Introduction
The intertidal zone has long been an entrance and window on the ocean: people have foraged for food since pre-history (Thompson et al., Reference Thompson, Crowe and Hawkins2002); pioneering studies were made by the first generations of modern naturalists (Audouin & Edwards, Reference Audouin and Edwards1833; Darwin, Reference Darwin1854); and it was the playground for the early eco-tourists of the Victorian era (Gosse, Reference Gosse1856; Kingsley, Reference Kingsley1859; Hawkins et al., Reference Hawkins, Hiscock, Marsterson, Moore, Denny and Gaines2007). Early marine biologists used the seashore for collecting specimens for taxonomic, morphological, developmental, physiological and behavioural research (Hawkins et al., Reference Hawkins, Mieszkowska, Firth, Bohn, Burrows, Maclean, Thompson, Chan, Little and Williams2016). Here we review the contribution that experimental studies on rocky shores have made to more general ecological concepts and theory.
Formal ecological studies on rocky shores stretch back at least 200 years with Audouin & Edwards (Reference Audouin and Edwards1833) describing zonation patterns on the rocky shores of northern France. There then followed a long period of qualitative description and classification of broad patterns of distribution of conspicuous organisms on rocky shores. How zonation patterns were shaped by tidal height and wave exposure locally and how these differed geographically, culminated in the work of Stephenson & Stephenson (Reference Stephenson and Stephenson1949, Reference Stephenson and Stephenson1972), who proposed a universal scheme of zonation. Similar work, often by their many disciples, led to descriptions of zonation patterns worldwide, sometimes using versions of their three-zone scheme (e.g. Dakin, Reference Dakin1953, in Australia; Morton & Miller, Reference Morton and Miller1968, in New Zealand; Lewis, Reference Lewis1964, in the British Isles and Ireland; Morton & Morton, Reference Morton and Morton1983, in Hong Kong; Morton et al., Reference Morton, De Frias Martins and Britton1998, in the Azores) or developing new schemes fitting local or regional contexts (e.g. Ricketts & Calvin, Reference Ricketts and Calvin1968, on the Pacific Coast of America; Branch & Branch, Reference Branch and Branch1981, Reference Branch and Branch1986, in South Africa, updated in Branch and Branch, Reference Branch and Branch2018; see Raffaelli and Hawkins, Reference Raffaelli and Hawkins1996, for review of classical descriptive zonation schemes worldwide). T.A. Stephenson was a superb artist, as were many of his later emulators, although as colour photography emerged this aided description (see plates in Lewis, Reference Lewis1964). Many caveats arose for such qualitative classifications, especially on the seaweed-dominated sheltered shores of the North Atlantic (see Lewis, Reference Lewis1964 and Lewis's chapter in Stephenson & Stephenson, Reference Stephenson and Stephenson1972). Subsequently, Hawkins & Hartnoll (Reference Hawkins and Hartnoll1983a), from the basis of largely experimental studies, provided a possible explanation of the causes of the three-zone system on non-sheltered north-east Atlantic shores.
Qualitative studies gave way to more quantitative descriptions of distribution patterns, with precise levelling of intertidal elevation and counts made in quadrats (Southward & Orton, Reference Southward and Orton1954), or using semi-quantitative abundance scales (Moyse & Nelson-Smith, Reference Moyse and Nelson-Smith1963). Colman (Reference Colman1933), publishing in the Journal of the Marine Biological Association of the United Kingdom (JMBA), related the limits of individual species to critical tidal levels on the shore (see also Doty, Reference Doty1946; Evans, Reference Evans1947). Colman's work was later revisited and statistically refuted by Underwood (Reference Underwood1978). The phytosociological approach of the Zurich–Montpelier school was deployed to describe algal associations primarily in the Mediterranean Sea (above and below the water line; for examples see Boudouresque, Reference Boudouresque1971; Coppejans, Reference Coppejans1980). Phytosociology was also used by Russell (Reference Russell1972, Reference Russell1973) along with some early multivariate classification analysis to describe zonation patterns in the Irish Sea.
In parallel to descriptions, laboratory experiments were used to explore differential tolerances of species of algae (Baker, Reference Baker1909, Reference Baker1910) and invertebrates (Broekhuysen, Reference Broekhuysen1940; Foster, Reference Foster1971) from different tidal heights. Higher zoned organisms were shown to be more tolerant to desiccation and temperature extremes than those lower on the shore. Wolcott (Reference Wolcott1973) showed convincingly for mobile animals that whilst tolerance limits broadly matched zonation position, most limpet species stayed well within their tolerance limits, except for those bordering unexploited resources high on the shore; these took risks and got caught out when conditions changed. Experiments in the laboratory and on artificial panels on rafts, as well as on the shore, explored selective larval settlement behaviour. Barnacles and spirorbids were investigated by Knight-Jones (Reference Knight-Jones1951, Reference Knight-Jones1953), then taken on by Crisp and colleagues (Crisp & Knight-Jones, Reference Crisp and Knight-Jones1953; Crisp & Barnes, Reference Crisp and Barnes1954), initially at Menai Bridge in North Wales and then further afield in Britain (e.g. Millport, Barnes, Reference Barnes1956) and around the world (e.g. at Beaufort, North Carolina, Rittschof et al., Reference Rittschof, Branscomb and Costlow1984), mostly on barnacles related to fouling.
The first experiments in the field probably started in north-west France before the First World War (Hariot, Reference Hariot1909). Fischer-Piette and Hatton examined many aspects of biological interactions from the 1920s onwards at the Dinard Laboratory of the Paris Natural History Museum, using what would be recognized now as controlled field experiments (Hatton & Fischer-Piette, Reference Hatton and Fischer-Piette1932; Hatton, Reference Hatton1938). Post-war and encouraged by Orton, such approaches were taken up by Jones (Reference Jones1946, Reference Jones1948), Lodge (Reference Lodge1948), Burrows & Lodge (Reference Burrows and Lodge1951) and Southward (Reference Southward1956) at Port Erin, Isle of Man exploring the role of limpet grazing – although visibility of such work was not helped by publication in largely parochial journals (see Southward, Reference Southward1964 for summary). Connell (Reference Connell1961a, Reference Connell1961b) experimented on the shore at Millport in the early 1950s and subsequently took this approach back to California. Perhaps prompted by Renouf & Rees (Reference Renouf and Rees1932), Kitching, Ebling and colleagues and students used Lough Hyne in south-west Ireland as a model experimental system starting in the 1950s (Kitching et al., Reference Kitching, Sloane and Ebling1959; Kitching & Ebling, Reference Kitching and Ebling1961; Ebling et al., Reference Ebling, Sloane, Kitching and Davies1962). Some early work was also done in South Africa (Broekhuysen, Reference Broekhuysen1940) and Australia (Guiler, Reference Guiler1954; May et al., Reference May, Bennett and Thompson1970). Paine started his experimental work in the early 1960s on the North-west Pacific Coast of the USA (Paine, Reference Paine1966; Paine & Vadas, Reference Paine and Vadas1969) and elsewhere (New Zealand, Paine, Reference Paine1971; Chile, Paine et al., Reference Paine, Castillo and Cancino1985; summarized in Paine, Reference Paine1994). Such manipulative approaches expanded rapidly from the 1970s onwards, by the first students of Paine (Dayton, Reference Dayton1971, Reference Dayton1975; Menge, Reference Menge1976, Reference Menge1983; Vadas et al., Reference Vadas, Wright and Miller1990, Reference Vadas, Burrows and Hughes1994) and Connell (Choat, Reference Choat1977; Sousa, Reference Sousa1979a, Reference Sousa1979b) and in turn their numerous subsequent students, mentees and admirers (Lubchenco, Reference Lubchenco1978, Reference Lubchenco1980, Reference Lubchenco1983; Lubchenco & Menge, Reference Lubchenco and Menge1978; Gaines et al., Reference Gaines and Roughgarden1985) around the world. This led to significant early contributions in the southern hemisphere in Chile (Moreno & Jaramillo, Reference Moreno and Jaramillo1983; Paine et al., Reference Paine, Castillo and Cancino1985; Castilla & Paine, Reference Castilla and Paine1987; including the classic papers on excluding humans collecting seaweeds and invertebrates by Castilla and co-workers, Castilla & Duran, Reference Castilla and Duran1985; Castilla & Bustamante, Reference Castilla and Bustamante1989), New Zealand (Luckens, Reference Luckens1970, Reference Luckens1975a, Reference Luckens1975b) and Southern Africa (Branch, Reference Branch1975, Reference Branch1976). Much of this work focused on the role of biological interactions among species in shaping distributions or community structure.
Following in the footsteps of Paine and Connell, much excellent work has been done since the 1970s on both coasts of North America, by Dayton, Menge, Lubchenco, Gaines, Vadas, Bertness and many others (for recent overviews see Dudgeon & Petraitis, Reference Dudgeon, Petraitis, Hawkins, Bohn, Firth and Williams2019; Fenberg & Menge, Reference Fenberg, Menge, Hawkins, Bohn, Firth and Williams2019). This has in turn influenced work in Chile (Broitman et al., Reference Broitman, Navarrete, Smith and Gaines2001; Aguilera & Navarrete, Reference Aguilera and Navarrete2007, Reference Aguilera and Navarrete2012; Aguilera et al., Reference Aguilera, Navarrete and Broitman2013, Reference Aguilera, Broitman, Vásquez, Camus, Hawkins, Bohn, Firth and Williams2019a) and Argentina (Bertness et al., Reference Bertness, Crain, Silliman, Bazterrica, Reyna, Hildago and Farina2006; Silliman et al., Reference Silliman, Bertness, Altieri, Griffin, Bazterrica, Hidalgo, Crain and Reyna2011; Palomo et al., Reference Palomo, Bagur, Calla, Dalton, Soria, Hawkins, Hawkins, Bohn, Firth and Williams2019).
Underwood established a strong experimental ecology school in Sydney, Australia from the 1970s (e.g. Underwood, Reference Underwood1976, Reference Underwood1978, Reference Underwood1980, Reference Underwood1984, Reference Underwood1998; Underwood & Jernakoff, Reference Underwood and Jernakoff1981, Reference Underwood and Jernakoff1984; Underwood et al., Reference Underwood, Denley and Moran1983) with worldwide influence. He emphasized the importance of hypotheses tested using well-designed experiments and rigorous statistical analyses – any experiments done should be done properly (Underwood, Reference Underwood1981, Reference Underwood1985, Reference Underwood, Kikkawa and Anderson1986, Reference Underwood, Chelazzi and Vannini1988, Reference Underwood1991a, Reference Underwood, John, Hawkins and Price1992a). His approach was enthusiastically embraced by his numerous students (earlier ones including Creese & Underwood, Reference Creese and Underwood1982; Chapman, Reference Chapman1986; Fairweather, Reference Fairweather1988; Chapman & Underwood, Reference Chapman and Underwood1992), post-docs, visiting fellows, and a like-minded international network (e.g. Benedetti-Cecchi, A.R.O. Chapman, Coleman, Aberg, Pavia, Petraitis, Vadas).
Thus experimental studies on rocky shores had become mainstream from the 1980s – with several subsequent generations of students and post-docs worldwide (see reviews in John et al., Reference John, Price, Lawson, John, Hawkins and Price1992; Bertness et al., Reference Bertness, Gaines and Hay2001, Reference Bertness, Bruno, Silliman and Stachowicz2014; Hawkins et al., Reference Hawkins, Bohn, Firth and Williams2019a, Reference Hawkins, Pack, Firth, Mieszkowska, Evans, Martins, Åberg, Adams, Arenas, Boaventura, Bohn, Borges, Castro, Coleman, Crowe, Cruz, Davies, Epstein, Faria, Ferreira, Frost, Griffin, Hanley, Herbert, Hyder, Johnson, Lima, Masterson-Algar, Moore, Moschella, Notman, Pannacciulli, Ribeiro, Santos, Silva, Skov, Sugden, Vale, Wangkulangkul, Wort, Thompson, Hartnoll, Burrows, Jenkins, Hawkins, Bohn, Firth and Williams2019b, Reference Hawkins, Bohn, Lemasson, Williams, Schiel, Jenkins, Firth, Hawkins, Bohn, Firth and Williams2019c). In New Zealand, Choat brought back insights from his time with Connell in California (Choat, Reference Choat1977) and was an early champion of experimental approaches. Subsequently, Schiel has combined insight into the natural history of systems with rigorous experimental approaches (Schiel et al., Reference Schiel, Underwood, Chapman, Hawkins, Bohn, Firth and Williams2019a). In South Africa, Branch, McQuaid and students built on the classical work by the Stephensons to better understand, through experimentation, underlying processes (McQuaid & Blamey, Reference McQuaid, Blamey, Hawkins, Bohn, Firth and Williams2019). Experimental approaches have also prospered in Hong Kong and beyond in greater China (Williams et al., Reference Williams, Chan, Dong, Hawkins, Bohn, Firth and Williams2019).
The viewpoint of intertidal experimental ecology presented here started in the Isle of Man in the mid-1970s when the lead author (SJH) was prompted by Hartnoll to revisit the experimental work done by Jones, Lodge, Burrows and Southward in the late 1940s and 1950s. At the time key influences were the emerging experimental work by Paine, Connell, Menge and Lubchenco as well as the work by Southward at the Marine Biological Association (on long-term climate fluctuations and recruitment, Southward, Reference Southward1963, Reference Southward1967; recovery from oil-spills, Southward & Southward, Reference Southward and Southward1978; plus Lewis, Reference Lewis1964, and colleagues at Robin Hoods Bay on the role of recruitment processes on rocky shores and environmental monitoring, Lewis, Reference Lewis1976; Lewis & Bowman, Reference Lewis and Bowman1975; Bowman & Lewis, Reference Bowman and Lewis1977). Another influence was Norton at Glasgow, a former student of Burrows, who had also commenced experimental work on fucoid distributions on the shore at Millport (e.g. Schonbeck & Norton, Reference Schonbeck and Norton1978, Reference Schonbeck and Norton1980a). SJH with subsequent students, many of whom have become colleagues (including two co-authors on this paper) continued the tradition at Port Erin, on the Isle of Man with further experiments (e.g. Jenkins et al., Reference Jenkins, Norton and Hawkins1999a, Reference Jenkins, Hawkins and Norton1999b, Reference Jenkins, Hawkins and Norton1999c, 2005; Thompson et al., Reference Thompson, Norton and Hawkins2004 jointly working with Hartnoll and Norton), extending into spatial statistics (Johnson et al., Reference Johnson, Burrows, Hartnoll and Hawkins1997) and modelling (Burrows & Hawkins, Reference Burrows and Hawkins1998; Hyder et al., Reference Hyder, Johnson, Hawkins and Gurney1998; Johnson et al., Reference Johnson, Burrows and Hawkins1998a, Reference Johnson, Hughes, Burrows and Hawkins1998b, Reference Johnson, Hawkins, Hartnoll and Norton1998c). This has been aided by a wider network of colleagues in Europe (including an Italian co-author first met at a conference in Liverpool on plant–animal interactions in 1990), all interested in experimental ecology, and able to work together within European-funded projects and networks. This network of collaborators has been profoundly influenced by the Underwoodian approach to experimental ecology, promulgated by numerous training workshops by Underwood and Chapman and their advisory work on European projects such as EUROROCK.
Underwood et al. (Reference Underwood, Chapman and Connell2000) succinctly define and discuss different approaches to describing pattern in ecology, advocating the importance of logical and structured quantitative observations or surveys testing hypotheses about pattern, so-called mensurative experiments, as the essential basis upon which experimental studies of process can proceed. Such studies have advanced understanding of rocky shore ecology, and are often a stand-alone precursor (Menconi et al., Reference Menconi, Benedetti-Cecchi and Cinelli1999; Benedetti-Cecchi et al., Reference Benedetti-Cecchi, Acunto, Bulleri and Cinelli2000; Jenkins et al., Reference Jenkins, Åberg, Cervin, Coleman, Delany, Della Santina, Hawkins, Lacroix, Myers, Lindegarth, Power, Roberts and Hartnoll2000; Johnson et al., Reference Johnson, Frost, Mosley, Roberts and Hawkins2003; Martins et al., Reference Martins, Hawkins, Thompson and Jenkins2007, Reference Martins, Neto, Thompson, Hawkins and Jenkins2008) or a component of wider manipulative studies (Sousa, Reference Sousa1979a; Thompson et al., Reference Thompson, Norton and Hawkins2004) generating hypotheses or informing modelling approaches (Hyder et al., Reference Hyder, Johnson, Hawkins and Gurney1998, Reference Hyder, Åberg, Johnson and Hawkins2001; Svensson et al., Reference Svensson, Jenkins, Hawkins and Åberg2005). We have not, however, extensively considered them here, other than in the context of supply-side ecology where experimental manipulations are less feasible.
