INTRODUCTION
Cycads are ancient plants (Gao & Thomas Reference GAO and THOMAS1989) that may provide insight into the evolution of complex host-specific pollination mutualisms with insects. Cycads in the family Zamiaceae are well known for their obligate pollination mutualisms, typically with various species of beetle that appear to have evolved a one-to-one relationship with their cycad hosts, with wind playing a negligible role in pollination. This symbiosis has been documented across the broad geographic range of the Zamiaceae, including the Americas (Norstog et al. Reference NORSTOG, STEVENSON and NIKLAS1986, Tang Reference TANG1987a), Africa (Donaldson Reference DONALDSON1997, Proches & Johnson Reference PROCHES and JOHNSON2009, Suinyuy et al. Reference SUINYUY, DONALDSON and JOHNSON2009) and Australia (Hall et al. Reference HALL, WALTER, BERGSTROM and MACHIN2004, Terry Reference TERRY2001, Wilson Reference WILSON2002). By contrast, there is evidence that at least some cycads in the genus Cycas may be ambophilous, for the most part pollinated by insects in a specific mutualism comparable to that of other cycads, but retaining the potential for a limited degree of wind pollination not seen in the Zamiaceae (Hamada et al. Reference HAMADA, TERRY and MARLER2015a, Reference HAMADA, TERRY, ROEMER and MARLERb; Kono & Tobe Reference KONO and TOBE2007, Raju & Jonathan Reference RAJU and JONATHAN2010a, Reference RAJU and JONATHANb; Wang et al. Reference WANG, LI, YANG, HUANG and CHEN1997, Yang et al. Reference YANG, Li, Li, LIN and YU2010).
Cycas, the only genus in the family Cycadaceae, includes some 100 species, widely distributed across tropical and subtropical regions of Asia, Australia, Oceania, India and Madagascar (Jones Reference JONES2002). While potential beetle pollinators are often present in Cycas cones, field studies that have quantified airborne loads of Cycas pollen directly have all concluded that wind pollination could also be effective, albeit restricted to situations where male and female plants occur only short distances apart (~2–10 m) and in relatively open habitats (Hamada et al. Reference HAMADA, TERRY and MARLER2015a, Kono & Tobe Reference KONO and TOBE2007, Wang et al. Reference WANG, LI, YANG, HUANG and CHEN1997, Yang et al. Reference YANG, Li, Li, LIN and YU2010). One situation where this may apply relates to Cycas subsection Rumphiae, a derived subgroup of Cycas with floating seeds that have colonized oceanic islands (Hamada et al. Reference HAMADA, TERRY and MARLER2015a, Reference HAMADA, TERRY, ROEMER and MARLER2015b; Hill Reference HILL1994, Keppel et al. Reference KEPPEL, HODGSKISS and PLUNKETT2008, Marler Reference MARLER2010, Terry et al. Reference TERRY, ROE, TANG and MARLER2009), this being a dispersal biology that seems incompatible with requirements for obligate, host-specific insect pollinators. Significantly, different insects are associated with Cycas cones in different parts of the world, such as Asia (Tang et al. Reference TANG, OBERPRIELER, YANG and Chen1999, Skelley et al. Reference SKELLEY, XU, TANG, LINDSTROM, MARLER, KHURAIJAM, SINGH, RADHA and RICH2017, Xu et al. Reference XU, TANG, SKELLEY, LIU and RICH2015), the Pacific Islands (Marler Reference MARLER2010, Terry et al. Reference TERRY, ROE, TANG and MARLER2009) and Australia (Forster et al. Reference FORSTER, MACHIN, MOUND and WILSON1994, Ornduff Reference ORNDUFF1991, Reference ORNDUFF1992), implying that pollination mutualisms with cone-associated insects have evolved more than once.
The pollination adaptations of Cycas are potentially ancient, since the divergence between the Zamiaceae and Cycadaceae lines dates from the Mesozoic (Salas-Leiva et al. Reference SALAS-LEIVA, MEEROW, CALONJE, GRIFFITH, FRANCISCO-ORTEGA, NAKAMURA, STEVENSON, LEWIS and NAMOFF2013). Reflecting this separation, the female reproductive structures of Cycas differ markedly from the more enclosed cones of the Zamiaceae, consisting of a radiating crown of megasporophylls that closely resemble fossils of the oldest Palaeozoic cycads (Gao & Thomas Reference GAO and THOMAS1989). Could Cycas therefore preserve some kind of transitional state between wind and insect pollination? Or alternatively, could ambophily be a derived feature that has helped Cycas species to become the most diverse and geographically widespread of living cycad genera?
Our study is the first to quantify Cycas pollination in Australia, this continent being an important centre of endemism for the group, with 35 of the world's ~100 species (Jones Reference JONES2002). Investigating the potential for ambophily in Cycas ophiolitica from central Queensland, we tested the hypotheses that (1) the beetles on C. ophiolitica cones are effective pollen carriers that can pollinate female cones after exclusion of windborne pollen; and (2) that the airborne pollen load in the vicinity of C. ophiolitica is sufficient for wind pollination to be effective in the absence of insects. We subsequently discuss the broader significance of the potential for ambophily in Cycas, and the role it may have played in allowing new, complex host-specific pollination mutualisms to arise relatively quickly through the pre-adaptation of component parts.
