INTRODUCTION
Sea turtles face a variety of threats throughout their lives, of which the most significant are habitat loss, fisheries by-catch, climate change, marine pollution and infectious diseases (Hamann et al., Reference Hamann, Godfrey, Seminoff, Arthur, Barata, Bjorndal, Bolten, Broderick, Campbell, Carreras, Casale, Chaloupka, Chan, Coyne, Crowder, Diez, Dutton, Epperly, FitzSimmons, Formia, Girondot, Hays, Cheng, Kaska, Lewison, Mortimer, Nichols, Reina, Shanker, Spotila, Tomás, Wallace, Work, Zbinden and Godley2010). These conservation threats are largely related to habitat degradation and coastal development (Seminoff, Reference Seminoff2004; Santos et al., Reference Santos, Almeida, Santos, Gallo, Giffoni, Baptistotte, Coelho, Lima, Sales, Lopez, Stahelin, Becker, Castilhos, Thomé, Wanderlinde, Marcovaldi, Mendilaharsu, Damasceno, Barata, Sforza, Marcovaldi, Santos and Sales2011). While fisheries by-catch and marine pollution are well-documented factors leading to mortality and changes in the distribution of sea turtles, little is known about the effects of the environment or climate change and disease in the population viability of wild sea turtles (Hamann et al., Reference Hamann, Godfrey, Seminoff, Arthur, Barata, Bjorndal, Bolten, Broderick, Campbell, Carreras, Casale, Chaloupka, Chan, Coyne, Crowder, Diez, Dutton, Epperly, FitzSimmons, Formia, Girondot, Hays, Cheng, Kaska, Lewison, Mortimer, Nichols, Reina, Shanker, Spotila, Tomás, Wallace, Work, Zbinden and Godley2010).
Fibropapillomatosis (FP) is a debilitating neoplastic disease known since the 1930s (Smith & Coates, Reference Smith and Coates1938). It is one of the most important threats to the conservation of green turtles (Work & Balazs, Reference Work, Balazs, Epperly and Braun1997) and is a major cause of turtle strandings in Hawaii (Chaloupka et al., Reference Chaloupka, Work, Balazs, Murakawa and Morris2008) and Florida (Foley et al., Reference Foley, Schroeder, Redlow, Fick-Child and Teas2005). Initially, viral particles suggestive of herpesvirus were observed in FP tumours (Jacobson et al., Reference Jacobson, Buergelt, Williams and Harris1991), and the disease was shown to be transmissible through the inoculation of cell-free extracts of FP tumours (Herbst et al., Reference Herbst, Jacobson, Moretti, Brown, Sunberg and Klein1995, Reference Herbst, Jacobson, Klein, Balazs, Moretti, Brown and Sundenberg1999). Molecular studies later identified an alpha-herpesvirus, chelonid fibropapillomatosis-associated herpesvirus (CFPHV), and suggested this could be the primary aetiological agent of FP (Ene et al., Reference Ene, Su, Lemaire, Rose, Schaff, Moretti, Lenz and Herbst2005). Four regional variants of the virus were identified, and their phylogeographic distribution was found to partly reflect the population dynamics of the sea turtles (Patrício et al., Reference Patrício, Herbst, Duarte, Vélez-Zuazo, Loureiro, Pereira, Tavares and Toranzos2012). Despite the strong evidence for a viral aetiology, however, the onset of FP also seems related to human-altered environments (Foley et al., Reference Foley, Schroeder, Redlow, Fick-Child and Teas2005; Van Houtan et al., Reference Van Houtan, Hargrove and Balazs2010).