Here we focus on the use of rocky seashores as a convenient tractable system for manipulative field experiments on processes that have contributed to ecological concepts and theory. Firstly, we outline the reasons why the shore is so amenable to descriptive and experimental research. Next, we summarize the contributions that manipulative experimental intertidal ecological studies have made to general ecological theory at population, community and ecosystem levels. We then discuss the intertwining of theory and experiment, the importance of advances in methodology in developing the field and the opportunities arising from such new technologies and approaches. We conclude by considering how experimental approaches have helped understand the role of biological interactions in responses of marine life to anthropogenic climate change as well as informing environmentally sensitive adaptation by society to climate-driven rising seas in the context of widespread coastal urbanization. We stress this is a selective and personal view of the topic – not an exhaustive review. We focus mainly on the seashore but occasionally stray into the shallow sub-tidal zone, especially in the virtually tide-less Mediterranean. Furthermore, we have highlighted work done in Britain and Ireland plus neighbouring north-east Atlantic and Mediterranean countries – the traditional hinterland of JMBA. This work has led to the shores of the north-east Atlantic being some of the most studied in the world (Hawkins et al., Reference Aguilera, Valdivia, Jenkins, Navarrete and Broitman2019b), with much recent progress in the Mediterranean (Benedetti-Cecchi et al., Reference Benedetti-Cecchi, Airoldi, Bulleri, Fraschetti, Terlizzi, Hawkins, Bohn, Firth and Williams2019).
The seashore as a tractable system
Paine (Reference Paine1966, Reference Paine1969, Reference Paine1971, Reference Paine1974) and Connell (Reference Connell1961a, Reference Connell1961b, Reference Connell1972) as pioneers of experimental ecology have espoused the values of manipulative field experiments (Connell, Reference Connell and Mariscal1974; Paine, Reference Paine1994) and the appropriateness of the seashore as a test system (see also Menge & Branch, Reference Menge, Branch, Bertness, Gaines and Hay2001). As Connell (Reference Connell and Mariscal1974) neatly summarized: in a laboratory experiment all conditions are held constant other than those being tested; in contrast, in a field experiment all the variables are allowed to vary, presumably the same way in adjacent similar treatment and control plots, other than those being manipulated (often one or two, rarely more at the time of his writing, but more complex designs are now more commonplace). The seashore has a sharp vertical environmental stress gradient from low water to beyond the influence of sea spray. This gradient is largely unidirectional, increasing up shore for the majority of organisms on rocky shores that have marine evolutionary affinities. For the few organisms of terrestrial origins, the stress gradient is reversed (Raffaelli & Hawkins, Reference Raffaelli and Hawkins1996). The environmental extremes encountered over the short, sharp intertidal gradient are experienced over much larger distances in other systems. For example, moving a few metres up a rocky shore from low water can be the equivalent of ascending a 3000 m mountain in terrestrial systems. There is a horizontal wave action or exposure gradient between sheltered bays and exposed headlands. This is not unidirectional and many species have optima at intermediate points between the two extremes (Raffaelli & Hawkins, Reference Raffaelli and Hawkins1996). These sharp gradients are locally modified by the topography of the shore with rockpools, cracks and crevices plus shaded and unshaded areas reflecting geomorphologically generated habitat complexity (Johnson et al., Reference Johnson, Frost, Mosley, Roberts and Hawkins2003). This variety of environmental conditions in close proximity has made transplant experiments (with appropriate controls) a powerful tool in understanding the direct and indirect influences of the environment on the distribution, abundance, growth and survival of species (e.g. Schonbeck & Norton, Reference Schonbeck and Norton1978, Reference Schonbeck and Norton1980a). The modulation of the intensity of biological interactions by the physical environment can also be explored along these sharp local gradients (Bertness & Leonard, Reference Bertness and Leonard1997), and amongst local mosaics of micro-habitats (Johnson et al., Reference Johnson, Hughes, Burrows and Hawkins1998b, Reference Johnson, Edwards, Bunker and Maggs2005).
The nature of rocky intertidal organisms also makes them amenable to manipulation. Most intertidal organisms are reasonably sized with the biggest algae being at most 2–3 m in length. Few animals are bigger than 30 cm and most are less than 10 cm. Most of the suspension feeders are sessile, and many of the grazers and predators are reasonably slow-moving and sedentary enabling removal and exclusion experiments. Work over the last 20 years, however, has emphasized the importance of mobile predators such as foraging by crabs (Silva et al., Reference Silva, Hawkins, Boaventura and Thompson2008, Reference Silva, Hawkins, Clarke, Boaventura and Thompson2010, Reference Silva, Boaventura, Thompson and Hawkins2014; Christofoletti et al., Reference Christofoletti, Almeida and Ciotti2011) and fish (Taylor & Schiel, Reference Taylor and Schiel2010) when the tide is in, and birds when the tide is out (Hockey & Underhill, Reference Hockey and Underhill1984; Coleman et al., Reference Coleman, Goss-Custard, Durell and Hawkins1999; Coleman & Hockey, Reference Coleman and Hockey2008) or by diving (e.g. eider ducks, Hamilton et al., Reference Hamilton, Nudds and Neate1999). Few of the algae and animals live more than a decade or so – although there are exceptions such as Ascophyllum nodosum where genetic individuals may live well over 100 years, even if individual fronds are rarely older than 10 (Åberg, Reference Åberg1992). The relatively low cost and easy access to rocky shores also greatly facilitates the manipulation of these organisms for experiments.
Most conspicuous sessile animals and algae compete for a clearly definable and measurable resource – two-dimensional space to live on the rocks – providing access to light for plants and particulate food for suspension-feeders. The flora and fauna growing on rocky shores can also be partitioned into layers (canopy, understorey turf, encrustations) enabling non-destructive quantification of abundance and elucidation of interaction. There are none of the invisible and complex below-ground interactions that occur in soils and sediments – although bio-erosion by burrowing endolithic cyanobacteria and invertebrates such as sea urchins and molluscs, particularly burrowing piddocks (Pinn et al., Reference Pinn, Thompson and Hawkins2008) can modify softer rock habitats, creating complexity. These attributes make the flora and fauna easy to quantify and manipulate, and to measure response variables primarily non-destructively. Short lifespans and rapid growth mean that clearance or removal experiments of dominant canopy-forming or rock-covering species can occur within 3–5 years – the duration of most PhDs (but see note of caution from Jenkins & Uyà (Reference Jenkins and Uyà2016) and a plea for longer duration of manipulative marine studies). Some species do take longer, with maybe 20 or more years for the recovery of A. nodosum following canopy removal (Jenkins et al., Reference Jenkins, Norton and Hawkins2004; Ingólfsson & Hawkins, Reference Ingólfsson and Hawkins2008). Recovery times are, however, generally much swifter than in terrestrial systems where, for example, removal of trees may take hundreds of years to recover. When recovery does not occur, it can point to interesting explanations such as alternative stable states (Benedetti-Cecchi et al., Reference Benedetti-Cecchi, Tamburello, Maggi and Bulleri2015; Petraitis & Dudgeon, Reference Petraitis and Dudgeon2015; Menge et al., Reference Menge, Bracken, Lubchenco and Leslie2017).
The hard nature of rocks enables various devices such as exclusion fences or cages, settlement plates or tiles, or transplanted rocks to be attached via screws or quick-set cement or epoxy glues. Once technological constraints were overcome (e.g. early use of generators and electric-drills, Dayton Reference Dayton1970, or compressed air drills, Hawkins & Hartnoll, Reference Hawkins and Hartnoll1979), the experimental approach proliferated, particularly since the introduction of petrol-driven and cordless drills and better fast-setting underwater epoxies. Thus, grazers and predators have been excluded or enclosed at varying densities, algal canopies and understorey turfs and encrusting invertebrates removed, thinned or transplanted, and the responses of individual species or whole assemblages measured.
In most regions of the world, the taxonomy of the common and important species is well understood. Cryptic speciation can, however, cause problems (e.g. in the North Atlantic and Mediterranean: Chthamalus stellatus, into C. stellatus and Chthamalus montagui by Southward, Reference Southward1976; Fucus spiralis into F. spiralis and Fucus guiryi by Zardi et al., Reference Zardi, Nicastro, Canovas, Ferreira Costa, Serrão and Pearson2011). Recent developments in molecular-based genetics and taxonomy have accelerated the detection of cryptic species (Chan et al., Reference Chan, Tsang and Chu2007, Reference Chan, Kolbasov and Cheang2012). However, whilst taxonomic accuracy is essential at the population level, it is not critical for many community and ecosystem level studies. For example many community-level studies such as those making comparisons amongst biogeographic regions, usefully lump algae and sessile animal species into functional categories (canopies, turfs, encrustations, barnacles) or more nuanced functional groups (e.g. for algae, Steneck & Dethier, Reference Steneck and Dethier1994).
Contributions to general ecology theory
All of the above attributes have earned rocky shores the label as the fruit fly of ecology (Berlow, Reference Berlow1997). Below we consider how experimental research on rocky shores has contributed to general ecological theory at the population and especially the community level. Studies on rocky shores have made a major contribution to emphasizing how recruitment drives populations and the consequences for communities – what has become known as supply-side ecology (Lewin, Reference Lewin1986). Ground-breaking experiments on the roles of biological interactions in determining distributions and shaping communities through competition, grazing and predation, disturbance and succession, plus positive interactions are then outlined before turning to alternative stable states, the relationship between biodiversity and ecosystem functioning, and the relative importance of bottom-up forcing and top-down control in ecosystems.
Supply-side ecology
The mechanisms governing the distribution and abundance of populations of marine benthic invertebrates have been much debated. The majority of invertebrates living on rocky shores exhibit a complex life cycle with a pelagic larval phase. Hence local reproduction and input of new individuals to a particular site is often decoupled leading to extensive debate regarding the importance of processes that determine input of individuals (larval transport, supply and settlement) versus processes occurring after arrival (competition, predation, disturbance, facilitation following settlement) in determining patterns of adult distribution and abundance. Much early research on rocky shores focused on the dominant role of post-settlement processes in determining observed patterns (Connell, Reference Connell1961a, Reference Connell1961b; Paine, Reference Paine1966; Dayton, Reference Dayton1971; Menge & Sutherland, Reference Menge and Sutherland1976). For example, Connell's classic work on competition in Millport, Scotland showed clearly that the distribution of Chthamalus spp. on the shore was determined not by the pattern of settlement but by strong interspecific competition with Semibalanus balanoides. Connell (Reference Connell1961b) was very aware of the existence of failure years when S. balanoides recruitment did not occur, indicating a role for pre-settlement processes. The importance of larval supply and settlement in determining adult distribution can be represented by the recruitment limitation hypothesis (sensu Doherty, Reference Doherty1981) which states that when larval supply is insufficient for the total population size to reach a carrying capacity, increases in recruitment will lead to increases in adult population size (Connell, Reference Connell1985; Menge, Reference Menge1991).
The supply and settlement of both invertebrate larvae (Hawkins & Hartnoll, Reference Hawkins and Hartnoll1982a; Gaines & Roughgarden, Reference Gaines and Roughgarden1985; Roughgarden et al., Reference Roughgarden, Gaines and Possingham1988; Hunt & Scheibling, Reference Hunt and Scheibling1996; Jenkins et al., Reference Jenkins, Åberg, Cervin, Coleman, Delany, Della Santina, Hawkins, Lacroix, Myers, Lindegarth, Power, Roberts and Hartnoll2000; O'Riordan & Murphy, Reference O'Riordan and Murphy2000; McQuaid & Lawrie, Reference McQuaid and Lawrie2005) and algal propagules (Reed & Foster, Reference Reed and Foster1984; Åberg & Pavia, Reference Åberg and Pavia1997) varies greatly in both space and time. Observational studies that identify the scales at which variation occurs have given important insight into the mechanisms determining the distribution of larvae and ultimately supply to the shore (Gaines & Roughgarden, Reference Gaines and Roughgarden1985). Small-scale local variation tends to be ubiquitous (Fraschetti et al., Reference Fraschetti, Terlizzi and Benedetti-Cecchi2005), reflecting interactions occurring at or soon after settlement although larger-scale variability is common indicating the importance of patterns in the regional larval pool (Barnes, Reference Barnes1956) and regional physical transport processes (Hughes et al., Reference Hughes, Baird, Dinsdale, Moltschaniwskyj, Pratchett, Tanner and Willis1999). Decades of research have demonstrated clearly that understanding of processes such as upwelling (Roughgarden et al., Reference Roughgarden, Gaines and Possingham1988), tidal and wind-driven currents (Hawkins & Hartnoll, Reference Hawkins and Hartnoll1982a, Reference Hawkins and Hartnoll1982b; Bertness et al., Reference Bertness, Gaines and Wahle1996; McQuaid & Phillips, Reference McQuaid and Phillips2000), internal waves (Shanks, Reference Shanks1983), surf zone barriers (Rilov et al., Reference Rilov, Dudas, Menge, Grantham, Lubchenco and Schiel2008) and wave action (Pfaff et al., Reference Pfaff, Branch, Wieters, Branch and Broitman2011), and the way these processes interact with local and regional coastal topography (Archambault & Bourget, Reference Archambault and Bourget1996) is required to provide predictive understanding of supply to the rocky shore. Work of Roughgarden and colleagues on the central Californian coast showed convincingly that inter-annual variability in upwelling determines patterns of recruitment across large areas (Roughgarden et al., Reference Roughgarden, Gaines and Possingham1988; Farrell et al., Reference Farrell, Bracher and Roughgarden1991); while further north on the coast of Washington, barnacle recruitment rates are influenced by shoreward transport of offshore plankton, in surface slicks, generated by tidally forced internal waves (Shanks, Reference Shanks1983, Reference Shanks1986; Shanks & Wright, Reference Shanks and Wright1986).
While physical transport processes determine the rate and timing of arrival of larvae and propagules to the shore, consideration of behaviour is paramount in determining settlement patterns (Jenkins, Reference Jenkins2005). Some population or community models assume that settlement is a function of larval supply and the amount of free space available (Gaines et al., Reference Gaines, Brown and Roughgarden1985; Roughgarden et al., Reference Roughgarden, Iwasa and Baxter1985; Hyder et al., Reference Hyder, Åberg, Johnson and Hawkins2001). However, reality may be far more complex. For example, Minchinton (Reference Minchinton1997) demonstrated that recruitment of the tubeworm Galeolaria caespitosa into patches of free space was related not to patch area but to patch perimeter suggesting an important role for gregarious settlement close to conspecifics. The work of Crisp and co-workers in Menai Bridge, Wales throughout the 1950s and 1960s was critical in demonstrating the complex patterns of behaviour and finely tuned discriminatory abilities of invertebrate larvae in response to biological and physical cues to ensure that settlement occurs in a habitat that is conducive to survival, growth and, ultimately, reproduction. For example, the microbiofilm coating the rocky shore is the point of first attachment for settling larvae and propagules. Such films have been shown to promote settlement in a range of invertebrate larvae (Todd & Keough, Reference Todd and Keough1994; Qian et al., Reference Qian, Lau, Dahms, Dobretsov and Harder2007), with settlers responding to cues such as age of the film, specific taxa such as diatoms, and film characteristics related to tidal height (Strathmann et al., Reference Strathmann, Branscomb and Vedder1981). Laboratory analyses of behaviour have demonstrated the discriminatory abilities of invertebrate larvae to a range of cues, but perhaps field tests of how larvae respond to multiple cues are more relevant. Thompson et al. (Reference Thompson, Norton and Hawkins1998) showed that settling barnacle cyprids could discriminate among different microbial films in the laboratory environment, but this was irrelevant under field conditions where the presence of conspecific cues overruled cues from microbiota. Grosberg (Reference Grosberg1981) demonstrated that the larvae of species that are vulnerable to overgrowth by competitors avoid settling in the presence of a dominant space occupant, the colonial tunicate Botryllus schlosseri; whereas no avoidance was displayed by species that are not vulnerable. However, a contrasting response was recorded by Bullard et al. (Reference Bullard, Whitlatch and Osman2004), who failed to detect any avoidance of competitors during larval settlement.