METHODS
Study site and organisms
Cycas ophiolitica K.D.Hill is a typical representative of the Australian species of Cycas (Figure 1a) occurring between Marlborough and Rockhampton in Central Eastern Queensland (Jones Reference JONES2002). The 27 species of Australian Cycas (Hill Reference HILL and McCarthy1998) are closely related, having radiated since the arrival of a common ancestor from Asia during the Miocene (Hill Reference HILL and Chen1999). Note that, since the independent megasporophylls of the Cycas female reproductive structure are not attached to a single rachis, this structure is technically not a true cone, but for convenience, it is referred to as such throughout this paper.
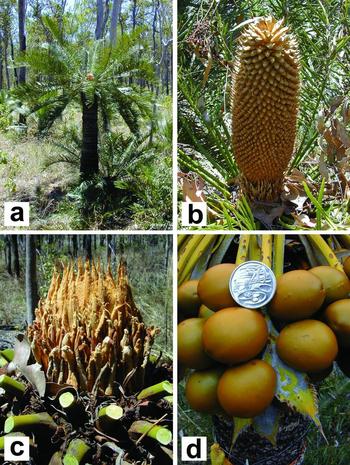
Figure 1. Reproductive structures of Cycas ophiolitica, Central Queensland, Australia. Plant in habitat (a). Note receptive female cone at trunk apex, surrounded by foliage crown. Male cone (b). Male cones grow ~400 mm high at pollen shed. Note the screening effect of the plant's own foliage surrounding the cone. Female reproductive structure at pollen receptivity (c). This consists of a dense cluster of ovule-bearing megasporophylls, emerging from the spine-like cataphylls that protect the apical meristem. Pollinating ovules are ~15 mm diameter, and are sheltered by both the megasporophylls, and the foliage that surrounds them (the leaves have been cut away in this photograph). Only in the months after pollination do the megasporophylls lengthen and become pendant (d). The seed and fleshy sarcotesta are together ~40 mm diameter, and conspicuously exposed to potential dispersal fauna. Unpollinated ovules do not increase in size in this way.
The study was undertaken on privately owned bushland adjacent to Mount Archer National Park, on the south-facing slopes of Mount Sleipner (summit 23°22′11″S, 150°36′57″E). The canopy of this dry sclerophyll woodland is dominated by Eucalyptus tereticornis Smith, E. creba F.Muell., E. platyphylla F.Muell., Corymbia citriodora Hook. and Lophostemon suaveolens Sol. ex Gaertn. The open, grassy understorey is dominated by two cycad species, C. ophiolitica and Macrozamia miquelii F.Muell., and the grass tree Xanthorrhoea johnsonii A.T.Lee. The study was undertaken over the years 2003–2007, the coning season usually beginning in December and finishing by February, although the precise timing was variable.
The three beetle species observed as potential pollinators of C. ophiolitica pollination were the same as those collected by Forster et al. (Reference FORSTER, MACHIN, MOUND and WILSON1994) from male cones of this species – an undescribed weevil around 4 mm long (Curculionidae); an erotylid beetle, Hapalips sp. around 6.5 mm long (Erotylidae) and a tenebrionid beetle Ulomoides sp. around 5 mm long (Tenebrionidae). Note that at the time Forster et al. (Reference FORSTER, MACHIN, MOUND and WILSON1994) published, Hapalips was placed in the Languriidae, but a taxonomic revision by Leschen (Reference LESCHEN2003) now places it in the Erotylidae.
Beetles were hand-collected from C. ophiolitica during haphazard and opportunistic dissections of cones of both sexes in situ. Other insects, such as crickets and moths, were occasionally encountered sheltering in cones, but the beetles noted above were the only insects that were associated with female cones consistently and in numbers. Voucher specimens of these beetles were lodged with The University of Queensland Insect Collection, which has since been amalgamated into the Queensland Museum Collection.
Pollinator exclusion experiments
Pollinator exclusion experiments were established to compare relative pollination success after the exclusion of either wind or insects (as per the approach of previous studies, e.g. Kono & Tobe Reference KONO and TOBE2007). Treatments were established on immature female cones at the earliest possible stage of development to ensure they had not yet become receptive. The insect exclusion treatment (n = 10, Figure 2a) presented a barrier to beetles and other insects of similar size or larger, while still allowing potentially pollen-bearing wind currents to reach the female cone. A mesh bag (of hexagonal cells, 2 mm diameter at widest point) was placed over the cone, wired in place, and further sealed at the edges with a layer of engine grease. The netting of the bag was held relatively taut (so as not to impede air movement) by means of an open-sided plastic cylindrical frame. The join between bag and trunk was further sealed using space-filling foam. To apply the exclusion treatment, all leaves had to be removed from the plant, a measure demonstrated not to affect cycad cone development and pollination in other cycad genera (Hall et al. Reference HALL, WALTER, BERGSTROM and MACHIN2004, Terry et al. Reference TERRY, WALTER, DONALDSON, SNOW, FORSTER and MACHIN2005). Nevertheless, a control for the effect of such leaf removal in Cycas was included. To check for insect visitors smaller than the mesh diameter, sticky traps consisting of plastic surfaces smeared with Tanglefoot ® (area of adhesive ~100 × 100 mm) were wired adjacent to the cone structure before the netting was sealed in place.

