INTRODUCTION
Evolution of the form—evolution of morphogenetic processes leading to the present shape of an organism—is one of the most intriguing questions of modern developmental biology. Recently many efforts have been made to understand the pattern formation and its regulation, genetic basis of cell differentiation and morphogenesis, and finally the differences between species. However, to propose an adequate hypothesis for interpretation of the evolution of the organism form it is necessary to have as far as possible the whole series of intermediate forms starting with the ancestral and ending with modern ones. At the same time, dealing with invertebrates in most cases it is difficult even to reconstruct this sequence. For most of the soft-tissue invertebrates there are too few paeleontological data and the reconstruction of the morphogenetic evolution is based on the comparative study of embryonic development and morphology of related taxa.
That is true in full measure for solitary (unitary) organisms that undergo the whole set of morphogenetic processes only once during their ontogenesis. Different is the characteristic for modular (colonial) organisms such as plants, colonial invertebrates (representatives of cnidarians, bryozoans, kamptozoans, tunicates etc.), fungi and some microorganisms (Rosen, Reference Rosen, Larwood and Rosen1979). During their regular mode of growth, colonial organisms undergo cyclic morphogenesis. Their body is constructed on the basis of repetition of a certain small number of elements (modules). That means that modular organisms grow continuously during their entire ontogenesis by addition of new elements to the colony. Such a property of modular organisms provides a good opportunity and model to investigate the morphogenetic processes themselves. Moreover, the cyclic morphogenesis makes available higher plasticity of patterning, allowing changes in spatial organization of modules without altering vitality of the whole organism (e.g. Kosevich, Reference Kosevich2006). Plasticity in the patterning of a modular organism increases its ecological fitness (Buss, Reference Buss, Larwood and Rosen1979; Ponczek & Blackstone, Reference Ponczek and Blackstone2001). Such plasticity is revealed mostly in different environments (e.g. in plants (Malygin, Reference Malygin2001)) and growth conditions (e.g. substrate-dependent growth in bryozoans (Nikulina, Reference Nikulina2000) and changes in zooid composition in polymorphic hydroid colonies (Frank et al., Reference Frank, Leitz and Muller2001)).
Recently the regular changes in patterning during colony development was described for some species of thecate hydroids (Hydrozoa: Leptomedusae) (Kosevich & Marfenin, Reference Kosevich and Marfenin1986; Kosevich, Reference Kosevich and Novikov2003, Reference Kosevich2006; Pyataeva & Kosevich, Reference Pyataeva and Kosevich2008). It was shown that during development from the settled planula larva, the spatial arrangement of the main elements (hydrothecae) within colony shoots undergo modifications in specified sequence depending on the size and nutrition of the colony. So the pattern of the elements of development and spatial organization is controlled epigenetically. These findings indicate that the morphogenetic programmes responsible for different patterning of elements within the hydroid colony can coexist within the individual and become apparent at a certain moment of its development. In such a case, the knowledge of the development during the entire life cycle of a modular organism allows to reconstruct at least in part its morphogenetic evolution. Still this is hardly realizable for many species.
In the present paper we argue for the other encouraging approach enabling an insight into the morphogenetic evolution of colonial hydroids and possibly other modular organisms. It is based on the analysis of the non-dominant morphotypes within shoot organization found during extensive study of the natural material. For the first time the search for different morphotypes was performed by N. Marfenin with colleagues (Marfenin, Reference Marfenin1975, Reference Marfenin1988; Marfenin et al., Reference Marfenin, Margulis and Mayer1995, Reference Marfenin, Malutin, Epelbaum and Novikov2003) for several species of Sertulariid hydroids: Dynamena pumila (Linnaeus, 1758), Diphasia fallax (Johnston, 1847), Sertularia mirabilis (Verrill, 1873) and Hydrallmania falcata (Linnaeus, 1758). The general number of the non-dominant morphotypes is relatively high but the frequency of individual appearance in natural populations of hydroids is very low. Most of such morphotypes are real ‘abnormalities’ caused by destructive influence of the environment, trauma or predators gnawing. The others are the signs of the severe disturbance of the morphogenetic programme and often prevent further development of the colony structure. Such ‘abnormal morphotypes’ are mostly unique (observed once and does not appear in repetitive manner) for each species of hydroids that confirm their anomalous origin. However, certain groups of rare morphotypes repeat themselves in different species with similar or even different spatial organization of shoots. Such rare morphotypes are functionally viable and bear most of the species-specific characters (of the hydrothecae, for example). Often such morphotypes repeat during several cycles of morphogenesis, whereupon the shoot organization returns to the dominant (‘normal’) morphotype. This is evidence that the insignificant and temporary disturbances of the morphogenetic programme cause emergence of such ‘deviations’ from the dominant morphotype but not the somatic mutations. Repetitive appearance of such ‘non-dominant’ morphotypes within different colonies or even within the same colony of a certain species points to temporary switching from one morphogenetic programme to the other one available within the whole set of morphogenetic programmes inherent for the species. If that supposition is true then the analysis of the non-dominant morphotypes can help us in reconstructing the sequence of the morphogenetic evolution in colonial hydroids.
