.11 cm y-1) (adjusted mean G = 0.42 ± 0.02 cm y-1 in non-flowering trees, 0.31 ± 0.01 cm y-1 in flowering trees) and within the range of possible error due to stem shrinkage after death.
Table 3. Comparisons of DBH, age and relative growth rate (RGR) of flowering and non-flowering trees for Cerberiopsis candelabra at four study sites in 2018. Study sites are included that have a minimum of five trees per category, and data are given for all trees ≥ 5 cm DBH on these sites (5.3 cm DBH was the smallest tree recorded flowering in 2017). Values are means ± SEM, with the range in brackets, plus results of t-tests. QSK, Quantitative symmetric Kulczynski similarity (converted from dissimilarity).

Graphical depiction of flowering status across a range of tree sizes at Tontouta (Figure 7) supported our two predictions about the influence of growth trajectory on optimal flowering size. (a) Slow growers tended to flower at a narrow range of small sizes (c. 5–12 cm DBH), and fast growers at a narrow range of larger sizes (c. 20–30 cm DBH), while trees with intermediate RGR flowered across a wide size range (c. 13–38 cm DBH) (clusters (a) in Figure 7; also seen using 2011 DBH and RGR data, not affected by any stem shrinkage due to post-flowering mortality, S2). (b) Large non-flowering trees tended to have higher growth rates than flowering trees of similar or smaller size (cluster (b) in Figure 7).

Figure 7. The relationship between growth rate (RGR) and DBH for flowering and non-flowering trees of Cerberiopsis candelabra ≥5 cm DBH at Tontouta study sites. Flowering trees, filled points; non-flowering trees, open points. Clusters are outlined that suggest support for the model proposed by Burd et al. (Reference Burd, Read, Sanson and Jaffré2006): see text for explanation of (a) comparison of flowering size range among groups with different growth rates (three clusters with short dashes) and (b) comparison of growth rates between flowering and non-flowering trees at a given size (long dashes). The arrow indicates a point not conforming to prediction (a).
Discussion
Regeneration dynamics of C. candelabra and population persistence
By analysing age structures and monitoring seedling mortality on permanent plots, we have shown that C. candelabra has not been regenerating continuously in recent decades and may not be persistent (sensu Connell & Lowman Reference Connell and Lowman1989) in these forests. The wide age range of trees at each site indicates multiple cohorts, as predicted. However, while growth rings were shown by carbon dating to be generally annual, ring counts for many trees differed among replicate radii, and relatively few cores could be cross-dated to improve accuracy. This inaccuracy was small at most sites, and not likely to significantly influence size-age relationships, or conclusions regarding continuous regeneration based on age structures. Estimation of age at coring height is an additional source of error, due to variation in height growth rates of seedlings within and among sites, even in open conditions where recruitment is most common. These two sources of error potentially obscure discrete establishment episodes, especially at Aerodrome 2 where within-tree variability in ring counts was highest. We assume most establishments occurred after mass-flowering events rather than occasional flowering of a few trees, given the association between seedling density and light availability (Read et al. Reference Read, Sanson, Burd and Jaffré2008) in this relatively shade-intolerant species (Read et al. Reference Read, McCoy and Jaffré2015). The exception may be where small numbers of trees flower following a disturbance, for example the wildfire adjacent to CNG-A.
Notably, the long establishment period has been followed by a recruitment gap spanning decades at each study site. Little or no flowering has been seen in two study sites (Bois du Sud and Forêt Stephane), and hence there has been no local seed input and opening of the canopy via flowering and death. The recruitment gap is more difficult to explain in other sites, where flowering was recorded in 2003 and generally also in 1996 (Read et al. Reference Read, Sanson, Burd and Jaffré2008). Seedling densities after flowering events vary across sites and also within sites after different flowering events, due in part to variation in the degree of canopy opening caused by death of flowering trees (Read et al. Reference Read, Sanson, Burd and Jaffré2008). Even when initial seedling densities are high, less than a year after fruiting, later mortality is often high, as recorded in some other masting trees (Newbery et al. Reference Newbery, Chuyong, Zimmermann and Praz2006). Although the recruitment gap appears to be largely caused by poor survival of juveniles in shaded conditions, other factors such as drought may play a role, as well as deaths of poorly competitive small/young trees in the understorey through stem-thinning processes.
