Introduction
Sponges belonging to the genus Timea Gray, Reference Gray1867 (Tethyida: Timeidae) are usually small and thinly encrusting species living in marine cryptic habitats. They are characterized by megascleres that are styles, with transitions to tylo- and subtylostyles, and microscleres that are euasters (Rützler, Reference Rützler, Hooper and Van Soest2002). Originally, Timea was erected by Gray (Reference Gray1867) in the family Tethyidae, but after the Systema Porifera revision (Hooper & Van Soest, Reference Hooper and Van Soest2002), it was the only genus that remained in the family Timeidae, belonging to the order Hadromerida (see Rützler, Reference Rützler, Hooper and Van Soest2002). However, in the most current sponge systematic restructuring, the order Hadromerida was dissolved, and the family Timeidae was relocated in the order Tethyida containing two more families, Tethyidae and Hemiasterellidae (Morrow & Cárdenas, Reference Morrow and Cárdenas2015).
Currently, Timea contains about 57 valid species distributed around the world (Van Soest et al., Reference Van Soest, Boury-Esnault, Hooper, Rützler, de Voogd, Alvarez, Hajdu, Pisera, Manconi, Schönberg, Klautau, Kelly, Vacelet, Dohrmann, Díaz, Cárdenas, Carballo, Ríos, Downey and Morrow2019). This number could be an underestimation, owing to the fact that cryptic habitats of the species are underexplored and that there is the potential of cryptic species complexes, such as Timea unistellata (Topsent, Reference Topsent1892) which is recorded with extremely wide distribution. The morphological taxonomy of Timea is mainly based on the size and shape of euasters (including small details in ornamentation). However, it is not easy to determine whether the degree of euaster morphological variation is enough to discriminate between species, or if it is the result of a high intraspecific phenotypic plasticity (Carballo & Cruz-Barraza, Reference Carballo and Cruz-Barraza2006). In most cases, the simplicity of skeletal structure does not provide enough diagnostic characters for species discrimination, but some external characteristics, such as colour and surface ornamentation, may contribute suitable information for separating species.
In the last years, molecular markers have significantly contributed to sponge systematics (see Morrow & Cárdenas, Reference Morrow and Cárdenas2015) and taxonomy (e.g. Blanquer & Uriz, Reference Blanquer and Uriz2008; Cruz-Barraza et al., Reference Cruz-Barraza, Vega, Ávila and Vazquez-Maldonado2017; among others). However, to date, there is only a small number of sequences for Timea species, making it difficult to understand its diversity and phylogenetic relationships (see Kober & Nichols, Reference Kober and Nichols2007; Redmond et al., Reference Redmond, Morrow, Thacker, Diaz, Boury-Esnault, Cárdenas, Hajdu, Lôbo-Hajdu, Picton and Collins2013; Lim et al., Reference Lim, Wiklund, Glover, Dahlgren and Tana2017).
Currently, 21 species of Timea are known from the Western Atlantic (Macola & Menegola, Reference Macola and Menegola2018; see discussion), of which only one species has been recorded in the Gulf of Mexico (Van Soest et al., Reference Van Soest, Boury-Esnault, Hooper, Rützler, de Voogd, Alvarez, Hajdu, Pisera, Manconi, Schönberg, Klautau, Kelly, Vacelet, Dohrmann, Díaz, Cárdenas, Carballo, Ríos, Downey and Morrow2019) (USA coast). Here we report the first records of the genus Timea from the Mexican coast of the Gulf of Mexico, including the description of a new species and the re-description of T. hechteli Lehnert & Heimler, Reference Lehnert and Heimler2001, based on old and new material. Additionally, we obtained partial sequences of mitochondrial COI mtDNA (‘DNA Barcoding’) and ITS's ribosomal region rDNA of these species, aimed to complement the morphological interpretation and taxonomic assignation of our species into Timea (integrative taxonomy). Also, we give information about their phylogenetic relationships and systematics position with respect to other Tethyida. Finally, we include a taxonomic key, following Lehnert & Heimler's (Reference Lehnert and Heimler2001) and Van Soest's (Reference Van Soest2009) efforts for the Central West Atlantic, which are based mainly on euaster morphology.
Materials and methods
Specimen collection and morphological analyses
Six Timea specimens were collected from shallow-water rocky-sedimentary substrates by snorkelling at two locations in Campeche State (south-eastern Gulf of Mexico) (Figure 1). Specimens and spicule slides analysed have been deposited in the ‘Colección de Esponjas’ (LEB-ICML-UNAM), of the Instituto de Ciencias del Mar y Limnología, UNAM, in Mazatlán (Mexico). The type material of T. hechteli is located in the Smithsonian's National Museum of Natural History (USNM).

Fig. 1. Sampling localities and distribution of Timea alcoladoi sp. nov. and T. hechteli along the coast of the Gulf of Mexico. The black circles show sample localities for this study, and the white circle shows the type locality of T. hechteli.
Collected specimens were labelled and small fragments of fresh samples were preserved in 100% ethanol for molecular analysis. Then, specimens were fixed in 4% formaldehyde for 24 h, and transferred to 70% ethanol for storage. External morphology and skeletal elements and their arrangement were recorded for each individual. Spicule preparation followed the techniques described by Carballo & Cruz-Barraza (Reference Carballo and Cruz-Barraza2008) for light and scanning electron microscopy (SEM).
Twenty-five or more spicules chosen at random were measured for each specimen studied. Megasclere measurements were taken to include total length widths of shaft and head; microsclere (euaster) measurements are of the total diameter. In each description and in the tables, the number in parentheses is the mean of all measurements. Sponge-specific terms are used according to Boury-Esnault & Rützler (Reference Boury-Esnault and Rützler1997), but also from the most current literature related to genus Timea.