MATERIALS AND METHODS
The thecate hydroid colony is composed of two main parts—the net of tubular branching stolons of hydrorhiza creeping over the substrate and set of branching shoots on the upper side of stolons (Figure 1A). Each shoot consists of numerous internodes with hydrothecae—the hydranth (zooid) protective housing. The stolons and shoots elongate at their termini due to the cyclic functioning of the growing tips (Beloussov, Reference Beloussov1973; Kosevich, Reference Kosevich and Davis2005). As a result the youngest parts of the colony are located at the periphery of the colony while the eldest parts are in its centre.

Fig. 1. Scheme of Dynamena pumila colony organization. (A) General scheme of the colony structure (modified after (Kuhn, Reference Kuhn and Spengel1914)); (B) enlarged part of the shoot with lateral branch (from (Cornelius, Reference Cornelius1979)). br, branch of a shoot; hy, hydrotheca; nd, nodal constriction between shoot internodes; sh, shoot; sht, shoot stem; sl, stolon of a hydrorhiza. Stolon and shoot growing tips are labelled black.
Animal description
For this paper we chose well studied species of thecate colonial hydroid Dynamena pumila (Linnaeus, 1758) (Hydrozoa: Leptomedusae: Sertulariidae). The colony consists of a net of the tube-form stolons and shoots protruding into surrounding water (Figure 1A). The following description is based on works of Cornelius (Reference Cornelius1979) and Naumov (Reference Naumov1969). The erect monosiphonic shoots over the stolons are more or less regularly spaced. The shoots branch sparsely and irregularly in one plane. The stem and branch divided in internodes of the same organization with nodal constrictions between every one, two or three internodes (Figure 1B). Each internode bears two hydrothecae in opposite to sub-opposite pairs. The hydrothecae of successive internodes form two longitudinal rows along the axis of the stem or a branch. The hydrothecae are tubular, curved outwards, 2/3 adnate to the axis of the stem or branch, aperture 2-cusped, operculum fragile with two flaps. The branches appear mostly one at a time at the base of one of the hydrothecae in the internode. They emerge not in every internode, but the successive branches mainly alternate, and lie in the plane of the internode so the whole shoot is feather-form.
The material was collected near the N.A. Pertsov White Sea Biological Station of the M.V. Lomonosov Moscow State University in the lower intertidal and upper subtidal zones. The voluminous samples were investigated under a dissecting microscope and the shoots with ‘non-dominant’ morphotypes were dissected from the colonies and fixed with 2.5% glutaraldehyde in phosphate buffer (pH 7.4).
Scanning electron microscopy
For scanning electron microscopy (SEM) investigation the fixed material was dehydrated through the graded ethanol series ending in 100% ethanol, acetone and critical-point-dried using CO2 in the Hitachi critical point dryer HCP-1. After mounting upon holders the specimens were sputtered with palladium-gold coat in Eiko IB-3 and examined in scanning electron microscopes (Hitachi S-405A and CamScan-S2).
RESULTS
The general description of colony morphology corresponds to the dominant internode morphotypes of Dynamena pumila. Nevertheless, at first we will stress some peculiarities of the shoot internode structure of the dominant morphotypeFootnote 1 (Figure 2).

