INTRODUCTION
All known species of the caridean family Alvinocarididae Christoffersen, Reference Christoffersen1986 occur exclusively in hydrothermal vents or cold (brine and hydrocarbon) seeps (see Table 1 and references therein). Alvinocaridids are known from a considerable number of vents and seeps in the Atlantic, Pacific and Indian Oceans (Table 1). Alvinocaridid shrimp are the dominant macrofaunal invertebrates at several vents along the Mid-Atlantic Ridge (MAR) (e.g. Van Dover et al., Reference Van Dover, Fry, Grassle, Humphris and Rona1988; Segonzac, Reference Segonzac1992; Gebruk et al., Reference Gebruk, Pimenov and Savvichev1993, Reference Gebruk, Galkin, Vereshchaka, Moskalev and Southward1997; Van Dover, Reference Van Dover2000; Martin & Shank, Reference Martin and Shank2005) and at known vent fields in the Indian Ocean (Hashimoto et al., Reference Hashimoto, Ohta, Gamo, Chiba, Yamaguchi, Tsuchida, Okudaira, Watabe, Yamanaka and Kitazawa2001; Van Dover et al., Reference Van Dover, Humphris, Fornari, Cavanaugh, Collier, Goffredi, Hashimoto, Lilley, Reysenbach, Shank, Von Damm, Banta, Gallant, Gotz, Green, Hall, Harmer, Hurtado, Johnson, McKiness, Meredith, Olson, Pan, Turnipseed, Won, Young and Vrijenhoek2001; Watabe & Hashimoto, Reference Watabe and Hashimoto2002). The Alvinocarididae presently comprises 26 described species in 8 genera, all from chemosynthetic environments in the bathymetric range 252–4088 m (Table 1).
Table 1. Summary of known geographical distribution, bathymetric range and habitat of alvinocaridid shrimp species (confirmed locations and fully described species only).

CIR, Central Indian Ridge; ELSC, East Lau Spreading Centre; EPR, East Pacific Rise; GoM, Gulf of Mexico; GR, Galapagos Rift; KR, Kermadec Ridge, New Zealand; LB, Lau Basin; MAR, Mid-Atlantic Ridge; MBAB, Mariana Back-Arc Basin: MCSC, Mid-Cayman Spreading Centre; OT, Okinawa Trough; SB, Sagami Bay.
Alvinocaris Williams & Chace, Reference Williams and Chace1982 is presently the only genus known to inhabit both hydrothermal vents and cold seeps (Table 1). Alvinocaris, Rimicaris Williams & Rona, Reference Williams and Rona1986, Chorocaris Martin & Hessler, Reference Martin and Hessler1990 and Opaepele Williams & Dobbs, Reference Williams and Dobbs1995 were originally assigned to the caridean family Bresiliidae Calman, Reference Calman1896 (see Martin & Davis, Reference Martin and Davis2001; Komai & Segonzac, Reference Komai and Segonzac2003), whereas the family Mirocarididae Vereshchaka, Reference Vereshchaka1997 was established to accommodate the genus Mirocaris Vereshchaka, Reference Vereshchaka1997. Komai & Segonzac (Reference Komai and Segonzac2003) subsequently assigned all these genera to the Alvinocarididae, and synonymized the family Mirocarididae with the Alvinocarididae.
The family Bresiliidae is now only represented by two genera (De Grave et al., Reference De Grave, Pentcheff, Ahyong, Chan, Crandall, Dworschak, Felder, Feldmann, Fransen, Goulding, Lemaitre, Low, Martin, Ng, Schweitzer, Tan, Tshudy and Wetzer2009), Bresilia Calman, Reference Calman1896 and the monotypic Encantada Wicksten, Reference Wicksten1989, neither of which are known to occur in chemosynthetic habitats (Wicksten, Reference Wicksten1989; Komai & Yamada, Reference Komai and Yamada2010, Reference Komai and Yamada2011). The family Alvinocarididae (Christoffersen, Reference Christoffersen1986, Reference Christoffersen1990; Segonzac et al., Reference Segonzac, de Saint Laurent and Casanova1993; Komai & Segonzac, Reference Komai and Segonzac2003) is now regarded as a valid monophyletic family (e.g. Komai & Segonzac, Reference Komai and Segonzac2005, Reference Komai and Segonzac2008; Martin & Haney, Reference Martin and Haney2005; De Grave et al., Reference De Grave, Pentcheff, Ahyong, Chan, Crandall, Dworschak, Felder, Feldmann, Fransen, Goulding, Lemaitre, Low, Martin, Ng, Schweitzer, Tan, Tshudy and Wetzer2009), morphologically distinct from opportunistic shrimp species recorded from vents and seeps (see Martin & Haney, Reference Martin and Haney2005; Desbruyères et al., Reference Desbruyères, Segonzac and Bright2006 for recent reviews).
Recently, two high-temperature hydrothermal vent fields and chemosynthetic communities were discovered on the world's deepest seafloor spreading centre, the Mid-Cayman Spreading Centre (MCSC), Caribbean (Connelly et al., in press). The ~110 km long, ultraslow-spreading (15 mm yr−1) MCSC has been active for approximately 49 My (Rosencrantz et al., Reference Rosencrantz, Ross and Sclater1988; German et al., Reference German, Bowen, Coleman, Honig, Huber, Jakuba, Kinsey, Kurz, Leroy, McDermott, Mercier de Lepinay, Nakamura, Seewald, Smith, Sylva, Van Dover, Whitcomb and Yoerger2010) and is located in a deep trough, geographically and tectonically isolated from the global mid-ocean ridge system (Ballard et al., Reference Ballard, Bryan, Dick, Emery, Thompson, Uchupi, Davis, De Boer, Delong, Fox, Spydell, Stroup, Melson and Wright1979).
The Beebe Vent Field (BVF) consists of a sulphide mound (80 m diameter, 50 m height) surmounted with several actively venting sulphide chimneys and, at 4960 m, it is the world's deepest known vent field (Connelly et al., in press). The Von Damm Vent Field (VDVF) occurs 30 km from the BVF, on the upper slopes of an off-axis massif, at 2300 m depth (Connelly et al., in press). The VDVF comprises a sulphide mound (100 m diameter, 30 m height) venting predominantly clear, buoyant, high-temperature (>140°C) fluids from orifices at its peak (Connelly et al., in press). Study of the fauna inhabiting these unique vents has the potential to enhance current understanding of the dispersal, isolation, and evolution of vent taxa and patterns of vent biogeography.

Fig. 1. Rimicaris hybisae sp. nov., live specimen, from the Beebe Vent Field, Mid-Cayman Spreading Centre. P denotes twin pores in the dorsal organ. 69 × 57 mm (150 × 150 DPI).
The fauna at both vent fields is dominated by dense aggregations of Rimicaris hybisae (Figure 1), a new species of alvinocaridid shrimp, with a greatly reduced rostrum, and a four-lobed dorsal organ, similar to the photoreceptor of Rimicaris (Van Dover et al., Reference Van Dover, Szuts, Chamberlain and Cann1989). Rimicaris hybisae sp. nov., described and illustrated herein, is the first taxon to be described from vent fields on the MCSC. In addition to enhancing existing knowledge about biodiversity, this record extends the known geographical range of Rimicaris (previously only recorded from Atlantic and Indian Ocean vents; Table 1) westwards into the Caribbean Sea, and extends the known bathymetric range of the Alvinocarididae by 872 m.
