INTRODUCTION
Scavenging amphipods are a vital component of the secondary production cycles that support numerous deep-sea ecosystems through organic carbon cycling and dispersal from large food falls (Stockton & DeLaca, Reference Stockton and DeLaca1982; Britton & Morton, Reference Britton and Morton1994; Payne & Moore, Reference Payne and Moore2006; Higgs et al., Reference Higgs, Gates and Jones2014). However, knowledge of the population ecology and life histories of this group of necrophages is limited. Recent efforts in the study of these organisms have improved our understanding of their community level ecology (e.g. Thurston, Reference Thurston1990; Jamieson et al., Reference Jamieson, Kilgallen, Rowden, Fujii, Horton, Lörz, Kitazawad and Priede2011; Duffy et al., Reference Duffy, Horton and Billett2012; Horton et al., Reference Horton, Thurston and Duffy2013), but studies examining the factors that affect population structure and dynamics of deep-sea scavenging amphipods are underrepresented.
Population studies carried out on shallow-water amphipod species (see Sainte-Marie, Reference Sainte-Marie1991, for a review of pre-1990 literature; Arndt & Beuchel, Reference Arndt and Beuchel2005; Nygård et al., Reference Nygård, Vihtakari and Berge2009) provide a robust framework when examining and analysing deep-sea scavenger populations. However, deep-sea amphipod population studies have primarily targeted populations of deep-sea amphipod species from vent (Sheader et al., Reference Sheader, Van Dover and Shank2000, Reference Sheader, Van Dover and Thurston2004; Sheader & Van Dover, Reference Sheader and Van Dover2007), canyon (Kaïm-Malka, Reference Kaïm-Malka2003, Reference Kaïm-Malka2004, Reference Kaïm-Malka2005) and trench environments (Perrone et al., Reference Perrone, Dell'Anno, Danovaro, Della Croce and Thurston2002; Thurston et al., Reference Thurston, Petrillo and Della Croce2002; Blankenship et al., Reference Blankenship, Yayanos, Cadien and Levin2006, Eustace et al., Reference Eustace, Kilgallen, Lacey and Jamieson2013). Cosmopolitan species have been studied to a limited extent with Eurythenes gryllus (Lichtenstein in Mandt, Reference Lichtenstein and Mandt1822) (Ingram & Hessler, Reference Ingram and Hessler1987; Thurston et al., Reference Thurston, Petrillo and Della Croce2002; Premke et al., Reference Premke, Klages and Arntz2006; d'Udekem d'Acoz & Havermans, Reference d'Udekem d'Acoz and Havermans2015) a notable example.
Paralicella caperesca Shulenberger & Barnard, Reference Shulenberger and Barnard1976 has a pan-oceanic distribution in lower latitudes. It has been recorded from widespread localities in the Pacific Ocean (e.g. Shulenberger & Barnard, Reference Shulenberger and Barnard1976; Yayanos, Reference Yayanos1981; Ingram & Hessler, Reference Ingram and Hessler1983; Hendrycks & Conlan, Reference Hendrycks and Conlan2003; Jamieson et al., Reference Jamieson, Kilgallen, Rowden, Fujii, Horton, Lörz, Kitazawad and Priede2011; Fujii et al., Reference Fujii, Kilgallen, Rowden and Jamieson2013) within a depth range of 1720–6200 m (Wilson et al., Reference Wilson, Smith and Rosenblatt1985; Hasegawa et al., Reference Hasegawa, Kurohiji, Takayanagi, Sawadaishi and Yao1986), from the Indian Ocean (Treude et al., Reference Treude, Janssen, Queisser and Witte2002; Cousins et al., Reference Cousins, Horton, Wigham and Bagley2013) and the Atlantic Ocean. The species has not been recorded in the Southern Ocean south of the Antarctic Polar Front (De Broyer et al., Reference De Broyer, Nyssen and Dauby2004, Reference De Broyer, Lowry, Jażdżewski, Robert and De Broyer2007) or in the Arctic Ocean. There are many records from the North Atlantic (Barnard & Shulenberger, Reference Barnard and Shulenberger1976; Thurston, Reference Thurston1979, Reference Thurston1990; Macdonald & Gilchrist, Reference Macdonald and Gilchrist1980, Reference Macdonald and Gilchrist1982; Smith & Baldwin, Reference Smith and Baldwin1982; Lampitt et al., Reference Lampitt, Merrett and Thurston1983; Desbruyères et al., Reference Desbruyères, Geistdoerfer, Ingram, Khripounoff, Lagardère, Laubier and Monniot1985; Barnard & Karaman, Reference Barnard and Karaman1991; Jones et al., Reference Jones, Collins, Bagley, Addison and Priede1998; Bühring & Christiansen, Reference Bühring and Christiansen2001; Duffy et al., Reference Duffy, Horton and Billett2012; Horton et al., Reference Horton, Thurston and Duffy2013), where it has been found in baited traps deployed at 2448 m on the Mid-Atlantic ridge (Horton et al., Reference Horton, Thurston and Duffy2013) down to 5940 m on the Iberian Abyssal Plain (Barnard & Shulenberger, Reference Barnard and Shulenberger1976). Records from the Tropical and South Atlantic (Thurston, Reference Thurston1990; Hendrycks et al., Reference Hendrycks, De Broyer and Havermans2010) suggest that the species has a pan-Atlantic distribution. Virtually all of the P. caperesca specimens reported have come from baited traps set on, or within a few metres of, the sea floor but the species can occur well up in the water column. It has been taken in mid-water trawls more than 700 m above bottom in the Atlantic (Thurston, Reference Thurston1990) and more than 900 m above bottom in the Pacific (Ingram & Hessler, Reference Ingram and Hessler1983). This broad geographic and bathymetric distribution is in spite of the limited dispersive ability, obligate brooding, and direct development that is characteristic of scavenging amphipods (Lincoln, Reference Lincoln1979).