DNA purification, amplification and sequencing
Total genomic DNA was extracted from four specimens of both species, following the steps described in detail in Cruz-Barraza et al. (Reference Cruz-Barraza, Vega, Ávila and Vazquez-Maldonado2017). Originally, we tried to obtain different loci fragments from mitochondrial and ribosomal DNA using several sets of primers, but after numerous assays, we only could amplify the COI standard barcoding fragment for the holotype of Timea alcoladoi sp. nov. and two specimens of T. hechteli, and the ITS1-5.8S-ITS2 rDNA for the holotype of Timea alcoladoi sp. nov., and three specimens of T. hechteli. For COI mtDNA we used primers PLAKLCOdegF (modified from Folmer et al., Reference Folmer, Black, Hoeh, Lutz and Vrijenhoek1994 by Cruz-Barraza et al., Reference Cruz-Barraza, Vega and Carballo2014): 5′-TCW ACD AAY CAT AAA GAY ATW GG-3′; and C1J2165-R (from Misof et al., Reference Misof, Erpenbeck and Sauer2000 modified as reverse): 5′-CCN GGT AAAATT AAAATA TAA ACT TC-3′. For the ITS1-5.8S-ITS2 gene ribosomal region, we used primers from White et al. (Reference White, Bruns, Lee, Taylor, Innis, Gelfand, Sninsky and White1990), universal primers ITS4 5′-TCC TCC GCT TAT TGA TAT GC-3′ and ITS5 5′- GGA AGT AAA AGT CGT AAC AAG G-3′. PCR reactions were carried out in a volume of 12.5 μl and consisted of 6.0 μl distilled H2O (sterile MilliQ), 0.75 μl deoxyribonucleotide triphosphates (0.2 mM), 0.75 μl MgCl2 (8 mM), 0.70 μl of each primer (10 μM), 2.50 μl 5× PCR buffer (Promega), 0.2 μl Taq DNA polymerase and 1 μl genomic DNA (c. 50– 100 ng). Thermal cycling conditions were: initial denaturation step at 94°C for 2 min, and 35 cycles of 94°C for 30 s, 50°C for 30 s, 72°C for 1 min, and a final extension of 72°C for 5 min. PCR-products were run in a 1.5% agarose gel to corroborate the positive amplification. Products were purified using the Wizard purification kit (Promega) and sequenced in both directions using Applied Biosystems 3730xl DNA analysers by Macrogen, Korea.
Sequence analyses
Sequences were edited with the program Codon Code Aligner 7.1.2 (CodonCode Corporation), and verified with the bioinformatics tool BLAST (Search National Centre for Biotechnology Information/Blast) to confirm the species identity. Phylogenetic reconstructions (COI mtDNA and ITS rDNA) were made separately and included all available sequences from order Tethyida in GenBank. For COI analysis, additional sequences from other Heteroscleromorpha groups were included, because two sequences of Timea (obtained from GenBank) showed a closer relationship with Poecilosclerida than Tethyida. Here, we used the freshwater sponge Racekiela montemflumina Carballo, Cruz-Barraza, Yáñez & Gómez, Reference Carballo, Cruz-Barraza, Yáñez and Gómez2018 to root the tree, because recent studies have suggested Haplosclerida as the sister group to the remaining Heteroscleromorpha (Lavrov et al., Reference Lavrov, Wang and Kelly2008; Morrow & Cárdenas, Reference Morrow and Cárdenas2015). For the ribosomal region, a few sequences of species of Order Clionaida were used as outgroups. Owing to the low number of available sequences of Timea, we included original sequences (COI and ITSs) of a specimen of Timea cf. authia from Eastern Pacific Ocean, only to strengthen the Timeidae clade. The specimen is characterized by small tylasters from 12.5–22.5 μm in diameter, and tylostyles from 345–750 μm length.
Sequences were initially aligned in Mega 7 (Kumar et al., Reference Kumar, Stecher and Tamura2016), using the CLUSTALW alignment under the default opening–gap extension parameters (15.0–6.66). For analysis of ribosomal region, we also used the program Gblocks 0.91b (Castresana, Reference Castresana2000) in order to determine and exclude ambiguously aligned regions under the following parameters: minimum number of sequences for a conserved position: seven; minimum number of sequences for a flanking position: 11; maximum number of contiguous non-conserved positions: eight; minimum length of a block: 10; allowed gap positions: with half; use similarity matrices: yes. For both loci, Bayesian inference analysis was performed with MrBayes 3.2.1 (Ronquist & Huelsenbeck, Reference Ronquist and Huelsenbeck2003) using the Hasegawa–Kishino–Yano plus Invariant sites plus Gamma distributed model of sequence evolution, which was selected through the JModelTest 2.0.1 program (Posada, Reference Posada2008) using the Bayesian information criterion. The program was run with four simultaneous Monte Carlo Markov chains for 10,000,000 generations, which were sampled every 200 trees with a burn-in of 20%. In addition, maximum likelihood (ML) analyses were generated with RAxML 8.1.11 (Stamatakis, Reference Stamatakis2014) on the CIPRES science gateway v.3.3 portal (http://www.phylo.org) (Miller et al., Reference Miller, Pfeiffer and Schwartz2010).
Sequences of the species presented here are available in the database of GenBank with the codes: Timea alcoladoi sp. nov. holotype LEB-ICML-UNAM-3140 (COI: MN562235, ITS: MN556959) and T. hechteli, specimens: LEB-ICML-UNAM-2232 (COI: MN562233, ITS: MN556962), LEB-ICML-UNAM-2573 (COI: MN562234), LEB-ICML-UNAM-2878 (ITS: MN556961) and LEB-ICML-UNAM-3131 (ITS: MN556963). Additional sequences of Timea cf. authia LEB-ICML-UNAM-1950 also are available (COI: MN562236, ITS: MN556960).