FP has a heterogeneous geographic distribution. Its prevalence varies widely across regions, time and age groups, ranging from 1.4 to 90% and tending to be higher in marine environments suffering the impact of human activities (Aguirre et al., Reference Aguirre, Balazs, Zimmerman and Spraker1994b; Herbst, Reference Herbst1994; Arthur et al., Reference Arthur, Limpus, Balazs, Capper, Udy, Shaw, Keuper-Bennett and Bennett2008). Along the Brazilian coast, the average prevalence is 15.4% (Baptistotte, Reference Baptistotte2007). Some researchers have suggested that the geographic variation in FP prevalence could be the consequence of the high fidelity of sea turtles to their feeding areas, as this would allow or favour chronic exposure to pollutants or other tumour-promoting agents (Taquet et al., Reference Taquet, Taquet, Dempster, Soria, Ciccione, Roos and Dagorn2006; Arthur et al., Reference Arthur, Limpus, Balazs, Capper, Udy, Shaw, Keuper-Bennett and Bennett2008). Several studies have supported the interpretation that environmental co-factors play a role in the pathogenesis of FP, including natural tumour-promoting compounds (Herbst, Reference Herbst1994; Herbst & Klein, Reference Herbst and Klein1995; Landsberg et al., Reference Landsberg, Balazs, Steidinger, Baden, Work and Russell1999; Arthur et al., Reference Arthur, Limpus, Balazs, Capper, Udy, Shaw, Keuper-Bennett and Bennett2008), UV rays and chemical compounds (Weisburger, Reference Weisburger and Sirica1989; Ananthaswamy & Pierceall, Reference Ananthaswamy and Pierceall1990). Recent investigations also identified a high prevalence of FP in coastal waters characterized by habitat degradation and pollution, shallow-water areas and low energy waves (Foley et al., Reference Foley, Schroeder, Redlow, Fick-Child and Teas2005). Epidemiological links have also been identified between FP and nitrogen-footprints, invasive macroalgae and overall environmental quality (dos Santos et al., Reference dos Santos, Martins, Torezani, Baptistotte, Farias, Horta, Work and Balazs2010; Van Houtan et al., Reference Van Houtan, Hargrove and Balazs2010).
Because herpesviruses can become latent following an acute infection (Hoff & Hoff, Reference Hoff, Hoff, Hoff, Frye and Jacobson1984), environmental factors that negatively affect the immune response could favour a relapse of the disease (Herbst, Reference Herbst1994; Herbst et al., Reference Herbst, Greiner, Ehrhart, Bagley and Klein1998). Several pollutants are known to produce immune suppression and/or to have carcinogenic effects, and it has been suggested that these substances might induce a latent CFPHV infection to become active, leading to the development of FP (Balazs, Reference Balazs1991; Herbst & Klein, Reference Herbst and Klein1995).
Among others, organochlorine compounds (OCs) are particularly relevant due to their toxicity and persistence in the environment, which resulted in these chemicals being classified as persistent organic pollutants (POPs) (Almeida et al., Reference Almeida, Centeno, Bisinoti and Jardim2007). Some of these compounds are pesticides whose usage in Brazil began in 1946 (MMA, 2006). In the following decades, large quantities of OCs were employed by farms, public health authorities and industry until restrictions were introduced in the 1980s (Directives MAPA No. 329/1985 and No. 153/1988, MIC/MI/MME No. 19/1981).
Due to their persistency, toxicity and ability to bioaccumulate, OCs can be found in high concentrations and have adverse health effects in long-lived species such as sea turtles (Aguirre & Lutz, Reference Aguirre and Lutz2004). It has been shown OCs may cause modulation of the immune system of these animals (Keller et al., Reference Keller, Kucklick, Stamper, Harms and McClellan-Green2004b, Reference Keller, McClellan-Green, Kucklick, Keil and Peden-Adams2006), which leads to the hypothesis that these compounds could increase the susceptibility to infectious pathogens; however, relatively few studies have attempted to determine whether POPs contribute to the development of FP (Aguirre et al., Reference Aguirre, Balazs, Zimmerman and Galey1994a; Miao et al., Reference Miao, Balazs, Murakawa and Li2001; Keller et al., Reference Keller, Balazs, Nilsen, Rice, Work and Jensen2014). In fact, the lack of knowledge about the role played by environmental factors in the development of FP is one of the specific stated concerns of field experts (Hamann et al., Reference Hamann, Godfrey, Seminoff, Arthur, Barata, Bjorndal, Bolten, Broderick, Campbell, Carreras, Casale, Chaloupka, Chan, Coyne, Crowder, Diez, Dutton, Epperly, FitzSimmons, Formia, Girondot, Hays, Cheng, Kaska, Lewison, Mortimer, Nichols, Reina, Shanker, Spotila, Tomás, Wallace, Work, Zbinden and Godley2010). In this study, we quantified the concentrations of several OCs in the tissues of green turtles caught at three feeding areas off south-eastern Brazil, aiming to correlate the concentrations of these pollutants with the occurrence of FP.