Figure 2. Pollination experiments on Cycas ophiolitica, Central Queensland, Australia. Insect exclusion of fine netting over a plastic frame, secured at the base with wire and space-filling foam (a). Wind exclusion by means of a cloth bag wired loosely in place over the cone (b). Pollen traps in the immediate vicinity of a female cone (c). Typical light microscope image of Cycas pollen recorded on an airborne trap (d). The cycad pollen typically aggregates in clumps rather than dispersing as isolated grains. (i) = Individual grain of cycad pollen, boat- shaped with a central longitudinal furrow. (ii) = Two clumps of cycad pollen consisting of many dozens of individual grains.
The wind exclusion treatment (n = 10, Figure 2b) comprised a large cloth bag, wired in place over the cone to baffle wind currents, but left open at the base so that beetles could enter by crawling upwards (which they do readily). Again, all leaves were removed. Two control groups were established, the first consisted of plants left to pollinate in their natural state (n = 10), the second had all foliage removed but the cones left to develop intact (n = 10). The exclusion experiment was established in December 2006 and the cones collected for dissection in February 2007. Pollinated and non-pollinated ovules could be distinguished from one another on the basis of size difference, since in Cycas non-pollinated ovules fail to develop (G. Kreutzmann, pers. comm). This was confirmed by the dissection of several ovules, that revealed suspensor development in large pollinated ovules, and absence of such a structure in small non-pollinated ovules.
Airborne load of cycad pollen
A series of pollen traps was used to assess the airborne load of C. ophiolitica pollen in the vicinity of both male and female plants (Figure 2c; Hall et al. Reference HALL, WALTER, BERGSTROM and MACHIN2004), to test whether airborne pollen load was consistent with the large quantities required, in principle, for wind pollination to be effective. Lines of pollen traps were deployed at increasing distances from male cones (n = 10) that were nearing pollen-shed. Isolated cones were selected, to ensure that the sampled male cone was the source of any trapped pollen. Each trap was elevated on a stake so that it was at the same height above the ground as the male cone it was facing. Across the site, the height of the male cones sampled ranged between ~0.5 and 1.5 m. The traps consisted of microscope slides smeared with a thin layer of Carberla's solution adhesive, fitted into a small open-sided box to shelter the adhesive surface from rain, and fixed to a stake of the required height. Each male cone was surrounded by three equidistantly spaced and outwardly radiating trap-lines, with the adhesive surface facing back towards the pollen source (the equidistant trap-lines around different males were randomly orientated with respect to each other). Pollen traps were placed at 0.5, 2, 5 and 10 m along each trap-line, and were left in the field for the entire period of pollen-shed (4 wk).
A second experiment quantified the amount of windborne pollen reaching female cones during the pollen-shed period. Three, equidistantly spaced pollen traps were positioned within 30 cm of each of 10 receptive female cones, the adhesive surface pointing outwards towards any potential pollen sources (the equidistantly spaced pollen traps around different females were randomly orientated with respect to each other). These female plants were the same as those used as the ‘intact foliage’ control group in the exclusion experiment, so their pollination success was also quantified, as was the density and proximity of male cones nearby. Each of these traps was elevated on a stake so that it was at the same height above the ground as the adjacent female cone. Note that C. ophiolitica is an understorey plant of only moderate stature, and hence the height from the ground of all observed cones at the site, both male and female, ranged between ~0.05 and 2 m.
For all pollen traps, cycad pollen capture was quantified by a microscope count within an 81-mm2 quadrat on the slide at 10× magnification. Cycad pollen has a distinctive cymbiform or boat-shaped morphology (Figure 2d; Dehgan & Dehgan Reference DEHGAN and DEHGAN1988, Marshall et al. Reference MARSHALL, GROBBELAAR, COETZEE and OSBORNE1989) that allowed it to be positively identified during microscopic examination of the pollen traps. The sympatric Macrozamia cycads at the study site were not coning at the same time as the Cycas, so there could be no confusion between them in regard to pollen capture on the slides.
A guideline for evaluating airborne loads as a test of the effectiveness of wind pollination
In evaluating airborne pollen loads, Proctor et al. (Reference PROCTOR, YEO and LACK1996, p. 265) made the useful suggestion that ‘every square metre of the [wind pollinated] plant's habitat must receive around a million pollen grains to make pollination reasonably certain’. This estimate is derived from the receptive surface of female reproductive structures on most plants (such as the angiosperm stigma, or the micropyle on cycad ovules) typically being a square millimetre or less in capture area, and that there are one million square millimetres per square metre (M. Proctor pers. comm.). We have used this conjectural estimate to give context to our airborne pollen load results, since some kind of guideline of this sort is essential when evaluating the potential for wind pollination from first principles when the pollination vector is unknown. We note that mitigating factors, such as the hypothetical possibility of rainwater or insects secondarily moving airborne pollen deposited upon cone surfaces and concentrating it at the micropyle, could allow for wind pollination to be effective below this threshold. But there remains a fundamental physical imperative in wind pollination to maximize airborne loads (Ackerman Reference ACKERMANN2000, Dafni Reference DAFNI1992, Faegri & Van der Pijl Reference FAEGRI and VAN DER PIJL1979, Proctor et al. Reference PROCTOR, YEO and LACK1996) and hence we considered this guideline to be a valid approach in interpreting our data.