Very little is known of the life history of P. caperesca beyond inferences made from mouthpart and gut morphology. The mandibular molars are conico-laminate and non-triturative, and appear to act as a ratchet mechanism pulling strips of food cut by the broad incisor processes towards the oesophagus in a manner similar to that reported for Alicella gigantea Chevreux, Reference Chevreux1899 (De Broyer & Thurston, Reference De Broyer and Thurston1987). The gut is capable of huge expansion, allowing the ingestion of large amounts of food implying the species is a highly specialized scavenger (Barnard & Shulenberger, Reference Barnard and Shulenberger1976; Shulenberger & Barnard, Reference Shulenberger and Barnard1976; Thurston, Reference Thurston1979). Thurston (Reference Thurston1979) noted that sexual dimorphism of P. caperesca is limited to relative antenna length and that it is unlikely that females of this species are capable of producing multiple broods. Further study will provide vital information on the life history of this species and will lead to a better understanding of specialized scavengers in the deep sea.
Paralicella caperesca has been identified from many traps deployed on the abyssal plains of the North Atlantic, and has also been found in particularly high numbers within submarine canyons of the Iberian Peninsula, dominating traps deployed there (Duffy et al., Reference Duffy, Horton and Billett2012). This allows for comparisons between populations of P. caperesca from submarine canyons and their counterparts from non-canyon environments. Submarine canyons form an interesting environment in which to study scavenging populations as they are often identified as hotspots of secondary production (Vetter, Reference Vetter1995; Soliman & Rowe, Reference Soliman and Rowe2008; De Leo et al., Reference De Leo, Smith, Rowden, Bowden and Clark2010; van Oevelen et al., Reference van Oevelen, Soetaert, García, de Stigter, Cunha, Pusceddu and Danovaro2011), which may result in an increase in the number of food falls (Higgs et al., Reference Higgs, Gates and Jones2014). This comparative study will allow a detailed examination of how the heightened secondary production of canyon environments is affecting growth and reproduction in scavenging amphipod species while elucidating life history traits of P. caperesca.
MATERIALS AND METHODS
Sample collection
Canyon populations of Paralicella caperesca were represented by specimens from seven samples, collected using baited traps, and sorted for community composition analyses (Duffy et al., Reference Duffy, Horton and Billett2012; Table 1). All canyon samples were collected from submarine canyons off the western Iberian Peninsula as part of the HERMES project (Figure 1; Weaver & Gunn, Reference Weaver and Gunn2009). Four samples were from baited trap deployments in Nazaré Canyon, two were from Setúbal Canyon, and one was from Cascais Canyon.

Fig. 1. Bathymetric chart of study areas coloured by water depth. Porcupine Abyssal Plain/Seabight and Iberian Margin shown in detail. Circles indicate non-canyon sampling sites and triangles indicate canyon sampling sites. See Table 1 for detailed sample collection information.
Table 1. Baited trap deployments for Paralicella caperesca used for this analysis. Shaded samples are from canyon sites using material from Duffy et al. (Reference Duffy, Horton and Billett2012) with canyon name indicated in area column. Non-shaded samples are from non-canyon sites.

Area abbreviations: PSB, Porcupine Seabight; CVP, Cape Verde Abyssal Plain; PAP, Porcupine Abyssal Plain; MAP, Madeira Abyssal Plain.
To allow comparison between populations in submarine canyons and those of non-canyon environments, commensurate samples were identified from the Discovery Collections (National Oceanography Centre, Southampton, UK). These non-canyon samples were chosen on the basis that they contained sufficient numbers (>50) of P. caperesca and were collected at a similar depth, during the same year or at a similar time of year as the HERMES canyon samples (Table 1). Eight of these samples were from abyssal plains in the NE Atlantic (Figure 1; Porcupine, Madeira and Cape Verde Abyssal Plains), and one was from the Porcupine Seabight. The P. caperesca components of canyon and non-canyon samples were analysed as outlined below.
Data collection
For large samples (>600 P. caperesca individuals) all specimens were assigned a unique identifier number and a random number generator was used to select a random subsample of 200 individuals. Selected individuals were dissected and measured under a stereo-dissecting microscope. Specimens were preserved in a variety of postures and many were damaged, making it difficult to obtain total body length measurements for all individuals. Past studies have resolved this problem using a single body-part measurement as a proxy for total body length (Chapelle, Reference Chapelle1995; Sheader et al., Reference Sheader, Van Dover and Shank2000, Reference Sheader, Van Dover and Thurston2004; Thurston et al., Reference Thurston, Petrillo and Della Croce2002; Blankenship et al., Reference Blankenship, Yayanos, Cadien and Levin2006; Sheader & Van Dover, Reference Sheader and Van Dover2007; Nygård et al., Reference Nygård, Vihtakari and Berge2009; Duffy et al., Reference Duffy, Horton, Sheader and Thurston2013). In this study coxal plate 4 was used as a proxy for total body length.