Results
SYSTEMATICS
Order TETHYIDA Morrow & Cárdenas, 2015
Family TIMEIDAE Topsent, Reference Topsent1928
Diagnosis: Tethyida presenting encrusting growth form with tylostyles arranged in tracts ending as bouquets, which generally pierce the surface (hispid). Tracts may be absent, in which case tylostyles are organized as bouquets. Additional tylostyles may occur in criss-cross fashion between the tracts or sparsely disposed tangentially to the surface or to the substrate. Microscleres are euasters dispersed in the choanosome, denser at the substrate and towards the surface, and forming a crust at the surface (Leite et al., Reference Leite, Fonseca, Leal and Hajdu2015).
Genus Timea Gray, Reference Gray1867
Diagnosis: Same as family.
Timea alcoladoi sp. nov.
(Figures 2 & 3)
ZooBank LSID for the new species: urn:lsid:zoobank.org:act:2D31E3DB-B780-4435-BFBD-0E67D4ED6F87
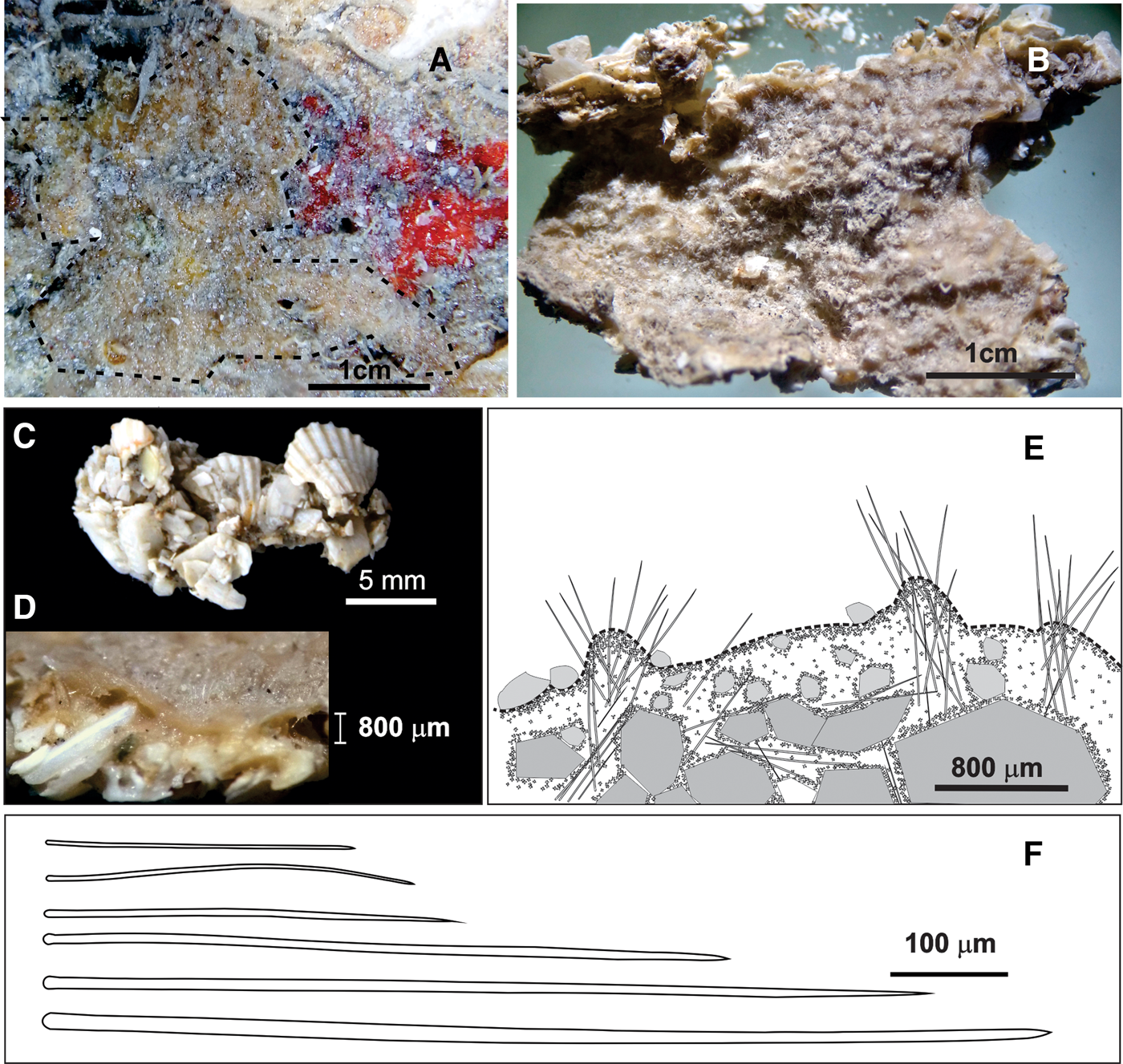
Fig. 2. External morphology and skeletal characteristics of Timea alcoladoi sp. nov.: (A, B) alive and preserved holotype (LEB-ICML-UNAM-3140); (C, D) View of the bases and cross-section of a fragment of holotype, showing the dense proportion of sand and shell fragments in the sponge's body; (E) Drawings of cross-section of the skeletal structure; (F) Drawings of spicule styles and subtylostyles morphologies.

Fig. 3. Scanning electron microscopy images of spicules of Timea alcoladoi sp. nov.: (A) head of the style subtylostyles; (B–D) spherostrongylasters; (B) typical with 6 rays; (C) with 7 rays; (D) with 4 rays.
MATERIAL EXAMINED
Holotype: LEB-ICML-UNAM-3140, Puerto Real, Isla del Carmen (Campeche, Mexico), 18°46′44.1″N 91°31′51″W, 11.4.2015, 0.5–1 m depth. Paratype: LEB-ICML-UNAM-3141, Puerto Real, Isla del Carmen (Campeche, Mexico), 18°46′44.1″N 91°31′51″W, 11.4.2015, 0.5–1 m depth.