The relevance of spatial and temporal variation in larval supply to adult populations is ultimately dictated by the fate of settlers. No matter how variable the supply of larvae to the shore, if the post-settlement process is density-dependent then the variability will be dampened out (Holm, Reference Holm1990; Jenkins et al., Reference Jenkins, Moore, Burrows, Garbary, Hawkins, Ingólfsson, Sebens, Snelgrove, Wethey and Woodin2008a). New arrivals to the rocky shore are subject to radically different environmental conditions than those experienced in the pelagic larval environment, and early post-settlement mortality is high. For example, Gosselin & Qian (Reference Gosselin and Qian1997) reported levels of over 90% mortality during the juvenile period in 20 out of 30 studies reviewed on invertebrates. The same is true for algae; for example, Wright et al. (Reference Wright, Williams and Dethier2004) showed that only 2 of 5395 embryos of Fucus gardneri survived to become visible recruits. Sources of mortality include desiccation and disturbance, both physical (Vadas et al., Reference Vadas, Wright and Miller1990) and biological (Jenkins et al., Reference Jenkins, Norton and Hawkins1999a).
The integration of levels of larval supply, patterns of settlement and post-settlement mortality dictate the abundance and distribution of adults on rocky shores. Following much debate, based on work on rocky shores and by extensive work on coral reef fish (Doherty & Williams, Reference Doherty and Williams1988; Shima, Reference Shima2001; Wilson & Osenberg, Reference Wilson and Osenberg2002), a general consensus was reached whereby populations may be limited by arrival of new individuals when supply and settlement is relatively low, but at high recruitment sites variability in supply and settlement has little impact on adult abundance owing to strong density-dependent post-settlement processes. This recruit-adult hypothesis (sensu Menge, Reference Menge2000a) states that the relative importance of recruitment declines with increasing density of recruits (Connell, Reference Connell1985; Roughgarden et al., Reference Roughgarden, Iwasa and Baxter1985; Sutherland, Reference Sutherland1990; Menge, Reference Menge2000b). Manipulative experimental work in North Wales on the intertidal barnacle S. balanoides which shows meso-scale variation in density and recruitment around Anglesey (Bennell, Reference Bennell1981; Hyder et al., Reference Hyder, Johnson, Hawkins and Gurney1998), partially supports this view, but also shows that recruitment can be a useful predictor of adult density across all recruit densities, from very low to very high (Jenkins et al., Reference Jenkins, Moore, Burrows, Garbary, Hawkins, Ingólfsson, Sebens, Snelgrove, Wethey and Woodin2008a). Here the relationship between recruitment and adult density switched from positive, at low levels, to negative at high levels (Figure 1) owing to strong over-compensatory density-dependent mortality. Such considerations are clearly relevant to understand how populations are regulated. However, the supply of individuals across a range of taxa can also be used in a predictive manner to consider the structuring forces in whole communities. Consistent large-scale differences in recruitment intensity observed along the Pacific coast of North America (Connolly et al., Reference Connolly, Menge and Roughgarden2001) can lead to differences in the strength of interspecific interactions. Experimental determination of community organization in Oregon and Washington where recruitment is high has emphasized the role of competition and predation. In contrast, in California, where upwelling-induced larval loss from the coastline is high, the influence of settlement variation has been stressed (Connolly & Roughgarden, Reference Connolly and Roughgarden1998).

Fig. 1. The relationship between the density of manipulated recruits of S. balanoides in June 2002 on shores of North Wales and the density of surviving adults 2 years later. On both shores, adult density is positively related with recruitment at low densities, switching to no relationship at moderate densities and a negative relationship when recruitment is very high. From Jenkins et al. (Reference Jenkins, Moore, Burrows, Garbary, Hawkins, Ingólfsson, Sebens, Snelgrove, Wethey and Woodin2008a).
The interplay of ecological theory, data collection, and modelling generated many excellent examples of early interdisciplinary research. Here, collaborations between ecologists and modellers led to significant advances in understanding of ecology, where cyclic interactions between experimental work and modelling occurred. Data were generated to understand processes that were then used to parameterize models; models highlighted data gaps and generated hypotheses for further experiments, which in turn improved models.
Mechanistic models of barnacles have successfully linked small-scale dynamics of adult benthic populations to large-scale hydrodynamic models of the larval stage (Roughgarden et al., Reference Roughgarden, Iwasa and Baxter1985, Reference Roughgarden, Gaines and Pacala1987, Reference Roughgarden, Running and Matson1991; Possingham & Roughgarden, Reference Possingham and Roughgarden1990; Shkedy & Roughgarden, Reference Shkedy and Roughgarden1997; Connolly & Roughgarden, Reference Connolly and Roughgarden1998). Thus, a demographic theory for an open population with space-limited recruitment has been proposed and tested using data for the barnacle Balanus glandula in California (Gaines et al., Reference Gaines, Brown and Roughgarden1985; Gaines & Roughgarden, Reference Gaines and Roughgarden1985; Roughgarden et al., Reference Roughgarden, Iwasa and Baxter1985). The model predicted cyclic fluctuations in free space at high settlement rates, but could not explain the 30-week period of these cycles (Roughgarden et al., Reference Roughgarden, Iwasa and Baxter1985), which led to further experiments and modelling to develop understanding of the system. Later models showed that at critical density of barnacles they are subject to density-dependent predation by starfish; but at low density the threshold for predation is not reached and free space is always present (Possingham et al., Reference Possingham, Tuljapurkar, Roughgarden and Wilks1994). Coupled models of oceanic processes of the larval stage and adult benthic stage were developed (Possingham & Roughgarden, Reference Possingham and Roughgarden1990; Roughgarden et al., Reference Roughgarden, Running and Matson1991). Farrell et al. (Reference Farrell, Bracher and Roughgarden1991) showed that the supply of larvae was inversely proportional to the strength of upwelling and that larvae were passively transported offshore to the oceanic front. This gave rise to models where the larvae were produced and passively transported to the frontal boundary. The collapse of upwelling leads to areas of the front colliding with the shore and giving peaks of recruitment (Roughgarden et al., Reference Roughgarden, Running and Matson1991). The predictions from models coupling large-scale oceanic processes that affect the larvae with small-scale benthic dynamics have led to development of a community model for California and Oregon (Connolly & Roughgarden, Reference Connolly and Roughgarden1998).
Modelling has also been used to examine the factors structuring barnacle populations across Europe (Hyder et al., Reference Hyder, Johnson, Hawkins and Gurney1998, Reference Hyder, Åberg, Johnson and Hawkins2001; Svensson et al., Reference Svensson, Jenkins, Hawkins and Åberg2005). For example, Hyder et al. (Reference Hyder, Åberg, Johnson and Hawkins2001) applied open models with space-limited recruitment to large-scale observations of Chthamalus montagui and other barnacles across Europe (Jenkins et al., Reference Jenkins, Åberg, Cervin, Coleman, Delany, Hawkins, Hyder, Myers, Paula and Power2001; O'Riordan et al., Reference O'Riordan, Arenas, Arrontes, Castro, Cruz, Delany, Martinez, Fernandez, Hawkins, McGrath, Myers, Oliveros, Pannacciulli, Power, Relini, Rico and Silva2004). Modelling demonstrated differences between the processes structuring barnacle populations in the Atlantic and Mediterranean (Hyder et al., Reference Hyder, Åberg, Johnson and Hawkins2001). The models showed the amount of free space varied within a year, but the pattern differed between sites in the Atlantic and Mediterranean (Hyder et al., Reference Hyder, Åberg, Johnson and Hawkins2001). Modelled free space represented the balance between space-filling and space-creating processes, which may reflect differences in the timing of processes in the Atlantic (mortality due to storms and low growth in winter) and Mediterranean (mortality due to desiccation and low growth in summer) (Hyder et al., Reference Hyder, Åberg, Johnson and Hawkins2001). Genetic differentiation has been found between Mediterranean and Atlantic C. montagui (Dando & Southward, Reference Dando and Southward1980; Pannacciulli et al., Reference Pannacciulli, Bishop and Hawkins1997), which could be driven by neutral processes resulting from hydrographic separation and/or variation in timing of selective pressures. This combination of modelling and experimental/empirical work has led to major advances in understanding of the processes driving open populations for which barnacles have proved an excellent test system.
Zonation along environmental gradients
Connell's (Reference Connell1961a) classical experiment on competition between fast-growing mid and low shore Semibalanus balanoides and slower-growing high shore Chthamalus montagui at Millport showed the importance of biological interactions along environmental gradients in setting lower limits of zonation of individual species (for similar work in New Zealand see Luckens, Reference Luckens1970, Reference Luckens1975a, Reference Luckens1975b). This idea was reinforced through observations of predation setting lower limits (Connell, Reference Connell1961b, Reference Connell1970; Paine, Reference Paine1971, Reference Paine1974; Paine et al., Reference Paine, Castillo and Cancino1985). In contrast laboratory simulations, early transplant/environment modification experiments (Luckens, Reference Luckens1970) and field observations of mortality at upper limits led Connell (Reference Connell1972) to propose that the upper limits of zonation of species were generally set by the direct effects of physical factors with lower limits being set by biological interactions. Connell's conceptual leap forward on understanding zonation patterns of species along environmental gradients was to suggest that the proximate causes of upper and lower limits had to be treated separately. Subsequent experimental removal and transplant studies, backed by realistic laboratory tolerance experiments on fucoid zonation on sheltered shores on both sides of the Atlantic, confirmed the role of physical factors setting upper limits (Schonbeck & Norton, Reference Schonbeck and Norton1978, Reference Schonbeck and Norton1979a, Reference Schonbeck and Norton1979b, Reference Schonbeck and Norton1979c, Reference Schonbeck and Norton1980b) and biological interactions, particularly competition in setting lower limits (Lubchenco, Reference Lubchenco1980; Schonbeck & Norton, Reference Schonbeck and Norton1980a).
Some exceptions have since emerged to Connell's synthesis. Low on the shore, upper limits were shown to be set by grazing on more wave-exposed shores (e.g. Southward & Southward, Reference Southward and Southward1978; Underwood, Reference Underwood1980; Underwood & Jernakoff, Reference Underwood and Jernakoff1981; Boaventura et al., Reference Boaventura, Alexander, Santina, Smith, Ré, da Fonseca and Hawkins2002a). On sheltered shores, competition was shown to set the upper limits of some low and mid-shore fucoids, as well as lower limits (Hawkins & Hartnoll, Reference Hawkins and Hartnoll1985, but see comments by Underwood, 1991a). Ascophyllum in particular was shown in removal experiments to exclude both higher shore Fucus vesiculosus and lower shore Fucus serratus from midshore plots (Jenkins et al., Reference Jenkins, Hawkins and Norton1999b, Reference Jenkins, Hawkins and Norton1999c, Reference Jenkins, Norton and Hawkins2004); this competitive ability is because it can grow large via vegetative proliferation and its longevity rather than fast early growth (Johnson et al., Reference Johnson, Hawkins, Hartnoll and Norton1998c). Ultimately the physical stress gradient from low shore to high shore determines survival, growth and hence competitive performance of algae and sessile invertebrate species. Proximately upper limits, especially for higher shore species, are set by physical factors and although generally lower limits are set by biological interactions, upper limits of mid and low shore species can also be set by biological interactions such as competition and grazing. There is no convincing evidence that lower limits of essentially marine organisms are set directly by too much emersion – in Pelvetia canaliculata, which rots when transplanted lower down the shore (Schonbeck & Norton, Reference Schonbeck and Norton1980a; Rugg & Norton, Reference Rugg and Norton1986), a pathogen has been shown to cause mortality at lower tidal levels.
Competition
Much valuable work has been done on competition amongst mobile animals, particularly grazing gastropods. Experimental designs that segregate intra- from interspecific competition are crucial when understanding interactions between species; such designs were pioneered by Underwood (e.g. Reference Underwood1978, Reference Underwood1984, Reference Underwood, Kikkawa and Anderson1986, Reference Underwood, Chelazzi and Vannini1988, Reference Underwood, John, Hawkins and Price1992a) working with his students (e.g. Creese & Underwood, Reference Creese and Underwood1982; Fletcher & Creese, Reference Fletcher and Creese1985). Intraspecific competition as expected is often more intense than interspecific competition (Creese & Underwood, Reference Creese and Underwood1982; Ortega, Reference Ortega1985; Boaventura et al., Reference Boaventura, da Fonseca and Hawkins2002b), enabling competitively inferior species to persist, especially if they, like Siphonaria, have access to a resource untapped by the superior competitor such as algae growing on Cellana shells (Creese & Underwood, Reference Creese and Underwood1982; see comments in Underwood, Reference Underwood, John, Hawkins and Price1992a, Reference Underwood1992b). Inter-age class interactions within a species can also be important, with larger animals impacting smaller size classes (e.g. Boaventura et al., Reference Boaventura, da Fonesca and Hawkins2003), probably through competition for both food and space. The converse has also been found, with faster growing smaller animals outcompeting larger limpets (Marshall & Keough, Reference Marshall and Keough1994). Recently the potential role of competitive interactions among gastropods in limiting range expansion and hence determining range limits has been examined using scurrinid limpets in the south-east Pacific as a model system. Aguilera et al. (Reference Aguilera, Valdivia, Jenkins, Navarrete and Broitman2019b) showed novel evidence of asymmetric competition between two congeners potentially limiting range expansion by Scurria viridula.
Whilst competition is clearly important there are emerging context-dependent caveats. Firstly resources, whether space or food, need to be in short supply. This can depend very much on local and mesoscale differences in larval or propagule supply discussed above. Competitive outcomes can also be modified by both fluctuating environmental conditions and recruitment – very common in intertidal systems – leading to co-existence of species due to absence of the equilibrium conditions theoretically needed for competitive exclusion. For instance, Connell (Reference Connell1961a) was able to demonstrate intense competition between barnacle species because space is highly saturated due to larval retention by fronts in the Firth of Clyde. Such retention leads to very high local recruitment compared for example with more dispersive coastal environments such as the Isle of Man (Hawkins & Hartnoll, Reference Hawkins and Hartnoll1982a). Work elsewhere can lead to different outcomes (e.g. Gordon & Knights, Reference Gordon and Knights2018), especially where space is undersaturated due to mesoscale recruitment differences (Jenkins et al., Reference Jenkins, Åberg, Cervin, Coleman, Delany, Hawkins, Hyder, Myers, Paula and Power2001; Burrows et al., Reference Burrows, Jenkins, Robb and Harvey2010), or to low recruitment including failure years (Svensson et al., Reference Svensson, Jenkins, Hawkins and Åberg2005). Further south in the geographic range of Semibalanus in south-west England, early juvenile mortality of the dominant Semibalanus in warm years probably releases slower growing chthamalids from competition (Poloczanska et al., Reference Poloczanska, Hawkins, Southward and Burrows2008). Even where space is apparently undersaturated, gregarious settlement behaviour can lead to intra-specific competition (e.g. Gordon & Knights, Reference Gordon and Knights2018), whilst maximizing survival and mating opportunities (Kent et al., Reference Kent, Hawkins and Doncaster2003).
Predation and grazing
Pioneering work by Paine (Reference Paine1966), in which the large predator Pisaster ochraceus was experimentally removed from a mussel-dominated (Mytilus californianus) shore in Washington State, led to the keystone predator concept. Paine showed that diversity of conspicuous fauna declined in the absence of this large predator. In addition, continuation of this removal experiment for several years showed that predation could also set the lower limit of mussels (Paine, Reference Paine1974). Paine went on to repeat this experiment in New Zealand (Paine, Reference Paine1971) and Chile (Paine et al., Reference Paine, Castillo and Cancino1985), showing that in biogeographic settings where large starfish were prevalent (Stichaster australis in New Zealand and Heliaster helianthus in Chile) and mussels were the dominant space occupier, that a major ‘keystone’ predator could have a disproportionate effect on the diversity and distribution of species (Paine, Reference Paine1969).