The total body length (distance along the dorsal margin between the anterior margin of the head and the tip of the telson) of 50 intact, randomly selected, P. caperesca from sample D297/15734 was measured using a digital graphics tablet and HTML-assisted Measuring System (HaMS; Duffy et al., Reference Duffy, Horton, Sheader and Thurston2013). These data were subsequently correlated to diagonal linear measures of coxal plate 4 (Figure 2; as in Chapelle, Reference Chapelle1995), which were made using a stage graticule. Estimation of total body length for all remaining specimens was possible using the coxal plate 4 measurements as a proxy. Coxal plate 4 measurements, rather than estimated total body lengths, were used for population characterization analyses (see below) to reduce the effect of rounding errors that would be magnified through the scaling up of measurements.
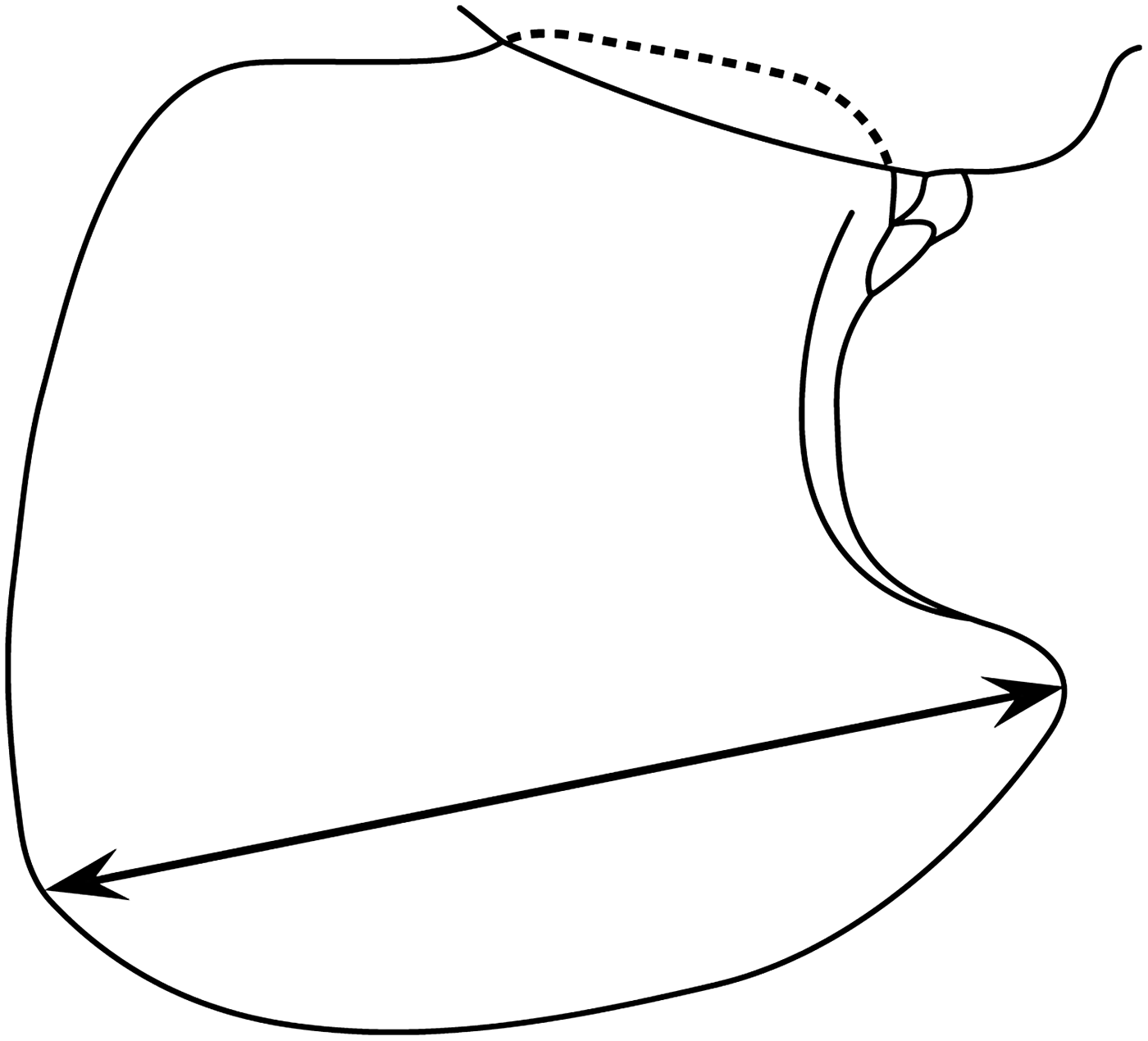
Fig. 2. Representation of coxal plate 4 of Paralicella caperesca (redrawn from Shulenberger & Barnard, Reference Barnard and Shulenberger1976). Arrowed line indicates the diagonal linear measurement (as in Chapelle, Reference Chapelle1995) used in this study as a proxy for total body length.
Primary and secondary sexual characteristics permitted sexing of all but the smallest individuals, which were classified as unsexed juveniles. Together with coxal plate 4, antenna 1 (length, number of articles, accessory flagellum length), antenna 2 (length, number of articles), and secondary sexual characteristics (oostegite length and presence of oostegite setae in females, presence of genital papillae in males) were also measured. Oocytes within ovaries of females at varying stages of sexual maturity were measured and counted.