MATERIALS AND METHODS
Sampling
Through the research and conservational efforts of TAMAR-ICMBio and Biopesca Projects during 2011 and 2012, green turtle carcasses were sampled following standard necropsy procedures (Flint et al., Reference Flint, Patterson-Kane, Limpus, Work, Blair and Mills2009a, Reference Flint, Patterson-Kane, Mills and Limpusb). In total, 64 fresh or moderately decomposed specimens (carcass condition codes D2 and D3) (Flint et al., Reference Flint, Patterson-Kane, Limpus, Work, Blair and Mills2009a, Reference Flint, Patterson-Kane, Mills and Limpusb) were studied at three feeding areas in south-eastern Brazil: Praia Grande (N = 16) and Ubatuba (N = 26), State of São Paulo, and Vitória (N = 22), State of Espírito Santo (Figure 1). The average FP prevalence is 10.7% in São Paulo state, and 27.4% in Espírito Santo state (Baptistotte, Reference Baptistotte2007).
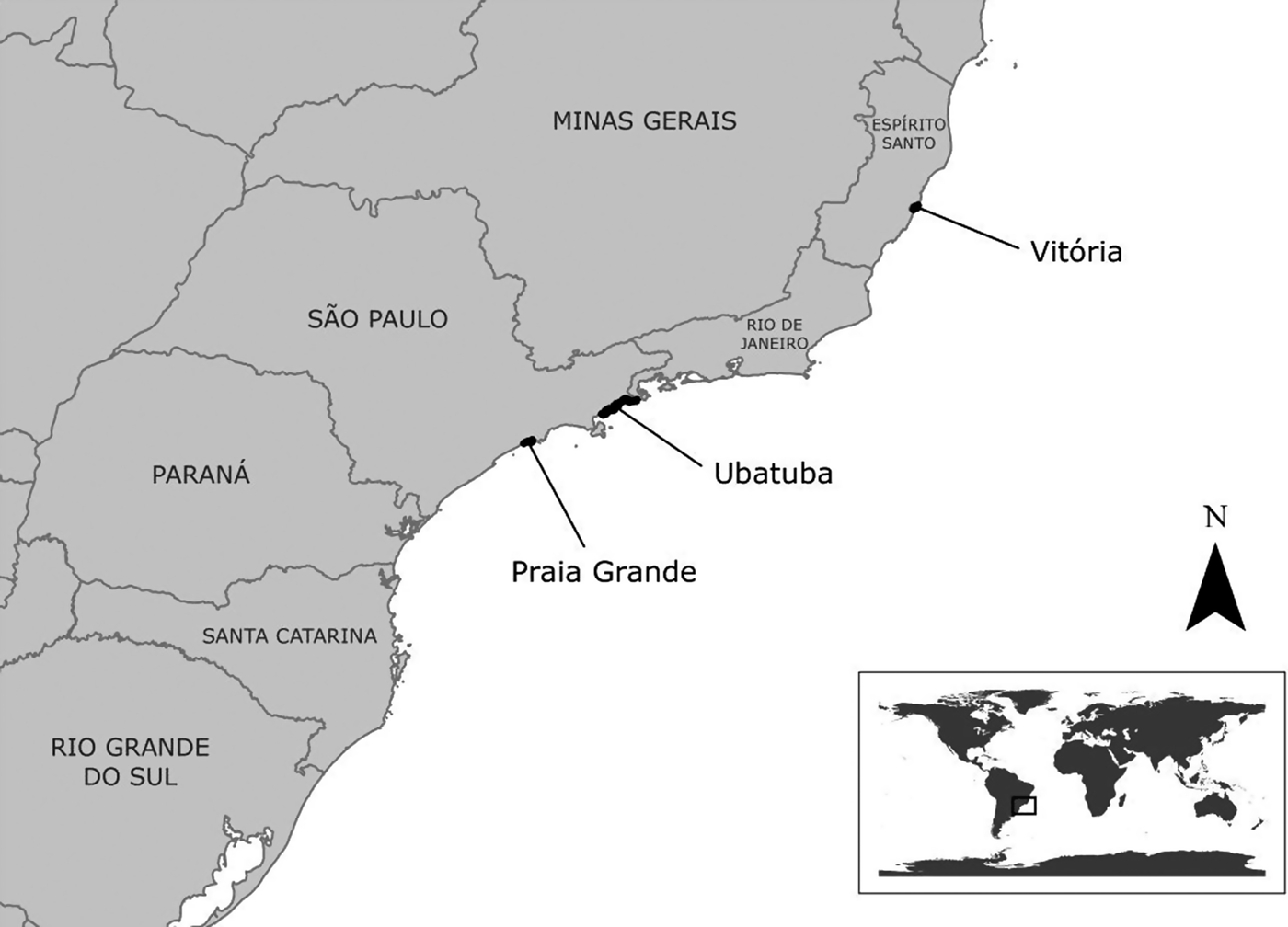
Fig. 1. Geographic distribution of studied areas at Brazilian south-eastern coast.