MATERIAL EXAMINED FOR COMPARISON
Paratypes of Timea chiasterina Carballo & Cruz-Barraza, Reference Carballo and Cruz-Barraza2006, LEB-ICML-UNAM-244, Isla Tunosa (Topolobampo, Sinaloa, Mexico), 25°34′58″N 109°00′51″W, 22.6.2000, 1.5 m depth, under rocks. LEB-ICML-UNAM-1174 and ICML-UNAM-1175, Antiguo Corral del Risco (Punta Mita, Nayarit, Mexico), 20°46′20″N 105°32′49″W, 18.7.2005, 2 m depth, on rocks.
Diagnosis
Small and thinly encrusting orange sponge. Megascleres are styles straight or slightly curved, sometimes with very incipient heads ‘subtylostyles’ (from 185–1010 μm length). Microscleres are spherostrongylasters (type chiaster) (from 5–15 μm diameter), with a well-marked rounded centre and typically six rays (although spicules with four and seven rays also are present), which end in small bouquets of diverging conical spines.
Description
Thickly encrusting specimens, from 1–3.5 mm in thickness, covering a surface of 3 × 5 cm (holotype) and 2 × 3 cm (paratype) on rocks (Figure 2A). The specimens broke up into several fragments, 1–2 cm, owing to the high content of foreign material embedded in the choanosome (Figure 2B, C). The surface is slightly micro-conulose and hispid, with some small sub-ectosomal channels (<1 mm in diameter). Conules are 300–550 μm in diameter and are characterized by the tips of the choanosomal megasclere tracts protruding in bouquets, from 100–350 μm. The consistency is firm to hard but fragile and brittle. Colour in life is orange and light brown in alcohol (Figure 2A, B).
Skeleton
It is difficult to observe due to a dense concentration of foreign material (Figure 2C–E), but it is possible to distinguish a very thin sub-ectosomal layer from 0.3–1 mm in thickness (Figure 2D), with styles arranged in tracts (from 80–150 μm in diameter) ending as bouquets at the top of superficial conules, giving a hispid aspect to sponge surface. Megascleres are also present free or forming vague tracts between foreign material in the choanosome. Spherostrongylasters are distributed throughout the entire sponge body but more densely towards the surface and around the foreign material (Figure 2E).
Spicules
Megascleres are mostly styles with a straight to slightly curved or even flexuous shaft and a sharp point, although some of them show a small annular rim in the upper end, turning them to incipient subtylostyles (Figures 2F & 3A, Table 1). Length: 185–(571)–1010 μm; shaft diameter: 4–(10)–20 μm. Microscleres are small strongylasters (type chiaster), very homogenous in size. They are characterized by a spherical, well-marked centrum ‘spherostrongylaster’ commonly with six rays (Figure 3B), although there also are with seven (Figure 3C) and four (Figure 3D) rays, in less proportion. The rays are typically short and robust, ending in bouquets with diverging conical spines (Figure 3B, Table 1). Rarely, one of the rays ends in a sharp point. Measurements: 5–(10.3)–15 μm.
Table 1. Comparative data for the dimensions of the spicules (in μm) and distribution of Timea alcoladoi sp. nov. and the morphologically closest species Timea chiasterina Carballo & Cruz Barraza, Reference Carballo and Cruz-Barraza2006. Shaft length × width; head diameter is given for megascleres and diameter for euasters

Values in parentheses are means.
Etymology
The species is named after Dr Pedro Alcolado, from La Habana, Cuba, for his substantial contributions to our knowledge of the Caribbean sponge fauna.
Distribution and ecology
Specimens of this species are difficult to observe in vivo because they are commonly almost completely covered by sediments. A large number of sites were sampled along the coast of the Gulf of Mexico and Mexican Caribbean, but the species was found only in shallow waters, about 1.5 m depth, encrusting the lower rock surfaces on sand-muddy bottoms at Puerto Real, Campeche (Figure 1).
Remarks
Timea alcoladoi sp. nov. is characterized by the presence of megascleres in the form of styles, some of them with a transition to incipient subtylostyles, and by a single category of microscleres, spherostrongylasters, which are very homogenous in size and shape (see Table 1).
The morphologically closest species to Timea alcoladoi sp. nov. is Timea chiasterina, described from the Gulf of California (Mexican Pacific). Both species possess a single category of microscleres euasters similar in morphology. However, they differ in external morphology: Timea alcoladoi sp. nov. presents a granular surface (Figure 2A, B), while T. chiasterina is characterized by a relatively smooth surface (Carballo & Cruz-Barraza, Reference Carballo and Cruz-Barraza2006). Megascleres are also different between both species, T. alcoladoi sp. nov. presents mostly styles (sometimes with a small annular rim), which are wider and with a larger range of size than those of T. chiasterina which are thinner tylostyles (with well-rounded heads) with smaller range of size (185–1010 × 4–20 μm vs 92–750 × 2–13; heads from 3–15 μm, respectively, Table 1). Microscleres in both species are also similar in size and general morphology, but in T. chiasterina the strongylasters possess commonly 7 and 8 (in a range of 6–9) large and thin rays diverging from a small centrum, while in the new species, the strongylaster's centre is commonly stout and wide, typically with 6 (in a range of 4–7) short and wide rays. After a detailed revision of slides of both species, we never found strongylasters of four rays in T. chiasterina, whereas those of 8 or 9 rays were absent in the new species.