Fig. 2. Details of the shoot organization and dominant internode morphotype structure in Dynamena pumila. (A) Part of a shoot, frontal view; (B) shoot internode of dominant morphotype, enlarged; (C) cross-section of the developing shoot internode over the diaphragm level. Thin arrows, planes of hydrothecae symmetry; thick arrow shows the plane of shoot symmetry and direction of the corresponding stolon growth; (D) lateral view on the shoot base. Stolon growing tip is on the right. hy, hydrotheca; nd, nodal constriction between shoot internodes; op, operculum; sha, shoot axis; sh, shoot; sl, stolon of a hydrorhiza. Arrowheads indicate the level of the hydrothecae diaphragms.
Dominant morphotype
The shoot internode with a pair of opposite hydrothecae is not flat. The adnate hydrothecae are bilaterally symmetrical. The planes of symmetry of both hydrothecae do not coincide with one another but form an angle of about 150–160° (Figure 2C). Therefore, in fact the internode itself is bilaterally symmetrical (Figure 2A,B). As all the internodes of the stem lie in one plane, the whole axis shows bilateral symmetry too. An interesting point is that the direction of the hydrothecae shift against the stem axis agrees with the direction of growth of the stolon bearing this shoot (Figure 2C). The base of the shoot stem itself tilts along the line of the stolon growth (Figure 2D). The irregularity of branching upset the bilateral symmetry of the whole shoot. The most usual variation in the shape of the D. pumila dominant morphotype is the degree of the nodal constriction between successive internodes and relative length of the proximal part of the internode (below the hydrothecae diaphragms) (Figure 2A,B).
Some non-dominant morphotypes
We will examine two rather frequent non-dominant morphotypes that are described as ‘uniserial’ and ‘sup-opposite’ hydrothecae arrangement along the stem axis.
‘Uniserial’ morphotype is characterized by formation of only one hydrothecae per internode. This morphotype mostly repeats in several successive internodes. The formed hydrotheca has typical shape and dimensions but lies in the plane of stem symmetry facing the stolons growing tip (Figure 3A–C). In most cases the ‘uniserial’ morphotype appears abruptly and suddenly switches to the dominant morphotype too. However, sometimes one can find the transitional zone including one to several internodes. Such a ‘transitional’ zone contains internodes revealing gradual transfer from dominant morphotype towards the ‘uniserial’ and vice versa. The main variants of ‘transient’ internodes are:
– the opposite hydrothecae are shifted towards one another so much that their walls come into contact (Figure 3D)—adnate frontally;
– the internode bears one large hydrotheca with two adjacent sets of flaps over the orifice (Figure 3F,G); two sets of operculum are the only sign of hydrothecae fusion;
– the internode has one hydrotheca of ordinary structure the width of which distinctly exceeds that of the dominant type (Figure 3E).

Fig. 3. Organization of the ‘uniserial’ and ‘transitional’ morphotypes of shoot internodes in Dynamena pumila. (A–C) Parts of the shoots with internodes of ‘uniserial’ morphotype. (A) Frontal view; (B, C) lateral view. (D–G) Parts of the shoots with internodes of ‘transitional’ morphotypes; (D) hydrothecae contiguous frontally; (E) enlarged hydrotheca of ordinary structure in internode of ‘uniserial’ morphotype; (F) internode of ‘uniserial’ morphotype bearing large hydrotheca with two adjacent sets of flaps over the orifice; (G) enlarged view of hydrotheca with two adjacent sets of flaps over the orifice. dmi, internode of dominant morphotype; hy, hydrotheca; op, operculum; sha, shoot axis; tmi, internode of ‘transitional’ morphotype; umi, internode of ‘uniserial’ morphotype.
Such ‘transient’ morphotypes emerge much more rarely than non-dominant ones and their appearance is associated with development of the ‘uniserial’ morphotype.
Sometimes one can find the other variant of ‘pseudo-uniserial’ hydrothecae arrangement. The single hydrotheca settles itself in normal position like in the dominant morphotype, but lacks an opposing one. In our opinion, that is the case of underdevelopment of one of the hydrothecae for some reason. The bulge over the stem axis at the level of the hydrotheca base on the corresponding side (Figure 4A) and the unicity of such internode in the overwhelming majority of observed cases support this idea.