MATERIALS AND METHODS
The specimens were collected during the 44th cruise of the RRS ‘James Cook' in April 2010 from Beebe (4960 m) and Von Damm (2300 m) hydrothermal vent fields on the MCSC, Caribbean. Shrimp samples were taken using a grab (Von Damm) and suction sampler (Beebe) attached to the manoueverable TV grab HyBIS (Hydraulic Benthic Interactive Sampler), together with still photographs and video recordings of them in situ at both sites. Material for morphological study was immediately fixed in 10% neutralized formalin and subsequently transferred to 80% industrial methylated spirits on return to the laboratory. Material for molecular analysis was immediately placed in 100% ethanol.
The measurements taken for each specimen (Table 2) were measured to the nearest 0.1 mm using Vernier callipers. Post-orbital carapace length (CL) was measured from the midpoint of the posterodorsal margin to the level of the posterior margin of the orbit. Maximum total length (TL) was measured from the posterior margin of the telson to the anterior margin of the antennal scale. Maximum carapace width (CW) and maximum carapace depth (CD) were measured at the widest and deepest points of the carapace respectively. Specimen size herein is indicated by post-orbital CL.
Table 2. Morphological variation in Rimicaris hybisae sp. nov. Mid-Cayman Spreading Centre vent fields are abbreviated as VDVF (Von Damm) and BVF (Beebe).

CL, post-orbital carapace length; CW, maximum carapace width; CD, maximum carapace depth; TL, total length; AS4, abdominal somite 4, posterolateral margin; AS5, abdominal somite 5, posterolateral margin; ovi, ovigerous; x, measurement could not be taken due to condition of carapace; *, with visibly mature ovary.
Individuals were sexed under a dissecting microscope. Males were distinguished by an asymmetrical mesial extension on the endopod of pleopod 1 and the presence of the appendix masculina on the second pleopod (Willliams, 1988). The sex of specimens CL 6.7 mm and smaller could not be determined by this method; those specimens are referred to as juveniles.
Drawings were prepared with the aid of a camera lucida mounted onto a Leica MZ8 stereomicroscope, scanned and digitally inked using Adobe® Illustrator® and a WACOM™ digitizer, as described by Coleman (Reference Coleman2003, Reference Coleman2009). The material examined is deposited in the Natural History Museum, UK (NHMUK). The descriptive terminology used follows that of Komai & Segonzac (Reference Komai and Segonzac2008).
One specimen from the BVF was used for scanning electron microscopy (SEM) and was not designated as a paratype. This specimen was dehydrated through a graded ethanol series, critical point dried and sputter coated with gold palladium prior to examination with a FEI Quanta 200 scanning electron microscope at accelerating voltage of 10 kV.
Abdominal muscle for DNA extraction was cut from the shrimp of ethanol-preserved specimens (from both the Beebe and Von Damm vent fields) and the carapace removed. Genomic DNA was extracted using the CTAB (cetyltrimethyl ammonium bromide) procedure (Doyle & Dickson, Reference Doyle and Dickson1987). Regions of the mitochondrial genes cytochrome c oxidase subunit I gene (COI) and 16S ribosomal RNA, and of the nuclear 18S ribosomal RNA gene were amplified by performing polymerase chain reactions (PCR).
The COI region was amplified with universal primers LCO1490 and HCO2198 (Folmer et al., Reference Folmer, Black, Hoeh, Lutz and Vrijenhoek1994). The 20 µl amplification mixture contained 1X buffer reagent (200 mM Tris pH 8.8, 500 mM KCL, 0.1% Trixton X-100, 2 mg/ml bovine serum albumen), 2 mM MgCl2, 0.2 mM of each dNTP, 0.5 mM of each primer, 1 U Taq DNA polymerase (Bioline), 5 µl of template DNA and sterile H2O to final volume. Thermal cycling conditions were: 94°C/2 minutes; followed by 5 cycles at (94°C/35 seconds; 45°C/35 seconds; 72°C/1:20 minutes) and 35 cycles at (94°C/35 seconds; 50°C/35 seconds; 72°C/1:20 minutes) with a final extension of 72°C/ 10 minutes.
For the 16S gene, PCR amplifications were performed using the universal primers 16Sar and 16Sbr (Palumbi, Reference Palumbi, Hillis, Moritz and Mable1996) and a 20 µl amplification mixture: 1X reaction buffer (same as for COI), 2.5 mM MgCl2, 0.13 mM of each dNTP, 0.38 mM of each primer, 1 U Taq DNA polymerase (Bioline), 2.5 µl of template DNA and sterile H2O to final volume. Thermal cycling conditions were: 94°C/4 minutes; 30 cycles at (94°C/30 seconds; 52°C/1 minutes; 72°C/2 minutes) and 72°C/5 minutes.
Polymerase chain reaction amplifications of the 18S gene were performed using universal primers 18SuniF and 18SuniR (Sogin, Reference Sogin, Innis, Gelfand, Sninsky and White1990) in an amplification mixture as described for COI. Thermal cycling conditions were: 95°C/5 minutes; 30 cycles at (94°C/1 minute; 64°C/1 minute; 72°C/2:30 minutes) and 72°C/10 minutes. Negative controls were included as standard and sterile procedures were consistently followed for all PCR experiments.
Polymerase chain reaction products were purified with the ExoAP treatment by adding the following ExoAP mixture to 15 µl PCR product: 0.2 µl 10X ExoAP buffer (50 mM Bis-Tris, 1mM MgCl2, 0.1 mM ZnSO4), 0.05 µl 5000 U/ml Antarctic Phosphatase (New England Biolabs: Ipswich, MA), 0.05 µl 20000 U/ml Exonuclease I, and 3.7 µl sterile H2O) and thermal-cycler incubation (37°C/60 minutes; 85°C/15 minutes). Sequencing reactions were performed using BigDye Terminator Reactions following the manufacturer's protocol (Applied Biosystems: Foster, CA) with the same primer sets used for amplifications. For COI, the thermal-cycler reaction was performed as: 94°C/30 seconds followed by 25 cycles at (94°C/15 seconds; 50°C/15 seconds; 60°C/3 minutes). For 16S and 18S the PCR conditions were identical to those described for COI, except for the use of a 52°C and 64°C annealing temperature respectively. Sequencing reaction products were purified with the AMPure magnetic bead system (Agencourt: Morrisville, NC) following the manufacturer's protocol and were subsequently run on an ABI 3730x1 DNA Analyzer (Applied Biosystems International).
The sequence strands for each gene were proof-read and assembled with CodonCode Aligner, version 3.7.1 (CodonCode Corporation, Dedham, MA, USA), to produce a continuous fragment. Sequences were compared with those in GenBank using the nucleotide BLAST program (NCBI Basic Alignment Search Tool) and manually aligned in BioEdit (Hall, Reference Hall1999). Phylogenetic trees were constructed with MEGA5 (Tamura et al., Reference Tamura, Peterson, Peterson, Stecher, Nei and Kumar2011) using the neighbour-joining (NJ) (Saitou & Nei, Reference Saitou and Nei1987) and maximum-likelihood (ML) (Kimura, Reference Kimura1980) methods on 460- and 540-base pair (bp) alignments for COI and 18S respectively. Bootstrap values were calculated on 1000 re-sampling replicates.
GenBank accession numbers for partial sequences of the 16S, COI and 18S regions are JN850606, JN850607 and JN850608 respectively.
Rimicaris Williams & Rona, Reference Williams and Rona1986: 447 (in part); Martin & Hessler, Reference Martin and Hessler1990: 8; Holthuis, Reference Holthuis1993; Iorania Vereshchaka, Reference Vereshchaka1996: 952; Martin & Haney, Reference Martin and Haney2005: 467; Komai & Segonzac, Reference Komai and Segonzac2008: 22.