Ahead of these experiments, removals of patellid limpets in large squares or strips by Jones (Reference Jones1946, Reference Jones1948) on the Isle of Man showed that the control of fucoid algal vegetation on moderately exposed rocky shores was by limpet grazing, not by wave action as previously assumed (see also Conway, Reference Conway1946, for work in the Firth of Clyde). Although some of the work was published in Nature, the remainder of the more detailed follow-up work on the Isle of Man was largely published in local journals (Jones, Reference Jones1948; Lodge, Reference Lodge1948; Burrows & Lodge, Reference Burrows and Lodge1950; Southward, Reference Southward1956). Southward (Reference Southward1964) summarized this work in a chapter for a conference organized by the British Ecological Society. The importance of limpet grazing was starkly confirmed by the toxic effects of first-generation dispersants used to clean up the Torrey Canyon oil spill in 1967 (Nelson-Smith, Reference Nelson-Smith1968; Smith, Reference Smith1968). This was one of the first spills from the newer generation of super-tankers that ran onto reefs off the west Cornish coast. Vast amounts of ‘detergents’, a euphemism for a mix of surfactant, organic solvent and stabilizer, were applied to the crude oil that came ashore. A total of 10,000 tons of dispersant were applied to ~14,000 tons of oil that came ashore in Cornwall (Smith, Reference Smith1968). This led to vast kills of limpets and other grazers leading to an immediate bloom of green algae, followed by fucoids, which even occurred on some of the most exposed shores in Cornwall such as outside Sennen Cove (Southward & Southward, Reference Southward and Southward1978). Recovery of these treated shores took between 10–15 years (Southward & Southward, Reference Southward and Southward1978; Hawkins & Southward, Reference Hawkins, Southward and Thayer1992; Hawkins et al., Reference Hawkins, Southward and Barrett1983, Reference Hawkins, Evans, Mieszkowska, Adams, Bray, Burrows, Firth, Genner, Leung, Moore, Pack, Schuster, Sims, Whittington and Southward2017a, Reference Hawkins, Evans, Moore, Whittington, Pack, Firth, Adams, Moore, Masterson-Algar, Mieszkowska and Southward2017b). Those observations confirmed that grazing, rather than physical factors, controlled algal vegetation and that limpets can be considered a keystone grazer on the rocky shores of the north-east Atlantic (Hawkins & Hartnoll, Reference Hawkins and Hartnoll1983a).
Starting with removing limpets from strips or patches (Hawkins, Reference Hawkins1981a), a more nuanced approach using limpet exclusion fences (Hawkins, Reference Hawkins1981b) was then adopted by the next generation of researchers on the Isle of Man (reviewed in Hawkins & Hartnoll, Reference Hawkins and Hartnoll1983a). This teased out the interactions between limpets, barnacles and fucoids (Hawkins & Hartnoll, Reference Hawkins and Hartnoll1982b, Reference Hawkins and Hartnoll1983b; Hawkins, Reference Hawkins1983), and the role of recruitment fluctuations (Hawkins & Hartnoll, Reference Hawkins and Hartnoll1982a) in generating patchiness on exposed shores (Hawkins & Hartnoll, Reference Hawkins and Hartnoll1983a; Hartnoll & Hawkins, Reference Hartnoll and Hawkins1985). Some of this work was correctly criticized for lack of replication (Chapman, Reference Chapman1995). Subsequent studies used extensive replication over replicated patches, seasons and shores and on large geographic scales (Arrontes et al., Reference Arrontes, Arenas, Fernandez, Rico, Oliveros, Martinez, Viejo and Alvarez2004; Jenkins et al., Reference Jenkins, Coleman, Santina, Hawkins, Burrows and Hartnoll2005; Coleman et al., Reference Coleman, Underwood, Benedetti-Cecchi, Åberg, Arenas, Arrontes, Castro, Hartnoll, Jenkins, Paula, Santina and Hawkins2006) confirmed the importance of limpet grazing in controlling algal vegetation, especially fucoids, in more northerly latitudes. Further north in Europe (i.e. the Isle of Man) the response to limpet removal was highly deterministic, but even in southern Britain a greater stochastic element was apparent (Jenkins et al., Reference Jenkins, Coleman, Santina, Hawkins, Burrows and Hartnoll2005). Follow-up work comparing the factors determining fucoid recruitment in Portugal vs the British Isles showed that interactions between reproductive output, propagule supply and physical factors, particularly desiccation and grazing, influenced fucoid recruitment (Ferreira et al., Reference Ferreira, Hawkins and Jenkins2015). In the south of Portugal, fucoid cover and biomass is reduced, propagule supply is less and physical factors reduce early growth and recruitment; whereas in the north, in less stressful conditions with ample propagule supply, grazing has a prominent regulatory role (Ferreira et al., Reference Ferreira, Arenas, Martínez, Hawkins and Jenkins2014).
Spatial mapping of mosaics using geo-statistical tools (Johnson et al., Reference Johnson, Burrows, Hartnoll and Hawkins1997), probabilistic individual-based modelling (Johnson et al., Reference Johnson, Burrows and Hawkins1998a) and approaches using cellular automata (Burrows & Hawkins, Reference Burrows and Hawkins1998) have built on the underlying experiments (summarized above and in Hawkins et al., Reference Hawkins, Hartnoll, Kain, Norton, John, Hawkins and Price1992) enabling simulation of the processes involved generating patchy mosaics on moderately exposed rocky shores. Jonsson et al. (Reference Jonsson, Granhag, Moschella, Åberg, Hawkins and Thompson2006), using a combination of experiments and modelling on artificial breakwaters, showed conclusively that limpets prevent establishment of fucoids, but wave action determines their persistence once established.
The keystone concept has received some criticism (Mills et al., Reference Mills, Soulé and Doak1993; Power et al., Reference Power, Tilman, Estes, Menge, Bond, Mills, Daily, Castilla, Lubchenco and Paine1996). It is clearly context-dependent: appropriate large-bodied predatory and grazing species have to be present to be able to perform this role (i.e. large starfish in the Indo-Pacific; large limpets in the north-east Atlantic) and there have to be dominant space occupiers such as mussels or fucoid canopy-forming algae that are controlled by an important consumer (Power et al., Reference Power, Tilman, Estes, Menge, Bond, Mills, Daily, Castilla, Lubchenco and Paine1996).
Disturbance and succession
Rocky shores are naturally disturbed environments. The direct action of waves and indirect action through scouring as a consequence of movements of sand, gravel, and turning over cobbles and boulders (Shanks & Wright, Reference Shanks and Wright1986) remove biota thereby starting secondary succession (Connell & Slatyer, Reference Connell and Slatyer1977). Primary succession can also be initiated by chunks of rock becoming cleaved away or the placement of new blocks on artificial shores (Hawkins et al., Reference Hawkins, Southward and Barrett1983; Moschella et al., Reference Moschella, Abbiati, Åberg, Airoldi, Anderson, Bacchiocchi, Bulleri, Dinesen, Frost, Gacia, Granhag, Jonsson, Satta, Sundelöf, Thompson and Hawkins2005) creating virgin surfaces. In addition to physical processes, biological disturbance can arise from grazing, predation, sweeping or whiplash by canopy algae such as kelps or fucoids (Velimirov & Griffiths, Reference Velimirov and Griffiths1979; Hawkins, Reference Hawkins1983), and even intense intraspecific competition leading to hummocking of barnacles (Barnes & Powell, Reference Barnes and Powell1950). All these biological processes can lead to space being made available for secondary or even tertiary succession (on top of existing biota). This is particularly the case when grazing is relaxed, leading to algal colonization (Hawkins, Reference Hawkins1981b). Grazing pressure can keep an invisible lawn of biofilm on apparently bare rock and on barnacles (Hill & Hawkins, Reference Hill and Hawkins1991).
It is, therefore, not surprising that empirical studies of disturbance and succession have been made on rocky shores, and this work has led to conceptual advances (Connell & Slatyer, Reference Connell and Slatyer1977; Sousa, Reference Sousa1979a, Reference Sousa1979b, Reference Sousa1984a, Reference Sousa1984b). Connell & Slatyer (Reference Connell and Slatyer1977) proposed three models of succession: classical facilitation where early stages are essential for progression to later arriving species; inhibition where early or mid-successional species delayed progression to later arriving species; and the tolerance model (which nowadays would be termed a neutral model) where there are no positive or negative interactions, but species eventually came to predominate because of life history characteristics such as longevity.
In the literature, there are many examples of facilitation and inhibition (Menge, Reference Menge1995), although less for the tolerance model (but see Farrell, Reference Farrell1991). Facilitation can be particularly important during primary succession (Wahl, Reference Wahl1989), when microbial films condition the surface. There is, however, some evidence of inhibition even in microbial films (Wieczorek et al., Reference Wieczorek, Clare and Todd1995). Inhibition commonly occurs when early colonizing macro-algae such as ephemeral greens and browns come to dominate rocks. Sousa (Reference Sousa1979a) determined the importance of grazing in breaking dominance by intermediate stages thereby breaking inhibition to allow succession to proceed. The paucity of evidence for the tolerance model may simply be because studies have not been run for long enough (Jenkins & Uyà, Reference Jenkins and Uyà2016, for consideration of the temporal scale of manipulative experiments). In long-term experiments on Ascophyllum-dominated sheltered shores of the north-east Atlantic examples of the tolerance model may be found. Clearance areas were initially dominated by Fucus serratus and F. vesiculosus. However, slow recolonization over 10–20 years by A. nodosum occurred through its ability to grow up through an overlying canopy of shorter-lived competitors that led toward its return to dominance, maintained by proliferating vegetatively in long-lived clumps (Jenkins et al., Reference Jenkins, Hawkins and Norton1999b, Reference Jenkins, Norton and Hawkins2004; Ingolfsson & Hawkins, Reference Ingólfsson and Hawkins2008). These models are more classifications than a predictive framework, with Hawkins & Hartnoll (Reference Hawkins and Hartnoll1983b) observing that some successional sequences can involve more than one model.
Benedetti-Cecchi (Reference Benedetti-Cecchi2000a) revisited the successional models of Connell & Slatyer (Reference Connell and Slatyer1977). He used a combination of traits from knowledge of natural history and outcomes of past experiments on the importance of positive and negative interactions to turn Connell & Slatyer's (Reference Connell and Slatyer1977) models of succession into predictive, but still qualitative models of succession (Figure 2). This study shows how positive indirect interactions may facilitate the establishment of species that characterize early successional stages later in succession, challenging the idea of succession as a directional sequence of species replacements.
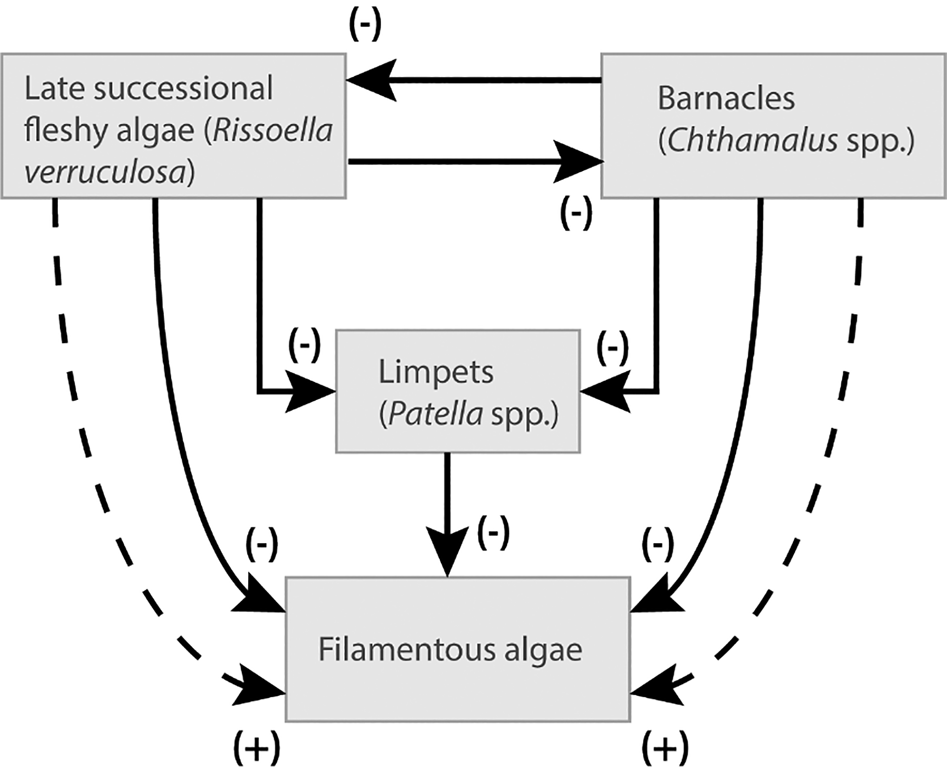
Fig. 2. Network of species interactions shaping rocky shores assemblages at late stages of succession on a Mediterranean rocky shore. Strong competition for space between late successional species (fleshy algae and barnacles) and limpets reduces grazing pressure in the mature assemblage, indirectly facilitating the persistence of early colonizers (filamentous algae) at late stages of succession. Continuous (dashed) lines indicate direct (indirect) interactions. (+) positive interaction; (−) negative interaction. Redrawn from Benedetti-Cecchi (Reference Benedetti-Cecchi2000a).
Connell & Slatyer (Reference Connell and Slatyer1977) stated that ‘succession never stops’ and if the broadest definition of succession as a sequence of colonization following a disturbance event is adopted, then this is certainly the case, especially on rocky shores where both physical and biological disturbance is prevalent. The intermediate disturbance hypothesis (Connell, Reference Connell1978) has been extensively explored on rocky shores. Sousa (Reference Sousa1979b) worked on boulders of different sizes and their likelihood of turning over from wave action, showing middle-sized boulders experiencing intermediate disturbance had the highest algae diversity. Lubchenco (Reference Lubchenco1978) examined biological disturbance by grazing littorinids in rockpools, finding the highest diversity of algae occurred in pools with intermediate levels of grazing. Intensity of wave action has also been shown to yield a parabolic pattern of species richness, which is lowest at very low disturbance sheltered shores and at exposed shores with very high levels of wave force (Blamey & Branch, Reference Blamey and Branch2009).
Succession following disturbance is ultimately driven by a combination of physical and biological processes. For example, the intensity of biological interactions may vary in relation to the physical attributes of the disturbed patches. Such interactions were identified by Sousa (Reference Sousa1984a) in an elegant experiment in which the removal of gastropod grazers resulted in different patterns of succession depending on the size of the experimental clearings in mussel beds. Small clearings (25 × 25 cm) were entirely dominated by grazing-resistant encrusting coralline algae. In contrast, large clearings (50 × 50 cm) became colonized by fleshy macroalgae in the central part, with encrusting corallines developing only along the borders. Complementary measurements indicated that herbivores were more abundant and foraged mostly along the margins of large patches, whereas they could explore all the available space in small clearings. These patterns were likely a response of grazers to predators and to desiccation stress, both of which were mitigated by the intact mussel assemblage surrounding the disturbed patches. Thus, the central part of large patches offered a refuge from grazing to palatable algae, whereas only grazing-resistant species could survive in areas under the reach of herbivores. Patchy mosaics resulting from local escapes from grazing (tertiary succession) can also lead to higher diversity intertidal landscapes (Hawkins & Hartnoll, Reference Hawkins and Hartnoll1983a).
Positive interactions
Positive interactions between species have been known for a long time, especially in the intertidal zone (Bertness & Leonard, Reference Bertness and Leonard1997; Bertness et al., Reference Bertness, Leonard, Levine, Schmidt and Ingraham1999). These can involve habitat provision or amelioration of conditions for other species during succession following disturbance or steady-state conditions. Some of the first experimental work identifying positive interactions was undertaken by Hatton (Reference Hatton1938) in France (for reviews, see Connell, Reference Connell1972; Hawkins et al., Reference Hawkins, Mieszkowska, Firth, Bohn, Burrows, Maclean, Thompson, Chan, Little and Williams2016). Hatton noted that higher on the shore positive interactions were important, whilst lower down negative interactions such as competition were more prevalent.
More recently, the term ‘facilitation’ borrowed from successional studies has been brought into ecological mainstream studies with work on both rocky shores (Silliman et al., Reference Silliman, Bertness, Altieri, Griffin, Bazterrica, Hidalgo, Crain and Reyna2011) and saltmarshes (Bertness & Shumway, Reference Bertness and Shumway1993; Bertness & Callaway, Reference Bertness and Callaway1994; Shumway & Bertness, Reference Shumway and Bertness1994). This was formalized by Bertness & Callaway (Reference Bertness and Callaway1994) in the stress-gradient hypothesis (Figure 3).
