INTRODUCTION
The Mediterranean fan worm, Sabella spallanzanii (Gmelin, Reference Gmelin and Gmelin1791), native to the Mediterranean Sea and southern European Atlantic coast to the English Channel, is a highly invasive pest species introduced to Australia and New Zealand (Patti & Gambi, Reference Patti and Gambi2001; Read et al., Reference Read, Inglis, Stratford and Ahyong2011). It is a tube dwelling filter-feeder that, outside of its native environment, can densely cover the sediment substrate and artificial surfaces, altering marine ecosystems and outcompeting native species for food and space (Currie et al., Reference Currie, McArthur and Cohen2000; Holloway & Keough, Reference Holloway and Keough2002; O'Brien et al., Reference O'Brien, Ross and Keough2006). Sabella spallanzanii can form clumps of 200–300 individuals in an area smaller than 1 m2, and up to 13 individuals m−2 on soft sediments over large areas (Parry et al., Reference Parry, Lockett, Crookes, Coleman and Sinclair1996). These densities decrease after the initial stages of colonization and have been measured to be about 1–5 individuals m−2 in years after (e.g. Parry et al., Reference Parry, Lockett, Crookes, Coleman and Sinclair1996; Cohen et al., Reference Cohen, Currie and McArthur2000; Ross et al., Reference Ross, Keough, Longmore and Knott2007), but biomass (both number of individuals and size) is related to the food available, being greater in eutrophic environments (Giangrande et al., Reference Giangrande, Pierri, Fanelli, Schirosi, Licciano and Stabili2014). With tubes up to 0.5 m in length, S. spallanzanii also has the potential to influence aquaculture operations, both as a nuisance fouler and as a competitor with cultured filter-feeding species such as oysters and mussels. In both Australia and New Zealand, S. spallanzanii is regarded as a high impact, notifiable invasive species (Hayes et al., Reference Hayes, Silwa, Migus, McEnnulty and Dunstan2005; Read et al., Reference Read, Inglis, Stratford and Ahyong2011; http://www.dpi.nsw.gov.au/fishing/pests-diseases/marine-pests/found-in-nsw/european-fan-worm; http://www.biosecurity.govt.nz/pests/mediterranean-fanworm).
In Australia, Sabella spallanzanii was first collected from Albany, Western Australia, in 1965 (Clapin & Evans, Reference Clapin and Evans1995), and has since been recorded from Victoria, South Australia, Tasmania and Twofold Bay in far-southern New South Wales (Knight-Jones & Perkins, Reference Knight-Jones and Perkins1998; Huisman et al., Reference Huisman, Jones, Wells and Burton2008) (Figure 1). In New Zealand, S. spallanzanii was first detected in March 2008 in Lyttelton Harbour, South Island. By January 2010 it had spread to multiple locations in the North and South islands, New Zealand (Read et al., Reference Read, Inglis, Stratford and Ahyong2011). In April 2013, Australian Museum scientists discovered S. spallanzanii in Botany Bay, near Sydney, New South Wales, some 500 km north of previous records from Twofold Bay (Murray & Keable, Reference Murray and Keable2013).
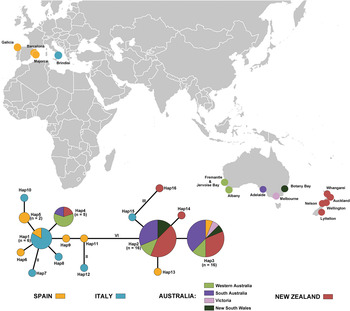
Fig. 1. Collecting localities of Sabella spallanzanii (Gmelin, Reference Gmelin and Gmelin1791) used in this study and haplotype network based on COI sequences of Sabella spallanzanii (Gmelin, Reference Gmelin and Gmelin1791). Areas of circles proportional to numbers of specimens. Lines between haplotypes represent one mutational step unless otherwise indicated.
The barcoding region of the cytochrome c oxidase gene, subunit 1 (COI), has been widely used to investigate marine phylogeography, including that of invasive species (e.g. crabs, Roman & Palumbi, Reference Roman and Palumbi2004; shrimp, Lejeusne et al., Reference Lejeusne, Saunier, Petit, Béguer, Otani, Carlton, Rico and Green2014; ascidians, Dias et al., Reference Dias, Rocha, Godwin, Tovar-Hernández, Delahoz, McKirdy, de Lestang, McDonald and Snow2016; and polychaetes, Capa et al., Reference Capa, Bybee and Bybee2010). In the present study, we use COI to investigate the phylogeography of the Australian and New Zealand populations of S. spallanzanii, including the possible origins of the recent New Zealand and eastern Australian incursions.