The population age structures suggest C. candelabra will decline in density in mixed-canopy forests, unless future flowering follows a sufficiently large disturbance (Read et al. Reference Read, Sanson, Burd and Jaffré2008). For example, most C. candelabra trees have flowered and died in a forest at Col de Mouirange where it formed 13% of the total basal area in 1991 (Read et al. Reference Read, Sanson, Burd and Jaffré2008), with a few seedlings remaining below only two of the 50 dead parents in 2011. C. candelabra also forms monodominant forests (≥50% of canopy trees contributed by one species: Connell & Lowman Reference Connell and Lowman1989). In these forests, synchronous flowering and death of a large proportion of trees can produce substantial opening of the canopy and increase access to below-ground resources; this could lead to in situ population renewal, particularly after a cyclone in which other canopy species are damaged (Read et al. Reference Read, Sanson, Burd and Jaffré2008), that is, transient dominance (Newbery et al. Reference Newbery, van der Burgt, Worbes and Chuyong2013). Seedling densities below dead parents in 2004, less than a year after fruiting, correlated strongly with light availability and the density of flowering parents (Read et al. Reference Read, Sanson, Burd and Jaffré2008). The same trend may apply to older seedlings, with possibly higher survival at sites with high densities of flowering trees in both 1996 and 2003 and consequently more opening of the canopy (e.g. Forêt Nord 2 and St Raphael: Read et al. Reference Read, Sanson, Burd and Jaffré2008). Repeated episodes of flowering (with subsequent tree death) or disturbance may enhance recruitment of seedlings that established earlier (Brienen & Zuidema Reference Brienen and Zuidema2006, Newbery et al. Reference Newbery, van der Burgt and Moravie2004).
Establishment could also occur ex-situ, that is, by invasion (Connell & Lowman Reference Connell and Lowman1989, Newbery et al. Reference Newbery, van der Burgt, Worbes and Chuyong2013). C. candelabra fruits are winged but large, and most fall below the parent tree (Read et al. Reference Read, Sanson, Burd and Jaffré2008, Veillon Reference Veillon1971). Nevertheless, seedlings and saplings are sometimes common at forest boundaries with maquis (low evergreen shrubland) (CDT, CNG-A, Mouirange and Forêt Nord 1) or river banks (Tontouta sites), or as isolated individuals in maquis and exposed areas. New stands may develop at the periphery of populations, or from isolated individuals in open conditions, if greater resource availability (particularly of light and nutrients), including after fires and cyclones, increases growth rates and competitive success. Hence, patches of ex-situ establishment and recruitment might shift through the landscape, i.e. mosaic theory (Newbery et al. Reference Newbery, van der Burgt, Worbes and Chuyong2013), although spatial dispersion may be constrained, at least temporally, by relatively poor seed dispersal. The degree to which C. candelabra populations represent ex-situ versus in-situ establishment is uncertain. Wide within-site age ranges, plus comparison of C. candelabra ages with those of co-occurring A. lanceolata, suggest that in-situ establishment in forest has been common in the past. Based on age comparisons, A. lanceolata appears to have survived the large disturbances, probably cyclones or wildfire, that may have facilitated in situ establishment episodes of C. candelabra, as well as of co-occurring species such as Nothofagus aequilateralis (Baum.-Bod.) Steenis (Nothofagaceae) (Read & Jaffré Reference Read and Jaffré2013).
Static analyses, such as those of population age structures, reflect past environmental conditions and might not predict future population trends. The recruitment of C. candelabra to large size classes over a prolonged period in the past in all study sites suggests that the later recruitment gaps might not be the normal condition within forests, at least historically, i.e. recruitment may have declined in response to possible changes in climate or disturbance regimes. Alternatively, the historical recruitment represented in the age structures, and monodominance, may result from past anthropogenic land-use practices. Disturbance linked to prospecting, mining and logging, including wildfire, was more widespread on the southern massif from the 1870s until the mid-1900s (McCoy et al. Reference McCoy, Jaffre, Rigault and Ash1999, Stevenson & Hope Reference Stevenson and Hope2005, Valette Reference Valette2006), possibly favouring recruitment by C. candelabra during that period and subsequent dominance of some forests.