The geographic distance and continental barrier that separates both species are other important factors to consider in species differentiation, especially considering that following sampling along the Mexican Pacific and Atlantic coasts, species present a very restricted distribution (T. chiasterina in two localities in the Gulf of California vs T. alcoladoi sp. nov., in one locality from the south of Gulf of Mexico). Contemporary authors also have discussed the importance of the Isthmus of Panama (raised about 3.5 MA ago) for Atlantic and Pacific sponge fauna, and the possible absence of connectivity of putative conspecific sponge populations from both sides of the Isthmus (Boury-Esnault et al., Reference Boury-Esnault, Klautau, Bézac, Wulf and Solé-Cava1999), preferring to keep separated names for related species (see Hajdu & Rützler, Reference Hajdu and Rützler1998; Carballo & Cruz-Barraza, Reference Carballo and Cruz-Barraza2010).
Another Timea species morphologically close to Timea alcoladoi sp. nov. is T. tylasterina Van Soest, Reference Van Soest2017 from the Guyana Shelf. This species is characterized by the presence of tylostyles and tylasters. It differs from Timea alcoladoi sp. nov. by its tylostyles (characterized by a rather elongate tyle), which are very much smaller than the styles of T. alcoladoi sp. nov. (204–388 × 2–(3.2)–4 μm vs 140–1000 × 4–(11)–20 μm, respectively). Timea tylasterina also possesses two categories of tylasters, the larger one (13–15.5 μm) 8–10 rays ending in a crown with 3–7 spines, and the smaller one (7–10 μm) with 7–11 short rays (Table 2), each ending in a profusely spined crown, often with double circles of spines. Timea alcoladoi sp. nov. possesses a single category, which is very consistent in morphology, with stout centre and commonly 6 (less commonly 4 and 7) divergent rays.
Table 2. Comparative data for the dimensions of the spicules (in μm) and distribution of Timea hechteli Lehnert & Heimler, Reference Lehnert and Heimler2001 re-described here, in addition to some species of Timea for comparison with T. alcoladoi sp. nov. Shaft length × width; head diameter is given for megascleres and diameter for euasters

Values in parentheses are means.
Other Timea-species bearing a variety of tylasters to strongylasters (9 spp.) have been described from the Western Atlantic, but they differ from Timea alcoladoi sp. nov. by possessing at least one other complementary euaster category (see also Table 2): T. hechteli Lehnert & Heimler, Reference Lehnert and Heimler2001, also has oxyasters (see next description); T. bioxyasterina Mothes, Santos & Campos, Reference Mothes, Santos and Campos2004, additionally has two oxyasters categories; T. berlincki Leite, Fonseca, Leal & Hajdu, Reference Leite, Fonseca, Leal and Hajdu2015, has acanthose spheroxyasters, with conical rays ending in sharp or blunt extremities; T. clandestina Leite, Fonseca, Leal & Hajdu, Reference Leite, Fonseca, Leal and Hajdu2015 has spherostrongylasters (with smooth conical rays) to tylasters (rays ending in extremities with distally oriented spines); T. curacaoensis Van Soest, Reference Van Soest2009, has a large and not truly asymmetrical euaster category, with branching rays, proliferated at their endings;
Timea perastra de Laubenfels, Reference de Laubenfels1936, possesses a combination of tylasters of 12 rays and 12 μm in diameter, and armed asters from 8 μm in diameter; T. secirm Moraes, Reference Moraes2011 possesses microspined oxyspherasters, and finally, T. parasitica (Higgin, Reference Higgin1877) has spheroxyasters. The other species of Timea known from the Western Atlantic (12 spp.) are easy to differentiate from Timea alcoladoi sp. nov. because they present a very different variety of euasters morphology.
Timea hechteli Lehnert & Heimler, Reference Lehnert and Heimler2001
(Figures 4 & 5)
Synonymy
Halicometes stellata sensu Little, Reference Little1963, p. 56.
Halicometes stellata sensu de Laubenfels, Reference de Laubenfels1950, p. 99.
Non Halicometes stellata (Schmidt, Reference Schmidt1870)

Fig. 4. External morphology and skeletal characteristics of Timea hechteli: (A, B) alive specimens LEB-ICML-UNAM-2232 and LEB-ICML-UNAM-2573, respectively; (C) light microscopy (LM) image of cross section of the skeletal structure; (D) Drawings of spicule tylostyles morphologies.

Fig. 5. Scanning electron microscopy images of spicules of Timea hechteli: (A–C) spicules of the Holotype (recorded as Halicometes stellata USNM 23571); (D–F) spicules from specimen LEB-ICML-UNAM-2232; (G–I) spicules from specimen LEB-ICML-UNAM-2573. (A, D, G) details of heads and ends of the subtylostyles; (B, E, H) smooth oxyasters; (C, F, I) spiny strongylasters.
MATERIAL EXAMINED
Holotype: USNM 23571. Panama City (Florida, USA), 1958 collected by Dr Meredith Jones.
New material: LEB-ICML-UNAM-2232, Puerto Real, Isla del Carmen (Campeche), 18°46′38″N 91°31′47.4″W, 6.3.2013, 1.5 m depth. LEB-ICML-UNAM-2573, XPICOB (Campeche), 19°43′15.89″N 90°40′9.11″W, 7.10.2013, 0.5 m depth. LEB-ICML-UNAM-3131, Puerto Real, Isla del Carmen (Campeche), 18°46′44.1″N 91°31′51″W 11.4.2014, 1.5 m depth. LEB-ICML-UNAM-3147, Puerto Real, Isla del Carmen (Campeche), 18°46′38″N 91°31′47.4″W, 2.2.2017, 1.5 m depth.
Description
Encrusting sponge, 8 mm thick, covering areas of 8 × 5 cm, over rocks. Specimens are almost completely covered by sediment, and they are visible mainly by orange meandering ectosomal channels, which ornament the surface (Figure 4A, B). The surface is smooth to slightly conulose to the naked eye, but under the microscope is unevenly hispid with the tips of the tylostyles protruding from the surface as small bouquets. Oscula are oval-circular shaped from 1–3 mm in diameter. Consistency is firm but easy to tear. The specimens are ochre to light yellow in life and pale ochre when preserved.