All individuals were juveniles with curved carapace lengths between 30 and 57.5 cm (mean ± SD = 38.68 ± 5.41 cm) and body masses between 2.5 and 15.5 kg (mean ± SD = 6.44 ± 2.94 kg). Turtles were classified according to the presence of fibropapilloma tumours on the external body surface: ‘With FP’ (N = 24) and ‘No FP’ (N = 40). No tumours were observed in the viscera. Fragments of 5–10 g fat (N = 51) and liver (N = 64) were collected and stored frozen at −20°C for laboratory analysis.
Laboratory analyses
Samples of adipose and liver tissue were homogenized with a scalpel and Ultraturrax® (IKA, Staufen, Germany). Extraction and clean-up were performed by adapting a previous protocol (Castillo et al., Reference Castillo, González and Miralles2011). Extraction was performed twice using 1 g of tissue with 8 mL acetonitrile saturated with 18% n-hexane. A pre-clean-up step was performed by putting the extract in an ultra-freezer (−80°C) for 20 min to promote lipid precipitation and phase separation. The first clean-up was done by dispersive solid-phase extraction (d-SPE) based on QuEChERS (Anastassiades et al., Reference Anastassiades, Lehotay, Stajnbaher and Schenck2003) by mixing 8 mL of acetonitrile extract with 1.2 g of magnesium sulphate (MgSO4) and 0.4 g of primary and secondary amine (PSA). For the second clean-up, extracts were transferred to mini-columns of 1 g silica gel and 1 g of anhydrous sodium sulphate and eluted with a n-hexane:toluene (v:v, 65:35) solution, followed by a toluene elution (Ciscato, Reference Ciscato2008).
The chosen target compounds for analysis are of historical and economic interest in Brazil: α-BHC, β-BHC, α-endosulphan, β-endosulphan, endosulphan sulphate, pp′-DDD, op′-DDD, pp′-DDE, op′-DDE, heptachlor, dicofol and mirex. All OC analytical standards had over 98.9% purity (Dr Ehrenstorfer®, Augsburg, Germany; Chemservice, West Chester, USA) and solvent purities were higher than 98% (JT Baker®; Tedia Company Inc®; Macron™ Chemicals). Separation, detection and quantification of OCs was performed using a gas-phase chromatographic (GC) system (Agilent 7890A) with an autosampler (Agilent 7683) in a pulsed-split mode, Agilent HP capillary column (30 m × 320 µm × 0.25 µm) and a micro-electron capture detector (μECD) coupled to ChemStation B.04.02 software. Operating conditions of the GC- μECD system were as follows: initial temperature 100°C; followed by an increase of 20°C min−1 to 210°C, held for 3 min; an increase of 15°C min−1 to 230°C, maintained for 5 min; an increase of 10°C min−1 to 280°C, held for 3 min; carrier gas (N2) with a constant flow of 1 ml min−1; make-up gas (N2) at 39 mL min−1; detector temperature at 300°C. The total analytical run time was 22.8 min.
The method was validated based on the guidelines of the Eurachem guide (Magnusson & Örnemark, Reference Magnusson and Örnemark2014). The limits of quantification (LQs) were lower than 5.3 ng g−1 for all compounds; a medium matrix effect was observed with signal suppression; the matrix extract-calibration curve showed linearity in the range of 0.5–100 ng mL−1; recovery was satisfactory at concentrations of 6 and 60 ng g−1. The quality control (QC) was performed by injection of blank samples and not showing interference above 30% of LQs, and fortified samples injected with all real samples, with recoveries at 60 ng g−1 between 70% and 120%, with recoveries higher than 120% only observed in a few cases for β-BHC and Dicofol (validation and QC results not displayed).
Statistical analysis
The following concentration sums were calculated: ΣHCHs (α-BHC, β-BHC), ΣDDTs (op′-DDE, pp′-DDE, op′-DDD, pp′-DDD), Σendosulphans (α-endosulphan, β-endosulphan, endosulphan sulphate) and ΣOCs (all analysed compounds).