TYPE SPECIES
Rimicaris exoculata Williams & Rona, Reference Williams and Rona1986
DIAGNOSIS (EMENDED)
Carapace greatly inflated laterally, distinctly broader than pleon, dorsal surface rounded, pitted with scattered, shallow punctuations. Pterygostomial expansion produced, exceeding antennal lobe, covering greater part of antennal bascicerite, rounded or blunt. Rostrum reduced to broadly rounded lobe. Eyes lacking pigment, eyestalks flattened, greatly reduced and medially fused. Antennal scale broadly oval, bearing distolateral transverse suture. Mandible with two-segmented palp, distinct separation between incisor and molar processes. Maxilla with scaphognathite greatly expanded anteriorly and conspicuously setose on dorsal and ventral surfaces. First maxilliped with greatly expanded exopod, similar to scaphognathite, conspicuously setose on dorsal and ventral surfaces. Third maxilliped with three long segments and coxa.
COMPOSITION
Rimicaris exoculata Williams & Rona, Reference Williams and Rona1986 (MAR, 45°N–4°47S), Rimicaris kairei Watabe & Hashimoto, Reference Watabe and Hashimoto2002 (Central Indian Ridge, Kairei and Edmond vent fields) and Rimicaris hybisae sp. nov. (Beebe and Von Damm vent fields, MCSC, Caribbean).
TYPE MATERIAL
Holotype: adult female, CL 15.3 mm. VDVF, MCSC, Caribbean Sea; co-ordinates: 18°22.605′N81°47.875′W; water depth: 2300 m, [NHMUK 2011.8054]. Collected on the 44th voyage of RRS ‘James Cook', on 18 April 2010.
Paratypes: adult male, CL 9.4 mm. VDVF, MCSC, Caribbean Sea; co-ordinates: 18°22.605′N81°47.875′W; water depth: 2300 m, [NHMUK 2011.8055]. Collected during the 44th cruise of the RRS ‘James Cook’, on 18 April 2010. Adult female, CL 10.8 mm; adult female with visible mature ovary, CL 7.0 mm; seven adult males, CL 7.0–11.8 mm; six juveniles, CL 3.6–6.7 mm. BVF, MCSC, Caribbean Sea; co-ordinates: 18°32.785′N 81°43.080′W; water depth: 4960 m, [NHMUK 2011.8056-8070]. Collected on the 44th voyage of RRS ‘James Cook', on 15 April, 2010.
COMPARATIVE MATERIAL EXAMINED
Chorocaris chacei (Williams & Rona, Reference Williams and Rona1986). Ten males, CL 9.7–13.0 mm [MNHN–Na17811]. MAR (Lucky Strike: Tour Eiffel; 37°17′N 32°17′W). Collected by net, 1689 m depth.
Chorocaris vandoverae Martin & Hessler, Reference Martin and Hessler1990. Paratypes: seven females, CL 8.0–12.6 mm [USNM 243947]. Mariana Back Arc Basin (Alice Spring Vent Field; 18°12.599′N 144°42.231′E). Collected by net, 3640 m depth.
Rimicaris exoculata Williams & Rona, Reference Williams and Rona1986. Paratypes: ten females, CL 14.3–18.4 mm [USNM 228447]. MAR (TAG; 26°08.18′N 44°49.36′W). Collected by dredge, 3620–2650 m depth. Paratypes: five juveniles, CL 8.4–9.2 mm [USNM 228454]. Labelled as Rimicaris chacei; classified as R. exoculata juveniles at stage B by Komai & Segonzac (Reference Komai and Segonzac2008). MAR (TAG; 26°08.3′N 44°49.6′W). Collected by dredge, 2650–3620 m depth. Twenty-Four additional specimens: eleven males, CL 11.3–18.1; thirteen females, CL 8.8–18.4 mm from J. Copley's reference collection. MAR (TAG).
Rimicaris kairei Watabe & Hashimoto, Reference Watabe and Hashimoto2002. Paratype: one female, CL 19.3 mm [USNM 1005217]. Central Indian Ridge, Rodriguez Triple Junction (Kairei Vent Field; 25°19.16′S 70°02.40′E). Collected by suction sampler, 2454 m.
Opaepele loihi Williams & Dobbs, Reference Williams and Dobbs1995. Paratypes: twenty females, CL 8.0–11.7 mm [USNM 1005217]. North Pacific Ocean (Loihi Seamount; 18°55′N 155°16′W). Collected by baited traps, 990 m depth.
DIAGNOSIS
Rostrum reduced to broadly rounded lobe, nearly reaching, reaching or slightly overreaching anterior margins of medially-fused eyes; ventral surface flat or slightly convex. Carapace and pleon with scattered minute setae; antennal lobe broadly triangular or rounded; pterygostomial lobe rounded or blunt. Fourth pleonal pleuron posterolateral margin unarmed or armed with one tooth; produced into subacute posteroventral angle. Fifth pleonal pleuron posterolateral margin unarmed marginally or armed with 1–4 teeth; produced into acute posteroventral angle. Antennae not operculiform; distolateral tooth first antennal peduncle subacute or blunt; antennal scale distolateral tooth subacute. Scaphognathite of maxilla and caridean lobe of first maxilliped bearing numerous plumose setae-like structures on dorsal and ventral surfaces; exopodal flagellum of first maxilliped completely reduced. Appendix masculina tapering distally with 7–8 spiniform setae restricted to tip. Uropodal protopod posterolateral projection triangular with acute tip.
In life, with four-lobed dorsal organ; lobes fused anteriorly; posterior lobes with paired ‘pores' (Figure 1).
DESCRIPTION
Body integument smooth, firm, pitted with scattered, very shallow punctuations bearing minute scattered setae (including rostrum). Carapace (Figure 2) ovate–oblong, generally broader than deep in greatest dimensions (Table 2), distinctly broader than pleon; branchial regions distinctly inflated but to a lesser extent than that of Rimicaris exoculata Williams & Rona, Reference Williams and Rona1986. Rostrum reduced to broadly rounded lobe; nearly reaching, reaching or slightly overreaching anterior margins of fused eye-stalks; dorsal surface rounded, ventral surface flat or slightly convex. Carapace dorsal surface rounded; obsolescent epigastric ridge present, broader than breadth between antennal lobes, defined by conspicuous grooves extending from bases of antennal lobes; ventral margin reinforced by low submarginal ridge, most robust and farthest from margin posteriorly. Antennal lobe rounded or broadly triangular, tip blunt or subacute; anterolateral margin between antennal lobe and pterygostomial expansion slightly convex. Pterygostomial expansion produced, exceeding antennal lobe, covering greater part of antennal bascicerite in larger specimens, rounded or blunt. Posterior submarginal groove present, poorly defined.

Fig. 2. Rimicaris hybisae sp. nov., holotype, female (carapace length 15.3 mm), [NHMUK 2011.8054], from the Von Damm Vent Field, Mid-Cayman Spreading Centre: (A) entire animal, lateral view; (B) carapace and cephalic appendages, lateral view; (C) carapace and cephalic appendages, dorsal view. Scale bars = 2 mm. 185 × 195 mm (150 × 150 DPI).