Population characterization
Characterization of amphipod populations relies upon the identification of discrete cohorts, or growth stages, representing successive moults. Each of these cohorts contains individuals of similar size but not necessarily similar age, owing to growth rate being affected by a range of environmental factors. Identification of cohorts is often possible by identifying normal distributions from polymodal length frequency distribution data of total body length or a correlated proxy (Sheader et al., Reference Sheader, Van Dover and Shank2000, Reference Sheader, Van Dover and Thurston2004; Thurston et al., Reference Thurston, Petrillo and Della Croce2002; Kaïm-Malka, Reference Kaïm-Malka2003, Reference Kaïm-Malka2004, Reference Kaïm-Malka2005; Blankenship et al., Reference Blankenship, Yayanos, Cadien and Levin2006; Premke et al., Reference Premke, Klages and Arntz2006; Sheader & Van Dover, Reference Sheader and Van Dover2007; Nygård et al., Reference Nygård, Vihtakari and Berge2009; Duffy et al., Reference Duffy, Horton, Sheader and Thurston2013).
Oostegite measurements for females and coxal plate 4 measurements for males, females, and unsexed juveniles were plotted as probability density histograms. The identification of Gaussian components, each representing an oostegite stage or cohort, was performed initially by eye. The presence and parameters of each of these distributions were confirmed using the probability paper method (Cassie, Reference Cassie1954; Harding, Reference Harding2009) and the ‘mixdist’ package (Macdonald & Pitcher, Reference Macdonald and Pitcher1979; Macdonald & Du, Reference Macdonald and Du2011) in R statistical software (R Development Core Team, 2015). The goodness of fit of each of the modelled distributions was confirmed using the ANOVA function included in the ‘mixdist’ package.
Comparison of populations
Mann–Whitney U tests were used to compare distributions of male:female and juvenile:non-juvenile ratios between canyon and non-canyon samples. Once parameters for cohorts were accurately determined, individuals from each sample were assigned to the appropriate cohort based on 95% confidence limits (2 SD from the mean). Where overlap of these limits occurred individuals were assigned to cohorts based on the relative proportions of each cohort. Following classification, population composition data were analysed using multivariate tests in PRIMER 6 (Plymouth Routines in Multivariate Ecological Research; Clarke & Gorley, Reference Clarke and Gorley2006) statistical software. Data were root-transformed to reduce skewing and standardized to account for differing sample sizes. Following the creation of a Bray–Curtis similarity matrix, populations were compared using an ANOSIM test (Clarke, Reference Clarke1993) with sampling area (canyon, non-canyon) as the lone factor.
RESULTS
In total, 1422 Paralicella caperesca from canyon samples and 1575 individuals from non-canyon samples were dissected and measured. Males, females and juveniles were identified in all samples. No mature or ovigerous females were found. There was a positive correlation between coxal plate 4 length and total body length (L = 6.8902C + 1.0610, R 2 = 0.6517; where L is the estimated total body length and C is the linear measure of coxal plate 4, Figure 2). Although untransformed coxal plate 4 measurements were used for subsequent analyses, this relationship did permit the use of coxal plate 4 measurements as an indicative proxy to estimate total body length and allow for basic morphometric description and comparison of P. caperesca with other scavenging amphipod species.
Estimated total body length ranged from 1.70 to 22.34 mm. No individuals smaller than 4.65 mm showed primary or secondary sexual characteristics and were classed as juveniles. All individuals of 8.00 mm in length or more consistently exhibited primary and secondary sexual characteristics. A number of individuals smaller than 8.00 mm, mainly from canyon samples, could be sexed.
Sexual dimorphism
Estimated total body length in males ranged from 4.65 to 18.97 mm (median = 10.12 mm, IQ range = 8.86–10.55 mm) and in females from 4.65 to 22.34 mm long (median = 10.97 mm, IQ range = 8.86–13.07 mm). There was a significant difference between the size distributions of males and females (Figure 3; Mann–Whitney U-test: N = 963, U = 82,146, P < 0.001). Males possessed significantly longer antenna 1 and antenna 2, relative to coxal plate 4 length, than females (MWU: N = 948, U = 152,385, P < 0.001; N = 950, U = 171,933, P < 0.001 respectively). The antennal differences between the sexes was most pronounced in antenna 2, with the antenna 1 to antenna 2 ratio significantly lower for males than females (MWU: N = 940, U = 45,246, P < 0.001) with males more commonly possessing second antennae of equal or greater length than their first antennae. As a function of antenna length (articles mm−1) males possessed significantly more articles per mm of antenna length than females (MWU: antenna 1, N = 753, U = 68,733, P = 0.015; antenna 2, N = 755, U = 84,405, P = 0.001).

Fig. 3. Comparison between juvenile, male and female Paralicella caperesca from all sampling sites. Estimated total body length, using coxal plate 4 as a proxy, for males, juveniles, and females (A). Length of antennae as a function of coxal plate 4 length for antenna 1 and antenna 2 in male and female P. caperesca (B). Median and interquartile ranges shown, closed circles indicate individuals with estimated body length outside of the interquartile range.
Sexual development
A pair of ovaries could be identified between pereonites 3 and 7, lying either side of the gut, in females as small as 4.65 mm. Complete ovaries were recoverable from 59 individuals. Both ovaries contained approximately equal numbers of spheroid oocytes of comparable size, densely packed in a three-tiered linear arrangement. Oocyte size, calculated as the area of an ellipse, showed a weak positive correlation with coxal plate 4 length, a proxy of total body length (Figure 4A). The total number of oocytes within each whole ovary ranged from 26 to 75, with a mean of 42.4 oocytes per ovary, but there was no apparent relationship between oocyte count and estimated body size (Figure 4B).