There are few data from other long-lived monocarpic trees that allow comparison of population dynamics. The monocarpic rain-forest tree Tachygali versicolor (Caesalpiniaceae, Costa Rica to Colombia) shows synchronous but incomplete mass flowering, appears to recruit well in light gaps, and seedlings may survive in the shaded understorey until canopy gaps form (Foster Reference Foster1977; Kitajima & Augspurger Reference Kitajima and Augspurger1989, Loveless et al. Reference Loveless, Hamrick and Foster1998), with high per-adult sapling recruitment (Welden et al. Reference Welden, Hewett, Hubbell and Foster1991); hence, it may persist in these forests. However, T. vasquezii (Bolivian Amazon), also monocarpic but not a mass flowerer (Poorter et al. Reference Poorter, Zuidema, Peña-Claros and Boot2005), is considered an early-successional species (Peña-Claros Reference Peña-Claros2003, Poorter et al. Reference Poorter, Zuidema, Peña-Claros and Boot2005), is relatively light-demanding and grows rapidly, living only to c. 60 y (Brienen & Zuidema Reference Brienen and Zuidema2006). The limited data suggest variability in patterns of regeneration and persistence among species. That is, there may be multiple ways to be a successful long-lived monocarpic tree.
Evidence for a proximate environmental cue for flowering
Mass flowering is common in monocarpic perennials, and may be a pre-condition for the evolution of monocarpy (Keeley & Bond Reference Keeley and Bond1999). The degree of flowering synchrony in C. candelabra can vary among and within regions, and also across flowering events. For example, in 2003, 24–94% of trees flowered at 15 of 18 study sites (Read et al. Reference Read, Sanson, Burd and Jaffré2008), more than in 2017. Flowering in C. candelabra probably involves an environmental cue, as suggested for mass-flowering polycarps (Ashton et al. Reference Ashton, Givnish and Appanah1988, Kelly et al. Reference Kelly, Geldenhuis, James, Holland, Plank, Brockie, Cowan, Harper, Lee, Maitland, Mark, Mills, Wilson and Byrom2013, Pearse et al. Reference Pearse, Koenig and Kelly2016). Its reproductive fitness may be enhanced by synchronous flowering, following the economies-of-scale hypothesis (Kelly & Sork Reference Kelly and Sork2002). For the monocarpic C. candelabra, within-population flowering synchrony has the particular benefit of synchronous death, which increases gap size and enhances offspring recruitment, at least where there are high densities of large trees (Read et al. Reference Read, Sanson, Burd and Jaffré2008). A cue that also signals a likely increase in resources is even more advantageous (Kelly et al. Reference Kelly, Geldenhuis, James, Holland, Plank, Brockie, Cowan, Harper, Lee, Maitland, Mark, Mills, Wilson and Byrom2013). For example, cyclones or droughts that cause canopy damage across many species may synchronise flowering (Henkel & Mayor Reference Henkel and Mayor2019, Hopkins & Graham Reference Hopkins and Graham1987, Pearse et al. Reference Pearse, Koenig and Kelly2016) and also signal the likelihood of increased resource availability (e.g. light and soil nutrients), i.e. the environmental-prediction hypothesis (Kelly & Sork Reference Kelly and Sork2002, Pearse et al. Reference Pearse, Koenig and Kelly2016). In this scenario, the mechanism may be some aspect of damage, such as mechanical stress or defoliation, or a linked feature, for example precipitation or nutrient input in the case of cyclones, or altered conditions of temperature, water and light associated with canopy damage (Hopkins & Graham Reference Hopkins and Graham1987). Sub-lethal fire damage also appears to trigger flowering, but C. candelabra is frequently killed by fires. Wildfire may promote seedling recruitment at or beyond forest edges, with heights of c. 1 m reached in only 2 y on burnt sites, but its role in promoting widespread in situ regeneration is uncertain.