Abdomen (Figure 3A) evenly rounded dorsally, without carination; anterior three pleonal pleura unarmed marginally, posteroventral angle rounded. Fourth pleonal pleuron posterolateral margin unarmed or armed with one tooth; produced into subacute posteroventral angle. Fifth pleonal pleuron posterolateral margin unarmed marginally or armed with 1–4 teeth; produced into acute posteroventral angle. Sixth pleonal pleura 1.5–1.8 times longer than fifth in dorsal midline, 1.5 times longer than high; broadly notched for insertion of uropods; posterolateral process terminating in acute triangular tooth overlapping base of telson, posteroventral corner subacute. Armature of pleonal sternites as described for Chorocaris chacei Williams & Rona, Reference Williams and Rona1986.

Fig. 3. Rimicaris hybisae sp. nov., holotype, female (carapace length 15.3 mm), [NHMUK 2011.8054], from the Von Damm Vent Field, Mid-Cayman Spreading Centre: (A) third to sixth abdominal somites, lateral view; (B) telson and uropods, dorsal view; (C) posterior margin of telson, dorsal view. Scale bars = 1 mm. 219 × 275 mm (150 × 150 DPI).
Telson (Figure 3B, C) 1.5–1.7 times length of sixth pleonal pleura in dorsal midline, slightly narrowed posteriorly, length 2.2–2.9 times greatest width; posterior margin broadly convex, bearing row of 8–35 plumose setae and 2 spines at both lateral ends; 4–8 (sometimes asymmetrical) dorsolateral spines arranged in sinuous row.
Uropods (Figure 3B) with broad rami exceeding distal margin of telson; exopod with distinct transverse suture and two or three small spines at distolateral angle; endopod shorter and narrower than exopod; posterolateral projection of protopod triangular with acute tip.
Eye-stalks (Figure 2C) broadly fused mesially, lacking pigmentation, anterior surface without conspicuous tubercles.
Antennae (Figure 2) of normal structure. Antennular peduncles stout, dorsoventrally depressed, mesial surface rounded, not closely approximated; first and third segments nearly equal in length, second segment slightly longer; first segment with small distomesial tooth, strong distolateral tooth subacute or blunt, reaching or overreaching midlength of second segment, dorsal face convex; stylocerite moderately slender, lateral margin slightly convex, distinctly separated from first segment, extending beyond midlength of second segment; prominent proximolateral tubercle; second segment longer than broad or nearly as long as broad with small distomesial tooth. Antennular flagella robust, thick, similar in structure, tapering, sensory setae at joints between annuli, inserted next to each other on oblique terminal margin of third article, sweeping posterolaterally in gentle arc.
Antennal peduncles (Figure 2) shorter than antennular peduncles, both exceeded by antennal scale (scaphocerite); flagella larger (all dimensions) than those of antennule and of similar structure, sensory setae at joints of annuli, sweeping in gentle curve lateral to carapace, reaching as far as midlength of fourth pleonal pleuron in holotype female. Antennal scale (Figures 2 & 4A) broadly oval; convex lateral margin ending in subacute tooth directed forward, short transverse suture extending mesially from base of tooth; plumose setae fringing broadly rounded distal margin and convex mesial margin. Antennal basicerite bearing acute ventrolateral and ventromesial tooth.

Fig. 4. Rimicaris hybisae sp. nov., holotype, female (carapace length 15.3 mm), [NHMUK 2011.8054], from the Von Damm Vent Field, Mid-Cayman Spreading Centre: (A) right antennal peduncle, scale and proximal part of flagellum, ventral view; (B) right mandible, dorsal view; (C) right maxillule (first maxilla), ventral view; (D) right maxillule (second maxilla), ventral view; (E) right first maxilliped, ventral view; (F) right first maxilliped, dorsal view; (G) right second maxilliped, ventral view; (H) right third maxilliped, lateral view. Scale bars = 1 mm. 255 × 373 mm (150 × 150 DPI).
Mouthparts (Figure 4) typical of Alvinocarididae. Mandible (Figure 4B) bearing 7 unequal acute teeth on mesial margin of broad incisor process (4 large and 3 small in holotype female), distalmost tooth distinctly separated from remaining teeth; molar process slightly upcurved, slender, unarmed, not reaching incisor; palp biarticulate, proximal article weakly curved, bearing 2 long plumose setae on distolateral margin, distal article stout, distinctly shorter than basal article, bearing numerous plumose setae of variable lengths on all margins and ventral face.
Maxillule (first maxilla) (Figure 4C) with both endites strongly curved toward mouth; coxal endite semitriangular, bearing long, dense, stiff setae; basial endite more rounded dorsally and distally, armed with row of stiff setae (shorter than those of coxal endite) and 3 rows of spines along mesial margin, each successive row becoming more regularly spaced, bearing spines in greater number and size; lateral margin bearing row of long plumose setae; palp weakly curved, slightly bilobed distally with proximal lobe bearing long plumose seta, distal lobe bearing short plumose seta.
Maxilla (second maxilla) (Figure 4D) with densely setose mesial endites; proximal endite curved, straplike lobe; distal endite composed of two lobes, separated from each other by a deep notch and suture; flanked by dorsoventrally compressed tapering palp; scaphognathite enormously expanded, numerous plumose-setae like structures conspicuous on dorsal and ventral surfaces and lateral and mesial margins, supporting dense coverage of numerous filamentous bacteria-like structures (Figure 5); posterior lobe elongate, subtriangular, convex mesial margin distinctly notched, distomesial margin fringed with very long, wiry, tangled setae, preceded by much shorter plumose setae along proximomesial margin.

Fig. 5. Rimicaris hybisae sp. nov., male, from the Beebe Vent Field, Mid-Cayman Spreading Centre. Scanning electron micrographs of: (A) plumose-setae like structures, margin of maxilla; (B) plumose-setae like structures, ventral surface of maxilla; (C) close-up of filamentous bacteria-like structures, maxilla; (D) carpal brush on distal segment of carpus, first pereopod; (E) close-up of carpal brush setae and bacteria-like structures; (F) close-up of dorsal surface of carpal brush seta and setules with filamentous and non-filamentous bacteria-like structures. Scale bars: A = 300 µm; B, D = 200 µm; C = 50 µm; E = 20 µm; F = 2 µm. 227 × 296 mm (150 × 150 DPI).
First maxilliped (Figure 4E, F) with irregularly fusiform, heavily setose mesial endite; palp concealed by caridean lobe, short and bilobed, proximal lobe straplike bearing plumose setae along margins, distal lobe triangular, without ornamentation; caridean lobe broad, similar in shape and ornamentation to scaphognathite, lacking flagellum; large epipod subrectangular, curving dorsally, scattered setae on distolateral margin only.
Second maxilliped (Figure 4G) composed of six segments as in other Alvinocarididae; coxa expanded mesially, bearing numerous plumose setae on mesial margin; merus and ischium-basis fused segments moderately stout with numerous plumose setae on curved lateral surfaces, row of dorsally curved setae on nearly straight mesial surface; articulation between merus and carpus oblique; carpus short, long plumose setae on outer face; propodus with moderately long plumose setae mesially; articulation between propodus and dactylus oblique; dactylus longer than propodus, tapering to blunt distal margin, bearing very dense patch of short setae on mesial to distal margins forming brush-like structure; triangular epipod with slender rudimentary protobranch overreaching distal margin of epipod.
Third maxilliped (Figure 4H) overreaching antennal peduncle by one-third of ultimate segment, comprising coxa and three long segments; coxa heavily setose, with large, bilobed epipod; epipod without strap-like process; antepenultimate segment longest (consisting of fused basis–ischium–merus, but fusion between basis and ischium incomplete with partial suture on dorsal surface and corresponding indentations on lateral and mesial margins), dorsoventrally flattened, sinuously curved in dorsal view, armed with 1–2 spines at distolateral ventral angle, bearing numerous plumose setae on margins, dense cluster of long setulose setae on low elevation at proximomesial section, elevation length one-fifth of segment length, mesial margin notched where elevation terminates; penultimate segment with plumose setae on dorsolateral surfaces, rows of very dense, short, stiff setae on ventromesial face distal to one-third length; ultimate segment strongly curved, stout, about 1.5 times longer than penultimate segment, tapering distally to truncate tip bearing several terminal spines (7 on holotype female), trigonal in cross-section, lateral surface longitudinally carinate, with row of stiff setae, ventromesial surface flat with rows of very dense, short, stiff setae.