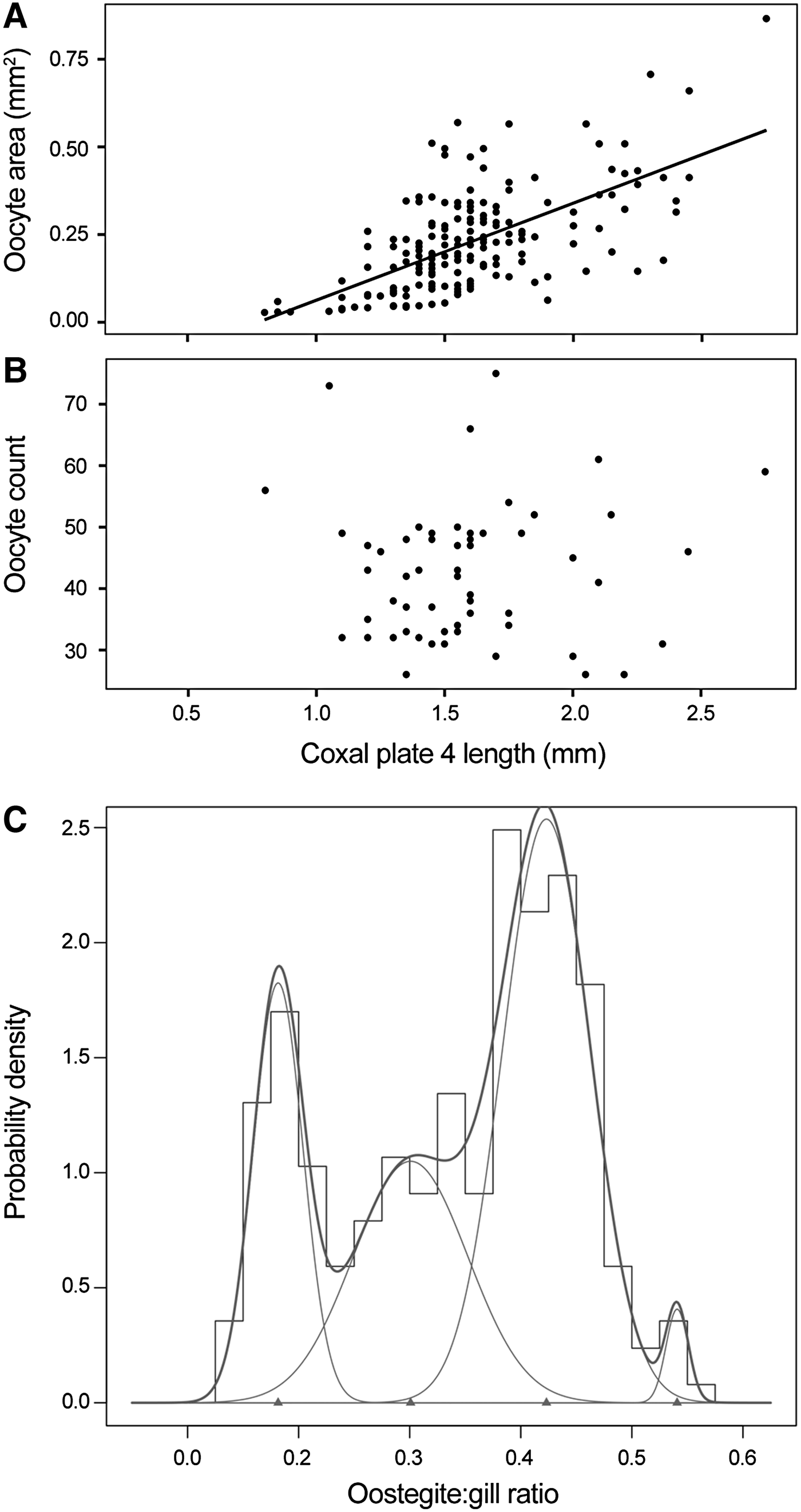
Fig. 4. Reproductive characteristics of female Paralicella caperesca individuals examined in this study. Oocyte size (A) and number of oocytes in each complete ovary (B) relative to the coxal plate 4 length, a proxy for body length, of each female where oocytes could be identified or complete ovaries were recovered. Oocyte size, calculated as the area of an ellipse, was weakly correlated to coxal plate 4 length (R 2 = 0.3946). Number of oocytes showed no correlation with coxal plate 4 length. Probability density histogram for oostegite:gill ratios for all female P. caperesca possessing oostegites from combined canyon and non-canyon samples (C). Normal distributions, identified using ‘mixdist’ package of R statistical software, indicated. Thin lines represent the distribution of each oostegite stage, thick line represents entire population.
The smallest female with visible oostegite buds was an estimated 4.65 mm in length but most females of this size had no detectable oostegites and could only be identified by ovary presence. Larger females possessed oostegites of varying size. A probability density histogram of oostegite length as a ratio of gill length (Figure 4C) identified four oostegite stages (OS1–OS4; Table 2) with minimal overlap. The presence and parameters of these stages were confirmed using the probability paper method.
Table 2. Counts of oostegite stage, identified by oostegite:gill ratios (OGR), for each female cohort of Paralicella caperesca from canyon and non-canyon sites.

No mature females with setose oostegites were found. Evidence of setal development was seen in some specimens possessing particularly large oostegites but in no case had setae evaginated. This suggests that these individuals were one moult away from sexual maturity. Testes were identifiable in the majority of males seen but were generally in poor condition making developmental classification impossible. Penile papillae in this species were small but could be identified on close examination of the underside of pereonite 7. The smallest male possessing visible penile papillae was an estimated 4.65 mm in body length.