Demography of coning
A census of coning demography was made to assess the degree to which plants in the population participated in the coning episode, and to test if the spatial pattern of plants was consistent with the distance limitations of wind pollination as implied by the airborne pollen load results. The coning status and location of all cycads presumed to be sexually mature within 10 circular plots of 30-m radius were recorded. Presumption of sexual maturity was based on development of >5 cm of arborescent trunk, the minimum plant size we observed presenting cones. The 10 plots were centred on the coning females of the intact foliage control group, whose pollination success and airborne pollen load were known.
Pollen load of cone-associated beetles
Individuals of the three potential beetle pollinators of C. ophiolitica pollination were collected from pollen-shedding male cones to test their relative ability to carry pollen on their bodies. Total pollen load was estimated by the centrifuge method of MacGillivray (Reference MACGILLIVRAY1987). Beetles (n = 10 for each of the three cone-associated species) were shaken individually in centrifuge tubes containing xylene and 400 ml of glycerol gelatine. The beetle was removed and the tube centrifuged at 15000 rpm for 1 min, embedding the xylene-suspended pollen into the glycerol gelatine. The glycerol gelatine pellet was then melted onto a microscope slide for examination of the pollen load. Cycad pollen on these slides was quantified by a microscope count within a 32-mm2 quadrat at 10× magnification (representing some 30% of the cover-slip area).
Statistical procedures
In all cases, the decision to use either parametric or non-parametric methods was made by applying a Shapiro–Wilk test to the data. For the pollinator exclusion experiment (non-parametric data) a Kruskal–Wallis one-way analysis of variance was conducted, and any significant differences between treatments were identified by a Games–Howell post hoc test. To test for a correlation between airborne pollen load in the vicinity of female cones and their subsequent pollination success (non-parametric data) we used Spearman's rank correlation. To test for a correlation between the pollination success of female cones and the distance to their nearest coning male neighbour (parametric data), we used the Pearson product-moment correlation. For beetle pollen loads (parametric data) a one-way ANOVA was applied to test for statistically significant differences between the species, with any such differences identified by the Tukey HSD post hoc test. All statistical analyses were performed in R (R Foundation for Statistical Computing, Vienna, Austria), with the package ‘userfriendlyscience’ (http://userfriendlyscience.com) used to perform the Games–Howell post hoc test.
RESULTS
Pollinator exclusion experiments
Although the pollination success of individual plants in the pollination experiment was highly variable (even within control treatments) the exclusion of insects from cones had the greatest effect in reducing the pollination success of Cycas ophiolitica (Figure 3). The median pollination success of the leaves-intact control group was ~84% of ovules on a cone, and the removal of foliage had no statistically significant impact on pollination success (Figure 3). Exclusion of wind decreased median pollination success to ~53% while the exclusion of insects decreased pollination success to ~13% (Figure 3). Both these results were significantly different from the leaves-removed control, but were not significantly different from each other. However, only the insect exclusion treatment had a statistically significant decrease in pollination success relative to the leaves-intact control (Figure 3). Potential beetle pollinators (weevils and Ulomoides sp.) and other insects (moths, crickets) were captured on sticky traps within six of the 10 insect exclusion treatments, but in low numbers (one or two per cone). No holes or ripping were present in the netting of the insect exclusion treatment when the experiment was concluded, and it is possible these organisms were present within the exclusion, sheltering among the megasporophylls, from the outset.

Figure 3. Pollination success (% of ovules pollinated) of Cycas ophiolitica female cones, Central Queensland, Australia, after exclusion of wind and insect vectors, as compared with two control groups, one with leaves intact and one with the leaves removed (this was done because leaves were also removed from the wind and insect exclusions). The exclusion of insects had a greater effect than the exclusion of wind in reducing pollination success. Boxes represent inter-quartile range, whiskers the range, and the central stripe the median. Treatments annotated with the same letter did not vary significantly from each other in pollination success (Games–Howell post hoc test of a Kruskal–Wallis one-way analysis of variance, α = 0.05, n = 10 for all treatments).
Airborne load of cycad pollen
Airborne pollen load was high within 0.5 m of the male cone (Table 1), but these traps were typically within the encircling crown of leaves. Beyond that, the airborne pollen load fell away dramatically (Table 1). At 2 m the average airborne pollen load was only 5% of that recorded within 0.5 m, further falling to 2% by 5 m and 0.6% by 10 m (Table 1). By our estimate, the potential for effective wind pollination of C. ophiolitica (i.e. >106 pollen grains m−2) falls away somewhere between 5 and 10 m from pollen-shedding male cones. Even at 5 m, few individual traps actually recorded sufficient pollen to indicate wind pollination might be effective (Table 1). The pollen had adhesive qualities, such that it tended to disperse through the air as aggregated clumps rather than single grains (Figure 2d). This clumping tendency of the pollen was a driver of the high variability in pollen capture between individual traps.