The analyses employed statistical methods appropriate to left-censored data and considered all the results, including those below the LQ. Means, medians and standard deviations for all OCs were estimated using the Kaplan–Meier approach. The Gehan–Wilcoxon test as modified by Peto & Peto was used to test for differences among locations (Vitória, Ubatuba, Praia Grande) and fibropapillomatosis (with FP or without FP), as recommended by Helsel (Reference Helsel2005) and Lee (Reference Lee2013). Analyses were performed using the open-source R software (R Core Team, 2014) and NADA package (Lee, Reference Lee2013). The significance level (α) was 0.05 for all analyses.
RESULTS
All compounds were found in concentrations higher than the LQ in at least one sample. Table 1 summarizes the OC concentrations in fat and liver samples. These concentrations were compared with respect to the location and occurrence of fibropapillomatosis and the results are presented in Tables 1 and 2.
Table 1. Concentrations of organochlorine compounds (ng g−1 wet weight) in the fat and livers of green sea turtles (Chelonia mydas) caught at the studied areas.
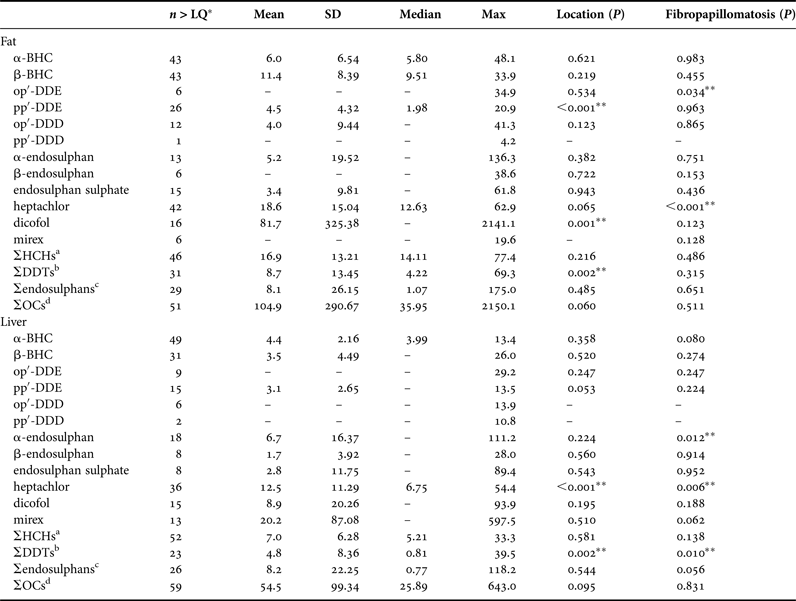
a Sum of α-BHC, β-BHC.
b Sum of op′-DDE, pp′-DDE, op′-DDD, pp′-DDD.
c Sum of α-endosulphan, β-endosulphan, endosulphan sulphate.
d Sum of all analysed compounds.
*Number of samples with concentrations >LQ; **Significant difference (P < 0.05). Some parameters could not be obtained due to an insufficient number of samples >LQ. Mean and SD were not estimated for compounds in which 80% or more of the individuals had concentrations below LQ.
Table 2. Comparison of the concentrations of organochlorine compounds (ng g−1 wet weight) in the fat and livers of green sea turtles (Chelonia mydas) having significant differences with respect to the location and/or occurrence of fibropapillomatosis.

a Sum of op′-DDE, pp′-DDE, op′-DDD, pp′-DDD.
*Number of samples with concentrations >LQ; **Parameters that could not be obtained due to an insufficient number of samples >LQ. Parameters are presented only for statistically significant comparisons.
DISCUSSION
The concentration of organochlorine compounds identified in this study contribute to the establishment of a toxicological baseline for the Brazilian coast, a region for which considerable gaps in our knowledge still exist (Keller, Reference Keller, Wyneken, Lohmann and Musick2013).