MATERIALS AND METHODS
Sabella spallanzanii was obtained from within its native range in the Mediterranean Sea (Brindisi, Italy, and three localities in Spain) and throughout its invasive range in New Zealand (Auckland; Wellington; Nelson; Lyttelton) and Australia (Western Australia: Fremantle and Albany; South Australia: North Haven, Adelaide; Victoria: Melbourne; New South Wales: Botany Bay and Twofold Bay). Specimens from New Zealand were collected by various marine biosecurity surveys and vouchered in the collections of the Marine Invasives Taxonomic Service, National Institute of Water & Atmospheric Research, Wellington (NIWA). Note that the specimen from Wellington was sampled from an Auckland vessel soon after its arrival into Wellington Harbour. Specimens from the Mediterranean Sea and Australia were freshly collected for the study; voucher specimens are deposited in the collections of the Australian Museum (AM), Museum Victoria (NMV), and the Norwegian University of Science and Technology Museum (NTNU) (Table 1). Being a closely related congener, Sabella pavonina Savigny, Reference Savigny1822, collected from Norway (NTNU VM 68754, 14 Mar 2014, POLNB1407-14.COI-5P), was used as outgroup.
Table 1. Sources of specimens, institutions and accession numbers of Sabella spallanzanii (Gmelin, Reference Gmelin and Gmelin1791).

NMV, Museum Victoria, Melbourne, Australia; AM, Australian Museum, Sydney, Australia; NIWA, National Institute of Water & Atmospheric Research, Wellington, New Zealand; NTNU, Norwegian University of Science and Technology Museum; NSW, New South Wales; SA, South Australia; VIC, Victoria; WA, Western Australia.
* The Wellington specimen was collected from biofouling on an Auckland vessel, immediately following its arrival into Wellington Harbour.
Genomic DNA was extracted from branchial - crown or muscle tissue using a DNeasy Blood and Tissue Kit (Qiagen, Düsseldorf, Germany) according to the manufacturer's protocol. A 658 bp fragment of COI was amplified by PCR under standard conditions using the Lobo et al. (Reference Lobo, Costa, Teixeira, Ferreira, Costa and Costa2013) COI primers. PCR conditions were as follows: an initial denaturation step at 94°C for 3 min, 40 cycles at 94°C for 30 s, 52°C for 30 s, 72°C for 60 s, with a final extension at 72°C for 4 min. PCR products were sequenced by Macrogen™, Korea. Sequence chromatograms were visualized in BioEdit (Hall, Reference Hall1999). Sequences were aligned manually.
Maximum likelihood analysis was conducted in IQ-Tree (Nguyen et al., Reference Nguyen, Schmidt, von Haeseler and Minh2015). The TMP2 + F + I model of nucleotide evolution was determined in IQ-Tree under the Bayesian Information Criterion. Topologies were viewed in FigTree 1.4.2 (Rambaut, Reference Rambaut2014). The haplotype network was constructed in TCS ver. 1.21 (Clement et al., Reference Clement, Posada and Crandall2000) following the statistical parsimony criterion (Templeton et al., Reference Templeton, Crandall and Sing1992) to infer the number of unique haplotypes, and thus, genetic variability within native and invasive populations.
RESULTS AND DISCUSSION
Fifty-six specimens of Sabella spallanzanii were successfully sequenced from throughout the known range of the species. Sequences are deposited in GenBank (KY472732–472787), representing the first taxonomically validated COI sequences of S. spallanzanii to be publicly available (Table 1). The single previous GenBank COI sequence listed as S. spallanzanii (AY436349) is misidentified and represents a fireworm, Hermodice carunculata (Pallas, Reference Pallas1766) (Amphinomidae). Unfortunately, DNA could not be amplified from the first Australian specimens of S. spallanzanii, collected from Albany, Western Australia in 1965, evidently having been formalin fixed. The TMP2 + F + I model of nucleotide evolution was identified as the most appropriate for maximum likelihood analysis; nucleotide frequencies were A = 0.23, C = 0.14, G = 0.22, T = 0.41.