Fig. 4. Scheme of some non-dominant morphotypes in Dynamena pumila (from (Marfenin et al., Reference Marfenin, Margulis and Mayer1995)). (A) ‘Pseudo-uniserial’ morphotype; (B) ‘sup-opposite’ (alternate) morphotype. bu, the bulge of the shoot axis at the position of underdeveloped hydrotheca; dmi, internode of dominant morphotype; hy, hydrotheca; sha, shoot axis.
‘Sup-opposite’ (alternate) morphotypes (Figure 4B) are usually found within one internode with internodes of the normal morphotype preceding and following it, sometimes including several successive internodes. Mostly they are found at the base of the lateral branches. In such a morphotype, the hydrothecae diaphragms are slightly shifted along the internode so that they do not lie at the same level opposing one another. However, the distance between the hydrothecae diaphragms of the same pair is noticeably smaller than the distance between the hydrothecae diaphragms of successive internodes.
DISCUSSION
Morphological variability in colonial thecate hydroids is a well-known characteristic for taxonomists of this group. Most of the works on taxonomy, or the biogeography of colonial hydroids, include remarks on variability in certain elements of the colony and their significance for exact identification of species. Nevertheless, there are few works on analysis of such variability in certain species of colonial hydroids and the data sometimes are contradictory. Still there are many cases when species description is based only on the investigation of a fragment of the colony. Afterwards further discovery of representational material allowed showing the limits of species variability and determining the exact species borders.
One of the reasons for relatively high morphological variability in thecate hydroids is that every outcome of the cyclic morphogenesis is fixed in shape of the outer rigid skeleton—the perisarc. Moreover, for the most part the morphology of the perisarc is the main characteristic feature for taxonomy of thecate hydroids. The period of the morphogenetic cycle (formation of the shoot or stolon internode) is relatively short—depending on temperature it is about 24–36 hours (Marfenin, Reference Marfenin1973; Kossevitch et al., Reference Kossevitch, Herrmann and Berking2001). Therefore, even short fluctuations in environmental parameters or disturbances of the morphogenetic programme can affect the morphology of the colony elements through altering the perisarc shaping (Kossevitch et al., Reference Kossevitch, Herrmann and Berking2001). Consequently, one can find many minor differences in perisarc morphology in successive shoot internodes. However, we pay attention to the morphological variations in shoot internode that are more or less stable—they repeat for no apparent reason in different parts of the colony in different moments of its development. So they are described as non-dominant morphotypes (Marfenin et al., Reference Marfenin, Margulis and Mayer1995). In addition, the most intriguing point is that certain morphotypes are not unique for exact species of thecate hydroids but appear in different species (Marfenin et al., Reference Marfenin, Margulis and Mayer1995, Reference Marfenin, Malutin, Epelbaum and Novikov2003; Kosevich, Reference Kosevich and Novikov2003).
The described dominant and ‘sup-opposite’ morphotypes of Dynamena pumila shoot internodes bear features characteristic for many sertulariid species. The shift of the hydrothecae towards one side of the stem axis is a well known feature for dominant morphotypes in different Dynamena and other sertulariid species with opposing hydrothecae arrangement (Dynamena dalmasi, D. disticha, Diphasia tropica, Sertularia distans, Tridentata distans and T. marginata) (Calder, Reference Calder1991; Migotto, Reference Migotto1996; Bouillon et al., Reference Bouillon, Medel, Pages, Gili, Boero and Gravili2004) (Figure 5A–C). Surprisingly, for Dynamena pumila it is not mentioned in most of the taxonomic descriptions.
The ‘sup-opposite’ morphotypes are described for the other ‘Dynamen’-type species of Sertulariids with opposite hydrothecae arrangement—Diphasia fallax (Naumov, Reference Naumov1969; Kosevich, Reference Kosevich and Novikov2003; Marfenin et al., Reference Marfenin, Malutin, Epelbaum and Novikov2003). In this species the appearance of such morphotypes is a transitory event too and observed mostly at the bases of the lateral branches. We believe that the ‘sup-opposite’ morphotype appears due to the influence of the maternal structures and smaller size of the growing tip of a new axis—the case of the lateral branch emergence. For comparison in several sertulariid species the diaphragms of two successive alternate hydrothecae can be located very close one to another, but still obviously at different levels (Figure 5D–G), e.g. Abietinaria filicula, A. articulata, Dynamena crisioides, D. nigra, D. disticha, Idiellana pristis, Sertularia cupressina, S. tolli and Sertularella reticulata (Naumov, Reference Naumov1969; Cornelius, Reference Cornelius1979; Migotto, Reference Migotto1996). Finally, we do not know any data on the transition from alternate to opposing hydrothecae arrangement in one species. In our opinion that means that the opposite (in pairs—‘Dynamena’-type) and alternate hydrotheca arrangement in two longitudinal rows have different origin and definitely have different morphogenetic programmes.