Skeleton
The skeleton is of the typical Timea structure, with tylostyle tracts (from 150–300 μm) distributed perpendicularly to the sponge surface, where they diverge into brushes, giving the surface a hispid appearance; length: 150–600 μm. Tylostyles free or forming tracts also are distributed parallel to the substrate surface. Euasters are randomly distributed throughout the sponge body but mainly concentrated in the sponge surface and near the substrate (Figure 4C).
Spicules
Tylostyles are mostly slightly curved or straight (Figure 5B), with a generally well-formed spherical or oval head (Figures 4D, 5A, D, G). They measure 410–(79)–1150 μm length, 8–(13.7)–20 μm shaft width, 10–(16.3)–25 μm head width (Table 2). Microscleres are euasters in two morphological categories. (I) Oxyaster, with about 10 large and thick, straight or slightly curved rays ending in sharp point (Figure 5B, E, H). In some stage of development (less common), euasters are oxysphaerasters, with a large centrum and short and stout, and sometimes mucronate rays, which end in sharp or rounded points (Figure 4E). Measurements: 7.5–(22.3)–35 μm diameter. (II) Strongylaster (type chiaster), very variable in size, but only one size category, with 8–10 stout rays diverging from a slender to moderated centre, and ending in small bouquets of 3–5 diverging, short conical spines (Figure 5C, F, I). Measurements: 3.7–(11)–22.5 μm diameter.
Ecology and distribution
Timea hechteli has never been recorded since its original description (as Halicometes stellata) by Little (Reference Little1963) in Panama City, Florida, USA, in the northern Gulf of Mexico (Figure 1). Specimens in this study were found in Puerto Real (Isla del Carmen) and XPICOB, at Campeche State (Gulf of Mexico). The species was found on rocks almost completely covered by sediment, living in shallow waters from 0.5 to 1 m depth, in sedimentary environments with strong wave action.
Remarks
Little (Reference Little1963) recorded Halicometes stellata from Panama City, Florida (northern Gulf of Mexico) based on some very small encrusting specimens which, according to the author, agree very well with the description of specimens from the Bermudas (de Laubenfels, Reference de Laubenfels1950). Later on, Hechtel (Reference Hechtel1969) transferred the records by Little (Reference Little1963) and de Laubenfels (Reference de Laubenfels1950) to the genus Timea, arguing that these represent a species with two euaster categories, but he did not propose a species assignation. In Timea, the specific name ‘stellata’ was already preoccupied by the type species T. stellata (Bowerbank, Reference Bowerbank1866), then Lehnert & Heimler (Reference Lehnert and Heimler2001) assigned a new name, Timea hechteli, to Little's (Reference Little1963) specimens. By giving this name, they erected a new species, but without a formal description of the type specimen (holotype). Due to the fact that Lehnert & Heimler (Reference Lehnert and Heimler2001) only referred to the record of Halicometes stellata sensu Little, it became the type specimen of T. hechteli.
Little (Reference Little1963) described a small specimen, 0.5–1.5 cm in diameter and 2–5 mm in height, which was used up for the spicule slide preparation. However, a very small portion of a specimen (about 3 mm in diameter) was found in the Invertebrate Zoology Collections in the Smithsonian National Museum of Natural History (Catalogue Number USNM 23571), which was used here to make a spicule slide and SEM photomicrographs, corroborating that the specimen corresponds to Little's original description. Little (Reference Little1963) stated that the species possesses tylostyles (211–(569.1)–1015 μm length), a few of which approach the shape of stylotes, which was confirmed by review of the spicules slide (original specimen), and in our own specimens (see Table 2 and Figure 5). The euasters were separated by Little into two types, I, oxyspherasters (18–33 μm, 26.4 μm average diameter) and II, chiasters (7 to 22 μm, 12.8 μm on average). Measurements were confirmed and complemented during the review of type material and agree with our specimens (see Table 2 and Figure 5). The species is well-differentiated from other Timea-species from the Western Atlantic by its typical spicular characteristics, specifically in size and shape (see Table 2).
Molecular taxonomy
In this study, we provide original DNA sequences for the new species Timea alcoladoi sp. nov. as well as for T. hechteli aiming to establish their taxonomic position among the Tethyida group. The COI fragment provided 579 pb (after clipping low-quality end-reads) for Timea alcoladoi sp. nov. (one sequence) and 709 pb for Timea hechteli (two sequences). The ribosomal region, ITS1 of T. alcoladoi sp. nov. (one sequence) was smaller with 323 pb than T. hechteli (three sequences) with 343 pb, while ITS2 provided 125 pb and 127 pb respectively. After a BLAST search (NCBI/Blast) comparison sequences of both species showed a high similarity to Tethyid species. For COI, both species showed major similarities with Tethya californiana de Laubenfels, Reference de Laubenfels1932 (KJ620403, AY561978), Tethya leysae Heim & Nickel, Reference Heim and Nickel2010 (GQ292532) and Tethya minuta Sarà, Sarà, Nickel & Brümmer, Reference Sarà, Sarà, Nickel and Brümmer2001 (EF584567) with 90% for Timea alcoladoi sp. nov. and 91% for T. hechteli. For the ribosomal region (ITS1-5.8S-ITS2), both species showed major similarities with Timea sp. (AJ633880), T. hechteli with 82% and T. alcoladoi sp. nov. with 83%.
The CO1 data set was larger (36 sequences) than the ITS data set (12 sequences) owing to the inclusion of other sponge groups by their proximity with sequences of Timea from GenBank; the alignment consisted of 483 pb with 206 variable sites, 181 of which were parsimony-informative. The rDNA region data set was considerably smaller due to the fewer sequences available from GenBank. After excluding ambiguous regions (through Gblocks), the alignment was 526 pb, where 262 were variable sites, with 189 parsimony-informative.