Branchial formula identical to that described in Rimicaris and Chorocaris (Williams & Rona, Reference Williams and Rona1986; Williams, Reference Williams1988; Martin & Hessler, Reference Martin and Hessler1990; Komai & Segonzac, Reference Komai and Segonzac2008).
Pereopods (Figure 6) without epipods. First pereopod typical of family, polymorphic, smaller than succeeding pereopods, typically folded upon itself at mero-carpal joint, reaching tip of antennal peduncle when extended; carpus distal margin obliquely truncate for accommodation of proximal part of palm, mesial face bearing grooming apparatus (carpal brush sensu Martin et al., Reference Martin, Signorovich and Patel1998: figures 1–3) comprised a triangular patch of serrate setae (some setules also serrate) arising from a recessed area, a row of distally-orientated serrate setae just proximal to the setal patch, and a stout spine proximal to the row of setae (Figure 5D); carpal brush setae bearing numerous filamentous and non-filamentous bacteria-like structures (Figure 5E, F); dactyl slightly overreaching fixed finger.

Fig. 6. Rimicaris hybisae sp. nov.: (A, B & D–K) holotype, female (carapace length (CL) 15.3 mm), [NHMUK2011.8054], from the Beebe Vent Field, Mid-Cayman Spreading Centre (MCSC); (C) paratype, male (CL 5.1 mm), [NHMUK2011.808066], from the Von Damm Vent Field (VDVF), MCSC; (L–M) paratype, male (CL 9.4 mm), [NHMUK2011.8055], from VDVF, MCSC; (A) right first pereopod, lateral view; (B) chela and carpus of right first pereopod, mesial view; (C) chela of left first pereopod, inner view; (D) right second pereopod, lateral view; (E) chela of right second pereopod, mesial view; (F) right third pereopod, lateral view; (G) dactylus and distal part of propodus of right third pereopod, lateral view; (H) dactylus of third right pereopod, posterior view; (I) right fourth pereopod; (J) right fifth pereopod; (K) endopod of right first pleopod, female, ventral view; (L) endopd of left first pleopod, male, ventral view; (M) appendix masculina and appendix interna, lateral view. Scale bars =1 mm. 250 × 359 mm (150 × 150 DPI).
Second pereopod more slender than first, slightly twisted, just overreaching tip of antennal peduncle if extended; merus and ischium unarmed; carpus and chela much weaker than those of first pereopod.
Third to fifth pereopods similar in structure, moderately stout, normally folded at mero-carpal articulation, meri and carpi somewhat twisted, meri and ischia unarmed, decreasing in length from third to fifth (e.g. 20.5–18.0–17.1 mm respectively in holotype female); carpi 1.1–1.2 length of propodi in third pereopod, 0.9–1.1 in second, 0.7–0.9 in third; propodi increasing in length from third to fifth, with irregular double (more or less) row of spinules on ventral surface and normally 4 spinules on ventrodistal margin; dactyli subconical, 0.2 length of propodi, each terminating in robust, curved, corneous unguis, flexor surface bearing marginal and plantar rows of distally curved, corneous spinules, increasing in strength distally.
First pleopod bearing sexually dimorphic endopod; in female, endopod (Figure 6K) simple, terminating in blunt apex; in male, endopod (Figure 6L) with asymmetrical distal notch separating much produced mesial lobe from smaller distolateral lobe. Second pleopod with slender appendix interna without cincinnuli; appendix masculina (Figure 6M) (males) tapering distally, bearing 7–8 spiniform setae distally. Third and fourth pleopods each with slender appendix interna, third without cincinnuli, fourth bearing a few tiny cincinnuli at tip. Fifth pereopod with stout, more robust appendix interna with many cincinnuli in subapical mesial cluster.
COLORATION
In life, carapace mostly pale translucent white; internal tissues neutral to greyish; integument of juveniles more translucent, oily orange globules visible underneath, tissues more orange.
Specimens from the BVF with rust-coloured deposits, most notably under antero-lateral area of carapace, on ventral surfaces of thorax, abdomen, frontal region, all areas of maxilla and first maxilliped; blackening common under antero-dorsal area of carapace (Figure 1), on tips of dactyli, third maxilliped and carpal brush; black sparkly particles clustered between mouthparts. Specimens from the VDVF are all ‘clean’ in appearance, lacking the rust-coloured deposits, blackened areas and black particulate matter observed on specimens collected from the BVF.
Eyes lacking coloration and pigment; juveniles brown pigmentation present in proximal area of fused eyes; orange tint in smallest juveniles (CL 3.6–4.2 mm).
Four-lobed dorsal organ (Figure 1) highly reflective with a slight pink tint; reflective property and coloration not preserved in death or after chemical fixation; white pigmentation visible in specimens preserved in 100% ethanol; brown pigmentation under carapace in juveniles (where dorsal organ is located in adults).
Gills typically bright white.
Third to fifth pereopods each ending in pale brown dactylus visible in preservation.
VARIATION
Specimens from both vent fields exhibit similar morphology. Variation of some features within and between specimens, most notably the posteroventral angle and armature of fourth and fifth pleonal pleura (Table 2), the number of dorsolateral spines on the telson and distolateral spines on the exopod of the uropod (noted above). Slight intra- and inter-specimen variation also observed in the degree of extension of the lateral lobe of the endopod of the first pleopod in males and the shape and reach of the distolateral tooth on the first antennal peduncle (noted above). Variability in these features does not appear to occur in any consistent combination, or in relation to sex or size.
The chela of the first pereopod appears to be polymorphic. In 16 specimens the chela of the first pereopod is slightly recurved, slender and somewhat delicate (Figure 6B). In contrast, one male (CL 5.1 mm), [NHMUK 2011.8066], BVF, has a stouter, more robust chela (Figure 6C).
No variation was observed between the female with a visible mature ovary (CL 7.0 mm) [NHMUK2011.8062] and other females.
One male specimen (CL 9.4 mm), [NHMUK 2011.8055] (Figure 7), from the VDVF has an acutely pointed rostrum, antennal lobe and pterygostomial expansion. All other specimens have a rounded or blunt antennal lobe and pterygostomial expansion with a rounded rostrum.
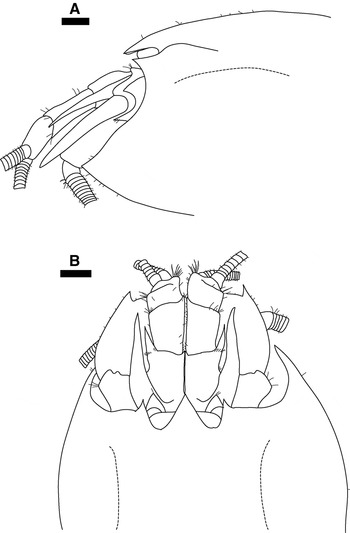
Fig. 7. Rimicaris hybisae sp. nov., paratype, male (carapace length 9.4 mm), [NHMUK 2011.8055], from the Von Damm Vent Field, Mid-Cayman Spreading Centre: (A) Carapace and cephalic appendages, lateral view; (B) carapace and cephalic appendages, dorsal view. Scale bars = 1 mm. 129 × 197 mm (150 × 150 DPI).
