Introduction
An ongoing international research endeavour is to combine larval morphology and DNA barcoding to investigate the diversity of fish species; this combination provides unprecedented precision in larval taxonomy (Pegg et al., Reference Pegg, Sinclair, Briskey and Aspen2006; Hui-Ling et al., Reference Hui-Ling, Yu-Tze, Tai-Sheng, Ming-An, Ming-Yih, Kuang-Zong, Wen-Yu and Kwang-Tsao2013). With the rise of molecular techniques, it is worth validating published fish larval taxonomic descriptions. This approach can also provide new information to identify larvae that currently lack diagnostic morphological criteria. The proportion of fish with known taxonomic descriptions of their larvae varies geographically, depending on regional species richness and the amount of time and expertise that has been invested in taxonomic research in that region (Fahay, Reference Fahay2007). There has been substantial effort to describe early larval stages of fish in the eastern Pacific. This research has been published in landmark identification guides from the North-east Pacific (Matarese et al., Reference Matarese, Kendall, Blood and Vinter1989), the California Current System (Moser, Reference Moser1996) and the Colombian Pacific (Beltrán-León & Ríos-Herrera, Reference Beltrán-León and Ríos-Herrera2000). However, these guides are still incomplete when compared with the fish species richness in the Mexican Pacific and Gulf of California, a transitional faunistic region among the California, Panamanian (Panamic) and Cortez biogeographic provinces (Spalding et al., Reference Spalding, Fox, Allen, Davidson, Ferdaña, Finlayson, Halpern, Jorge, Lombana, Lourie, Martin, McManus, Molnar, Recchia and Robertson2007; Briggs & Bowen, Reference Briggs and Bowen2012).
The species identification of fish larvae using diagnostic characteristics based on morphology, meristics and pigmentation is a difficult task, particularly for rare species or genera that include sibling and cryptic species. The precise identification of fish larvae has been largely solved by comparing diagnostic morphological features with modern molecular methods (Victor et al., Reference Victor, Hanner, Shivji, Hyde and Caldow2009; Matarese et al., Reference Matarese, Spies, Busby and Orr2011; Hui-Ling et al., Reference Hui-Ling, Yu-Tze, Tai-Sheng, Ming-An, Ming-Yih, Kuang-Zong, Wen-Yu and Kwang-Tsao2013). DNA barcoding provides additional information to discriminate among closely related species that are morphologically similar (Hebert et al., Reference Hebert, Cywinska, Ball and de Waard2003a, Reference Hebert, Ratnasingham and de Waard2003b; Hebert & Gregory, Reference Hebert and Gregory2005; Ward et al., Reference Ward, Zemlak, Innes, Last and Hebert2005). Indeed, this method has frequently provided decisive evidence to discriminate problematic taxonomic groups of species (Taylor & Watson, Reference Taylor and Watson2004; Watson et al., Reference Watson, Charter and Lawley2015). DNA barcoding has been widely used in taxonomic, ecological and biogeographic studies to identify fish eggs (Harada et al., Reference Harada, Lindgren, Hermsmeier, Rogowski, Terrill and Burton2015; Lewis et al., Reference Lewis, Richardson, Zakharov and Hanner2016; Ahern et al., Reference Ahern, Gómez-Gutiérrez, Aburto-Oropeza, Saldierna-Martínez, Johnson, Harada, Sánchez-Uvera, Erisman, Castro-Arvizú and Burton2018) and larvae (Pegg et al., Reference Pegg, Sinclair, Briskey and Aspen2006; Hubert et al., Reference Hubert, Meyer, Bruggemann, Guerin, Komeno, Espiau, Causse, Williams and Planes2012; Hui-Ling et al., Reference Hui-Ling, Yu-Tze, Tai-Sheng, Ming-An, Ming-Yih, Kuang-Zong, Wen-Yu and Kwang-Tsao2013; I-Shiung et al., Reference I-Shiung, Kwang-Tsao, Shao-Liang, Gwo-Ching, Yu-Chih and Ta-Kang2013; Thirumaraiselvi et al., Reference Thirumaraiselvi, Das, Ramanadevi and Thangaraj2015; Camacho-Gastélum et al., Reference Camacho-Gastélum, Díaz-Viloria, Sánchez-Velasco, Jiménez-Rosenberg and Perez-Enriquez2017).