Fig. 5. Examples of ‘Dynamena’-type internode morphotypes in Sertulariid species. (A–C) Opposing and contiguous hydrothecae in Sertularia distans Lamouroux, 1816 (A) and Dynamena disticha (Bosc, 1802) (B, C). (D–G) Alternate hydrothecae arrangement in Abietinaria articulata (D) (from (Naumov, Reference Naumov1969)), Idiellana pristis (E) (from (Migotto, Reference Migotto1996)), Abietinaria filicula (F) and Diphasia nigra (G) (from (Cornelius, Reference Cornelius1979)).
The details of the ‘dominant’ and ‘uniseriate’ internode morphotypes in D. pumila allows to propose the following sequence of the morphogenetic evolution that led to appearance of ‘Dynamena’-type shoots within Sertulariids. The ancestral state is the shoot with sympodial type of growth and hydrothecae arranged in one row along one frontal side of the shoot stem (Figure 6A). This is the so-called crescent-type of sympodial growth (Schenck, Reference Schenck1965). Such organization of the shoot stem is retained in Aglaopheniidae. It looks as though such type of the shoot growth was the primary variant. The first modification was the enlargement of the hydranth rudiment that takes place without corresponding enlargement of the stem rudiment (Figure 6B); but the size of the hydrotheca cannot exceed certain limits. As a result, the hydranth rudiment splits into two closely located ones (Figure 6C). However, according to the rules of patterning the interaction between identical rudiments cause their spatial separation at the time of initiation (Meinhardt, Reference Meinhardt1982; Meinhardt & Gierer, Reference Meinhardt and Gierer2000). Finally, they occupied their present positions: opposite but slightly shifted towards one side of the stem (Figure 6D). According to this scenario of morphogenetic evolution strictly opposite position of the hydrothecae, which is an unstable state, in D. pumila and similar species is never reached. Some additional features of shoot patterning in ‘Dynamena’-type species can support this scheme:
– branching in such species is mostly irregular; branch formation starts after internode formation (sign of not modified sympodial type of growth);
– in species with such shoot patterning one will never find whorls of hydrothecae; in all cases the pairs of hydrothecae are oriented along one plane of the shoot stem or branch;
– there is no real alternate hydrothecae arrangement—in some cases it looks like alternate, but for a short portion of the stem or branch the hydrothecae are drawn together in pairs.
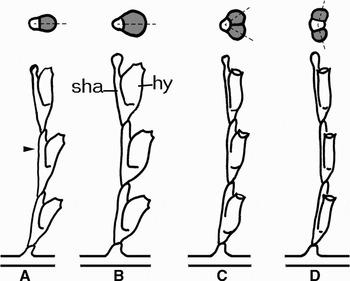
Fig. 6. Proposed scheme of evolutionary events led to ‘Dynamena’-type organization of shoots in sertulariids. Lower row—side view, stolon growing tip is on the right; upper row—scheme of the cross-section at the level indicated by the arrowhead. Hydranth rudiments (hy) are marked by grey colour. Sha, stem axis. Dotted lines indicate the plane of hydrotheca symmetry. (A) Crescent-type of sympodial growth in the ancestor shoot; (B) enlargement of the hydranth rudiment; (C) splitting of the enlarged hydranth rudiment into two rudiments of normal size; (D) separation of the hydranth rudiments—formation of the ‘Dynamena’-type organization.