In juveniles (Table 2), carapace less inflated; rostrum distinctly pointed downwards, lateral and dorsal surfaces convex, anterior margin ornamented with small, distally-projecting setae; smallest juveniles (CL 3.6–4.2 mm) [NHMUK 2011.8067, NHMUK 2011.8068, NHMUK 2011.8070] medially-fused eyes with tiny tubercle on anterior surface medially.
Variation in coloration noted above.
COMPARATIVE REMARKS
The present new species has been mentioned previously in the literature as ‘a new morphospecies of alvinocaridid shrimp' and ‘the MCSC vent shrimp' (Connelly et al., in press) (Figures 4 & 5A). It is morphologically most similar to Rimicaris exoculata, R. kairei and Chorocaris chacei because of the reduction of the rostrum to a broadly rounded lobe and nonacuminate antennal lobe of the carapace, the presence of plumose-seta like structures on the dorsal and ventral surfaces of the scaphognathite of the maxilla and caridean lobe of the first maxilliped, and an appendix masculina armed with distal setae only. The new species is easily differentiated from C. chacei by the more inflated anterolateral region of the carapace, possession of a four-lobed dorsal organ, and acute tip of the uropodal protopod. A four-lobed dorsal organ and inflated carapace are known only for R. hybisae sp. nov. and the two other known Rimicaris species. The new species, however, is the first example within the genus to possess paired ‘pores' on the posterior lobes of the dorsal organ. Furthermore, the carapaces of the new species and R. exoculata are ornamented with setae, whereas there are no setae on the carapace of R. kairei. The carapace of R. hybisae sp. nov. is slightly less inflated than that of R. exoculata and R. kairei. Of the three known Rimicaris species, the carapace is most strongly inflated in R. kairei. Other differentiating characters between R. hybisae sp. nov. and the two other Rimicaris species include the structure of the antennae and extent to which the eyes are fused and rostrum reduced. The characters differentiating between the new species and its closest relatives are discussed below.
DISTRIBUTION AND HABITAT
Known only from the type locality, the Von Damm (2300 m) and Beebe (4960 m) hydrothermal vent fields, Mid-Cayman Spreading Centre, Caribbean Sea. For preliminary descriptions of both vent fields, Connelly et al. (in press). Observed at the BVF in dense aggregations on the vent chimneys (>2000 individuals m−2, Connelly et al., in press), and with high abundances of anemones around crevices issuing visible diffuse flow in the central area of the sulphide mound (Connelly et al., in press). At the VDVF, in dense aggregations around actively venting orifices of the edifice peak (>2000 individuals m−2, Connelly et al., in press), along with another, numerically subordinate, morphotype of shrimp.
ETYMOLOGY
From the name of the British manoueverable TV grab HyBIS, in celebration of her first, and highly successful, scientific mission. The gender is feminine.
MOLECULAR PHYLOGENY
Partial sequences of the mitochondrial COI (460 bp) and 16S (549 bp) regions and the nuclear 18S (576 bp) region of Rimicaris hybisae sp. nov. were consistent among specimens from both sites. Unique and fixed mutations were observed in the partial sequences of the COI and 18S regions. Based on NJ and ML phylogenetic analyses for COI sequences available in GenBank, R. hybisae sp. nov. exhibits the smallest evolutionary distance to Alvinocaris sp. [AY163260.1], with a 1.96% divergence from this species, compared with a 7.39% divergence between R. hybisae sp. nov. and both R. exoculata and Chorocaris chacei. In the 16S region, no substitution was found between R. hybisae sp. nov. and C. chacei (0% divergence) based on the 300-bp sequence in GenBank for the latter species, whereas R. hybisae sp. nov. exhibits 0.47% divergence (424 bp) with R. exoculata. Phylogenetic analyses on the 300-bp region common among the species shows high bootstrap values (100% and 95% for NJ and ML methods respectively) separating the R. exoculata–R. hybisae sp. nov.–C. chacei clade from the other alvinocaridid species. Within this clade R. hybisae sp. nov. and C. chacei are separated from R. exoculata (88% bootstrap support, NJ and ML methods).
In GenBank, partial sequences of the 18S region for alvinocaridids are limited to Rimicaris exoculata, Chorocaris chacei, Alvinocaris muricola Williams, Reference Williams1988 and Alvinocaris longirostris Kikuchi & Ohta, Reference Kikuchi and Ohta1995. Because there is only a single partial sequence in the database for both R. exoculata and C. chacei, it is not known if the mutations observed in the 18S partial sequences of these species are fixed within each of these species. Based on a 540-bp alignment, NJ and ML phylogenetic trees place R. hybisae sp. nov. in the same clade as R. exoculata and C. chacei (88% and 90% for NJ and ML methods respectively) (Figure 8). For the available 18S partial sequences the new species is closest in evolutionary distance to C. chacei (0.56% and 0.88% divergence with C. chacei and R. exoculata respectively).

Fig. 8. Neighbour-joining tree of the Alvinocarididae based on a 540-base pair alignment of partial nucleotide sequences from the nuclear 18S ribosomal RNA region with Euganatonotus chacei Chan & Yu, Reference Chan and Yu1991 (Nematocarcinidae) as an outgroup. Evolutionary distances computed using the Jukes–Cantor method (Jukes & Cantor, Reference Jukes, Cantor and Munro1969) are represented by branch length; scale bar is proportional to inferred nucleotide divergence. Bootstrap support calculated on 100 re-sampling replicates is shown by the numbers along the branches (neighbour joining, roman text; maximum-likelihood, italic text). GenBank accession numbers are given after species names.
DISCUSSION
The presence of unique and fixed mutations in the partial sequences of the COI and 18S region suggest that Rimicaris hybisae sp. nov. is genetically distinct from all other species in the GenBank database. This supports the morphological evidence that R. hybisae is a new species. Although morphological variation was present amongst the specimens studied, partial sequences of the COI, 16S and 18S regions were consistent between specimens, confirming that those analysed from both MCSC vent fields are monospecific.
Based on morphology, and supported by the results from the molecular analyses, Rimicaris hybisae sp. nov. belongs within the Rimicaris–Chorocaris–Opaepele clade. Common features within this complex include a greatly reduced rostrum, broadly fused eyes, three or greater rows of accessory spines on the ventral surfaces of the dactyli of the third to fifth pereopods, the unarmed ischium of the third to fifth pereopods, and sinuous rows of dorsolateral spines on the telson (Komai & Segonzac, Reference Komai and Segonzac2005, Reference Komai and Segonzac2008). Rimicaris hybisae sp. nov. differs from Opaepele in possessing a more reduced rostrum, inflated carapace and non-acuminate antennal and pterygostomial lobes (Lunina & Vereshchaka, Reference Lunina and Vereshchaka2010). The assignment of the new species to a genus was a complex issue. Many morphological characteristics of Chorocaris are shared with Rimicaris (see Martin & Hessler, Reference Martin and Hessler1990; Komai & Segonzac, Reference Komai and Segonzac2008), as exemplified by the original assignment of C. chacei to the genus Rimicaris by Williams & Rona (Reference Williams and Rona1986). However, a suite of morphological traits distinguish the two genera.