The genus Caranx (Carangidae) currently includes 18 extant nominal species, with numerous cases of synonymies and misspelled names (Froese & Pauly, Reference Froese and Pauly2021). Only five nominal Caranx species are distributed in the Eastern Central Pacific, namely Caranx sexfasciatus Quoy & Gaimard, 1825; Caranx melampygus Cuvier, 1833; Caranx lugubris Poey, 1860; Caranx caninus Günther, 1867; and Caranx caballus Günther, 1868 (Froese & Pauly, Reference Froese and Pauly2021). The cytochrome c oxidase subunit I (COI) gene sequences for each of those species have been deposited in GenBank and/or BOLDSystems. However, there are only diagnostic descriptions of the larval stages of C. caballus and C. sexfasciatus (Sumida et al., Reference Sumida, Moser and Ahlstrom1985). The larvae of both species are distinguished by a characteristic pigmentation pattern (Sumida et al., Reference Sumida, Moser and Ahlstrom1985). The diagnostic characteristics used to identify C. caballus larvae (<4 mm standard length) are the presence of pigments on the dorsal margin of the body (Figure 1A); C. sexfasciatus reportedly has a conspicuously pigmented supraoccipital crest (Figure 2A) (Sumida et al., Reference Sumida, Moser and Ahlstrom1985). All other described carangid larvae lack pigmentation on the supraoccipital crest (Sumida et al., Reference Sumida, Moser and Ahlstrom1985). In this study, we combined diagnostic characteristics (morphology, meristics and pigmentation) and DNA barcoding using COI gene partial sequences to clarify and validate the taxonomic species identification of Caranx larvae. This integrative analysis supports the separation and identification of Caranx species, an approach to identify reproductive and nursery areas useful in management of coastal fisheries in the Eastern Central Pacific (Froese & Pauly, Reference Froese and Pauly2021).

Fig. 1. Illustrations of Caranx caballus larvae. (A) Original illustrations according to Sumida et al. (Reference Sumida, Moser and Ahlstrom1985) and (B) C. caballus larvae confirmed using DNA barcoding in the present study. Note the identical pigmentation patterns in larvae shown in A and B.
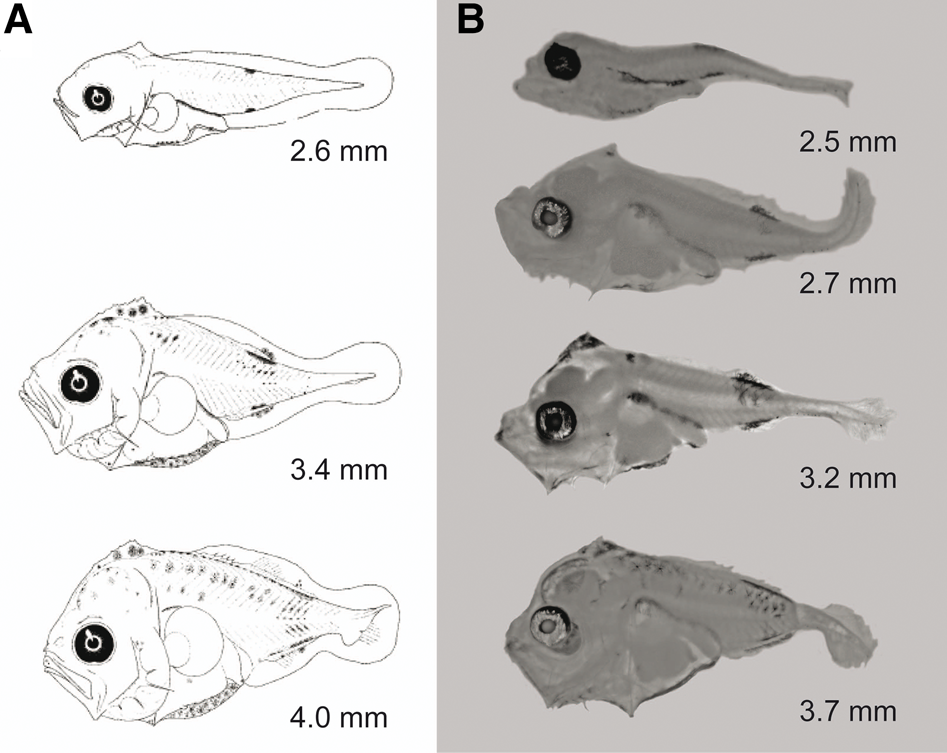