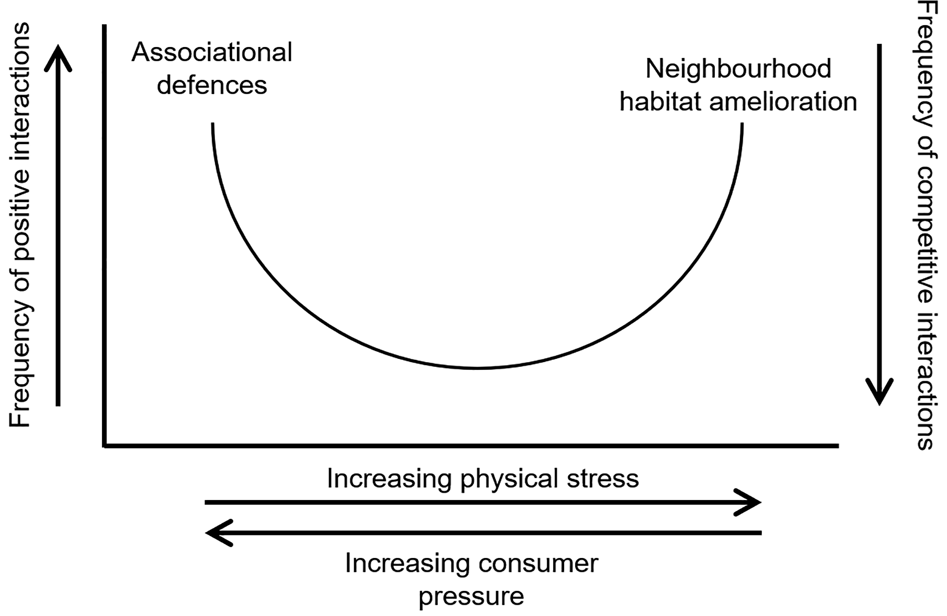
Fig. 3. Model of occurrence of positive interactions in natural communities. Positive interactions are predicted to be rare under mild physical conditions and consumer pressure. Associational defences under high consumer pressure, and neighbourhood habitat amelioration under high physical stress lead to a higher frequency of positive interactions. Redrawn from Bertness & Callaway (Reference Bertness and Callaway1994).
In physically stressed environments, both intra- and interspecific interactions can increase survival and influence demographic processes (Menge & Sutherland, Reference Menge and Sutherland1987). In areas with intense biological interactions, co-defences can be important in providing protection. Examples from rocky shores include work in the physically stressed low-humidity environments of Patagonia (Silliman et al., Reference Silliman, Bertness, Altieri, Griffin, Bazterrica, Hidalgo, Crain and Reyna2011) that emphasized the importance of positive interactions.
Mid-shore canopy-forming algae such as A. nodosum enable species to thrive higher up the shore, especially turf-forming algae (Jenkins et al., Reference Jenkins, Hawkins and Norton1999b, Reference Jenkins, Norton and Hawkins2004, 2005; Ingólfsson & Hawkins, Reference Ingólfsson and Hawkins2008; Pocklington et al., Reference Pocklington, Jenkins, Bellgrove, Keough, O'Hara, Masterson-Algar and Hawkins2018). These tend to die when the canopy is removed. The canopy also facilitates persistence of algal turfs that trap sand and inhibit limpet grazing (Airoldi & Hawkins, Reference Airoldi and Hawkins2007). Once the canopy is removed, the turf declines and limpet numbers increase (Jenkins et al., Reference Jenkins, Norton and Hawkins2004).
Some species can have both positive and negative effects on other species, depending on environmental context and life history stage (Bulleri et al., Reference Bulleri, Eriksson, Queirós, Airoldi, Arenas, Arvanitidis, Bouma, Crowe, Davoult, Guizien, Iveša, Jenkins, Michalet, Olabarria, Procaccini, Serrão, Wahl and Benedetti-Cecchi2018a). Canopy algae high on sheltered shores can enhance barnacle recruitment, presumably by reducing mortality of recently settled juveniles (Hawkins, Reference Hawkins1983). In contrast on more exposed shores, clumps of fucoids can reduce numbers of settling cyprids leading to reduced recruitment (Hawkins, Reference Hawkins1983). Lower on sheltered shores, F. serratus prevents settlement by sweeping (Hawkins, Reference Hawkins1983; Jenkins et al., Reference Jenkins, Norton and Hawkins1999a).
Whilst limpets can prevent establishment of fucoids in the north-east Atlantic, once a patch becomes established, juvenile Patella vulgata move under these patches along with adults (Hawkins & Hartnoll, Reference Hawkins and Hartnoll1983b; Hartnoll & Hawkins, Reference Hartnoll and Hawkins1985). Not only do the limpets benefit from shelter (amelioration of tide-out temperatures and relative humidity), but recent observations and work using isotopes (Davies et al., Reference Davies, Johnson and Maggs2007; Notman et al., Reference Notman, McGill, Hawkins and Burrows2016) have confirmed that the limpets also eat the fucoids, confirming earlier work (Jones, Reference Jones1948). Thus, a complex mix of positive and negative interactions plus chance recruitment events help generate and maintain patchiness on moderately exposed shores in the north-east Atlantic (Hawkins et al., Reference Hawkins, Hartnoll, Kain, Norton, John, Hawkins and Price1992). Similar processes have been shown in Australia (Underwood et al., Reference Underwood, Denley and Moran1983) and on the Pacific Coast of North America (Dungan, Reference Dungan1986).
In recent years, the concept of facilitation cascades has emerged (Stachowicz, Reference Stachowicz2001; Silliman & Bertness, Reference Silliman and Bertness2002), where a species provides habitat for another species that in turn provides habitat for a further species and so on (Stachowicz, Reference Stachowicz2001). Such cascades are typical of large macroalgae on rocky shores, but have also been described for sessile invertebrates (Peterson & Heck, Reference Peterson and Heck2001; Harley, Reference Harley2006; Zhang & Silliman, Reference Zhang and Silliman2019).
Alternative stable states
Although the earliest work on alternative stable states focused on lakes and ponds as model systems (Scheffer et al., Reference Scheffer, Hosper, Meijer, Moss and Jeppesen1993; Carpenter et al., Reference Carpenter, Cole, Pace, Batt, Brock, Cline, Coloso, Hodgson, Kitchell and Seekell2011; Hawkins et al., Reference Hawkins, Bohn and Doncaster2015), some work has been done in subtidal and intertidal rocky systems. In the shallow subtidal, comparisons between two physically comparable adjacent islands on the west coast of South Africa by Barkai & McQuaid (Reference Barkai and McQuaid1988) led them to argue that their communities existed in alternative stable states, one maintained by the predatory effects of rock lobsters that eliminate many prey species and promote kelp growth because of the absence of grazers, and the other by an unusual predator–prey reversal in which whelks consume and prevent colonization by lobsters. Sea urchin barrens prompted by overfishing (Steneck et al., Reference Steneck, Graham, Bourque, Corbett, Erlandson, Estes and Tegner2003) or driven by climate change (Ling et al., Reference Ling, Johnson, Frusher and Ridgway2009) have also been considered an alternative stable state. In Cystoseira-dominated shallow-water systems in the Mediterranean, storm-driven disturbances, the frequency of which are likely to have increased due to climate change, have been demonstrated to result in alternative stable states (Benedetti-Cecchi et al., Reference Benedetti-Cecchi, Tamburello, Maggi and Bulleri2015). Experiments and models have shown how this system followed non-linear dynamics and exhibited hysteresis (Figure 4), displaying a tipping point at about 70–75% of canopy loss, beyond which the canopy-dominated communities gave way to those dominated by algal turfs (Benedetti-Cecchi et al., Reference Benedetti-Cecchi, Tamburello, Maggi and Bulleri2015; Rindi et al., Reference Rindi, Dal Bello, Dai, Gore and Benedetti-Cecchi2017). This experimental work was used to probe statistical early warning signals of an approaching tipping point, such as rising variance and autocorrelation of response variables. Early warning signals of approaching regime shifts have been studied mostly under controlled laboratory conditions or using whole-ecosystem (yet pseudo-replicated) experiments (Carpenter et al., Reference Carpenter, Cole, Pace, Batt, Brock, Cline, Coloso, Hodgson, Kitchell and Seekell2011). Rocky shores have proved a tractable system to extend these tests under real world conditions, using properly replicated experiments.
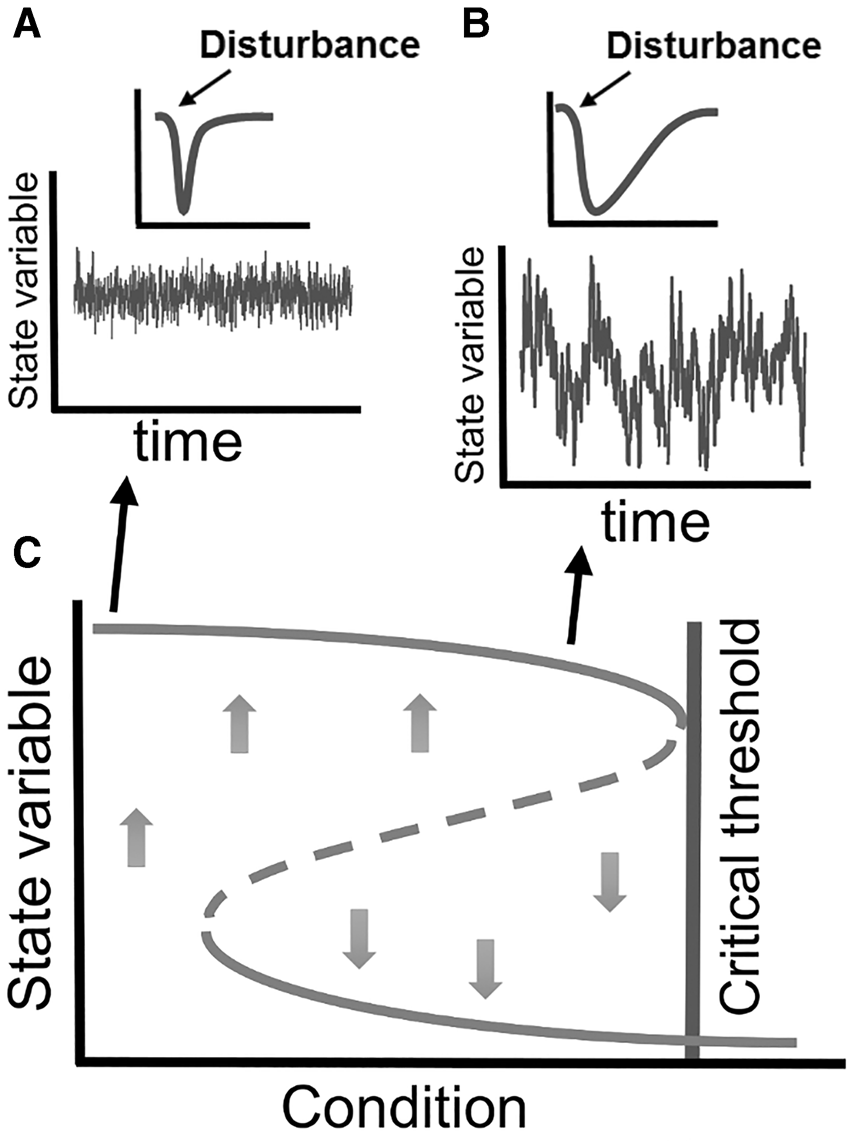
Fig. 4. How alternative stable states or extreme hysteresis can lead to slow or no recovery. Whilst a pulse disturbance can initiate succession or push a system to another state, press disturbance, repeated frequent pulse disturbance, or chronic pollution such as eutrophication or sedimentation can keep a system in another state unless they cease (Benedetti-Cecchi et al., Reference Benedetti-Cecchi, Airoldi, Bulleri, Fraschetti, Terlizzi, Hawkins, Bohn, Firth and Williams2019).
There is growing evidence to suggest that urbanization of coasts, especially increased silt-load can also lead to domination by turfs, both in subtidal and intertidal habitats (Benedetti-Cecchi et al., Reference Benedetti-Cecchi, Pannacciulli, Bulleri, Moschella, Airoldi, Relini and Cinelli2001; Airoldi, Reference Airoldi2003; Gorgula & Connell, Reference Gorgula and Connell2004). Using long-term observational data and path analysis, Bulleri et al. (Reference Bulleri, Cucco, Dal Bello, Maggi, Ravaglioli and Benedetti-Cecchi2018b) showed how wave action and anthropogenic disturbance may mediate the biological interactions that regulate regime shifts on rocky reefs in the north-west Mediterranean, including canopy-turf interactions and grazing by sea urchins. Indeed, algal canopies, turfs and barrens produced by sea urchins can form complex mosaics on subtidal rocky reefs, suggesting that these assemblages may be organized around three alternative states. Although the interactions that control the shift from one habitat type to another are reasonably well understood, the conditions maintaining the balance among the three putative alternative states have not been fully explored. In particular, improving knowledge of the reinforcing feedback mechanisms that maintain each habitat state is an important area for further research to understand the dynamics of algal-dominated rocky reefs globally.
Communities dominated by A. nodosum canopies in the mid-intertidal of the north Atlantic can be very slow to recover, taking over 20 years in Europe following experimental removal (Burrows, Reference Burrows1947; Jenkins et al., Reference Jenkins, Hawkins and Norton1999b, Reference Jenkins, Norton and Hawkins2004; Cervin et al., Reference Cervin, Åberg and Jenkins2005; Ingólfsson & Hawkins, Reference Ingólfsson and Hawkins2008; Pocklington et al., Reference Pocklington, Jenkins, Bellgrove, Keough, O'Hara, Masterson-Algar and Hawkins2018), or highly destructive harvesting (Boaden & Dring, Reference Boaden and Dring1980). Experimental work in North America has asserted that ice-scouring leading to loss of A. nodosum can lead to an alternative stable state – mussels (Petraitis & Dudgeon, Reference Petraitis and Dudgeon1999; Dudgeon & Petraitis, Reference Dudgeon and Petraitis2005). This interpretation has been challenged by other workers on New England shores (Bertness et al., Reference Bertness, Trussell, Ewanchuk and Silliman2002). More recently, Menge et al. (Reference Menge, Bracken, Lubchenco and Leslie2017) revisited sites experimentally cleared in 1974 and showed that Ascophyllum had not as yet recovered 39 years later, the cleared area being dominated by Fucus spp. They concluded that conditions for an alternative state were in operation. On European shores the limited recovery following A. nodosum removal after 20 years has been interpreted as very slow succession, with domination during mid-succession by other fucoids such as F. serratus and F. vesiculosus for some years. There are intrinsic differences in the biota on shores in New England and the British Isles. Most of the canopy species are the same (A. nodosum, F. vesiculosus, but with F. serratus largely absent except in Nova Scotia) and S. balanoides is the dominant barnacle in colder regions. However, due to differential post-glacial colonization processes, grazing by patellid limpets is absent and Littorina littorea is probably an invasive species in New England (Jenkins et al., Reference Jenkins, Murua and Burrows2008b). On the shores studied by Petraitis & Dudgeon (Reference Petraitis and Dudgeon1999), mussels dominated the alternative state, but they were generally absent from A. nodosum-dominated shores in north-west Europe. Context may play a role in whether alternative stable states occur or not. Such apparently stable states might still be an outcome of extremely slow succession, especially given the generally low recruitment of A. nodosum in some systems (Åberg & Pavia, Reference Åberg and Pavia1997). Very strong inhibition may, therefore, occur by species present in intermediate successional stages. Given the estimated lifespan of individual A. nodosum of over 100 years, it is not surprising that succession is slow given that their dominance is maintained by vegetative proliferation of clump-forming genetic individuals (Åberg, Reference Åberg1996).
Biodiversity and ecosystem functioning
The realization that biodiversity could drive the functioning of ecosystems has been a major topic of interest in mainstream ecology for the last three decades (Loreau et al., Reference Loreau, Naeem and Inchausti2002), having been explored in terrestrial (Hector et al., Reference Hector, Schmid, Beierkuhnlein, Caldeira, Diemer, Dimitrakopoulos, Finn, Freitas, Giller and Good1999; Loreau et al., Reference Loreau, Naeem, Inchausti, Bengtsson, Grime, Hector, Hooper, Huston, Raffaelli and Schmid2001), freshwater (Giller et al., Reference Giller, Hillebrand, Berninger, Gessner, Hawkins, Inchausti, Inglis, Leslie, Malmqvist and Monaghan2004; Woodward, Reference Woodward2009) and marine ecosystems (Solan et al., Reference Solan, Aspden and Paterson2012). Most work in marine ecosystems has used synthetic assemblages of species from soft sediment communities in mesocosms (Emmerson et al., Reference Emmerson, Solan, Emes, Paterson and Raffaelli2001; Solan et al., Reference Solan, Batty, Bulling and Godbold2008), strongly linking diversity to ecosystem processes such as nutrient remineralization (Emmerson et al., Reference Emmerson, Solan, Emes, Paterson and Raffaelli2001). Some pioneering work in the field on rocky shores explored the influence of biodiversity on primary production, showing strong idiosyncratic effects of individual dominant species such as Patella (O'Connor & Crowe, Reference O'Connor and Crowe2005). Rockpools have been used as semi-enclosed mesocosms in the field enabling ecosystem processes such as primary productivity to be measured as a response variable (Noël et al., Reference Noël, Hawkins, Jenkins and Thompson2009), whilst manipulating diversity in the pool at the species or functional group level (Griffin et al., Reference Griffin, Noël, Crowe, Burrows, Hawkins, Thompson and Jenkins2010).