Table 1. Changes in the airborne load of cycad pollen trapped at increasing distances from Cycas ophiolitica male cones. Mean (± SE) and range is presented for the pollen counted on the slide for 33 traps at each distance. This mean pollen count is also presented as a percentage of the mean pollen count at 0.5 m to illustrate the distance drop-off in airborne pollen load. These data were also extrapolated to a mean pollen count (estimated number of pollen grains m−2), and, based on this extrapolation, the percentage of traps at each distance yielding estimates of >106 pollen grains m−2 was noted, since this was the figure we used as the approximate theoretical limit for the effectiveness of wind pollination.

The airborne pollen load in the vicinity of female cones was also highly variable (Figure 4). While some airborne pollen was recorded in the vicinity of all female cones, only six of the 10 females had, by our estimate, an extrapolated mean airborne pollen sufficient for effective wind pollination. No correlation was detected between the estimated airborne pollen load in the vicinity of a female cone, and its subsequent pollination success (Spearman's rank correlation, n = 10, rs = 0.426, P = 0.22). Further, two of the four female cones experiencing a pollen rain of <106 grains m−2 had high pollination success (while for the other two it was low), suggesting that substantial airborne pollen loads are not required for high pollination success in these plants.

Figure 4. Extrapolated airborne pollen load (pollen grains m−2) in the immediate vicinity of 10 Cycas ophiolitica female cones, Central Queensland, Australia, against the pollination success achieved (% of ovules pollinated). Airborne pollen load estimates were extrapolated from mean cycad pollen captured on n = 3 pollen traps per cone. Although six of the 10 females achieved the pollen load of >106 m−2 deemed necessary for effective wind pollination, there was no correlation between airborne pollen load and subsequent pollination success (Spearman's rank correlation, rs = 0.426, P = 0.22). Further, two of the four female cones experiencing a pollen rain of <106 m−2 had high pollination success. Collectively, these results imply that while wind pollination is, in principle, sometimes possible for this species, insects are the more important pollination vector.
Demography of coning
Only a subset (21%, n = 686) of the adult plants present in the population participated in the coning event for that season (Table 2, Figure 5). The plots collectively recorded 86 male cones and 60 females, a sex ratio of about 4:3 coning males to females. These plots were centred on the ‘intact foliage’ female cone controls, whose pollination success was known (Table 2). No significant correlation was found between the pollination success of these females and the distance to the nearest male cone (Pearson product-moment correlation, n = 10, r = 0.360, P = 0.31). Based on airborne pollen trapping, a 5-m radius surrounding male cones was the estimated limit for effective wind pollination, and the area within this radius is indicated in two maps of demography plots given in Figure 5a (representing a relatively high density of plants) and Figure 5b (representing a relatively low density). In total, for all plots censused, only 21 female cones (35%) were recorded within 5 m of a pollinating male cone (Table 2). Hence, very close proximity of coning male and female plants was not a prerequisite for pollination success.
Table 2. Coning demography of Cycas ophiolitica in 10 circular plots of 30-m radius. Mature cycads were potentially reproductive based on plant size. Coning plants represented only a subset of the mature plants present. The percentage of coning females within 5 m of coning males represents the proportion of females within the estimated distance range for wind pollination to be effective. Each of the 10 demography plots was centred on a coning female. The pollination success (% ovules pollinated) and the distance to the nearest male are given for these females. There was no significant correlation between their pollination success and the distance to the nearest male cone (Pearson product-moment correlation, n = 10, r = 0.360, P = 0.31).


Figure 5. Coning demography of Cycas ophiolitica, Central Queensland, Australia, in a representative higher-density stand (a). This represents plot A in Table 2. The location and coning status of all adult plants was recorded within a 30-m radius of the coning female that defined the plot centre. The pollination success of the central female was 87.9%. As this map illustrates, at higher spatial densities within populations, female cones frequently occurred within our estimated 5-m limit for effective airborne pollen transport from male cones (indicated by the grey shading surrounding coning male plants). Compare this with the coning demography in a representative lower-density stand of the same species at the same site (b). This represents plot B in Table 2. The pollination success of the central female was 83.2%. At lower spatial densities, coning females often occurred beyond our estimated 5-m limit for effective airborne pollen transport from male cones. The high pollination for the female at plot centre suggests that proximity to males is not essential for pollination success.
Pollen load of cone-associated beetles
All three beetle species were observed in male and female cones, the weevils being the most abundant, although this was not quantified (census required cone destruction, and cones were required intact for other experiments). A random sample of beetles collected from pollen-shedding male cones indicated that all three species could potentially transport many thousands of pollen grains on their bodies (Figure 6). Pollen load varied across the species, probably on the basis of body size, surface area and pilosity, but only the difference in pollen load between the undescribed weevil and Ulomoides sp. was statistically significant (one-way ANOVA, F 2,27 = 3.35, P = 0.004; significant differences between treatments identified by the Tukey HSD post hoc test). The pollen load of beetles from female cones was not quantified, again because this would have required the destruction of cones needed for other experiments.