Population characterization
Size distributions differed between the canyon samples and non-canyon samples, therefore separate cohort analyses were performed for each of these environments. Individuals that could not be sexed were assigned to four identified juvenile stages (Table 3, Figures 5 & 6). The parameters for the identified cohorts are comparable for the smallest individuals (J1, J2) but the separation between canyon and non-canyon cohorts becomes more pronounced at greater body length (J3, J4). Canyon cohorts have consistently larger mean total body lengths than their non-canyon counterparts.

Fig. 5. Probability density histograms of coxal plate 4, a proxy for total body length, measures for all unsexed juvenile (unshaded) and male (shaded) Paralicella caperesca from canyon (A) and non-canyon (B) samples. Normal distributions, identified using ‘mixdist’ package of R statistical software, indicated. Thin lines represent distribution of each cohort, thick line represents entire population.

Fig. 6. Probability density histograms of coxal plate 4, a proxy for total body length, measures for all unsexed juvenile (unshaded) and female (shaded) Paralicella caperesca from canyon (A) and non-canyon (B) samples. Normal distributions, identified using ‘mixdist’ package of R statistical software, indicated. Thin lines represent distribution of each cohort, thick line represents entire population.
Table 3. Mean coxal plate 4 measurement and estimated total body length (TBL) for all cohorts of Paralicella caperesca identified in this study from canyon and non-canyon sites. Linear growth factor between successive cohorts in parentheses.
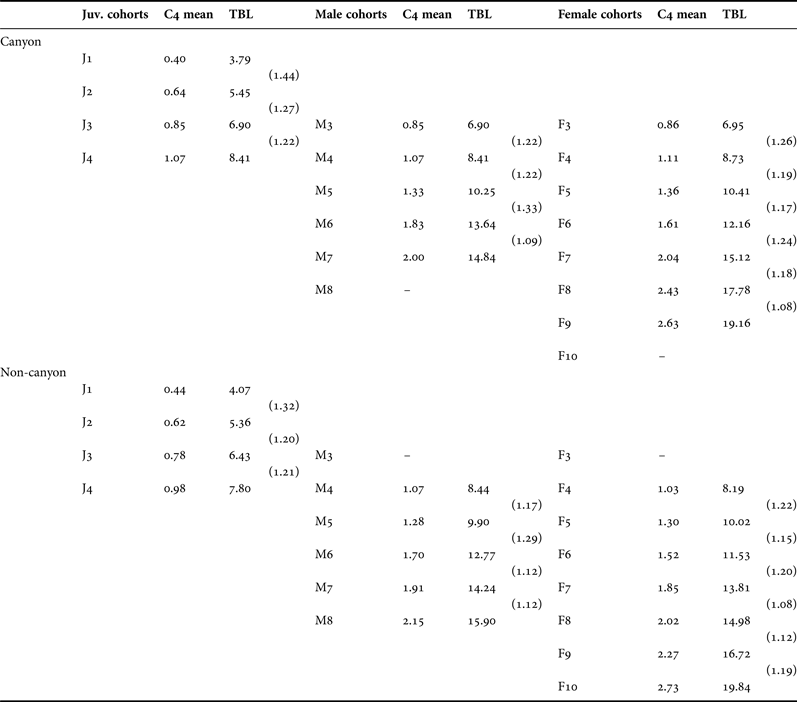
A total of five male (Figure 5; M3–M8) and seven female (Figure 6; F3–F10) cohorts were identified (Table 3). The occurrence and parameters of these cohorts were confirmed using the probability paper method and a ‘mixdist’ model fitted to the data (ANOVA: Canyon males, d.f. = 19, χ2 = 24.08, P = 0.1930; canyon females, d.f. = 25, χ2 = 51.88, P = 0.1243; non-canyon males, d.f. = 19, χ2 = 12.47, P = 0.8646; non-canyon females, d.f. = 20, χ2 = 25.53, P = 0.2634). There was direct overlap between the largest juvenile (J3, J4) and smallest sexed (M3, M4, F3, F4) cohorts. Cohorts were numbered to reflect this.
Cohorts M3 and F3 were found almost exclusively in canyon samples with individuals of similar size from non-canyon environments rarely showing any sexual characteristics and therefore classified as J3 juveniles. The two cohorts containing the largest individuals (M8, F10) were not represented in canyon samples. Canyon cohorts showed consistently larger mean total body lengths than their non-canyon counterparts (Table 3; Figures 5 & 6).
Linear growth factors between cohorts were comparable to those reported for A. abyssorum (Stebbing, Reference Stebbing1888) (Duffy et al., Reference Duffy, Horton, Sheader and Thurston2013) and early stages of E. gryllus (Thurston, Reference Thurston1979; Ingram & Hessler, Reference Ingram and Hessler1987). Increments between cohorts ranged from 1.10–1.44 with the largest growth increments between juvenile cohorts. Oostegite stages were distributed across female cohorts. More developed oostegites were more frequently observed in smaller individuals (F3–F6) from canyons than from non-canyon environments. The half-range of mature female body length (Sainte-Marie, Reference Sainte-Marie1991), a means proposed to distinguish between iteroparous and semelparous amphipod species, could not be calculated for P. caperesca as no mature females were available.
Population comparison
The male:female and juvenile:non-juvenile ratios did not differ significantly between canyon and non-canyon environments (Table 4; Mann–Whitney U-test: N = 14, U = 28.50, P = 0.620; N = 14, U = 27.50, P = 0.710 respectively). Both ratios varied widely within test groups. Multivariate analysis showed population composition based on cohort abundance varied significantly between canyon and non-canyon samples (ANOSIM: R = 0.284, P = 0.020).