The Bayesian inference (BI) and Maximum likelihood (ML) trees for both data sets (COI and ITS) were mostly congruent in topology. For both loci, tree topologies of BI are presented in Figures 6 and 7. The number at each node represents the Bayesian posterior probability (PP) followed by the Bootstrap proportion (BP) from ML analysis. All our analyses were congruent in the allocation of Timea hechteli and Timea alcoladoi sp. nov. as a sister group of the principal clade of Tethyida with acceptable values (COI: 98PP/65BP; ITSs 100PP/100BP). They also were consistent in the relationship among species in this clade, where T. hechteli was clustered together to Timea cf. authia in a well-supported clade (COI: 90PP/99PB; ITSs 100PP/100PB), and forming a sister group with Timea alcoladoi sp. nov. (COI: 93PP/80BP; ITSs 79PP, 72BP). Additionally, in rDNA region (ITS1-5.8-ITS2) other two Timea sequences (GenBank) were grouped to Timea alcoladoi sp. nov. strengthening the Timea clade (Figure 7).

Fig. 6. Tree topology of COI mtDNA fragment. The tree presented was obtained by Bayesian inference (MrBayes) and includes Maximum likelihood (RA × ML) values. The number at each node represents the posterior probability (%) (BI), followed by the Bootstrap proportion (ML); a (–) indicates that a particular analysis supported the node at less than 50%, or supported an alternative phylogenetic arrangement in ML tree. The GenBank sequence accession code is included after each species name.

Fig. 7. Tree topology of rDNA region ITS1-5.8-ITS2. The tree presented was obtained by Bayesian inference and includes Maximum likelihood (RA × ML) values. The number at each node represents the posterior probability (%) (BI), followed by the Bootstrap proportion (ML); a (–) indicates that a particular analysis supported the node at less than 50%, or supported an alternative phylogenetic arrangement in ML tree. The GenBank sequence accession code is included after each species name.
Regarding COI tree topology, genus Timea and the order Tethyida were not recovered as monophyletic. Although Timea-species sequenced here were clustered beside other tethyid species (including genera Tethya and Adreus), two sequences from GenBank Timea sp. (AY561968) and Timea unistellata (KC869427) were clustered in a Poecilosclerida clade (Figure 6). Sequences of Axos cliftoni Gray, Reference Gray1867 (Hemiasterellidae: Tethyida) also were clustered far from the main Tethyida group, in an Axinellida basal clade (including genera of family Stelligeridae: Paratimea, Halicnemia and Plenaster). For rDNA region Timea was monophyletic, but it is probably due to the scarce numbers of available sequences. Even so, tree topology was congruent with the COI hypothesis about the phylogenetic relationships of our species as a sister clade of all other Tethyida.
Discussion
Diversity and distribution of Timea
Including our new species, 58 species of Timea are currently recognized around the world (Van Soest et al., Reference Van Soest, Boury-Esnault, Hooper, Rützler, de Voogd, Alvarez, Hajdu, Pisera, Manconi, Schönberg, Klautau, Kelly, Vacelet, Dohrmann, Díaz, Cárdenas, Carballo, Ríos, Downey and Morrow2019). The West Atlantic is the richest area in Timea-species biodiversity, with about 22 species recorded, from which 16 remain clearly described today, but the rest are problematic: Timea agnani (Boury-Esnault, Reference Boury-Esnault1973) was reallocated to Cyamon (see Van Soest et al., Reference Van Soest, Carballo and Hooper2012); the specimen from south-eastern Brazil of Timea cf. authia (de Laubenfels, Reference de Laubenfels1956) needs to be described; Timea innocens Schmidt, Reference Schmidt1870 and Timea stelligera (Carter, Reference Carter1882) even though they are accepted as valid, the first is unrecognizable and the second one is probably not a proper Timea (see Van Soest, Reference Van Soest2009; Van Soest et al., Reference Van Soest, Boury-Esnault, Hooper, Rützler, de Voogd, Alvarez, Hajdu, Pisera, Manconi, Schönberg, Klautau, Kelly, Vacelet, Dohrmann, Díaz, Cárdenas, Carballo, Ríos, Downey and Morrow2019). Records of the Mediterranean species Timea stellifasciata sensu Boury-Esnault (Reference Boury-Esnault1973) and Timea mixta sensu Wiedenmayer (Reference Wiedenmayer1977) are considered inaccurate (see Van Soest, Reference Van Soest2009; Van Soest et al., Reference Van Soest, Boury-Esnault, Hooper, Rützler, de Voogd, Alvarez, Hajdu, Pisera, Manconi, Schönberg, Klautau, Kelly, Vacelet, Dohrmann, Díaz, Cárdenas, Carballo, Ríos, Downey and Morrow2019), but we prefer including them in the taxonomic key for future confirmation or specimen reallocation.
The Mediterranean Sea, Eastern Atlantic and Indian Ocean possess about 13 species each. The Western Pacific has 10 species recorded, while the Eastern Pacific only has six species (Van Soest et al., Reference Van Soest, Boury-Esnault, Hooper, Rützler, de Voogd, Alvarez, Hajdu, Pisera, Manconi, Schönberg, Klautau, Kelly, Vacelet, Dohrmann, Díaz, Cárdenas, Carballo, Ríos, Downey and Morrow2019). For the Gulf of Mexico area, the only previous record of Timea corresponds to T. hechteli (as Halicometes stellata sensu Little, Reference Little1963) from the USA coast. Although there is a record of Hymedesmia stellata (type species of genus Timea) by Topsent (Reference Topsent1889) from Campeche Bank, it is considered to be a misapplication (Van Soest et al., Reference Van Soest, Boury-Esnault, Hooper, Rützler, de Voogd, Alvarez, Hajdu, Pisera, Manconi, Schönberg, Klautau, Kelly, Vacelet, Dohrmann, Díaz, Cárdenas, Carballo, Ríos, Downey and Morrow2019). So, the species presented here correspond to the first record of the genus Timea for the Mexican coast of the Gulf of Mexico.