Fig. 2. Illustrations of Caranx caninus larvae. (A) Original illustrations of Caranx sexfasciatus according to Sumida et al. (Reference Sumida, Moser and Ahlstrom1985) and (B) C. caninus larvae confirmed using DNA barcoding in the present study. Note that these larvae were morphologically identical to those described previously as C sexfasciatus by Sumida et al. (Reference Sumida, Moser and Ahlstrom1985), but they genetically correspond to C. caninus larvae, with high mitochondrial cytochrome c oxidase subunit I (COI) similarity (99.7–100%).
Materials and methods
Taxonomic analysis of Caranx fish larvae was based on zooplankton samples collected from three regions of the Mexican Pacific, two in Baja California Sur (Cabo Pulmo National Park, sampled weekly between 2016 and 2017, and Ensenada de Muertos, October 2013) and a third in Jalisco (Cabo Corrientes, April 2015) (Table 1). Zooplankton samples were collected using a conical net (60-cm mouth diameter with a 333-μm mesh size) towed near the surface (<5 m depth), following a semicircular path at a mean speed of 1 m s−1 for 5 min. All zooplankton samples were collected during daylight hours and preserved in 95% ethanol, which was replaced after 24 h to ensure adequate preservation. A total of 149 Caranx fish larvae were identified to the most precise taxonomic level possible using meristic, morphometric and pigmentation criteria (Sumida et al., Reference Sumida, Moser and Ahlstrom1985). The standard length of all larvae was measured with a calibrated micrometer. The 35 best-preserved Caranx larvae were selected for molecular analysis; the specimens represented pre-flexion and flexion larval stages between 2.0 and 4.4 mm in length. These specimens provide adequate representation of their morphotypes. Specimens were photographed with a digital camera attached to a stereoscope. Curatorial information was uploaded to BOLDSystems (project: Identifying early life stages of fish from waters of the Mexican Pacific through DNA barcoding).
Table 1. Taxonomic identification of Caranx spp. fish larvae collected at three regions of the Mexican Pacific comparing morphological and molecular criteria

CP, Cabo Pulmo National Park (23°27′57.99″N 109°24′40.99″W); EM, Ensenada de Muertos (23°59′22.45″N 109°49′41.73″W), Baja California Sur; CC, Cabo Corrientes (20°17′ 50.31″N 105° 53′40.88″W), Jalisco, Mexico.
Similarity of COI barcoding of fish larvae collected north-west of Mexico compared with known DNA sequences typically from adults obtained from GenBank (GB) and BOLDSystems. Barcode Index Number assignation (BIN); clustered barcode sequences that create OTUs (operational taxonomic units) closely reflect species groupings.