Figure 6. Estimated mean pollen load (n = 10) for each of the three beetle species collected from Cycas ophiolitica male cones, Central Queensland, Australia. Inset shows relative body size of the beetles (scale bar interval = 1 mm). Mean ± SE body length (n = 6) for each of the three species was: undescribed weevil = 4.3 ± 0.2 mm; Hapalips sp. = 5.5 ± 0.2 mm; Ulomoides sp. = 4.8 ± 0.1 mm. Pollen load was likely related to body surface area. As shown by the inset, Ulomoides sp. has a broader body shape for its length. The difference in pollen load between the undescribed weevil and Ulomoides sp. was the only statistically significant difference among the three beetles (one-way ANOVA, F 2,27 = 3.35, P = 0.004; significant differences between treatments indicated by the Tukey HSD post hoc test).
DISCUSSION
The relative roles of beetles and wind in the pollination of Cycas ophiolitica
Our study provides further evidence of the Cycas-beetle pollination relationship first documented by Kono & Tobe (Reference KONO and TOBE2007) for C. revoluta on the Southern Japanese Islands, since, in the absence of wind, the Australian cycad C. ophiolitica is effectively pollinated by insect visitors (Figure 3). The beetle species Hapalips sp., Ulomoides sp. and an undescribed weevil were collected from both male and female cones, and pollen adhering to beetles from male cones suggested a high potential for pollen transport (Figure 6). In our pollination experiment the exclusion of these insects from cones resulted in the largest reduction in pollination success. The low level of pollination achieved when insects, but not wind, were excluded from cones could be taken as evidence for ambophily in Cycas, although this result should be interpreted with caution, since we were unable to completely rule out contamination of the treatment by beetles and other invertebrates which may have been carrying pollen.
Assessment of the airborne pollen load provides better evidence for a potentially meaningful but distance-limited role for wind pollination in C. ophiolitica. Airborne pollen load drops away dramatically with distance from male cones (Table 1). Our results imply that airborne pollen transport for C. ophiolitica ceases to be effective somewhere between 5 and 10 m, a result comparable to other Cycas species whose airborne pollen loads have been similarly quantified in the field (Hamada et al. Reference HAMADA, TERRY and MARLER2015a, Kono & Tobe Reference KONO and TOBE2007, Wang et al. Reference WANG, LI, YANG, HUANG and CHEN1997, Yang et al. Reference YANG, Li, Li, LIN and YU2010). This contrasts with the Zamiaceae cycad Lepidozamia peroffskyana, pollinated exclusively by weevils, for which airborne pollen (as quantified by an identical method) was not consistently recorded beyond 2 m from male cones (Hall et al. Reference HALL, WALTER, BERGSTROM and MACHIN2004). The potential for at least some wind pollination in Cycas, although very limited, contrasts dramatically with the lack of any compelling evidence that wind pollination occurs at all across a broad range of cycads in the sister family Zamiaceae (Hall et al. Reference HALL, WALTER, BERGSTROM and MACHIN2004, Norstog et al. Reference NORSTOG, STEVENSON and NIKLAS1986, Proches & Johnson Reference PROCHES and JOHNSON2009, Suinyuy et al. Reference SUINYUY, DONALDSON and JOHNSON2009, Terry et al. Reference TERRY, WALTER, DONALDSON, SNOW, FORSTER and MACHIN2005, Wilson Reference WILSON2002).
Even so, our results show that a high proportion of C. ophiolitica female plants occur too far away from coning males for wind pollination to be effective. In contrast to such metre-scale limitations of wind pollination in Cycas, studies of plants that characteristically rely on wind pollination indicate that airborne pollen transport is effective over many tens of metres, if not kilometres (Bannert & Stamp Reference BANNERT and STAMP2007, Bittencourt & Sebbenn Reference BITTENCOURT and SEBBENN2007, Reference BITTENCOURT and SEBBENN2008; Dow & Ashley Reference DOW and ASHLEY1998, McCartney & Lacey Reference MCCARTNEY and LACEY1991). But despite the rapid fall in airborne pollen with distance from male cones, we found that airborne pollen load in the immediate vicinity of C. ophiolitica female cones was sometimes high enough for wind pollination to be plausible (Figure 4), and Australian Cycas often (but not always) grow at the necessary high spatial densities (Figure 5; Griffiths et al. Reference GRIFFITHS, SCHULT and GORMAN2005, Ornduff, Reference ORNDUFF1991, Reference ORNDUFF1992; Watkinson & Powell Reference WATKINSON and POWELL1997, Woodall Reference WOODALL2016).
In summary, the variable and unreliable capture of airborne pollen on our traps, the lack of any consistent relationship between airborne pollen load and the pollination success of female cones, and the high pollination success of some female cones in the absence of high airborne pollen loads, all imply that insects, not wind, are the primary pollination vector for C. ophiolitica. This said, our assessment of the airborne pollen load implies that some degree of wind pollination could be possible for this species, but only at high spatial densities (female plants growing within ~5 m of coning males).