A recurring problem in sea turtle toxicological studies is that each investigation analyses different tissues from individuals with diverse combinations of age class, sex and body condition and reports on a different group of compounds employing distinct laboratory methods and concentration units; as a result, data from different investigations often cannot be compared (Keller, Reference Keller, Wyneken, Lohmann and Musick2013). Furthermore, it is important to keep in mind that the concentrations of POPs in the tissues of sea turtles are driven by complex interactions of biological (lipid content, body condition, trophic status, age, sex), environmental (water currents, air movements, temperature, precipitation, salinity, organic matter content, etc.) and human factors (localized uses and chemical releases within certain watersheds), and it is therefore difficult to distinguish the effect of these factors (Keller, Reference Keller, Wyneken, Lohmann and Musick2013). This, combined with the fact that sea turtles are migratory animals, warrants caution when interpreting the geographic patterns that may occur in the concentrations of POPs in the tissues of these animals.
In this study, the fact that we employed GC-μECD with a relatively short capillary column length (30 m) may have limited the accuracy and precision of the results. Validation data suggest that co-elution of halogenated compounds may have occurred to some extent, elevating the measured concentrations for some of the compounds studied. Bearing this in mind, future studies are advised to employ more selective gas chromatography methods such as mass spectrometry (MS), along with a longer capillary column (preferably 60 m), which should produce more faithful measurements of OCs concentrations in the species. With these limitations in mind, we briefly compare our findings to those of other investigations on the concentrations of organochlorine compounds in the tissues of green sea turtles.
The results of total dichlorodiphenyltrichloroethanes (ΣDDTs) from different studies are difficult to compare because often different DDT metabolites are quantified. Nevertheless, a few patterns are consistently observed across studies. Due to its highly persistent nature, the pp′-DDE isomer is often the predominant metabolite in sea turtles (McKim & Johnson, Reference McKim and Johnson1983; Rybitski et al., Reference Rybitski, Hale and Musick1995; McKenzie et al., Reference McKenzie, Godley, Furnes and Wells1999; Keller et al., Reference Keller, Kucklick, Harms and McClellan-Green2004a, Reference Keller, Kucklick, Stamper, Harms and McClellan-Greenb; van de Merwe et al., Reference van de Merwe, Hodge, Olszowy, Whittier and Lee2010; Malarvannan et al., Reference Malarvannan, Takahashi, Isobe, Kunisue, Sudaryanto, Miyagi, Nakamura, Yasumura and Tanabe2011), and in some cases may represent up to 80% of all DDT concentrations (Lazar et al., Reference Lazar, Maslov, Romanic, Gracan, Krauthacker, Holcer and Tvrtkovic2011). Concentrations of pp′-DDE in fat tissue in this study are close to those reported in juvenile green sea turtles at Cyprus (McKenzie et al., Reference McKenzie, Godley, Furnes and Wells1999), and the concentrations of this compound in the liver are similar to those reported in green sea turtles in East Florida (McKim & Johnson, Reference McKim and Johnson1983) and Cyprus (McKenzie et al., Reference McKenzie, Godley, Furnes and Wells1999). It is worth noting, however, that exceptions to this rule exist and pp′-DDD can also be a major contributor to the DDT group (Gardner et al., Reference Gardner, Pier, Wesselman and Juárez2003).
Residual DDT patterns in dolphins suggest that op′-DDT is more recalcitrant than pp′-DDT in the body of the animals and/or in the environment, and this compound seems to be preferentially converted into op′-DDD rather than op′-DDE (Yogui et al., Reference Yogui, Santos, Bertozzi and Montone2010). In this study a predominance of pp′-DDE was found in fat while pp′-DDD was predominant in the liver, with op′-DDE present in the lowest concentrations for both tissues. Moreover, the pattern observed in this study suggests a tendency to convert DDT metabolites into op′-DDD rather than op′-DDE. The DDT pattern could be better explained by the calculation of the DDE/ΣDDT ratio, an index used for assessment of the chronology of contaminant input into the ecosystem (Aguilar, Reference Aguilar1984). In this study, the pp′-DDE/ΣDDT ratio was low (0.52). Had op′-DDT and pp′-DDT metabolites been quantified, this index could have been lower, but not higher. A value lower than 0.6 may indicate recent application of DDTs (Aguilar, Reference Aguilar1984). This is consistent with the similarly low pp′-DDE/ΣDDT ratio (0.56) found in coastal marine mammals near metropolitan areas in north-eastern Brazil, suggesting exposure to new releases of DDTs, in addition to higher use and persistence in the environment (Santos-Neto et al., Reference Santos-Neto, Azevedo-Silva, Bisi, Santos, Meirelles, Carvalho, Azevedo, Guimarães and Lailson-Brito2014).