Maximum likelihood analysis placed all of the Australian and New Zealand specimens in a virtually unresolved clade together with selected Mediterranean specimens (Figure 2). Average pairwise intraspecific divergence among all specimens was 0.8%, between Mediterranean and Australian–New Zealand populations 1.4%, between Australia and New Zealand 0.2%, within Australia 0.1% and within New Zealand 0.2%. As expected, the Australian and New Zealand populations show markedly lower average internal divergence than overall divergence including European populations. The minimal haplotype diversity and consequent absence of phylogeographic structure of the Australian and New Zealand S. spallanzanii is consistent with the ‘founder effect’ of a single relatively recent successful introduction from the Mediterranean Sea. It should be noted, however, that low haplotype diversity can also result from multiple introductions of a single or few successful invasive haplotypes, as observed in some ascidians (Turón et al., Reference Turón, Tarjuelo, Durán and Pascual2003; Dias et al., Reference Dias, Rocha, Godwin, Tovar-Hernández, Delahoz, McKirdy, de Lestang, McDonald and Snow2016). We also note that two rare haplotypes were present only among New Zealand samples suggesting additional sampling from Australian and Mediterranean populations is required. Nevertheless, our interpretation of present mitochondrial results aligns well with the observed invasion history and corroborates results based on allozymes (Andrew & Ward, Reference Andrew and Ward1997) and nuclear sequences (ITS) (Patti & Gambi, Reference Patti and Gambi2001), which also indicated a small, relatively homogeneous founding population. Among the sequenced specimens, all Australian and New Zealand specimens correspond to five of 16 haplotypes, of which the majority of specimens from both regions correspond to two main haplotypes (Figure 1). Our phylogeographic data, however, are not sufficiently variable to resolve population-level distinctions between and within New Zealand and Australia. As a result, we cannot infer the sequence of S. spallanzanii invasions within Australia or New Zealand from the data alone. Long-term biosecurity monitoring in both countries, however, means the timing of incursions of S. spallanzanii is well-documented, indicating that Australia was the most likely source of the New Zealand population.

Fig. 2. Maximum likelihood phylogram of Mediterranean, Australian and New Zealand Sabella spallanzanii (Gmelin, Reference Gmelin and Gmelin1791) based on COI sequences (ln −1377.3525). Specimens colour coded by collecting locality. Analysis rooted to Sabella pavonina Savigny, Reference Savigny1822.
Sabella spallanzanii was first collected in Australia in 1965 from Albany, south of Perth, Western Australia; the identification was confirmed herein by examination of voucher specimens deposited in the Western Australian Museum (WAM V3692–3694). Since then, S. spallanzanii spread eastwards to South Australia, Victoria, Tasmania and by 1996 had reached Twofold Bay in southern New South Wales (NSW DPI, 2016). Our results show that S. spallanzanii throughout the Australian range is dominated by two main haplotypes, as are those from New Zealand. Given the similarity between Australian and New Zealand populations, and that the New Zealand incursion can be reliably dated to near 2007 (with a single localized population that rapidly spread northwards to other localities), it is reasonable to infer that Australia was the source of New Zealand S. spallanzanii. Likewise, although our data do not reveal population structure within New Zealand, the local spread of S. spallanzanii is well-documented as beginning at Lyttelton, South Island, and spreading northwards as biofouling on domestic barges and possibly also in ballast water (Read et al., Reference Read, Inglis, Stratford and Ahyong2011).
As with the spread of S. spallanzanii to and within New Zealand, the source population of the Botany Bay incursion in eastern Australia cannot be pinpointed from our molecular data alone. Whereas S. spallanzanii in Twofold Bay, southern New South Wales, is the nearest source to Botany Bay, primary vessel traffic is from the commercial fishing fleet and local recreational boating, with no long distance commercial cargo shipping, making Twofold Bay an unlikely source. Also, the site in Botany Bay at which S. spallanzanii has been found is close to a commercial container port (Port Botany) and commercial oil terminal (Kurnell). The limited haplotype diversity is consistent with either a southern Australian or New Zealand origin. Botany Bay is a major hub for domestic and international maritime traffic, receiving some 1600 vessel visitations in 2011–2012 (Sydney Ports, Reference Sydney2012). Given the patterns of vessel traffic between Australia and New Zealand, and within Australia, however, two major pathways emerge based on modelled risk assessments (Glasby & Lobb, Reference Glasby and Lobb2008). Glasby & Lobb's (Reference Glasby and Lobb2008) modelling identified S. spallanzanii as the second most likely invasive to be introduced into Botany Bay from a domestic port, citing the most likely vector and source as commercial shipping from the Port of Melbourne. Alternatively, among the five international ports most likely to result in new marine invasions according to Glasby & Lobb (Reference Glasby and Lobb2008), the only candidate ports that harbour S. spallanzanii are in New Zealand – Auckland and Tauranga. Thus, the most likely source of the Botany Bay incursion is either domestic (i.e. the Port of Melbourne), or ironically, an overseas population originally derived from Australia (i.e. Auckland or Tauranga). Reasonably adjudicating between these two alternatives is not possible with the present state of knowledge, but either way, further surveys of the Botany Bay population of S. spallanzanii are required, as are more detailed genetic analyses. In addition to wider population sampling to maximize haplotype diversity, additional markers such as the nuclear Internal Transcribed Spacer 2 region (ITS2) and mitochondrial Cytochrome B (CytB), which have been successfully applied to phylogeographic analyses of other polychaetes (e.g. Capa et al., Reference Capa, Pons and Hutchings2013; Nygren, Reference Nygren2014; Sun et al., Reference Sun, Wong, Tovar-Hernández, Williamson and Kupriyanova2016; Styan et al., Reference Styan, McCluskey, Sun and Kupriyanovain press), could improve resolution within populations of S. spallanzanii.