Rocky shore organisms have also been taken into the laboratory and experimentally assembled in mesocosms mimicking rock pools. Griffin et al. (Reference Griffin, De La Haye, Hawkins, Thompson and Jenkins2008) investigated the interaction of diversity and density in assemblages of predatory crabs. They showed the importance of considering density in such experimental work; predators were shown to occupy distinct fundamental niches but loss of predator diversity impacted ecosystem processes only at high predator densities. Using a similar mesocosm approach, the influence of spatial heterogeneity on the role of biodiversity was shown in assemblages of grazing gastropods (Griffin et al., Reference Griffin, Noël, Crowe, Burrows, Hawkins, Thompson and Jenkins2010). More complex mesocosms have been used to investigate the effect of predator diversity on subtidal food webs, showing that changes in the diversity of predatory fish can cascade to lower trophic levels by reducing grazer abundance, which in turn translates to different levels of increases in algal biomass (Bruno & O'Connor, Reference Bruno and O'Connor2005). Experiments by O'Connor et al. (Reference O'Connor, Emmerson, Crowe and Donohue2013) compared the influence of predator loss and independent manipulations of lower trophic level grazers on algal composition and biomass on exposed and sheltered shores. Cascading negative effects of predator loss were identified on grazers and indirect effects on primary producers, mediated by grazer identity.
The diversity of functional groups within a habitat has been suggested to be important in determining the invasibility of assemblages (Arenas et al., Reference Arenas, Sánchez, Hawkins and Jenkins2006). Using mosaics of tiles in rock pools with different diversity and assemblage compositions, Arenas et al. (Reference Arenas, Sánchez, Hawkins and Jenkins2006) showed that invasibility may be facilitated by the disappearance of whole functional groups, which is increasingly likely with human activity.
Whilst biodiversity has been demonstrated to have a role in shaping ecosystem processes, this has mainly been shown in closed systems – often artificially, such as in mesocosms. More attention has focused on biodiversity at the numbers and types of species level. In open highly fluid systems typical of most marine ecosystems, especially rocky shores, habitat patch diversity may be more important (Giller et al., Reference Giller, Hillebrand, Berninger, Gessner, Hawkins, Inchausti, Inglis, Leslie, Malmqvist and Monaghan2004; Hawkins, Reference Hawkins2004). This will influence the balance of primary producers, consumers such as filter feeders, predators and mosaics of hard substrata dominated by production processes and soft sediments as the primary site for remineralization and carbon sequestration. There will also be export and import of material between these habitat patches. Alsterberg et al. (Reference Alsterberg, Roger, Sundbäck, Juhanson, Hulth, Hallin and Gamfeldt2017) demonstrated this in sedimentary systems. Recent work on rocky shores has also emphasized the strong context-dependency of biodiversity-ecosystem functioning relationships (Mrowicki et al., Reference Mrowicki, Maggs and O'Connor2015), reminding us of the importance of environmental gradients (summarized in Raffaelli & Hawkins, Reference Raffaelli and Hawkins1996) in setting patterns and ultimately determining potential composition of biodiversity, outcomes of interactions and hence processes at the community and ecosystem level (Hawkins et al., Reference Hawkins, Pack, Firth, Mieszkowska, Evans, Martins, Åberg, Adams, Arenas, Boaventura, Bohn, Borges, Castro, Coleman, Crowe, Cruz, Davies, Epstein, Faria, Ferreira, Frost, Griffin, Hanley, Herbert, Hyder, Johnson, Lima, Masterson-Algar, Moore, Moschella, Notman, Pannacciulli, Ribeiro, Santos, Silva, Skov, Sugden, Vale, Wangkulangkul, Wort, Thompson, Hartnoll, Burrows, Jenkins, Hawkins, Bohn, Firth and Williams2019b, Reference Hawkins, Bohn, Lemasson, Williams, Schiel, Jenkins, Firth, Hawkins, Bohn, Firth and Williams2019c).
Bottom-up forcing and top-down control
The limnological and oceanographic research communities have long pondered the importance of bottom-up forcing by physico-chemical processes relative to top-down control by higher trophic levels. These concepts can be best explored in closed systems such as small lakes and ponds where bottom-up nutrients and top predators can both be manipulated at the scale of the whole ecosystem in very similar adjacent lakes, ponds or large mesocosms (Scheffer et al., Reference Scheffer, Hosper, Meijer, Moss and Jeppesen1993; Moss et al., Reference Moss, McGowan and Carvalho1994; Williams et al., Reference Williams, Moss and Eaton2002).
These concepts of bottom-up forcing have been explored in intertidal ecosystems, especially those strongly influenced by nutrient-rich upwelling on the Pacific coasts of North America (Menge, Reference Menge2000a; Menge & Menge, Reference Menge and Menge2013; Fenberg & Menge, Reference Fenberg, Menge, Hawkins, Bohn, Firth and Williams2019), New Zealand (Menge et al., Reference Menge, Daley, Lubchenco, Sanford, Dahlhoff, Halpin, Hudson and Burnaford1999), Chile (Wieters et al., Reference Wieters, Kaplan, Navarrete, Sotomayor, Largier, Nielsen and Veliz2003; Wieters, Reference Wieters2005; Reddin et al., Reference Reddin, Docmac, O'Connor, Bothwell and Harrod2015), and South Africa from the Atlantic to the Indian Ocean (Bustamante et al., Reference Bustamante, Branch and Eekhout1995a; Bustamante & Branch, Reference Bustamante and Branch1996; McQuaid & Lindsay, Reference McQuaid and Lindsay2000). Bottom-up forcing by greater nutrient supply can lead to greater productivity by both microbial biofilms and macro-algae on the shore, leading to faster growth and larger sizes of grazers and greater densities of filter feeders (Bustamante et al., Reference Bustamante, Branch and Eekhout1995a, Reference Bustamante, Branch, Eekhout, Robertson, Zoutendyk, Schleyer, Dye, Hanekom, Keats and Jurd1995b). Similarly, rich inshore phytoplankton can enhance growth of sessile filter feeders (Bertness et al., Reference Bertness, Gaines, Bermudez and Sanford1991; Xavier et al., Reference Xavier, Branch and Wieters2007), especially if it proliferates during upwelling events and is then transported onshore by downwelling, coined the intermittent upwelling hypothesis (Menge & Menge, Reference Menge and Menge2013). This in turn can support larger numbers of predatory animals. Larval supply can also be viewed as a form of bottom-up forcing as it intensifies both intraspecific (Jenkins et al., Reference Jenkins, Moore, Burrows, Garbary, Hawkins, Ingólfsson, Sebens, Snelgrove, Wethey and Woodin2008a) and interspecific interactions (e.g. competition in high recruitment areas such as the Firth of Clyde, Connell, Reference Connell1961a, Reference Connell1961b) as well as providing more food for predators.
Using both comparative studies and field experiments, research on South African west-coast islands that are occupied by dense colonies of seabirds has shown that guano boosts nutrient levels in the surrounding seawater (Bosman et al., Reference Bosman, Du Toit, Hockey and Branch1986). This elevates intertidal algal growth and infaunal abundance, thus respectively increasing food supplies for herbivores and waders such as turnstones. As a consequence of enhanced algal biomass, growth rates and sizes of limpets rise substantially. However, oystercatchers reach greater abundance on the islands because they are protected there from predators, and they exert a strong influence on limpet abundance, micro-habitat occupation and grazing; and smaller waders deplete the infauna of algal mats on a seasonal basis determined by their migration patterns (Hockey & Underhill, Reference Hockey and Underhill1984; Branch, Reference Branch1985). Bottom-up nutrient effects are thus countered to some extent by top-down predation.
Conversely the role of top-down control by predators (Paine, Reference Paine1966) and grazers (Jones, Reference Jones1946, Reference Jones1948) has been long known in intertidal systems with some of the first demonstrations of how lower trophic levels can be controlled. If bottom-up forcing is great then lower trophic levels can escape or swamp top-down control, especially if predation is constrained by water flow or wave action (Leonard et al., Reference Leonard, Levine, Schmidt and Bertness1998); but then in species like barnacles which hummock, intense intraspecific competition can lead to mass mortality (Barnes & Powell, Reference Barnes and Powell1950).
In many cases the relative importance of top-down control and bottom-up forcing is a balance, varying from place to place with mesoscale hydrography and coastal morphology (Menge, Reference Menge2000a). It can also change temporally with season in temperate zones, or with intensity of upwelling in boundary current dominated systems. In the plankton in temperate regions physico-chemical factors control primary production in the winter (low light, much turbulence even though nutrients are not limiting). With increased light in spring and a less turbulent water column due to stratification, phytoplankton can bloom, this being ended by a combination of lack of nutrients coupled with grazing by copepods that lag the phytoplankton bloom. Similar processes have been shown in cold-temperate intertidal rocky shores on the Isle of Man that are driven by microbial films (Thompson et al., Reference Thompson, Norton and Hawkins2004). In the winter light is limiting but in late winter/early spring as light increases there is an increase in biomass as shown by chlorophyll. Grazing activity is driven by temperature and is still low in February/March at the time of coldest sea temperatures enabling a window of opportunity for benthic diatoms to flourish. Later in the year this bloom is halted by increased grazing as well as light and desiccation stress, leading to cyanobacterial domination in the summer. Parallel experiments by Thompson et al. (Reference Thompson, Norton and Hawkins2004) showed the primacy of grazing and seasonal summer stresses over nutrient forcing, suggesting both bottom-up forcing, top-down control and what they termed lateral stresses were important in this simple system (see Figure 5). Thus, there is interplay between abiotic factors (some bottom-up, some modulating stresses) and biological control in this highly seasonal system.
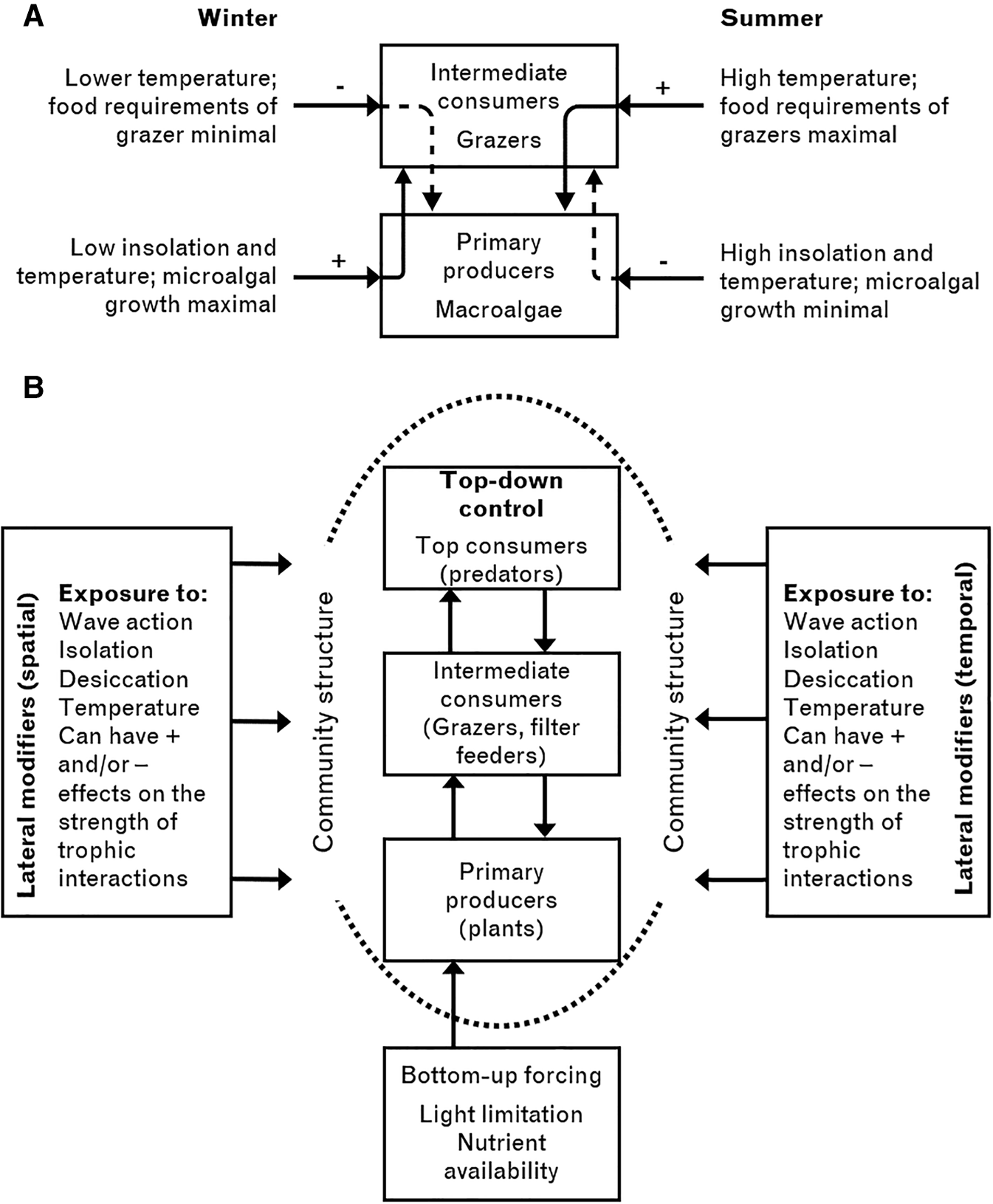
Fig. 5. Bottom-up forcing and top-down control of microbial films on rocky shores in the north-east Atlantic: (a) Regulation of the balance between producers (photosynthetic microbiota) and consumers (grazers) in the rocky intertidal during summer and winter. The positive (+) and negative (−) lateral effects of contrasting physical factors on bottom-up and top-down control of trophic interactions are illustrated. Strong effects are shown as solid lines, weak effects as dashed lines. (b) Conceptual model incorporating the role of physical stresses operating laterally at all trophic levels (sideways-facing arrows) together with bottom-up forcing (physical and chemical limiting factors; upward arrows) and top-down control (predation and grazing; downward arrows) in regulating community structure on temperate rocky shores (From Thompson et al., Reference Thompson, Norton and Hawkins2004).
Experimental work in rock pools (Masterson et al., Reference Masterson, Arenas, Thompson and Jenkins2008) has shown the interactive effects of top-down control by grazers and bottom-up nutrient enrichment on early successional algal assemblages following disturbance, with composition, cover and community productivity being used as response variables. Negative effects of grazing tended to override positive effects of nutrient-forcing, except at peak algal growth when top-down control was swamped. Interestingly, nutrient levels had an important influence on the identity of dominant macroalgae, leading to a mismatch between effect estimates based on cover and those based on productivity. While loss of grazers under ambient nutrient conditions led to high cover estimates (mainly of red algae such as Ceramium) but little enhancement of productivity, under elevated nutrients ephemeral green algae were favoured leading to a three-fold increase in productivity.
Overview and synthesis
Concepts and experiments
The above examples show how important experiments have been in understanding how patterns on rocky shores are shaped by processes (see various chapters in Hawkins et al., Reference Hawkins, Bohn, Firth and Williams2019a), but also, perhaps more importantly, how rocky shore experiments have contributed to the ecological mainstream across a range of concepts. The papers by Menge & Sutherland (Reference Menge and Sutherland1976, Reference Menge and Sutherland1987) perhaps epitomize the link between theory and empirical experimentation, taken further forward by Menge (Reference Menge2000a). Menge & Sutherland (Reference Menge and Sutherland1976) synthesized how environmental gradients of tidal elevation and exposure to wave action influenced the outcomes of interactions, especially the respective roles of competition, predation and what they at the time called temporal heterogeneity (in essence what now would be called disturbance). This synthesis was re-visited in Menge & Sutherland (Reference Menge and Sutherland1987), who clarified the role of disturbance (perhaps prompted by the work of Sousa, Reference Sousa1984b) and considered the importance of recruitment regimes as the importance of supply-side processes re-surfaced in the 1980s (Underwood & Fairweather, Reference Underwood and Fairweather1989). Menge (Reference Menge2000a) then considered how top-down control and bottom-up forcing could be incorporated to enhance understanding. He emphasized how environmental context on various spatial and temporal scales can influence how shore communities are structured – particularly by mesoscale nearshore oceanographic features such as upwelling influencing both productivity (Bustamante et al., Reference Bustamante, Branch, Eekhout, Robertson, Zoutendyk, Schleyer, Dye, Hanekom, Keats and Jurd1995b; Wieters et al., Reference Wieters, Kaplan, Navarrete, Sotomayor, Largier, Nielsen and Veliz2003; Xavier et al., Reference Xavier, Branch and Wieters2007) and larval delivery (Pineda, Reference Pineda1991).