Two of the most striking morphological features of Rimicaris hybisae sp. nov. are its inflated carapace and four-lobed dorsal organ. These features are, to date, unique to Rimicaris and support placement of the new species within the genus. The carapace is marginally less inflated than that of R. exoculata (which is less inflated than that of R. kairei Watabe & Hashimoto, Reference Watabe and Hashimoto2002 (authors, personal observation)). Rimicaris hybisae sp. nov. can be further distinguished from R. exoculata and R. kairei by the presence of paired ‘pores' on the posterior lobes of the dorsal organ. Variability has been documented in the shape of the dorsal organ in adult specimens of R. exoculata (O'Neill et al., Reference O'Neill, Jinks, Herzog, Batelle, Kass, Renninger and Chamberlain1995: Figure 5), but the presence of ‘pores' is, to our knowledge, a feature unique to R. hybisae sp. nov. The ‘dorsal eye' of R. exoculata (and presumably R. kairei) is an extremely efficient photoreceptor, used for detecting light emitted from the vents (Pelli & Chamberlain, Reference Peli and Chamberlain1989; Van Dover et al., Reference Van Dover, Cann, Cavanaugh, Chamberlain, Delaney, Janecky, Imhoff and Tyson1994, Reference Van Dover, Reynolds, Chave and Tyson1996; O'Neill et al., Reference O'Neill, Jinks, Herzog, Batelle, Kass, Renninger and Chamberlain1995). Shiny anterior spot-like organs have been described inside the carapaces of Chorocaris chacei, Mirocaris fortunata Martin & Christiansen, Reference Martin and Christiansen1995, Alvinocaridinides formosa Komai & Chan, Reference Komai and Chan2010, and species of Opaepele and Nautilocaris Komai & Segonzac, Reference Komai and Segonzac2004 (Desbruyères et al., Reference Desbruyères, Segonzac and Bright2006; Tsuchida et al., Reference Tsuchida, Yamaguchi, Komai, Watanabe, Fujikura, Okutani and Maruyama2008; Komai & Chan, Reference Komai and Chan2010). These spot-like organs may be homologous to the ‘dorsal eye' found in species of Rimicaris (Kuenzler et al., Reference Kuenzler, Kwasniewski, Jinks, Lakin, Battelle, Herzog, Renninger and Chamberlain1997; Lakin et al., Reference Lakin, Jinks, Batelle, Herzog, Kass, Renninger and Chamberlain1997; Komai & Chan, Reference Komai and Chan2010), but are smaller and do not comprise four lobes. Histological examination of the dorsal organ, requiring the collection of further specimens, would be necessary to determine if a dorsal photoreceptor has coevolved in R. hybisae sp. nov.
Martin & Hessler (Reference Martin and Hessler1990) hypothesized that Rimicaris, with its inflated carapace, opercular frontal region, dorsal organ, and dramatically reduced rostrum, is a derived genus that stemmed from Chorocaris or another morphologically similar deep-sea shrimp. The presence of an inflated carapace and four-lobed dorsal organ in R. hybisae sp. nov. suggest that this species may be more derived than species of Chorocaris. In contrast, the armature of the fourth and fifth pleonal pleura is a conservative feature, also found in more conservative genera (Opaepele, Alvinocaris and Shinkaicaris: Komai & Segonzac, Reference Komai and Segonzac2005; Komai et al., Reference Komai, Giere and Segonzac2007). Within Rimicaris and Chorocaris, the fourth and fifth pleonal pleura are subacutely or acutely pointed only at their posteroventral angles and are not marginally armed (Komai & Segonzac, Reference Komai and Segonzac2008).
Based on analyses of the COI gene (600 bp), Shank et al. (Reference Shank, Black, Halanych, Lutz and Vrijenhoek1999) proposed that Chorocaris is a paraphyletic assemblage with C. chacei being more closely related to Rimicaris exoculata. This is supported by the most recent and complete molecular phylogeny of the Alvinocarididae based on COI (600 bp), whereby C. chacei clusters with R. exoculata with strong statistical support (100% bootstrap values: Zelnio & Hourdez, Reference Zelnio and Hourdez2009). In R. hybisae sp. nov., Rimicaris species and C. chacei, the scaphognathite of the maxilla and caridean lobe of the first maxilliped are ornamented with numerous plumose-seta like structures on both their dorsal and ventral surfaces, whilst in C. vandoverae Martin & Hessler, Reference Martin and Hessler1990 and C. paulexa Martin & Shank, Reference Martin and Shank2005, their ventral surfaces are nearly naked (Martin & Hessler, Reference Martin and Hessler1990; Martin & Shank, Reference Martin and Shank2005; Komai & Segonzac, Reference Komai and Segonzac2008). The appendix masculina of R. hybisae sp. nov., C. chacei and the Rimicaris species bear distal setae only, whereas setae extend onto the dorsal surface of the appendix masculina in C. vandoverae. Komai & Segonzac (Reference Komai and Segonzac2008) suggest that this feature supports the close proximity between C. chacei and Rimicaris species and also consider C. chacei as a possible sister species of the Rimicaris clade. Rimicaris hybisae sp. nov., with shared morphological affinities with both C. chacei and Rimicaris, further supports the proximity between these taxa, although the more conservative features of the new species are not shared with either genus.
Features common to Chorocaris and absent in Rimicaris exoculata and R. karei include: possession of a strong distolateral tooth, a small distomesial tooth, and a prominent proximolateral tubercle on the first segment of the antennal peduncle; a clear separation of the stylocerite from the antennal peduncle; a carpal brush on the first pereopod; the armed antepenultimate segment of the third maxilliped; blunt or subacute antennal teeth; an increase in length from the third to fifth pereopods and a well-developed, functional appendix interna bearing cincinnuli on the fifth pleopod only (Martin & Hessler, Reference Martin and Hessler1990; Komai & Segonzac, Reference Komai and Segonzac2008; authors, personal observation). These traits are also exhibited by R. hybisae sp. nov., revealing some morphological affinities with the genus Chorocaris.
Notable autapomorphies of Rimicaris are: the complete fusion of the eyes into a transverse ocular plate; the reduction of the rostrum to a broadly rounded lobe, fitting closely to the posterior concavity of the ocular plate; the formation of the antennal components into an operculum-like structure and the presence of a mat of dense spinules on the flexor surface of the propodi of the third to fifth pereopods (Martin & Hessler, Reference Martin and Hessler1990; Komai & Segonzac, Reference Komai and Segonzac2008). These features were not observed in R. hybisae sp. nov., despite the examination of specimens comparable in size to adult R. exoculata and R. kairei.
In consideration of the morphological and molecular evidence presented here, it appears that Rimicaris hybisae sp. nov. may be intermediate between Chorocaris chacei and R. exoculata. Given the uncertainty concerning the Chorocaris genus, the establishment of a new genus for R. hybisae sp. nov. is premature. In reverence to the fact that C. chacei was originally Rimicaris chacei, the assignment of R. hybisae sp. nov. to the genus Rimicaris is the most conservative approach available. As Komai & Segonzac (Reference Komai and Segonzac2008) have already suggested, a comprehensive and extensive molecular phylogenetic analysis of the whole Rimicaris–Chorocaris–Opaepele clade is required to clarify the relationships between these taxa. Such a study has the potential to improve markedly our current understanding of the evolution, radiation and biogeographical patterns of these shrimp among deep-sea chemosynthetic environments in the world's oceans.