In addition to DDTs, other organochlorine compounds that can also be highlighted as particularly relevant are dicofol, mirex, heptachlor and HCHs. Although dicofol has not been evaluated in past toxicological studies of sea turtles, the levels reported here in fat tissue are similar to the total levels of PCBs (one of the most predominant POPs in sea turtles) reported in the fat of green sea turtles at the Canary Islands (Orós et al., Reference Orós, Gonzáles-Díaz and Monagas2009) and Cyprus (McKenzie et al., Reference McKenzie, Godley, Furnes and Wells1999) and are higher than the total PCBs in green sea turtles at Baja California, Mexico (Gardner et al., Reference Gardner, Pier, Wesselman and Juárez2003).
It is interesting to compare our results to studies at Lake Apopka, Florida, where a large spill of pesticides (dicofol, DDT and others) occurred in the 1980s and caused endocrine and reproductive problems in American alligators (Alligator mississippiensis), culminating in a 90% population decrease (Woodward et al., Reference Woodward, Percival, Jennings and Moore1993; Guillette et al., Reference Guillette, Gross, Masson, Matter, Percival and Woodward1994, Reference Guillette, Brock, Rooney and Woodward1999). In that case, plasma ΣDDTs was 7.8 ng mL−1 in males and 19.88 ng mL−1 in females (Crain et al., Reference Crain, Guillete, Pickford, Percival and Woodward1998). Because different biological samples were analysed, it is difficult to compare the results from alligators to the ΣDDTs concentrations in this study (8.7 and 4.8 ng g−1 in fat and liver, respectively). However, if we consider that ΣDDTs concentrations in the fat of sea turtles are approximately 100 times higher than those in the blood (Keller et al., Reference Keller, Kucklick, Harms and McClellan-Green2004a), this would imply that ΣDDTs concentrations in this study were approximately two orders of magnitude lower than those observed in alligators at Lake Apopka.
No clear pattern was observed for other compounds; mirex concentrations in the liver of sea turtles are higher in this study than those reported for the same species in Queensland, Australia (van de Merwe et al., Reference van de Merwe, Hodge, Olszowy, Whittier and Lee2010). Chlordanes such as heptachlor presented lower values in both fat and liver in this study than the total chlordanes in green sea turtles at Baja California, whereas α-endosulphan and β-endosulphan concentrations were higher than in this study (Gardner et al., Reference Gardner, Pier, Wesselman and Juárez2003). HCH concentrations in the fat and liver samples in this study were generally higher than in loggerhead turtles (Caretta caretta) in North Carolina. This finding is surprising given the lower trophic level of green sea turtles, as it would normally be expected that turtle species with the highest trophic status would accumulate higher POP concentrations (Keller, Reference Keller, Wyneken, Lohmann and Musick2013).
The OC concentrations observed in this study are at least to some extent probably due to a recent or large historical release of these compounds off the Brazilian coast. Additionally, the chemical properties of some of these compounds may also help explain the patterns observed. For example, mirex was used as both pesticide and flame retardant in Brazilian states, such São Paulo, and the higher chlorination level of this compound may also contribute to its persistence in the environment (Yogui et al., Reference Yogui, Santos, Bertozzi and Montone2010), explaining the relatively high values of mirex observed here. In this study mirex was detected at higher levels than HCHs, as previously reported on small cetaceans from Brazil (Yogui et al., Reference Yogui, Santos, Bertozzi and Montone2010). On the other hand, HCHs are highly volatile, and as a result it is reasonable to expect to find them in lower concentrations in the tissues of marine animals in tropical areas (Tanabe et al., Reference Tanabe, Subramanian, Ramesh, Kumaran, Miyazaki and Tatsukawa1993); however, this was not seen in this study, as relatively high concentrations of HCHs were observed. HCHs and mirex values reported in liver were higher than reported for locations such as Queensland, Australia and Ishigaki Island, Japan (Keller, Reference Keller, Wyneken, Lohmann and Musick2013). A possible explanation for the high HCH concentrations in this study is that they may have been overestimated due to co-elution of other halogenated compounds.