Records of mass-flowering events in C. candelabra are insufficient for detailed analysis of environmental cues, as undertaken for some masting polycarps (Ashton et al. Reference Ashton, Givnish and Appanah1988, Kelly et al. Reference Kelly, Geldenhuis, James, Holland, Plank, Brockie, Cowan, Harper, Lee, Maitland, Mark, Mills, Wilson and Byrom2013), and the limited accuracy of age determination prevents detection of flowering cues from dates of establishment. No evidence was found of any association with rainfall or temperature, including temperature differentials suggested to trigger flowering in some New Zealand mass-flowering polycarps (Kelly et al. Reference Kelly, Geldenhuis, James, Holland, Plank, Brockie, Cowan, Harper, Lee, Maitland, Mark, Mills, Wilson and Byrom2013). However, the three recent widespread flowering events of C. candelabra occurred in years of cyclones, as did the 1969 Touaourou event. Furthermore, flowering is currently occurring (July 2021) at numerous locations across the south of the island, including at Pic du Grand Kaori and Forêt Nord in forests damaged by Tropical Cyclone Niran in early March 2021 and Strong Tropical Depression Lucas a month earlier (S. McCoy, pers. observation). Notably, C. candelabra appears to have a high resistance to wind damage, due in part to cell-level traits plus its unique architecture (Read et al. Reference Read, Evans, Sanson, Kerr and Jaffré2011), essential if wind is a flowering cue since trees must survive the cyclone to reproduce. However, no cyclone was associated with the localised 1967 flowering event at the confluence of the Tontouta and Kalouéhola Rivers reported by Veillon (Reference Veillon1971). Stress is known to induce flowering across a range of species (Takeno Reference Takeno2016). Given that other types of stress or damage can trigger flowering in individual trees (Read et al. Reference Read, Sanson, Jaffré and Burd2006, Veillon Reference Veillon1971), mass flowering of C. candelabra might not always be triggered by the same cue. Flowering may be widespread when the stressor is widespread, for example a cyclone or drought, but more localised if the geographic extent of the stressor is narrow. For example, sudden floods can occur in the Tontouta Valley, leading to over-bank flooding, usually but not only associated with heavy rainfall occurring with cyclones (Terry et al. Reference Terry, Kostaschuk and Wotling2008), and potentially triggering flowering in riverine forests.
It is difficult to explain the absence of flowering at the Bois du Sud site in 1996, 2003 or 2017. Tree size and age (in 2018: 5–27 cm DBH; 40–78 y) overlapped that of flowering trees on other plots (in 2018: 5–60 cm DBH; 54–155 y). Lack of flowering might be due to reduced exposure to an environmental cue for flowering, but notably its topographic position is not likely to have provided protection from cyclones (cf. Boose et al. Reference Boose, Foster and Fluet1994), or to a more complex determinant of flowering competence than tree size or age alone.
Roles of tree size, age and growth trajectory in the onset of flowering
Flowering in C. candelabra does not generally appear to be precocious as suggested by Poorter et al. (Reference Poorter, Zuidema, Peña-Claros and Boot2005) for the monocarpic Tachygali vasquezii. Instead, it is often substantially delayed relative to co-occurring trees, for example emergent C. candelabra trees >100 y old. However, flowering of a relatively young stunted tree c. 19 y old indicates that early flowering is possible. We found no evidence of a simple threshold for flowering competence, given the wide range of size, age and RGR in flowering trees. The effect of size appears complex, with a similar DBH range of flowering and non-flowering trees ≥5 cm DBH, but a significant effect of DBH on flowering at one site in 2017 and at multiple sites in 2003 (Read et al. Reference Read, Sanson, Jaffré and Burd2006). Flowering probability increases with size across a range of short- and long-lived monocarps, with a threshold size for flowering (Kuss et al. Reference Kuss, Rees, Ægisdóttir, Ellner and Stöcklin2008, Metcalf et al. Reference Metcalf, Rose and Rees2003, Tissue & Nobel Reference Tissue and Nobel1990). However, as in C. candelabra, size may vary substantially among flowering individuals of some monocarpic species (Augspurger Reference Augspurger1985, Foster Reference Foster1977, Loveless et al. Reference Loveless, Hamrick and Foster1998, Metcalf et al. Reference Metcalf, Rose and Rees2003). This variation has been attributed to age effects on reproduction, genetic differences in programmed reproductive size, or differences in growth between the time the threshold is reached and flowering starts (Augspurger Reference Augspurger1985, Metcalf et al. Reference Metcalf, Rose and Rees2003). In C. candelabra, growth rate or its physiological determinants could be influencing flowering competence by signalling stress and a greater likelihood of death, rather than resource abundance (e.g. stored carbohydrates). This is consistent with the low incidence of death without prior flowering (Read et al. Reference Read, Sanson, Burd and Jaffré2008). Individual trees (within a population or isolated) can flower in years between mass-flowering events, associated with external damage or sometimes with no obvious damage (Read et al. Reference Read, Sanson, Jaffré and Burd2006, Veillon Reference Veillon1971) but possibly experiencing physiological stress.