DNA was extracted with a modified spin-column version of the fibreglass membrane method (Ivanova et al., Reference Ivanova, Dewaard and Hebert2006). A 651-base pair (bp) fragment was amplified from the 5′ region of the mitochondrial COI gene using primers FishF2-t1 (5′-TGTAAAACGACGGCCAGTCGACTAATCATAAAGATATCGGCAC-3′) and FishR2-t1 (5′-CAGGAAACAGCTATGACACTTCAGGGTGACCGAAGAATCAGAA-3′) (Ward et al., Reference Ward, Zemlak, Innes, Last and Hebert2005; Ivanova et al., Reference Ivanova, Zemlak, Hanner and Hebert2007). PCR amplifications were performed in 18-μl including 30 ng DNA template, 5× MyTaq Buffer (Bioline®), 10 μM of each primer, and 1 U of MyTaq DNA polymerase. PCR was performed in an Eppendorf Mastercycler Pro thermocycler, with the following thermal cycling conditions: 3 min at 96°C; 35 cycles of 30 s at 94°C, 40 s at 52°C, 1 min at 72°C; and a final extension of 5 min at 72°C. PCR products were visualized by electrophoresis in 1.0% agarose gels stained with ethidium bromide. PCR products were purified and sequenced in forward and reverse directions at the Instituto de Biología, Universidad Nacional Autónoma de México (IB-UNAM, Mexico City).
All COI sequences were manually edited and aligned using GENEIOUS® Prime 2020 software (https://www.geneious.com; Kearse et al., Reference Kearse, Moir, Wilson, Stones-Havas, Cheung, Sturrock, Buxton, Cooper, Markowitz, Duran, Thierer, Ashton, Mentjies and Drummond2012). We used the basic local alignment search tool (BLAST) included in GENEIOUS® and the Identification System of Barcode of Life Data Systems (BOLDSystems; http://www.boldsystems.org) to determine homology between the COI sequences from our study and previously deposited sequences. Each sequence was assigned a barcode index number (BIN) in BOLDSystems. The BIN was used for the interpretation of species boundaries based on the analysis of nucleotide variation patterns in the barcode region (Ratnasingham & Hebert, Reference Ratnasingham and Hebert2013). We used DnaSP software to obtain the number of haplotypes for each morphotype observed in the collected Caranx larvae and to remove redundancy in sequence data sets (Rozas et al., Reference Rozas, Sanchéz-DelBarrio, Messenguer and Rozas2003). The COI sequences of the five nominal Caranx species distributed in the Eastern Central Pacific were searched in the National Center for Biotechnology Information (NCBI) and BOLDSystems databases. Sequences of adult specimens with the same length (651 bp) were downloaded independently to the collection site. All sequences corresponded to the BINs of the five Caranx species: C. sexfasciatus (BOLD:AAB0584), C. melampygus (BOLD:AAB0585), C. lugubris (BOLD:AAI6630), C. caninus (BOLD:AAE2948) and C. caballus (BOLD:AAC4853). The haplotypes that characterized each adult Caranx species were also obtained from GenBank and BOLDSystems for comparison with the larval haplotypes obtained in the present study. All haplotypes were aligned using MEGA 10.0.5 software to calculate the intra- and inter-specific genetic distances (Kimura 2-parameter (K2P) method) and neighbour-joining (NJ) tree reconstruction with 10,000 bootstraps (Kumar et al., Reference Kumar, Stecher and Tamura2016). The mackerel scad, Decapterus macarellus (Cuvier, 1833), was used as an outgroup because the genus Decapterus has been placed in a sister clade of Caranx according to phylogenetic analyses of species of the family Carangidae (Reed et al., Reference Reed, Carpenter and de Gravelle2002). The complete D. macarellus COI genome sequence was downloaded from GenBank (accession number KM986880) (Zou et al., Reference Zou, Chen, Zhang and Li2016).