As Menge (Reference Menge2000a) pointed out: theory helps ecologists rise above a morass of local and idiosyncratic natural history studies enabling a predictive framework and some generalization. At the very least theory provides the concepts and vocabulary to formulate hypotheses and interpret experimental results. However, papers emphasizing the contextual nature of many ecological processes (Crowe et al., Reference Crowe, Frost and Hawkins2011; O'Connor & Donohue, Reference O'Connor and Donohue2013) are, perhaps, a warning about not overgeneralizing. Good natural history is essential in experimental ecology (Hawkins et al., Reference Hawkins, Mieszkowska, Firth, Bohn, Burrows, Maclean, Thompson, Chan, Little and Williams2016): it is often the first phase in exploring patterns by observation and getting to know traits of species. It helps stratify surveys and experimental designs and minimize variance influencing factors being tested experimentally and can help get rid of co-variance. Good natural history leads to more elegant experimentation; just as good physiology depends on expertise in morphology and anatomy.
Approach and methodology
The most important development in the last 30 years has been better experimental designs matched with appropriate statistical analyses in a formal hypothesis-testing framework. The seminal work of Hurlbert (Reference Hurlbert1984) on pseudo-replication made ecological studies more demanding and forced the ecological community to think more carefully about the nature and the design of ecological studies. Considerable progress has been made in this direction and for this Underwood can take much of the credit (Underwood, Reference Underwood1978, Reference Underwood1981; Underwood & Chapman, Reference Underwood and Chapman1985, Reference Underwood and Chapman1992). Underwood and Chapman have educated young experimental ecologists worldwide, calling for more rigour and logic in the way experiments are designed, conducted, analysed and interpreted. Underwood's work on the scientific method has made Popperian falsification widely accessible, defining an operative framework whereby observations, theories (models), hypotheses (predictions) and experiments are logically connected (Underwood, Reference Underwood1981, Reference Underwood1997; Underwood & Denley, Reference Underwood, Denley, Strong, Simberloff, Abele and Thistle1984). The framework offers a decision-making tool to arbitrate among alternative explanations and to eradicate false models, contributing to pursuing values of objectivity when interpreting the outcome of ecological experiments and observational studies. By emphasizing the cyclical nature of observations, models, hypotheses and experiments, the approach advocated by Underwood has disclosed the complexity of rocky shores in an unprecedented way, documenting variation in the structure of assemblages over multiple scales in space and time and challenging the assumed generality of many ecological processes. This work has spilled beyond the boundaries of rocky shore ecology and has influenced the work of peers in other fields as well. It has also stimulated better sampling designs to assess ecological impacts through beyond-BACI (Before-After/Control-Impact) sampling designs (Underwood, Reference Underwood1991b, Reference Underwood1992b, Reference Underwood1994). The Underwoodian approach has demonstrated the value of looking beyond the most obvious explanations, showing the power of disproving alternative models to break paradigms, eradicate myths and disclose the unexpected.
Focus on rigorous experimental design has allowed interactions between multiple factors to be disentangled plus encouraged hierarchical designs that test for generality over spatial scales (e.g. Coleman et al., Reference Coleman, Underwood, Benedetti-Cecchi, Åberg, Arenas, Arrontes, Castro, Hartnoll, Jenkins, Paula, Santina and Hawkins2006). Ease of testing multivariate data, for example, packages such as PRIMER with the PERMANOVA extension (Anderson et al., Reference Anderson, Gorley and Clarke2008), and free R software (R Core Team, 2019) have led to much more complex and effective statistical analyses. Nevertheless, increasing availability of libraries and functions to perform complex analyses may also lead to mistakes and abuse of statistics. Only the judicious choice and correct use of well understood methodologies will guarantee progress and ensure that the ecological community can take full advantage of the increasing availability of open statistical software.
New technological approaches in the last two decades such as digital photography, remote sensing, better and cheaper GPS, and online identification resources, have greatly increased the scope of what can be done between tides. Digital photography has revolutionized non-destructive sampling enabling much greater sample sizes, and faster processing of acquired images back in the more clement environment of the laboratory. Cheap and robust underwater cameras can provide insights into tide-in conditions, especially presence and activities of mobile predators (Silva et al., Reference Silva, Hawkins, Boaventura and Thompson2008; Harasti et al., Reference Harasti, Gallen, Malcolm, Tegart and Hughes2014). In situ sensors and data loggers can characterize the environment, especially temperatures, experienced at fine temporal and spatial scales over long periods and help better define environmental gradients and micro-scale mosaics of conditions (Lima & Wethey, Reference Lima and Wethey2009; Seabra et al., Reference Seabra, Wethey, Santos and Lima2011). Thus, the context of experimental manipulations can be much better described. Hard-to-access areas have become more accessible through the use of remote sensing technologies such as satellites and aerial photography using drones (Guichard et al., Reference Guichard, Bourget and Agnard2000; Brodie et al., Reference Brodie, Ash, Tittley and Yesson2018; Gomes et al., Reference Gomes, Peteiro, Bueno-Pardo, Albuquerque, Pérez-Jorge, Oliveira, Alves and Queiroga2018; Rosenthal et al., Reference Rosenthal, Byrnes, Cavanaugh, Bell, Harder, Haupt, Rassweiler, Pérez-Matus, Assis and Swanson2018). The ability to collect large numbers of images over a wide area has enabled assessments of species distribution and temporal and spatial fluctuations, such as examining distribution patterns of mussels in Portugal (Gomes et al., Reference Gomes, Peteiro, Bueno-Pardo, Albuquerque, Pérez-Jorge, Oliveira, Alves and Queiroga2018) and investigating changes in kelp forests over time on a global scale (Rosenthal et al., Reference Rosenthal, Byrnes, Cavanaugh, Bell, Harder, Haupt, Rassweiler, Pérez-Matus, Assis and Swanson2018). Incorporating remote sensing techniques into experimental design has applications for investigating large-scale impacts of disturbance on rocky shores, such as commercial macroalgal harvesting (Bennion et al., Reference Bennion, Fisher, Yesson and Brodie2019), oil spills (see Gilfillan et al., Reference Gilfillan, Maher, Krejsa, Lanphear, Ball, Meltzer and Page1995; Mishra et al., Reference Mishra, Cho, Ghosh, Fox, Downs, Merani, Kirui, Jackson and Mishra2012 for examples on coastal marshes) and earthquakes (Schiel et al., Reference Schiel, Alestra, Gerrity, Orchard, Dunmore, Pirker, Lilley, Tait, Hickford and Thomsen2019b).
Ease of access to rocky shores has made them amenable to citizen science, where volunteers carry out scientific tasks (Delaney et al., Reference Delaney, Sperling, Adams and Leung2008; Dickinson et al., Reference Dickinson, Shirk, Bonter, Bonney, Crain, Martin, Phillips and Purcell2012; Vye et al., Reference Vye, Dickens, Adams, Bohn, Chenery, Dobson, Dunn, Earp, Evans, Foster, Grist, Holt, Hull, Jenkins, Lamont, Long, Mieszkowska, Millard, Morrall, Pack, Parry-Wilson, Pocklington, Pottas, Richardson, Scott, Sugden, Watson, West, Winton, Delany and Burrows2020) such as sample collection, processing and analysis. This is not a new concept, with examples of bird surveys that have been done by citizen scientists for over 100 years (Silvertown, Reference Silvertown2009). This approach is becoming more common in marine and coastal environments (Hughes et al., Reference Hughes, Hughes and Smith2014; Cigliano & Ballard, Reference Cigliano and Ballard2017) and is increasingly being used to support policy and management (Hyder et al., Reference Hyder, Townhill, Anderson, Delany and Pinnegar2015; Townhill & Hyder, Reference Townhill, Hyder, Cigliano and Ballard2017). For rocky shores, most examples relate to descriptive observations or monitoring of biodiversity (Delaney et al., Reference Delaney, Sperling, Adams and Leung2008; Vye et al., Reference Vye, Dickens, Adams, Bohn, Chenery, Dobson, Dunn, Earp, Evans, Foster, Grist, Holt, Hull, Jenkins, Lamont, Long, Mieszkowska, Millard, Morrall, Pack, Parry-Wilson, Pocklington, Pottas, Richardson, Scott, Sugden, Watson, West, Winton, Delany and Burrows2020), but more recently experimental approaches (e.g. www.capturingourcoast.co.uk) and internet-based citizen science (e.g. www.zooniverse.org; Newman et al., Reference Newman, Wiggins, Crall, Graham, Newman and Crowston2012) have become more common. With care this can also include simple experimental procedures such as re-colonization or clearance experiments at multiple locations with appropriate controls. Obviously, there are training, ethical, conservation and quality-control issues to be overcome, but the scope is limitless for both describing patterns and understanding processes on broad spatial scales.
New spectroscopic approaches have also recently revolutionized intertidal ecology. Biomass of photosynthetic biofilms can be measured non-destructively and over much greater spatial extents (Murphy et al., Reference Murphy, Underwood, Pinkerton and Range2005, Reference Murphy, Underwood and Pinkerton2006), than with traditional destructive chlorophyll extraction techniques (Underwood, Reference Underwood1984; Hill & Hawkins, Reference Hill and Hawkins1990; Nagarkar & Williams, Reference Nagarkar and Williams1997; Thompson et al., Reference Thompson, Tobin, Hawkins and Norton1999). They have enabled photosynthesis of macro-algae to be measured including stress responses (Ferreira et al., Reference Ferreira, Arenas, Martínez, Hawkins and Jenkins2014).
Technological innovation for the acquisition, storage and dissemination of digital information makes large environmental and biological datasets increasingly available. This boost from large datasets opens new opportunities for ecological analysis at unprecedented spatial and temporal scales. Recent developments have encouraged a synthesis between observations and experiments using hybrid datasets resulting from the combination of observations and experiments (Benedetti-Cecchi et al., Reference Benedetti-Cecchi, Bulleri, Dal Bello, Maggi, Ravaglioli and Rindi2018). This approach leverages the scope of large-scale observational data with the ability to attribute causality typical of experimental studies. Large datasets are mostly observational, but distributed experiments across large spatial scales are becoming increasingly common (see Sanford & Bertness, Reference Sanford, Bertness, Witman and Kaustuv2009, for review). Building on recent developments in time-series analysis and spatial modelling, hybrid datasets can take advantage of the data generated by distributed experiments embedded in large-scale observation networks – combining observational and experimental data provides a promising approach to uncover causal relations in large-scale phenomena, beyond the limits of causal inference inherent in observations and beyond the scales encompassed by individual manipulative experiments.
The development of ever-more sophisticated and well-controlled mesocosms mimicking rock pools have allowed more controlled factorial experiments to understand how complex interactions between biodiversity loss and elements of global change (including rising temperatures, stormier seas, the introduction of non-native species and eutrophication) modify community composition and ecosystem functioning. Whilst strictly not field experimentation, such approaches have stemmed from work on rocky shores in general and rock pools in particular whether natural or drilled on the shore (Arrontes & Underwood, Reference Arrontes and Underwood1991; Atalah & Crowe, Reference Atalah and Crowe2010). Such mesocosms have been particularly powerful in disentangling the effects of multiple stressors or environmental context crossed with biodiversity loss (e.g. O'Connor et al., Reference O'Connor, Bracken, Crowe and Donohue2015 – biodiversity loss and nutrient enrichment; Mrowicki & O'Connor, Reference Mrowicki and O'Connor2015 – wave action on warming and biodiversity loss; White et al., Reference White, Donohue, Emmerson and O'Connor2018 – biodiversity of predators with warming and nutrient enrichment; Vye et al., Reference Vye, Emmerson, Arenas, Dick and O'Connor2015 – impacts of invasive species with water temperature and nutrient enrichment).
One of the criticisms of experimentation is that it is often done on very local scales. Broader-scale insights can come from rapid response to large cataclysmic events whether from major acute pollution incidents such as oil-spills (Southward & Southward, Reference Southward and Southward1978; Hawkins et al., Reference Hawkins, Evans, Mieszkowska, Adams, Bray, Burrows, Firth, Genner, Leung, Moore, Pack, Schuster, Sims, Whittington and Southward2017a, Reference Hawkins, Evans, Moore, Whittington, Pack, Firth, Adams, Moore, Masterson-Algar, Mieszkowska and Southward2017b), nuclear weapon testing lifting whole atolls (Lebednik, Reference Lebednik1973) or major tectonic events, particularly earthquakes. Earthquakes in Chile (Castilla & Oliva, Reference Castilla and Oliva1990), Mexico (Bodin & Klinger, Reference Bodin and Klinger1986) and New Zealand (Schiel et al., Reference Schiel, Alestra, Gerrity, Orchard, Dunmore, Pirker, Lilley, Tait, Hickford and Thomsen2019b) have provided insights into diverse topics such as factors setting zonation patterns due to uplift or submergence of coastal rock platforms, as well as connectivity during recolonization. Whether from acute pollution incidents such as oil-spills, outbreaks of pandemic disease such as COVID 19 reducing tourism and recreational use of the foreshore, or natural disasters such as earthquakes or tsunamis, insights come best when they impact well-studied sites with previous time-series data, ideally where experimentation has revealed some of the processes causing patterns. The network of sites studied by the Southwards for a decade before the Torrey Canyon oil spill (Southward & Southward, Reference Southward and Southward1978) provided an unintended beyond-BACI design of multiple impacted and un-impacted areas beyond the influence of the spill – ultimately allowing some disentangling of the impacts of the spill and the role of climate fluctuations in recovery processes (Hawkins et al., Reference Hawkins, Evans, Mieszkowska, Adams, Bray, Burrows, Firth, Genner, Leung, Moore, Pack, Schuster, Sims, Whittington and Southward2017a, Reference Hawkins, Evans, Moore, Whittington, Pack, Firth, Adams, Moore, Masterson-Algar, Mieszkowska and Southward2017b). Likewise in New Zealand the work at Kaikoura over many years by Schiel and colleagues will enable some major scientific insights to be recovered from the wreckage caused by the earthquake (Schiel et al., Reference Schiel, Alestra, Gerrity, Orchard, Dunmore, Pirker, Lilley, Tait, Hickford and Thomsen2019b), including the closure of the laboratory at Kaikoura.
Conservation and management of rocky shores in a rapidly changing world
Understanding from experiments has also contributed to better conservation and management of rocky shore ecosystems (Thompson et al., Reference Thompson, Crowe and Hawkins2002; Branch et al., Reference Branch, Thompson, Crowe, Castilla, Langmead, Hawkins and Polunin2008) including the use of rocky shore organisms as indicators of global change and understanding interactions with more regional and local scale impacts (explored in detail in Branch et al., Reference Branch, Thompson, Crowe, Castilla, Langmead, Hawkins and Polunin2008; Hawkins et al., Reference Hawkins, Bohn, Lemasson, Williams, Schiel, Jenkins, Firth, Hawkins, Bohn, Firth and Williams2019c). Here we focus mainly on global change, but emphasize that many of its more pernicious impacts are usually in concert with regional or local-scale impacts such as overfishing of nearshore predators (Ling et al., Reference Ling, Johnson, Frusher and Ridgway2009) or over-collection of intertidal grazers (Borges et al., Reference Borges, Hawkins, Doncaster and Crowe2015), sediment and nutrient run-off from land both from agricultural (Schiel & Howard-Williams, Reference Schiel and Howard-Williams2016) and urban sources (Airoldi, Reference Airoldi2003), plus habitat loss and fragmentation (Airoldi & Beck, Reference Airoldi and Beck2007), often as a result of urbanization or ocean sprawl (Bugnot et al., Reference Bugnot, Mayer-Pinto, Airoldi, Heery, Johnston, Critchley, Strain, Morris, Loke, Bishop, Sheehan, Coleman and Dafforn2020; Hawkins et al., Reference Hawkins, Firth and Evans2020).