Owing to logistical challenges and commercial sensitivities, neither Port Botany nor the Kurnell oil terminal has been surveyed for invasive species since the late 1990s/early 2000s (Hewitt et al., Reference Hewitt, Gibbs, Moore, Murfet and Ross1998; Australian Museum Business Services, 2002; Pollard & Pethebridge, Reference Pollard and Pethebridge2002), although recent underwater video transects in parts of Port Botany in 2013 by the New South Wales Department of Primary Industry failed to detect S. spallanzanii (T. Glasby, personal commmunication). It should be noted, however, that water clarity in Port Botany is usually very poor, and even in clear water, video transects are unlikely to detect small specimens or low density populations of S. spallanzanii amongst other dense biofouling. In situ sampling is required for reliable detection of S. spallanzanii. Regardless, S. spallanzanii is now established in Botany Bay, having been regularly observed by the authors at the original collecting sites off Inscription Point, Kurnell in 2014, 2015 and 2016 in addition to Bare Island, a site in Botany Bay close to Port Botany in 2016. It could probably extend its range into neighbouring estuaries in the Sydney Region (Port Hacking, Port Jackson and the Hawkesbury River) as well as estuaries further south, bridging the gap between Sydney and Twofold Bay. Significant northward expansion, however, appears less likely. Sea-surface temperature modelling indicates that water temperatures to the north of Sydney will usually remain above that of reproductive tolerance for S. spallanzanii (11–22°C) (Summerson et al., Reference Summerson, Darbyshire and Lawrence2007).
Further research is also required to identify the vectors underpinning the Botany Bay incursion. Sabella spallanzanii has one of the longest known pelagic larval phases for a polychaete, remaining in the water column for more than two weeks and affording it a high dispersal capability (Giangrande et al., Reference Giangrande, Licciano, Pagliara and Gambi2000). In addition, the strong ability to regenerate completely following significant damage (e.g. Licciano et al., Reference Licciano, Murray, Watson and Giangrande2012) enhances its invasive potential. The long larval phase of S. spallanzanii enables it to move long distances in ballast water of container ships anywhere around and between Australia and New Zealand. Even if international biosecurity management protocols are effective in halting or minimizing international translocations, however, domestic protocols are presently far less stringent. Within Australia and New Zealand, movement of ballast water and biofouling within national waters is effectively unencumbered. Sabella spallanzanii is known to have been spread within New Zealand by domestic biofouling, and it is likely to be the same in Australia (Murray & Keable, Reference Murray and Keable2013). Internal movement of invasive species would require more stringent biofouling and ballast water standards for the domestic fleet and recreational craft that at least complemented protocols governing international movements.
Our results indicate a southern Australian origin for the New Zealand incursion of S. spallanzanii, rather than as a new incursion from the Mediterranean Sea. The origin of the recent eastern Australian (Botany Bay) incursion, however, is less clear, plausibly deriving from southern Australia or as a ‘return’ from New Zealand. This highlights the ongoing importance of marine biosecurity surveillance and monitoring as well as improvements to biosecurity protocols for international and domestic vessels. This is important not only for detection but also for documentation of invasion history, which informs our understanding of invasion biology. Protocols are required that not only minimize the level of biofouling but also improve identification of high-risk vessels/craft as a focus for sampling. Evidently, current biosecurity protocols were not sufficient to prevent the spread of S. spallanzanii domestically or internationally in either Australia or New Zealand.
ACKNOWLEDGEMENTS
We thank Justin McDonald and Joana Dias (Western Australian Department of Fisheries), Aria Lee (University of New South Wales), Adriana Giangrande (University of Salento, Leece, Italy) and Maria Christina Gambi (Stazione Zoologica Anton Dohrn, Naples, Italy), Robin Wilson and Julian Finn (Museum Victoria), Eunice Wong (formerly Australian Museum), Tim Glasby (NSW DPI), Michael Bok (Lund University, Sweden) and family Oliva (Spain) for help with collecting material in Australia, New Zealand and Europe. Andrew Hosie and Ana Hara (Western Australian Museum) kindly provided specimens from Western Australian Museum collections. New Zealand specimens were collected under New Zealand Ministry for Primary Industries (MPI) contracts (RFP10623, SOW12099, ZBS2000-04, 2001-01, 2005-24 and 2009-24). Tim Riding (MPI) and two anonymous reviewers are gratefully acknowledged for constructive comments on the manuscript. We gratefully acknowledge POLYNOR, a subproject of NorBol (the Norwegian Barcoding Initiative) for use of the Sabella pavonina sequence. MC was supported by the ForBio Research School funding from the Research Council of Norway (project no. 248799) and the Norwegian Taxonomy Initiative (project no. 70184215). This study was funded by a grant from the Australian Museum Foundation to EK, STA and PH.