Results
A total of 149 Caranx fish larvae were analysed. The 35 best-preserved specimens were used to compare morphological and DNA barcoding information. Fourteen larvae were identified as C. caballus and 21 as C. sexfasciatus according to the diagnostic characteristics reported by Sumida et al. (Reference Sumida, Moser and Ahlstrom1985). The 14 larvae identified as C. caballus were based on the presence of sparse pigmentation in the larval stages; opposing dorsal, lateral, and ventral streaks on the body; and melanophores on the top of the head and over and along the abdominal region (Table 1, Figure 1). The remaining 21 larvae were identified as C. sexfasciatus based on the presence of a characteristic pigment in the supraoccipital crest, which is absent in other Carangidae species. The smallest larvae (2.4–2.6 mm) had no pigmentation on the crest (Table 1, Figure 2).
Each COI sequence obtained from the 35 Caranx larvae was 651 bp, without evidence of stop codons, insertions or deletions in the reading frame. Hence, these sequences represent functional COI sequences. All sequences were deposited in GenBank; the accession numbers are shown in Table 1. BLAST analysis showed that 10 of 14 larvae identified with diagnostics characters as C. caballus matched with C. caballus sequences from GenBank and BOLDsystems (99.8–100% similarity). Indeed, these 10 larvae were assigned a BIN (BOLD:AAC4853) that contained only C. caballus sequences. Thus, the diagnostic description of C. caballus larvae was genetically corroborated. However, the remaining four larval specimens identified as C. caballus matched C. sexfasciatus COI sequences (99.8–100% similarity); they were assigned a BIN (BOLD:AAB0584) that included only C. sexfasciatus sequences. The main diagnostic characteristics of these four fish larvae (2.7–4.0 mm in length) were the lack of pigmentation in the supraoccipital crest, over the gut, and in the terminal region of the gut (Figure 3). The 21 fish larvae previously identified as C. sexfasciatus based on the published diagnostic characteristics (Sumida et al., Reference Sumida, Moser and Ahlstrom1985) were genetically similar to C. caninus. Indeed, these specimens showed 99.7–100% similarity to C. caninus COI sequences and were assigned a BIN (BOLD:AAE2948) that exclusively included C. caninus sequences (Table 1).

Fig. 3. Illustrations of Caranx sexfasciatus larvae. The unidentified Caranx morphotype was later identified as C. sexfasciatus based on DNA barcoding evidence from larvae collected in the present study.
COI sequences of the 10 C. caballus larvae showed four distinct haplotypes (Hd = 0.73; π = 0.0014; three polymorphic sites) (Table 2). The COI sequences of the four C. sexfasciatus larvae included three distinct haplotypes (Hd = 0.83; π = 0.0017; two polymorphic sites). The COI sequences of the 21 C. caninus larvae included seven distinct haplotypes (Hd = 0.69; π = 0.0014; six polymorphic sites) (Table 2). Of the total of 91 Caranx COI sequences distributed in different regions of the world (downloaded from GenBank and/or BOLDSystems), four haplotypes belonged to C. caballus, 11 to C. sexfasciatus, three to C. caninus, seven to C. melampygus and two to C. lugubris (Table 3).