Even though some significant differences were found in OC levels between turtles with and without FP, there was not a consistent pattern. In many cases, OC concentrations were higher in turtles without FP than in those affected by this disease. The hypothesis that exposure to OCs could be a co-factor for FP occurrence is therefore not supported by the findings of this study; this agrees with similar studies in Hawaii (Aguirre et al., Reference Aguirre, Balazs, Zimmerman and Galey1994a; Miao et al., Reference Miao, Balazs, Murakawa and Li2001; Keller et al., Reference Keller, Balazs, Nilsen, Rice, Work and Jensen2014) and Brazil (Silva, Reference Silva2009; Rossi, Reference Rossi2014).
Regardless of the role played by OCs in the pathogenesis of FP, it should be noted that even the relatively low levels of OCs observed here may affect a wide variety of biological functions, including immunity, proteins and ion homeostasis. It is unlikely that evidence will be found of an acute toxic effect from such OC concentrations, but chronic effects, particularly on immunity, cannot be discarded (Rybitski et al., Reference Rybitski, Hale and Musick1995; Keller et al., Reference Keller, Kucklick, Stamper, Harms and McClellan-Green2004b, Reference Keller, McClellan-Green, Kucklick, Keil and Peden-Adams2006). Seals experimentally fed polluted fish from the Baltic Sea demonstrated suppression of immune functions (De Swart et al., Reference De Swart, Ross, Vedder, Timmerman, Heisterkamp, Van Loveren, Vos, Reijnders and Osterhaus1994) and impairment of the immune system against viral infections following exposure to PCBs has also been shown experimentally in ducks (Friend & Trainer, Reference Friend and Trainer1970) and in mice (Imanishi et al., Reference Imanishi, Nomura, Matsubara, Kita, Won, Mizutani and Kishida1980; Krzystyniak et al., Reference Krzystyniak, Hugo, Flipo and Fournier1985, Reference Krzystyniak, Bernier, Hugo and Fournier1986). Epidemiological evidence also suggests an association between the presence of pollutants in the tissues of marine animals and the occurrence of infections and physiological dysfunctions in free-ranging marine animals (De Guise et al., Reference De Guise, Lagacé and Béland1994, Reference De Guise, Lagacé, Béland, Girard and Higgins1995a, Reference De Guise, Martineau, Béland and Fournierb; Martineau et al., Reference Martineau, Lair, de Guise and Béland1995; Jepson et al., Reference Jepson, Bennett, Allchin, Law, Kuiken, Baker, Rogan and Kirkwood1999; Jenssen et al., Reference Jenssen, Haugen, Sormo and Skaare2003). In the case of sea turtles, recent studies have found evidence that chronic exposure to OCs may suppress innate immunity or enhance certain lymphocyte functions in loggerhead turtles (Keller et al., Reference Keller, McClellan-Green, Kucklick, Keil and Peden-Adams2006).
In conclusion, our study confirms that green sea turtles in the South-west Atlantic are exposed to persistent organic pollutants. Although no evidence was found to corroborate a direct relationship between OCs and fibropapillomatosis in the studied sea turtles, these compounds could play an indirect role in the pathogenesis of this disease and/or otherwise affect the health, survival and reproduction of these animals.
ACKNOWLEDGEMENTS
We would like to thank the Projeto TAMAR/ICMBio; the Programa de Pós-Graduação em Patologia Experimental e Comparada, Faculdade de Medicina Veterinária e Zootecnia, Universidade de São Paulo (FMVZ/USP); Cecília Baptistotte; José Henrique Becker; Renato Velloso da Silveira; Max Rondon Werneck; staff and collaborating fishermen from Projeto Biopesca; Jorge Oyakawa from Laboratório de Patologia Comparada de Animais Selvagens, Universidade de São Paulo; Rodrigo Pimpinato and Carlos Alberto Dorelli from Laboratório de Ecotoxicologia, Centro de Energia Nuclear na Agricultura, Universidade de São Paulo and Marcillo Altoé Boldrin from CTA-Meio Ambiente. The authors also wish to express their gratitude to Jonas Shimizu de Oliveira, Nicolle Queiroz-Hazarbassanov and an anonymous reviewer for the revision of the draft and our families whose support was really important in the completion of this research.
FINANCIAL SUPPORT
This study was supported by Fundação de Amparo à Pesquisa do Estado de São Paulo – FAPESP (grant number, 2012/14319-6), (A.M.S.S., grant number, 2011/04565-7), (S.R., grant number, 2010/01781-8), (R.E.T.V., grant number, 2009/53956-9); and Conselho Nacional de Desenvolvimento Científico e Tecnológico – CNPq (A.M.S.S., grant number, 130082/2011-2).