Table 4. Total counts of males, females, and juveniles for all samples. Population composition ratios used for univariate comparison shown.

DISCUSSION
Sexual dimorphism
Sexual dimorphism of Paralicella caperesca, while apparent, is less pronounced than in some other deep-sea scavenging amphipod species such as A. abyssorum (Duffy et al., Reference Duffy, Horton, Sheader and Thurston2013) and E. gryllus (Ingram & Hessler, Reference Ingram and Hessler1987). Despite the lack of mature individuals, females were marginally larger than males in terms of estimated body length (Figure 3). This is in agreement with the pattern of inter-sexual size differences in lysianassoids demonstrated by Sainte-Marie (Reference Sainte-Marie1991). Paralicella caperesca shares many characteristics with lysianassoids. As such, the observed size difference between males and females suggests that P. caperesca belongs to a group of species that practices non-mate-guarding pre-copulatory behaviour (Bousfield & Shih, Reference Bousfield and Shih1994; Bousfield, Reference Bousfield2001) as documented in lysianassoids by Conlan (Reference Conlan1991). Males had significantly longer antennae with significantly more articles per mm of antenna length. The elongate antennae of male amphipods are believed to enhance chemosensing ability (Kaufmann, Reference Kaufmann1994) and tactile detection during mate searching (Steele, Reference Steele1995).
Sexual development
No mature females were found amongst the 2997 specimens examined in the present study and Thurston (Reference Thurston1979) identified only two mature females from a sample of 330 individuals taken at a depth of 4855 m in the North-East Atlantic. Ingram & Hessler (Reference Ingram and Hessler1983) did not indicate the proportion of adult female P. caperesca in their samples, so it is unclear as to whether the present observations represent the norm. The near-absence of mature females in baited-traps suggests that P. caperesca is a semelparous organism as hypothesized by Thurston (Reference Thurston1979).
The size of oostegites in female P. caperesca varied considerably within instars (Table 3), indicating a wide overlap of size ranges for succeeding developmental stages. This agrees with the pattern documented by Thurston (Reference Thurston1979) in material trapped on the Horseshoe Abyssal Plain. A re-analysis of benthopelagic material from midwater hauls taken up to at least 720 m above the Cape Verde Abyssal Plain (Thurston, Reference Thurston1990) showed a similar pattern (juveniles 3–8 mm, juvenile females 6–12 mm, immature females 10–17 mm, adult females 9–11 mm) with the adult females taken at least 100 m above the sea floor. This common pattern indicates that sexual development is plastic in female P. caperesca and that maturity is likely reached in fewer instars in canyon populations than abyssal plain populations. In contrast, there is no size overlap between non-adult and adult males implying that development is fixed or at least less plastic than in females.
Based on the contents of all complete ovaries recovered, the maximum possible brood size of P. caperesca ranges from 52–150 offspring with a mean estimated brood size of 84. Contrary to the findings of Ingram & Hessler (Reference Ingram and Hessler1987) for E. gryllus, there is no apparent relationship between oocyte number and body length, in agreement with Thurston (Reference Thurston1979), who observed that oocyte number in female P. caperesca was ‘at most weakly related to body length’. Total oocyte counts were substantially higher than those reported by Duffy et al. (Reference Duffy, Horton, Sheader and Thurston2013) for the bathyal scavenging amphipod A. abyssorum. Again using lysianassoid amphipods as a reference point, brood size loosely correlates with female body length but varies widely (Sainte-Marie, Reference Sainte-Marie1991). The estimated brood size of P. caperesca is substantially larger than that of most lysianassoids of similar size. The potential to produce large broods is in keeping with the hypothesis that P. caperesca is semelparous, investing substantial resources into one large reproductive event.
The frequency of the most developed oostegite stages (OS3, OS4) increased with increasing size-class number, however, no oostegites were setose regardless of oostegite stage and size-class. The lack of mature and ovigerous females leaves an important gap in our understanding of this species. As a result of the apparently semelparous lifestyle of P. caperesca it is unlikely that either of these development-classes will ever be caught using baited traps. The risks of predation at a food fall can be high (Lampitt et al., Reference Lampitt, Merrett and Thurston1983; Jones et al., Reference Jones, Collins, Bagley, Addison and Priede1998; Janßen et al., Reference Janßen, Treude and Witte2000; Jamieson et al., Reference Jamieson, Fujii, Solan, Matsumoto, Bagle and Priede2009, Reference Jamieson, Kilgallen, Rowden, Fujii, Horton, Lörz, Kitazawad and Priede2011). Furthermore, the ability of P. caperesca to ingest huge quantities of food also results in a morphotype incompatible with brood retention (Shulenberger & Hessler, Reference Shulenberger and Hessler1974; Shulenberger & Barnard, Reference Shulenberger and Barnard1976; Thurston, Reference Thurston1979).
The hypothesis that P. caperesca is semelparous is further supported by the fact that no empty ovaries were identified in any females examined. A semelparous lifestyle has been identified as a successful reproductive strategy for insects in environments with stable and predictable nutrient input (Fritz et al., Reference Fritz, Stamp and Halverson1982, and references therein). If, as theorized, a semelparous strategy is practiced by P. caperesca this may go some way to explaining the dominance and apparent success of Paralicella species in submarine canyons (Duffy et al., Reference Duffy, Horton and Billett2012), where nutrient input is elevated and relatively consistent.