Table 2. Haplotypes of mitochondrial gene cytochrome c oxidase subunit I (COI, 651 bp) of larvae of the three Caranx species collected at the Mexican Pacific

Table 3. Haplotypes of mitochondrial gene cytochrome c oxidase subunit I (COI, 651 bp) of Caranx spp. sequenced from different regions of the world downloaded from GenBank and BOLDSystems

1International Barcode of Life (IBOL/BOLSystems); 2 Yancy et al. (Reference Yancy, Zemlak, Mason, Washington, Tenge, Nguyen, Barnett, Savary, Hill, Moore, Fry, Randolph, Rogers and Hebert2008); 3Hubert et al. (Reference Hubert, Meyer, Bruggemann, Guerin, Komeno, Espiau, Causse, Williams and Planes2012); 4Steinke et al. (Reference Steinke, Connell and Hebert2016); 5 Delrieu-Trottin et al. (Reference Delrieu-Trottin, Williams, Pitassy, Driskell, Hubert, Viviani, Cribb, Espiau, Galzin, Kulbicki, de Loma T, Meyer, Mourier, Mou-Tham, Parravicini, Plantard, Sasal, Siu, Tolou, Veuille, Weigt and Planes2019); 6 Steinke et al. (Reference Steinke, Dewaard, Gomon, Johnson, Larson, Lucanus, Moore, Reader and Ward2017); 7 Hou et al. (Reference Hou, Chen, Lu, Cheng and Xie2018); 8 Chang et al. (Reference Chang, Shao, Lin, Chiu, Lee, Liu and Lin2017); 9 Murakami et al. (Reference Murakami, James, Randall and Suzumoto2007), 10 Santos et al. (Reference Santos, Xiang and Tagawa2011); 11 Ward & Holmes (Reference Ward and Holmes2007); 12 Jaafar et al. (Reference Jaafar, Taylor, Nor, Bruyn and Carvalho2012); 13 Zhang & Hanner (Reference Zhang and Hanner2011); 14 Templonuevo et al. (Reference Templonuevo, Alcantara, Juanico and Yambot2018); 15 Justine et al. (Reference Justine, Rahmouni, Gey, Schoelinck and Hoberg2013); 16 Dahruddin et al. (Reference Dahruddin, Hutama, Busson, Sauri, Hanner, Keith, Hadiaty and Hubert2017); 17 Xu et al. (Reference Xu, Van-Damme, Li, Ji, Wang and Du2019); and 18 Unpublished.
Barcode Index Number (BIN); clustered barcode sequences that create OTUs (Operational Taxonomic Units) closely reflect species groupings.
The intraspecific genetic distances among the haplotypes of the five Caranx species were between 0.15–0.42%; and the interspecific between 6.16–14.34%. The low intraspecific genetic distance among haplotypes of Caranx larvae confirms the precise species identification of the larval morphotypes of the C. caballus (0.25%), C. caninus (0.27%) and C. sexfasciatus (0.26%) morphotypes collected in the present study (Table 4). The NJ tree of COI sequences shows five distinct clades that match the five nominal Caranx species; the haplotypes of the fish larvae sequenced in the present study were placed in each corresponding clade (Figure 4).

Fig. 4. Neighbour-joining tree using 19 haplotypes of cytochrome c oxidase subunit I (COI, 651 bp) of different Caranx species obtained from GenBank and/or BOLDSystems compared with 14 haplotypes of Caranx larval sequences obtained in the present study (haplotypes: ▴ = Caranx caninus; ■ = Caranx caballus; ♦ = Caranx sexfasciatus). Numbers shown on the tree branches indicate bootstrap values (>70%) based on 10,000 replicates. Numbers between parentheses are the sequences per haplotypes. The scale bar represents the genetic distance of the Kimura two-parameter model. The accession numbers of Caranx species sequences downloaded from GenBank and/or BOLDSystems are shown in Table 3.
Table 4. Kimura two-parameters model of genetic distance within Caranx species (intraspecific variability, bold font) and among Caranx species (interspecific variability) for haplotypes of mitochondrial gene cytochrome c oxidase subunit I (COI, 651 bp) of adults obtained from GenBank/BOLDSystems and fish larvae collected in the present study. Decapterus macarellus (Cuvier, 1833) was used as an outgroup

The percentage of genetic similarity, BIN assignments, genetic distances and reconstruction of the NJ tree strongly confirm the taxonomic identity of early larval stages of C. caballus. However, larvae identified as C. sexfasciatus according with diagnostic characteristics from Sumida et al. (Reference Sumida, Moser and Ahlstrom1985), matched with adult C. caninus sequences. This finding indicates the diagnostic characteristics used to identify C. sexfasciatus must be reassigned to describe C. caninus. Finally, COI sequence indicated that C. sexfasciatus larvae correspond to our previously undescribed morphotype (without pigmentation in the supraoccipital crest and over and along the terminal region of the gut; Figure 3). This morphotype was morphologically similar to C. caballus collected in the present study (Figure 1). Therefore, early larval stages of C. sexfasciatus and C. caballus identification is a taxonomic challenge using only diagnostic criteria.