Morphological variation is well documented in the Alvinocarididae and is acknowledged as common within alvinocaridid species (e.g. Kikuchi & Ohta, Reference Kikuchi and Ohta1995; Martin & Shank, Reference Martin and Shank2005; Komai & Segonzac, Reference Komai and Segonzac2008). The variation described between specimens of Rimicaris hybisae sp. nov. does not appear to be related to size, gender, or collection site. Polymorphism of the chela of the first pereopod has also been described for species of Chorocaris (Martin & Shank, Reference Martin and Shank2005; Komai & Segonzac, Reference Komai and Segonzac2008). Specimens of C. chacei from Lucky Strike (one female in Komai & Segonzac, Reference Komai, Segonzac, Desbruyères, Segonzac and Bright2006: Plate 3, p. 241; one male in Komai & Segonzac, Reference Komai and Segonzac2008: figure 9F, G) have been shown with an acutely pointed rostrum, antennal lobe and pterygostomial expansion; these specimens were considered by Komai & Segonzac (Reference Komai and Segonzac2008) to be an aberrant form of the species, and although this identification may not be fully justified, it is reasonable given the sampling location. Consequently the specimen illustrated in Figure 7 [NHMUK 2011.8055] is interpreted here as an aberrant form of R. hybisae sp. nov. The ‘clean' appearance of specimens from the VDVF and presence of black and rust-coloured deposits on specimens from the BVF may be due to the differences in the chemistry of their respective habitats (Connelly et al., in press).
Results from analysis of 460 bp of the COI region for Rimicaris hybisae sp. nov. suggest that for this gene, the new species is genetically closest to Alvinocaris sp. [AY163260.1] (Alvinocaris methanophila Komai, Shank & Van Dover, Reference Komai, Shank and Van Dover2005 from Blake Ridge; see Van Dover et al., Reference Van Dover, Aharon, Bernhard, Caylor, Doerries, Flickinger, Gilhooly, Goffredi, Knick, Macko, Rapoport, Raulfs, Ruppel, Salerno, Seitz, Sen Gupta, Shank, Turnipseed and Vrijenhoek2003). This result does not fit well with the evidence from morphology, 16S and 18S, thus highlighting the potential problem of relying on COI for genetic barcoding. While it is possible that there is a problem with the COI sequence for Alvinocaris sp., a second possibility is that there is more than one gene in the COI region. Work is in progress to test the second hypothesis (Plouviez et al., unpublished data). In the 16S region, R. hybisae sp. nov. exhibits fixed mutations that are identical to those observed in Chorocaris chacei. However, it was not possible to establish if these species share an identical nucleotide sequence for more than 300-bp of the 16S region. Results from analysis of the 18S region suggest that, for this slow-evolving gene, R. hybisae sp. nov. is closest in evolutionary distance to C. chacei, although this is based on a 576-bp alignment only.
Rimicaris hybisae sp. nov. is easily differentiated from Chorocaris chacei by the more inflated anterolateral region of the carapace, possession of a four-lobed dorsal organ, and acute tip of the uropodal protopod. Like juveniles of C. chacei, juveniles of R. hybisae sp. nov. bear plumose setae on the posterior margin of the telson, a conspicuous distolateral tooth on the first segment of the antennal peduncle and a carpal brush on the first pereopod. In a recent review, Komai & Segonzac (Reference Komai and Segonzac2008) noted differences in the morphology of ovigerous females in species of Chorocaris and Rimicaris. Samples of ovigerous females of R. hybisae sp. nov. are required to enable their morphology to be described and compared to other species.
In summary, morphological features and molecular analyses indicate that Rimicaris hybisae sp. nov. belongs within the Rimicaris–Chorocaris–Opaepele clade, closest to and intermediate between C. chacei and R. exoculata. The inflated carapace and four-lobed dorsal organ of R. hybisae sp. nov. are diagnostic and distinguishing morphological features, previously recorded only in R. exoculata and R. kairei, which support placement of the new species within the genus Rimicaris. The molecular evidence reported here confirms that R. hybisae sp. nov. is a genetically distinct species, closest to C. chacei, which was originally placed in the genus Rimicaris as R. chacei (Williams & Rona, Reference Williams and Rona1986). Phylogenies of the Alvinocarididae suggest that Chorocaris is polyphyletic, with C. chacei more closely related to Rimicaris than to other Chorocaris species (Shank et al., Reference Shank, Black, Halanych, Lutz and Vrijenhoek1999; Zelnio & Hourdez, Reference Zelnio and Hourdez2009). Moreover, on the basis of morphological traits shared between the two taxa, it has been proposed that C. chacei is a sister species of Rimicaris (Komai & Segonzac, Reference Komai and Segonzac2008). The most conservative approach is therefore to expand the diagnosis of Rimicaris to incorporate R. hybisae sp. nov., rather than to erect a new genus in the absence of highly corroborated phylogeny. The presence of features previously considered diagnostic of Rimicaris spp. and C. chacei in R. hybisae sp. nov., and the low genetic divergence between these taxa suggest that reassimilation of C. chacei within Rimicaris could also be considered in the future.
BIOGEOGRAPHY
Rimicaris hybisae sp. nov. represents the third named species in the genus, all of which are known only from hydrothermal vents in a particular area (Table 1).
Rimicaris karei is so far restricted to its type locality in the Indian Ocean, whereas R. exoculata is present at vents along the MAR, where it occurs sympatrically with Chorocaris chacei (Table 1). Rimicaris hybisae sp. nov. extends the distribution of the genus approximately 4000 km westwards into the Caribbean and increases the bathymetric range of the Alvinocarididae by 872 m to 4960 m. This may also be the first record of the Alvinocarididae in the Caribbean. Escobar-Briones & Villalobos Hiriart (Reference Escobar-Briones and Villalobos Hiriart2003) reported an indeterminate species of Alvinocaris from non-chemosynthetic environments on the Banco Chinchorro, northern Caribbean, at depths of 176–203 m but provided no molecular evidence or catalogue details.
Before the complete closure of the Isthmus of Panama by 3.1 Ma (Burton et al., Reference Burton, Ling and O'Nions1997), a deep-water connection existed between the eastern Pacific and Caribbean. Martin & Hessler (Reference Martin and Hessler1990) proposed that the presence of Chorocaris species in the Atlantic and Pacific indicates a faunal connection between the eastern Pacific and Mid-Atlantic vents. However, no specimens of Rimicaris have been collected from the Pacific Ocean, despite numerous active surveys at Pacific vents. In addition, Chorocaris may be paraphyletic, with the Atlantic species (C. chacei) belonging to Rimicaris. The recent discovery of hydrothermal vents and chemosynthetic communities on the MCSC provides an opportunity to test Martin & Hessler's (1990) hypothesis. The presence of shrimp-dominated communities at the BVF and the VDVF also indicate that the MCSC vent fauna shares similarities with MAR vent fauna. The descriptions of other novel taxa at MCSC vents (Nye et al., unpublished data) will further elucidate the factors determining vent biogeography of this region.
ACKNOWLEDGEMENTS
The authors thank the Master and ship's company of the RRS ‘James Cook', the UK National Marine Facilities staff, and fellow scientists on the 44th voyage of RRS ‘James Cook'. Professor P.A. Tyler and Professor C.L. Van Dover provided laboratory facilities at the University of Southampton and Duke University Marine Laboratory respectively. Dr A. Page provided scanning electron microscopy facilities at the Biomedical Imaging Unit (University of Southampton). Dr T. Horton (National Oceanography Centre Southampton) provided equipment and advice. Comparative material was obtained on loan from the Muséum National d'Histoire Naturelle (MNHN) collection, Paris and the National Museum of Natural History, Smithsonian (USNM) collection, Washington, DC. This work benefitted from two anonymous referees who gave valuable comments for the improvement of this paper. This work is supported by a UK NERC award (NE/F017774/1) to J.C. and NASA ASTEP Grant (NNX09AB75G) to Cindy Van Dover, which is gratefully acknowledged.