Ecosystem responses to climate change fluctuations (Southward & Crisp, Reference Southward and Crisp1954; Southward, Reference Southward1967) and subsequent rapid climate change have been detected using rocky shore indicators (Southward et al., Reference Southward, Hawkins and Burrows1995; Hawkins et al., Reference Hawkins, Southward and Genner2003; Mieszkowska et al., Reference Mieszkowska, Kendall, Hawkins, Leaper, Williamson, Hardman-Mountford and Southward2006, Reference Mieszkowska, Burrows, Pannacciulli and Hawkins2014). Knowledge of species interactions derived from experiments have shown the importance of modulation of climate warming by biological interactions. Based on the classical work of Connell (Reference Connell1961a), coupled with a space-limited model (Roughgarden et al., Reference Roughgarden, Iwasa and Baxter1985), modelling has shown how responses of cold-water and warm-water species of barnacles to climate were mediated by competition (Poloczanska et al., Reference Poloczanska, Hawkins, Southward and Burrows2008). In warmer years, the warm-water Chthamalus spp. prospered as they were released from competition with faster growing northern species. Predictive models using climate change scenarios only worked well when competition in addition to physical drivers was built into the models. Experiments have been used to explore how competitive interactions can be shaped by additional species expanding polewards in response to climate change (Firth et al., Reference Firth, Crowe, Moore, Thompson and Hawkins2009). How facilitation can modulate stresses resultant from climate change for mobile animals such as limpets has been shown by Moore et al. (Reference Moore, Hawkins and Thompson2007), finding lower survival of the northern species, P. vulgata, when clumps of Fucus were removed, unlike the more southern P. depressa that does not aggregate under algal clumps. Changes in the species composition of these two species have implications for the dynamic patchiness on rocky shores (Hartnoll & Hawkins, Reference Hartnoll and Hawkins1985; Hawkins et al., Reference Hawkins, Moore, Burrows, Poloczanska, Mieszkowska, Herbert, Jenkins, Thompson, Genner and Southward2008). Thinning of fucoid canopies resulting from climate change (Hawkins et al., Reference Hawkins, Sugden, Mieszkowska, Moore, Poloczanska, Leaper, Herbert, Genner, Moschella, Thompson, Jenkins, Southward and Burrows2009; Yesson et al., Reference Yesson, Bush, Davies, Maggs and Brodie2015) has been experimentally simulated and can lead to reductions in sub-canopy species (Pocklington et al., Reference Pocklington, Jenkins, Bellgrove, Keough, O'Hara, Masterson-Algar and Hawkins2018). Positive interactions have been advocated as a tool to combat loss of biodiversity in the face of climate change (Bulleri et al., Reference Bulleri, Eriksson, Queirós, Airoldi, Arenas, Arvanitidis, Bouma, Crowe, Davoult, Guizien, Iveša, Jenkins, Michalet, Olabarria, Procaccini, Serrão, Wahl and Benedetti-Cecchi2018a). Experimentally derived knowledge has also enabled the impacts of oil spills and their subsequent clean up to be better understood (Southward & Southward, Reference Southward and Southward1978; Hawkins & Southward, Reference Hawkins, Southward and Thayer1992; Hawkins et al., Reference Hawkins, Evans, Mieszkowska, Adams, Bray, Burrows, Firth, Genner, Leung, Moore, Pack, Schuster, Sims, Whittington and Southward2017a, Reference Hawkins, Evans, Moore, Whittington, Pack, Firth, Adams, Moore, Masterson-Algar, Mieszkowska and Southward2017b).
Extreme events such as hurricanes, marine heatwaves and floods are predicted to become more frequent with climate change (Easterling et al., Reference Easterling, Meehl, Parmesan, Changnon, Karl and Mearns2000; Oliver et al., Reference Oliver, Donat, Burrows, Moore, Smale, Alexander, Benthuysen, Feng, Gupta and Hobday2018). Climate models also predict that extreme events are becoming more clustered in time, which implies a change in the distribution of time intervals between consecutive disturbance events compared with historical climate scenarios (Easterling et al., Reference Easterling, Meehl, Parmesan, Changnon, Karl and Mearns2000). Experimental work has been performed to assess the ecological effects of changing the intensity and temporal distribution of perturbations on rocky shore assemblages. Some studies have done so by manipulating the frequency of events. This approach allows a direct test of the ecological effects due to the expected increased frequency of extreme events. However, manipulating the frequency of perturbations has the drawback of confounding intensity with temporal variability (clustered vs regularly distributed) of perturbations (Benedetti-Cecchi, Reference Benedetti-Cecchi2003). Appropriate experimental designs have been proposed to disentangle the effects of changes in mean intensity from those due to variation in the temporal patterning of events, also allowing tests of interactions between intensity and degree of temporal clustering of perturbations (Benedetti-Cecchi, Reference Benedetti-Cecchi2000b, Reference Benedetti-Cecchi2003).
These designs have been used to assess the impacts of waves (Bertocci et al., Reference Bertocci, Maggi, Vaselli and Benedetti-Cecchi2005, Reference Bertocci, Maggi, Vaselli and Benedetti-Cecchi2010; Maggi et al., Reference Maggi, Bulleri, Bertocci and Benedetti-Cecchi2012), desiccation stress (Benedetti-Cecchi et al., Reference Benedetti-Cecchi, Bertocci, Vaselli and Maggi2006), sediment regimes (Vaselli et al., Reference Vaselli, Bertocci, Maggi and Benedetti-Cecchi2008) and heatwaves (Dal Bello et al., Reference Dal Bello, Rindi and Benedetti-Cecchi2017) on rocky intertidal assemblages. Overall, the results have shown that the effects of increasing intensity of perturbations can be offset in part by increasing degree of temporal clustering. In other words, regardless of the nature of the perturbation, results have shown that the temporally clustered events are in general less detrimental to rocky shore assemblages than events that are more regularly distributed in time. Interestingly, an observational study on the impact of hurricanes on coral reefs in the Caribbean has shown a similar pattern: impacts were less severe in years when hurricanes occurred clustered in time compared with years when they were regularly distributed (Mumby et al., Reference Mumby, Vitolo and Stephenson2011).
The mechanisms behind this apparent general positive effect of temporal clustering of perturbations have not been elucidated yet, but one possibility is that a clustered perturbation scenario allows for longer periods of recovery compared with where the same number of perturbations is distributed regularly over the same time window. A longer period of recovery may be particularly advantageous if individual perturbations are intense enough so that one event is sufficient to wipe out most of the organisms from an area. In this scenario the cumulative effect of a cluster of perturbations will not be much more different from that of an individual one. Regardless of the specific mechanism, a positive response to increased clustering of extreme climate events injects some optimism in the ability of rocky shore assemblages to cope with the expected increase in intensity (and temporal clustering) of climate-related perturbations.
The zonation of intertidal organisms has been shown by observations and experiments to be squeezed from environmental extremes such as desiccation stress at the top of the shore coupled with greater consumer pressure from lower down due to biogeographic shifts (e.g. colder water fucoids in the Azores stressed by more frequent warmer weather and the spread of herbivorous fish northwards; Martins et al., Reference Martins, Harley, Faria, Vale, Hawkins, Neto and Arenas2019). Late winter and early spring seasonal blooms of light-driven micro-algae and macro-algae are likely to get curtailed by earlier onset of temperature-driven grazing pressure (Thompson et al., Reference Thompson, Norton and Hawkins2004) as has been shown on latitudinal gradients in Europe (Jenkins et al., Reference Jenkins, Åberg, Cervin, Coleman, Delany, Della Santina, Hawkins, Lacroix, Myers, Lindegarth, Power, Roberts and Hartnoll2000).
Experimental approaches have informed both ecological enhancement of highly modified urban coastlines as well as adaptational responses to climate change by design of environmentally sensitive sea defences in the face of rising and stormier seas (Morris et al., Reference Morris, Heery, Loke, Lau, Strain, Airoldi, Alexander, Bishop, Coleman and Cordell2019). Ironically, early studies of artificial structures used them primarily as simple surrogates of natural systems with low topographic complexity and hence ease of study – in many cases cubist rocky shores. Examples include describing distribution patterns in relation to tidal height and wave exposure (Southward & Orton, Reference Southward and Orton1954), plus succession on large breakwater blocks of known age (Hawkins et al., Reference Hawkins, Southward and Barrett1983) both using Plymouth Breakwater. The importance of grazing interactions in limiting the lower limit of kelp in the shallow subtidal zone was shown on the ruined breakwater in Port Erin, Isle Of Man (Jones & Kain, Reference Jones and Kain1967). Interactions between barnacles and limpets (Hawkins & Hartnoll, Reference Hawkins and Hartnoll1982b; Santini et al., Reference Santini, Thompson, Jenkins, Chelazzi, Hartnoll, Kay and Hawkins2019) and settlement patterns of barnacles in relation to wind patterns (Hawkins & Hartnoll, Reference Hawkins and Hartnoll1982a) were all investigated on the same Raglan Pier in Port Erin. The simplicity of replicated artificial shore parallel breakwaters at Elmer in Sussex, UK, enabled the respective roles of grazing by limpets in preventing establishment of fucoid algae and wave action reducing persistence of older plants to be disentangled by a combination of field experiments and modelling (Jonsson et al., Reference Jonsson, Granhag, Moschella, Åberg, Hawkins and Thompson2006).
The full battery of experimental approaches has been deployed to better understand the efficacy of various interventions to enhance biodiversity and deliver ecosystem services on artificial hard substrate habitats in ports and on sea defences – an approach now termed eco-engineering. Much of this work has been led from Sydney, Australia, with the development of artificial rockpools (Browne & Chapman, Reference Browne and Chapman2011) and boulder-fields (Chapman, Reference Chapman2017), including better understanding of the diversity deficit typical of artificial communities compared with natural ones (Bulleri & Chapman, Reference Bulleri and Chapman2010). Similarly in Europe the outcomes of experimental ecology were used in early efforts to inform guidelines on the design of sea defences (Moschella et al., Reference Moschella, Abbiati, Åberg, Airoldi, Anderson, Bacchiocchi, Bulleri, Dinesen, Frost, Gacia, Granhag, Jonsson, Satta, Sundelöf, Thompson and Hawkins2005; Burcharth et al., Reference Burcharth, Hawkins, Zanuttigh and Lamberti2007) and understanding their impacts (Martin et al., Reference Martin, Bertasi, Colangelo, De Vries, Frost, Hawkins, Macpherson, Moschella, Satta and Thompson2005), enabling putting this work into a broader ecological and conservation context (Airoldi et al., Reference Airoldi, Abbiati, Beck, Hawkins, Jonsson, Martin, Moschella, Sundelöf, Thompson and Åberg2005). This early work has proliferated to understand the impacts of what became known as ocean sprawl (Duarte et al., Reference Duarte, Pitt, Lucas, Purcell, Uye, Robinson, Brotz, Decker, Sutherland, Malej, Madin, Mainzan, Gili, Fuentes, Atienza, Pages, Breitburg, Malek, Graham and Condon2012; Firth et al., Reference Firth, Browne, Knights, Hawkins and Nash2016; Bugnot et al., Reference Bugnot, Mayer-Pinto, Airoldi, Heery, Johnston, Critchley, Strain, Morris, Loke, Bishop, Sheehan, Coleman and Dafforn2020; Hawkins et al., Reference Hawkins, Firth and Evans2020) and how these impacts can be at least partially offset by eco-engineering interventions (Dafforn et al., Reference Dafforn, Glasby, Airoldi, Rivero, Mayer-Pinto and Johnston2015; Morris et al., Reference Morris, Heery, Loke, Lau, Strain, Airoldi, Alexander, Bishop, Coleman and Cordell2019). Worldwide experimental trials have tested the efficacy of different types of crevices (Coombes et al., Reference Coombes, La Marca, Naylor and Thompson2015), complexity of tiles (Loke et al., Reference Loke, Jachowski, Bouma, Ladle and Todd2014, Reference Loke, Ladle, Bouma and Todd2015), boulder size and mix in gabions (Firth et al., Reference Ferreira, Arenas, Martínez, Hawkins and Jenkins2014) and retro-fitted artificial rockpools (Browne & Chapman, Reference Browne and Chapman2014; Evans et al., Reference Evans, Firth, Hawkins, Morris, Goudge and Moore2016). Guidelines on practice are emerging (Morris et al., Reference Morris, Heery, Loke, Lau, Strain, Airoldi, Alexander, Bishop, Coleman and Cordell2019; O'Shaughnessy et al., Reference O'Shaughnessy, Hawkins, Evans, Hanley, Lunt, Thompson, Francis, Hoggart, Moore and Iglesias2020) and this approach is now entering the planning (Evans et al., Reference Evans, Firth, Hawkins, Morris, Goudge and Moore2016; Evans et al., Reference Evans, Firth, Hawkins, Hall, Ironside, Thompson and Moore2019) and engineering mainstream (Burcharth et al., Reference Burcharth, Hawkins, Zanuttigh and Lamberti2007). Additionally along the way some interesting insights have emerged on rock pool ecology (Firth et al., Reference Firth, Thompson, White, Schofield, Skov, Hoggart, Jackson, Knights and Hawkins2013), topographic complexity and diversity (Loke et al., Reference Loke, Liao, Bouma and Todd2016; Loke & Todd, Reference Loke and Todd2016), mobile predators using the intertidal zone (Morris et al., Reference Morris, Chapman, Firth and Coleman2017), connectivity of matter and propagules (Bishop et al., Reference Bishop, Mayer-Pinto, Airoldi, Firth, Morris, Loke, Hawkins, Naylor, Coleman and Chee2017; Heery et al., Reference Heery, Bishop, Critchley, Bugnot, Airoldi, Mayer-Pinto, Sheehan, Coleman, Loke and Johnston2017) plus difficult-to-study boulder shores (Chapman, Reference Chapman2017). Furthermore, understanding of the nature of biogeographic boundaries has resulted with breakwaters acting as ‘experimental’ stepping-stones, enabling range expansions (Hawkins et al., Reference Hawkins, Sugden, Mieszkowska, Moore, Poloczanska, Leaper, Herbert, Genner, Moschella, Thompson, Jenkins, Southward and Burrows2009; Keith et al., Reference Keith, Herbert, Norton, Hawkins and Newton2011; Firth et al., Reference Firth, Mieszkowska, Grant, Bush, Davies, Frost, Moschella, Burrows, Cunningham, Dye and Hawkins2015) – an experiment that no ethical committee would ever allow (Sugden et al., Reference Sugden, Underwood, Hawkins and Wahl2009), nor would a funding body finance.
Concluding comments
Experiments on rocky shores have contributed to both ecological theory and better understanding of patterns and processes in coastal ecosystems. It is now informing responses to global change and creeping urbanization. The coastal zone is being increasingly squeezed and impacted by both development and climate-driven change. Experiments help explain patterns and can feed into forecast and prediction of future states. They can also inform better understanding and hence management of the interactions of multiple regional and local-scale impacts with global change.
Acknowledgements
SJH, LBC, SRJ, KH wish to thank the European-funded project EUROROCK which enabled us to all work together in such a fruitful and fun way, and was an important step in all our careers. EUROROCK was shaped by advice from Tony Underwood in its conception, experimental designs and reinforced by training received by the team from him and Gee Chapman. Eurorock's co-ordinator Guido Chelazzi herded cats well. SJH would also like to thank all his PhD students and Post-doc Fellows hosted, especially Richard Thompson, Nova Mieszkowska, Louise Firth, Mike Burrows, Mark Johnson, Ross Coleman, Tasman Crowe, Paula Moschella, Ally Evans who have helped him do much work and have been great fun on the shore and pub over the years. SJH would like to thank the Marine Biological Association of the UK for hosting his NERC-funded post-doc in 1979–1980 and providing lab space on subsequent visits from Manchester, Port Erin, Southampton, Bangor, allowing him to be Director from 1999 to 2007 and according him the privilege of a Lankester Research Fellowship as an Emeritus from Southampton – plus an office. SJH has a large personal and professional debt to Richard Hartnoll who helped get him a NERC studentship on experimental ecology and spotted the value of such an approach and helped finish many things off and get them published. George Russell always took an interest and introduced him to limpet-algal interactions on a field course in 1975. Alan Southward was a great inspiration and mentor for nearly 40 years – we dedicate this review to him – and we are sure he would have had some trenchant criticisms (‘too much theory and jargon’). SJH, SRJ and KH all benefitted from the unique atmosphere at Port Erin Marine Laboratory/Marine Biological Station (RIP). Over the years SJH has received a personal appeal NERC studentship, personal Post-doc Fellowship, and many grants and studentships from NERC, plus NERC grant-in-aid to the MBA (1999–2007). SRJ similarly received a NERC studentship and has been subsequently funded by them including grants, studentships and held a NERC grant-in-aid funded Fellowship at the MBA. We all thank Jane Lewis for this invitation to write this review and her patience. The review has benefitted from expert input from two referees: David Schiel and George Branch who contributed much to improving it.