The wide range of size, age and growth rate of flowering trees within populations suggests that flowering may be a response to an interaction of age with size (or life stage) (Young Reference Young1985, Burd et al. Reference Burd, Read, Sanson and Jaffré2006). Young (Reference Young1985) found, for example, that slower-growing plants of Lobelia telekii were more likely to flower than faster-growing plants in small rosettes, with the opposite trend in large rosettes. The life-history model of Burd et al. (Reference Burd, Read, Sanson and Jaffré2006) takes account of the way growth rate affects a plant’s size at any given age and the length of time it will be exposed to mortality factors before reaching a given size. Our first prediction, that slow- and fast-growers will flower at a limited range of sizes but trees with intermediate growth rates will flower over a wide size range, reflects differences in potential future fitness. Slow-growers stand to gain little by waiting to flower until the next environmental cue, and so do best to flower early in life, hence at a restricted range of sizes. Large, rapidly growing trees may also benefit little from future growth if, as is typical, growth trajectories eventually plateau due to accumulating support tissue relative to photosynthetic tissue (King Reference King2005). They should flower over a restricted range of large sizes before growing ‘too large’. In contrast, individuals with intermediate growth could profit from waiting for a future opportunity to reproduce, at which point variation in their growth rates will have amplified their size differences. Our second prediction, that larger non-flowering trees should be experiencing faster growth than smaller flowering trees, also reflects the tendency for fast growers to have greater opportunity to increase future fitness from further growth. Flowering and growth patterns among trees at Tontouta qualitatively support these predictions (Figure 7). However, this model requires further investigation, across larger numbers of individuals and across multiple sites and flowering events, particularly where it allows formal testing of quantitative predictions.
Conclusion
Studied populations of the monocarpic C. candelabra have unimodal age distributions, with trees alive in 2007–2008 having established over c. 15–81 y. This successful period of establishment contrasts with the later recruitment gap of c. 23–79 y (36–90 y by 2018). Recent flowering events have often led to high initial densities of C. candelabra seedlings, but with high mortality. Recruitment and persistence may rely on high densities of synchronously flowering trees or on large-scale disturbances (or in combination) for in-situ regeneration, or establishment in exposed conditions beyond forest edges (ex-situ regeneration). Further research is required to determine whether the disparity between past and present patterns of establishment reflects changing regimes of disturbance (including those of anthropogenic origin) or climate, which may affect both flowering patterns and recruitment. In particular, further research is required on the controls of flowering. Environmental flowering cues were not identified by dating tree establishment. The only apparent correlate with the last three mass-flowering events was the occurrence of cyclones earlier in the year, but other stresses may also trigger mass flowering, at least at a local scale. In 2017, flowering occurred across a wide range of tree size, age and growth rate. There was no support for a simple threshold for flowering competence. However, there is tentative support for the prediction that optimal flowering size and age depends on plant growth trajectory.
Acknowledgements
We are grateful to Province Sud for permission to undertake research in the southern forests (Permit #3224–2018), Prony Resources New Caledonia for access to Forêt Stephane, Société Minière Georges Montagnat for access to Tontouta Valley sites and M. Lucien for access to forest at Mouirange. We thank Météo France en Nouvelle-Calédonie, for meteorological data and U. Zoppi (dec.) for encouraging 14C dating. We also thank the Herbier de Nouvelle-Calédonie UMR AMAP, staff of IRD and Prony Resources New Caledonia and P. Peeters and R. Carpenter for assistance, and J.-M. Veillon and T. Jaffré for numerous helpful discussions.
Financial support
This research was funded by an Australian Institute of Nuclear Science and Engineering Grant (#06152P) for radiocarbon dating and National Geographic Society Grants (#7772-04 and #8798-10).
Competing interests
The authors declare none.
Supplementary material
To view supplementary material for this article, please visit https://doi.org/10.1017/S0266467421000389
Appendix Radiocarbon results
Bold font in two columns (‘Errors on ring counts’ and ‘Interpretation’) shows our interpretation of the data. Age calibration was performed using the bomb radiocarbon data for the Southern Hemisphere zone 1-2 (Hua et al. Reference Hua, Barbetti and Rakowski2013) and CALIBomb program (Reimer & Reimer Reference Reimer and Reimer2004). *Year of growth for Southern Hemisphere trees was assigned to that in which growth begins.