Discussion
Diagnostic characteristics (morphology, meristics and pigmentation) are useful to taxonomists and ecologists because they allow fast identification of fish larval specimens collected in the field. However, the identification of fish eggs and larval stages is considerably more complex than juveniles and adults when discriminating among rare species or sibling and cryptic species (Ahern et al., Reference Ahern, Gómez-Gutiérrez, Aburto-Oropeza, Saldierna-Martínez, Johnson, Harada, Sánchez-Uvera, Erisman, Castro-Arvizú and Burton2018). The combination of diagnostic characteristics and DNA barcoding allows for greater precision in species identification (Hui-Ling et al., Reference Hui-Ling, Yu-Tze, Tai-Sheng, Ming-An, Ming-Yih, Kuang-Zong, Wen-Yu and Kwang-Tsao2013). Both methodological approaches are required to evaluate closely related species that show overlap in meristic and/or morphological diagnostic characteristics (Victor et al., Reference Victor, Hanner, Shivji, Hyde and Caldow2009; Matarese et al., Reference Matarese, Spies, Busby and Orr2011), which are used for precise identification of target species in ecological studies. Species identification using molecular confirmation (e.g. DNA barcoding) allow the distinction among nominal species in regions with great fish diversity, such as the Mexican Pacific and the Gulf of California (Thomson et al., Reference Thomson, Findley and Kerstitch1979; Allen & Robertson, Reference Allen and Robertson1994; Fischer et al., Reference Fischer, Krupp, Schneider, Sommer, Carpenter and Niem1995; Camacho-Gastélum et al., Reference Camacho-Gastélum, Díaz-Viloria, Sánchez-Velasco, Jiménez-Rosenberg and Perez-Enriquez2017).
Although there has been significant progress in describing the larvae of fish distributed in the North-east Pacific, the California Current System and the Colombian Pacific (Matarese et al., Reference Matarese, Kendall, Blood and Vinter1989; Moser, Reference Moser1996; Beltrán-León & Ríos-Herrera, Reference Beltrán-León and Ríos-Herrera2000), only two of the five nominal Caranx species (C. caballus and C. sexfasciatus) distributed in the Eastern Central Pacific are currently known by their larval morphological, meristic and pigmentation descriptions (Sumida et al., Reference Sumida, Moser and Ahlstrom1985). Although C. caninus, C. lugubris and C. melampygus adults have also been recorded in the Eastern Central Pacific (Froese & Pauly, Reference Froese and Pauly2021), their larval morphology is unknown. Kim et al. (Reference Kim, Divakaran, Brown and Ostrowski2001) analysed the digestive enzymes of early larvae from C. melampygus females that spawned under laboratory conditions; however, they did not provide morphological descriptions or photographs of those larvae. Avendaño-Ibarra et al. (Reference Avendaño-Ibarra, Aceves-Medina, Domínguez, De Silva-Dávila, Jiménez-Rosenberg, Urias-Leyva and Robinson2014) reported C. caballus, C. sexfasciatus and other morphotypes identified as Caranx spp. in an updated taxonomic list of marine fish larvae from the region between the Gulf of California and Colima (19–30°N, Mexico). We initially identified C. caballus larvae based on the diagnostic characteristics described by Sumida et al. (Reference Sumida, Moser and Ahlstrom1985) and then confirmed their taxonomic identity using COI sequences from those larvae. However, larvae identified as C. sexfasciatus – according to the main diagnostic characteristic of a conspicuously pigmented supraoccipital crest (Sumida et al., Reference Sumida, Moser and Ahlstrom1985; Moser, Reference Moser1996) – were actually C. caninus confirmed by: high genetic similarity (99–100%); clustered into a particular BIN; and low genetic distance among C. caninus haplotypes (0.27%). The larval morphotype genetically identified as C. sexfasciatus has no pigment in the supraoccipital crest and lacks pigments over the gut and along the terminal region of the gut. Thus, the genetic evidence indicates that the previous morphological and pigmentation description of C. sexfasciatus reported by Sumida et al. (Reference Sumida, Moser and Ahlstrom1985) must be considered diagnostic characteristics of C. caninus larvae.