Features of Cycas biology suggest a longstanding adaption for insect pollination
Cycas is a diverse genus, with more than 100 species occurring in varied habitats across a broad geographic range (Jones Reference JONES2002). While this means generalizations about the genus can only be made tentatively, the fact that our results for ambophily in the Australian C. ophiolitica match so closely with those of Kono & Tobe (Reference KONO and TOBE2007) for the geographically and taxonomically distant C. revoluta in Japan suggest that some generalization is possible. The existence of ambophily in such an ancient plant group, whose reproductive structures closely resemble fossils of the oldest Palaeozoic cycads (Gao & Thomas Reference GAO and THOMAS1989), naturally suggests the hypothesis that Cycas might preserve some kind of transitional state between wind and insect pollination. Kono & Tobe (Reference KONO and TOBE2007) suggested that C. revoluta pollination by Carpophilus beetles represented an ‘initial mode of animal pollination’ in which a partially destructive herbivore on the plant also pollinates it. We interpret the significance of the potential for ambophily in Cycas differently – while a secondary role for wind pollination is possible in the special circumstances of plants growing in relatively open habitats and at high spatial density, Cycas species are otherwise poorly adapted for wind pollination in ways that imply that insects have been the primary pollinators of these plants over a long evolutionary history.
Specifically, Cycas species are not characterized by reproductive parts freely exposed to the wind, as is typical of wind pollinated or strongly ambophilous species (Culley et al. Reference CULLEY, WELLER and SAKAI2002, Meléndez-Ramírez et al. Reference MELÉNDEZ-RAMÍREZ, PARRA-TABLA, KEVAN, RAMÍREZ-MORILLO, HARRIES, FERNÀNDEZ-BARRERA and ZIZUMBO-VILLAREAL2004). For example, both Kono & Tobe (Reference KONO and TOBE2007) and our study found that the encircling leaf crown thwarts pollen movement away from male cones or towards female cones. Wind-tunnel tests that suggest wind pollination in Cycas is effective (Niklas & Norstog Reference NIKLAS and NORSTOG1984) did not take into account this filtering effect of the foliage. Several Australian Cycas species are seasonally drought deciduous (Jones Reference JONES2002, Ornduff Reference ORNDUFF1991, Reference ORNDUFF1992) and we observed some C. ophiolitica presenting cones (both male and female) in the absence of any leaves. This would dramatically increase the odds of successful wind pollination, yet this potential adaptation (seen in deciduous wind pollinated plants such as hazel, Corylus spp.) has apparently not been selected for in C. ophiolitica.
Further, while the Cycas female reproductive structure opens out by the time of seed dispersal (Figure 1d), at pollination the megasporophylls are not freely exposed to the wind, but rather form a dense architecture of sheltered internal spaces (Figure 1c). This may explain why some of the most widely cultivated Cycas species fail to pollinate in garden settings, even when male and female plants are grown in close proximity (Jones Reference JONES2002). The degree of megasporophyll overlap varies among species and, significantly, Hamada et al. (Reference HAMADA, TERRY and MARLER2015a) reported that the outer megasporophylls of Pacific Island Cycas species do become slightly more open at pollination, perhaps suggesting secondary adaptation towards more effective ambophily for these species. Ecologically, the island-dwelling Cycas species with floating seeds would seem the best possible candidates for primarily wind-pollinated cycads, but even then, the reported effectiveness of airborne pollen loads is limited to distances of ~6–8 m in open habitats, and in sheltered forest understorey habitats they are negligible, implying the presence of an insect pollinator (Hamada et al. Reference HAMADA, TERRY and MARLER2015a).
The tendency of Cycas pollen to adhere in aggregated clumps (Figure 2d) is typical of insect dispersal (Culley et al. Reference CULLEY, WELLER and SAKAI2002, Faegri & Van der Pijl Reference FAEGRI and VAN DER PIJL1979, Proctor et al. Reference PROCTOR, YEO and LACK1996), but greatly reduces the effectiveness of wind dispersal, as such pollen clumps fall out of the air relatively quickly (Hall & Walter Reference HALL and WALTER2011). Such clumping resulted in the variable and sporadic nature of airborne pollen capture in our study. Finally, in terms of the use of the male cone as a brood site, and specialist pollinator signals such as volatile odour release and thermogenesis (Azuma & Kono Reference AZUMA and KONO2006, Forster et al. Reference FORSTER, MACHIN, MOUND and WILSON1994, Oberprieler Reference OBERPRIELER and Vorster1995a, Reference OBERPRIELER and Vorsterb; Raju & Jonathan Reference RAJU and JONATHAN2010a, Reference RAJU and JONATHANb; Roemer et al. Reference ROEMER, TERRY and MARLER2013, Tang Reference TANG1987b, Tang et al. Reference TANG, OBERPRIELER, YANG and Chen1999), Cycas species seem no less adapted for complex insect pollination mutualisms than cycads in the sister family Zamiaceae. Why then, if the Zamiaceae cycads are so exclusively insect pollinated, have Cycas species from across a broad geographic range retained at least some potential for limited ambophily?