Morphology and molecular markers in Timea
The traditional taxonomy of Timea has been mostly based on spicules morphology and size, and especially the euasters ornamentation (Rützler, Reference Rützler, Hooper and Van Soest2002; Carballo & Cruz-Barraza, Reference Carballo and Cruz-Barraza2006). However, the lack of detailed euaster descriptions (mostly in old descriptions) associated with very complex terminology, make the species interpretation difficult (Carballo & Cruz-Barraza, Reference Carballo and Cruz-Barraza2006). The problem increases when a few species have shown an intraspecific variety of euaster morphology (Carballo & Cruz-Barraza, Reference Carballo and Cruz-Barraza2006), which could affect their utility as main diagnostic characters. The challenge in Timea taxonomy is not only at the intra- or interspecific level, sponges with quite similar morphology have been included in four different families from two orders: Timeidae, Tethyidae and Hemiasterellidae (order Tethyida), and Stelligeridae (order Axinellida) (Cruz-Barraza et al., Reference Cruz-Barraza, Vega, Ávila and Vazquez-Maldonado2017; Lim et al., Reference Lim, Wiklund, Glover, Dahlgren and Tana2017). Authors have discussed the narrow gap between some genera of Tethyidae and a few species of Timea (e.g. Timea bioxyasterina, T. clippertoni and T. tethyoides) due to the presence of ‘megaster-like’ asters that are rather larger than their ‘micraster-like’ counterparts (Leite et al., Reference Leite, Fonseca, Leal and Hajdu2015). However, the absence of a more detailed diagnosis for Timea hinders a correct species allocation.
Molecular markers have contributed to understanding the relationships of some Timea species and species with similar Timea morphology (see Redmond et al., Reference Redmond, Morrow, Thacker, Diaz, Boury-Esnault, Cárdenas, Hajdu, Lôbo-Hajdu, Picton and Collins2013; Thacker et al., Reference Thacker, Hill, Hill, Redmond, Collins, Morrow, Spicer, Carmack, Zappe, Pohlmann, Hall, Diaz and Bangalore2013; Lim et al., Reference Lim, Wiklund, Glover, Dahlgren and Tana2017). Nevertheless, there are still a low number of available sequences dispersed in different gene fragments (e.g. COI, 18S, ITS and 28S) hindering their use in a robust systematic approximation. Our COI tree was congruent with previous molecular approximations, showing Timea as polyphyletic (Redmond et al., Reference Redmond, Morrow, Thacker, Diaz, Boury-Esnault, Cárdenas, Hajdu, Lôbo-Hajdu, Picton and Collins2013; Thacker et al., Reference Thacker, Hill, Hill, Redmond, Collins, Morrow, Spicer, Carmack, Zappe, Pohlmann, Hall, Diaz and Bangalore2013; Lim et al., Reference Lim, Wiklund, Glover, Dahlgren and Tana2017) due to sequences of Timea sp. and T. uniestellata (from GenBank) that were clustered outside the Tethyida clade. In the future, it would be interesting to review the morphology of these samples to establish their taxonomic status. The tree topology of the rDNA region showed Timea as monophyletic, probably due to the low number of available sequences. Even so, the tree topologies of both fragments consistently show a robust clade of Timea (including our specimens) clustered as a sister group of the main Tethyida group. This clade, formed at the first time for COI, is not only congruent with previous 28S analyses (Thacker et al., Reference Thacker, Hill, Hill, Redmond, Collins, Morrow, Spicer, Carmack, Zappe, Pohlmann, Hall, Diaz and Bangalore2013; Cruz-Barraza et al., Reference Cruz-Barraza, Vega, Ávila and Vazquez-Maldonado2017), but also is according to the current systematics of Porifera (see Morrow & Cárdenas, Reference Morrow and Cárdenas2015) where Timeidae is one of the three families of Tethyida. So, this result together with the morphological analysis of our specimens supports our taxonomic decision to allocate our species into the genus Timea.
The Timea systematics is still difficult to resolve today. The low number of available sequences of three families of Tethyida; the non monophyly suggested by a few loci and the controversial similar morphology with species of other Porifera groups, involves the necessity to sequence the type species T. stellata as the first step to establishing their phylogeny. Meanwhile, for this morphologically difficult group, molecular markers with high resolution such as COI or ITS could be helpful to improve the interpretation of morphological characteristics for the taxonomic identification and genus assignation of the species.
Identification key for West Atlantic Timea species
This is to supplement the key provided by Lehnert & Heimler (Reference Lehnert and Heimler2001) and Van Soest (Reference Van Soest2009).

* Sensu Pulitzer-Finali (Reference Pulitzer-Finali1986) oxyspherasters 20–25 μm; Rützler et al. (Reference Rützler, Piantoni, Van Soest and Díaz2014) oxyspherasters 9–18 μm.
Acknowledgements
The authors thank Dr Enrique Avila, Laura Vazquez, Luz Botero and María de Los Angeles Herrera (ICML-Mazatlán), for their support in the sampling and laboratory, and David Bernal for English text review. We also thank Israel Gradilla Martínez (Centro de Nanociencias y Nanoestructuras, UNAM) and Yolanda Hornelas (ICMyL-UNAM) for the SEM photographs.
Financial support
This work was supported by Programa de Apoyo a Proyectos de Investigación e Innovación Tecnológica (PAPIIT-IA201614-2 and PAPIIT-IN210018) and Comisión Nacional para el Conocimiento y uso de la Biodiversidad (CONABIO-FB1621JF19312).