We believe that during evolution of cyclic morphogenetic processes new programmes of the element's development do not lead to the complete substitution of the initial one. Instead, the modification of the preceding morphogenetic programme adds the new variant to the species ‘genotype’. Due to the regulatory mechanisms, the new variant is dominantly expressed during species development in usual conditions. The previous variants become dormant (non-dominant), but are not lost. Still in the case of some external disturbances or mistakes of regulatory mechanisms, the non-dominant morphogenetic variant can be temporarily expressed. Such morphotypes—and in most cases they show the preceding steps in evolution—become apparent because the genetic base of their formation is not lost. Due to modularity of morphogenetic programmes, the information on such morphotypes development is preserved in the genotype of the species in dormant state. That means that probability of the non-dominant morphotypes appearance and their diversity depends on the number of steps passed by the species during morphogenetic evolution. One or several of the morphotypes in the set of species morphogenetic programme are dominant and expressed mostly during colony development and growth in spatial and temporal manner (e.g. Hydrallmania falcata (Kosevich, Reference Kosevich2006), Sertularia mirabilis (Pyataeva & Kosevich, Reference Pyataeva and Kosevich2008)). Therefore, we can suppose that the probability of the non-dominant morphotype appearance depends on the species complexity and duration of morphogenetic evolution. If this is a case then it can explain why in Obelia-like species with typical fan-like sympodial mode of growth (O. longissima, O. geniculata, G. loveni, Laomedea flexuosa, C. lacerata, etc.) the only discovered non-dominant morphotype is the crescent-like sympodial growth of the shoot (personal observations). There is only one sub-dominant morphotype: the fan-like sympodial growth developed from the crescent-like form due to single modification of maternal programme.
The different possible explanation of observed morphotype variations in D. pumila and other colonial hydroid species can be based on the pattern-forming system, proposed by S. Berking and colleagues (Berking et al., Reference Berking, Hesse and Herrmann2002; Berking, Reference Berking2006). This system with self-organizing properties explains well most of the shoot patterns in thecate hydroids: different types of sympodial and monopodial growth of shoots, mutual arrangement of hydranth and stem rudiments, etc. However, in our opinion the proposed mechanism cannot give clear explanation for all details of D. pumila morphotypes appearance under consideration:
(1) The proposed system explains the increase in number and mutual arrangement of several similar rudiments (hydranth) accompanying the apical area enlargement. However, it says nothing about the reasons of the area enlargement. Moreover, according to our experience in D. pumila there are no real whorls of hydranths—in those cases when we observe three hydranths in a ‘whorl’ two of them are located at the same level and one is at a slightly lower position. Therefore, in such a case there are two tightly brought together internodes: one with a single hydranth and the other with a pair of hydranths.
(2) The transitional sequence from the internode with single hydranth towards the internode of dominant morphotype with the internode with hydranth formed close to each other in-between (Figure 3F) fits well to the proposed mechanism of patterning. Nevertheless, it can be more difficult to explain the opposite sequence of morphotypes development: from dominant morphotype to the internode with single hydranth on the front side of the stem (Figure 3 A & D). According to the discussed mechanism one can expect the appearance of the internode with the hydranth on the lateral side (Figure 4A), or transition from the opposite hydranth arrangement towards alternate (Figure 4B).
(3) According to the proposed pattern-forming mechanism, the resultant pattern of the D. pumila internode is sensitive to small alterations of few main parameters (Berking, Reference Berking2006). If this is the case then why are the non-dominant morphotypes so rare and their number relatively low? In severe developmental conditions (for example—within intertidal zone) with strong alterations of environmental factors, one can expect more frequent appearance of non-dominant morphotypes in comparison with development under more constant conditions. That is not a fact (Marfenin et al., Reference Marfenin, Margulis and Mayer1995).
Overall, there is no contradiction between our scenario and the pattern-forming system proposed by S. Berking and colleagues. On the contrary, both ideas are mutually complementary. We proposed the evolutionary sequence of the dominant D. pumila morphotype appearance based on the modularity of the developmental programmes. The pattern-forming system by S. Berking and colleagues allows working out in detail the exact mechanisms of the internode and stem patterning in thecate hydroids. Further elaboration of the system with possible inclusion of some new parameters can help in explaining the evolution of pattern-forming mechanisms at least in thecate hydroids in more detail.
ACKNOWLEDGEMENTS
The author thanks Professor S. Berking and Dr K. Herrmann (Zoological Institute, Cologne), Professor N.N. Marfenin (Moscow State University) for helpful discussion and inspiration of the work. The work was supported by the Russian Foundation for Basic Research, grant numbers 05-04-48662 and 07-04-00736.