Ambophily in Cycas – dispersal and survival
All cycads possess one of the requisite adaptations for wind pollination, in that their relatively large (and often multiple) male cones produce massive amounts of pollen, at least some of which is shed into the air (Jones Reference JONES2002, Figure 1b). That at least some Cycas species exhibit the potential for limited ambophily, while Zamiaceae cycads do not, is perhaps a fortuitous effect of the different female reproductive architecture in the two groups, Zamiaceae ovules being fully enclosed within a cone, while the layered megasporophylls of Cycadaceae are at least nominally more exposed to external air movements, especially if, as has been suggested, vectors such as rain could channel wind-blown pollen from external surfaces down towards the ovules (Hamada et al. Reference HAMADA, TERRY and MARLER2015a, Niklas & Norstog Reference NIKLAS and NORSTOG1984). Cycad-insect pollination systems are host-specific and Cycas ambophily would have high survival value in contexts where the availability of specific insect pollinators is limited, population density is high, and the plants grow in an open and windy environment. Such conditions would be applicable to founder events, such as the Miocene ocean crossing to Australia from South-East Asia (Hill Reference HILL and Chen1999), or the colonization (by means of floating seeds) of oceanic islands or coastal situations (Hill Reference HILL1994, Keppel et al. Reference KEPPEL, HODGSKISS and PLUNKETT2008), one example being the expansion of C. thouarsii to mainland Africa (Goode Reference GOODE2001). Hence, even a limited potential for ambophily may explain why Cycas has the widest distribution of any cycad genus, and is the only cycad genus to range across multiple continents and remote islands.
The complexity of cycad relationships with pollinating insects invites comparison with other examples of sustained coevolution in host-specific pollination systems (Cook et al. Reference COOK, BEAN, POWER and DIXON2004, Godsoe et al. Reference GODSOE, YODER, SMITH and PELLMYR2008, Herre et al. Reference HERRE, JANDER and MACHADO2008, Pellmyr Reference PELLMYR2003, Weiblen Reference WEIBLEN2002). But a surprising aspect of Cycas pollination is that it represents a specific, obligate pollination mutualism in which the taxon of the insect pollinator has changed repeatedly over space and time. Examples include the seemingly recent relationship between Carpophilus beetles and C. revoluta on the southern Japanese islands (Kono & Tobe Reference KONO and TOBE2007), the different beetle pollinators of Cycas (Alphitobius beetles and Derelomus weevils) on the northern and southern ends of the Western Ghats in India (Raju & Jonathan Reference RAJU and JONATHAN2010a, Reference RAJU and JONATHANb) and the possible novel pollination relationship with Anatrachyntis moths on Guam (Marler Reference MARLER2010, Terry et al. Reference TERRY, ROE, TANG and MARLER2009). A taxonomic affinity between the Cycadophila beetle pollinators of Cycas species in Asia, and Pharaxonotha beetle pollinators of Zamia cycads in the Americas (Xu et al. Reference XU, TANG, SKELLEY, LIU and RICH2015) suggests that some of the oldest cycad-insect pollination relationships may be extremely ancient, pre-dating the isolation of cycad floras by continental drift.
These shifts of different pollinators into the cycad coning system imply that the pre-adaptation of component parts in the mutualism can ostensibly lead to the rapid development of intricate, specific mutualisms with new pollinators. In cycads, this would have centred on the signals, such as volatile odours and thermogenesis, used to communicate the potential of cones as insect feeding or brood sites, it being the recognition of these signals that drives the host-specificity of the mutualism (Brookes et al. Reference BROOKES, HEREWARD, TERRY and WALTER2015, Roemer et al. Reference ROEMER, TERRY, CHOCKLEY and JACOBSEN2005, Suinyuy et al. Reference SUINYUY, DONALDSON and JOHNSON2015, Terry et al. Reference TERRY, HULL, MOORE and WALTER2007). But once such signals exist, perhaps after having originally evolved for some other purpose, they evidently have the potential to capture new pollinators, even following the loss of the original partner in the mutualism. Cycads thus suggest that complex host-specific pollination mutualisms can arise relatively quickly through the pre-adaptation of component parts, similar in some respects to the origin proposed for the intimate relationship between yuccas and their pollinating moths (Pellmyr et al. Reference PELLMYR, THOMPSON, BROWN and HARRISON1996). In the case of Cycas, there is not only evidence that such new, specific insect pollination mutualisms have arisen repeatedly, but in the potential for ambophily there is a mechanism by which Cycas can persist in the interim when novel insect pollination relationships are still evolving.
ACKNOWLEDGEMENTS
This study would not have been possible without the generous and sustained assistance of Brian Perry and family (Rockhampton, Central Queensland) and we thank them for their kind permission to work on the beautiful Cycas ophiolitica growing on their property. We also thank the cycad horticulturalists Peter Machin and Gary Kreutzmann for sharing their detailed knowledge of cycad lore. We are indebted to Michael Proctor of the University of Exeter for his advice on wind pollination effectiveness and William Tang of the US Department of Agriculture for sharing his detailed knowledge of cycad beetle taxonomy. The experiments on wild cycads discussed in this paper were undertaken with the Queensland Government Department of Environment and Resource Management scientific purposes permit number WITK06373009.