Although there is a lack of diagnostic descriptions of the larval stage of C. lugubris and C. melampygus, both of which inhabit the Eastern Central Pacific, it is possible to identify the species within the genus Caranx using genetic divergence. In this sense, DNA barcoding is an effective tool to identify Carangidae species, based on their average genetic distance (K2P) among individuals (0.37%), species within genera (10.53%) and genera within the Carangidae family (16.56%) (Jaafar et al., Reference Jaafar, Taylor, Nor, Bruyn and Carvalho2012). The genetic distances obtained in the present study (intraspecific, 0.15–0.42%; interspecific, 6.16–14.34%) were similar to those reported by Jaafar et al. (Reference Jaafar, Taylor, Nor, Bruyn and Carvalho2012); these genetic distances increase with the change in the taxonomic level. This information can be used to identify and distinguish among highly related species (Ward et al., Reference Ward, Zemlak, Innes, Last and Hebert2005; Jaafar et al., Reference Jaafar, Taylor, Nor, Bruyn and Carvalho2012).
In summary, we have shown that the larval morphology of three of five Caranx species distributed in the Eastern Central Pacific is supported by their diagnostic characteristics and DNA barcoding evidence. Diagnostic and genetic characteristics of different ontogenetic larval stages of C. lugubris and C. melampygus must be investigated in the future. The present study is part of a continuous research effort to identify taxonomically larvae of poorly known species, genera or families; to collect their morphological descriptions; and to expand international COI sequence databases (GenBank, BOLDSystem). This taxonomic information will increase the precision of species identification in ecological, systematics, evolution and fishery management studies of marine fish resources.
Acknowledgements
We thank Patricia Cortés-Calva (Nodo CIBNOR Código de Barras), Laura Márquez-Valdelamar and Nelly María López-Ortiz (LaNaBio of Instituto de Biología, Universidad Nacional Autónoma de México) for their help with molecular analyses. We also thank David Castro Arvizu and his family for collecting zooplankton samples at Cabo Pulmo National Park from 2014–2017 and Rogelio González-Armas (CICIMAR-IPN) for individuals collected in Ensenada de Muertos. Thanks to Carlos A. Sánchez (UABCS), Octavio Aburto-Oropeza (SIO-UCSD) and Brad Erisman (University of Texas at Austin) for stimulating discussions and encouraging the realization of the zooplankton time series at CPNP. Furthermore, we thank SAGARPA and Carlos Ramón Godínez-Reyes (Comisión Nacional de Áreas Naturales Protegidas, CONANP) for providing the appropriate permission for this research project. Amigos de Cabo Pulmo and members of the Gulf of California Marine Program from Marine Biodiversity and Conservation Center (CMBC) helped with this research project, especially Juan José Cota-Nieto. We also thank Proof-Reading-Service Ltd, for editing the manuscript.
Financial support
Instituto Politécnico Nacional-CICIMAR (Coordinación General de Posgrado e Investigación grants: SIP-IPN 20200645, 20200696, 20210170 and 20210795); Secretaría de Educación Pública, Consejo Nacional de Ciencia y Tecnología (SEP-CONACYT) grant CB-2016-01-284201; and CONACyT Barcode of Life (MEXBOL, CONACyT 2018-295569) project ‘Identifying early life stages of fish from waters of the Mexican Pacific through DNA barcoding’ supported the present study.