Population characterization and comparison
The linear growth factors determined here for P. caperesca are comparable to those previously documented for this species (Thurston, Reference Thurston1979). These factors are lower than those of A. abyssorum (Duffy et al., Reference Duffy, Horton, Sheader and Thurston2013) and early instars of E. gryllus (Thurston, Reference Thurston1979; Ingram & Hessler, Reference Ingram and Hessler1987). They are similar to those reported for O. gerulicorbis (Thurston, Reference Thurston1979, as Orchomene), and higher than documented growth factors for Scopelocheirus hopei (Costa, Reference Costa1851) (Kaïm-Malka, Reference Kaïm-Malka2003) and Tmetonyx similis (Sars, Reference Sars1891) (Kaïm-Malka, Reference Kaïm-Malka2005).
There are marked differences in the population composition between the canyon samples and the non-canyon samples. The estimated mean total body length for each size-class was consistently higher for canyon size-classes than it was for their counterparts from the non-canyon environments. A number of environmental factors differentiating between these two sampling areas may be responsible for these differences. Canyon sites were generally shallower than non-canyon sites owing to their position on the continental slope. Therefore, canyon sites were also in marginally warmer water (Duffy et al., Reference Duffy, Horton and Billett2012). While the non-canyon group included one shallower site not from the abyssal plain (D092/9756/008, 3852 m), this sample group was nevertheless skewed toward deeper sampling. Amphipod growth rates and adult body size increase at warmer temperatures (Sutcliffe et al., Reference Sutcliffe, Carrick and Willoughby1981; Highsmith & Coyle, Reference Highsmith and Coyle1991; Pöckl, Reference Pöckl1992; Panov & McQueen, Reference Panov and McQueen1998; Maranhão & Marques, Reference Maranhão and Marques2003). However, the temperature difference between sample sites in canyons and non-canyon environments was very small (<0.1°C) compared with the temperature ranges used in laboratory experiments, making this explanation of the size differences between the populations unlikely.
Another possible explanation for the differing body-lengths between canyon and non-canyon populations is that the two environments support separate subspecies of P. caperesca. Despite the many records of this species from Pacific, Atlantic and Indian Oceans (see above), the only report of morphological variation is of minor differences between Pacific and Atlantic material (Barnard & Shulenberger, Reference Barnard and Shulenberger1976). All of the material used in this study was obtained from a relatively circumscribed area in the North-East Atlantic Ocean lacking obvious physical barriers. This apparent homogeneity is in contrast with the significant morphological variation reported for E. gryllus (Thurston et al., Reference Thurston, Petrillo and Della Croce2002; Stoddart & Lowry, Reference Stoddart and Lowry2004), shown subsequently to represent a species-complex containing at least five species (Havermans et al., Reference Havermans, Sonet, d'Udekem d'Acoz, Nagy, Martin, Brix, Riehl, Agrawal and Held2013; d'Udekem d'Acoz & Havermans, Reference d'Udekem d'Acoz and Havermans2015). The Eurythenes species-complex shows a bathymetric as well as a geographic element in the genetic divergences separating the included entities (Bucklin et al., Reference Bucklin, Wilson and Smith1987; Havermans et al., Reference Havermans, Sonet, d'Udekem d'Acoz, Nagy, Martin, Brix, Riehl, Agrawal and Held2013). All of the P. caperesca material used in this study came from depths exceeding 3000 m, a depth that appears critical for separating shallow and deep entities within the Eurythenes complex (Havermans et al., Reference Havermans, Sonet, d'Udekem d'Acoz, Nagy, Martin, Brix, Riehl, Agrawal and Held2013). This suggests that bathymetry is not a prime cause of the differences observed.
Supported by the differences seen between populations of A. abyssorum in areas of different surface productivity (Duffy et al., Reference Duffy, Horton, Sheader and Thurston2013), it appears that the most probable reason for larger total body length in canyon size-classes is a result of the increased nutrient input to canyon environments. While the availability of large food-falls to deep canyon sites is yet unknown, increased nutrient availability and heightened secondary production have been documented (Vetter, Reference Vetter1995; Soliman & Rowe, Reference Soliman and Rowe2008; De Leo et al., Reference De Leo, Smith, Rowden, Bowden and Clark2010; van Oevelen et al., Reference van Oevelen, Soetaert, García, de Stigter, Cunha, Pusceddu and Danovaro2011). The high abundances of scavenging amphipods found in submarine canyons (Duffy et al., Reference Duffy, Horton and Billett2012) lends further support to the theory that nutrient availability in an environment has significant effects on scavenging amphipods at both the community and population levels.
There are several possible explanations for the earlier onset of reproductive development in submarine canyon populations. As with growth rate, earlier reproductive development has also been attributed to temperature variations in other amphipod species (Sheader, Reference Sheader1983; Highsmith & Coyle, Reference Highsmith and Coyle1991), however, as previously discussed, water temperature in the sampled canyons was only marginally higher than at non-canyon sites (<0.1°C; Duffy et al., Reference Duffy, Horton and Billett2012). Once again, the difference in nutrient availability between the two areas is an important consideration. A link between nutrient availability and sexual development in P. caperesca has been previously hypothesized by Thurston (Reference Thurston1979). With an abundant food supply, individuals in canyons are able to apply resources to both growth and reproductive output allowing the onset of earlier reproductive development (Sutcliffe et al., Reference Sutcliffe, Carrick and Willoughby1981). Earlier maturation and oostegite development with nutrient-rich conditions has been recorded in the scavenging amphipod T. similis (Kaïm-Malka, Reference Kaïm-Malka2004). This developmental plasticity in response to food supply has also been observed in other amphipod species from very different habitats, such as the estuarine Gammarus duebeni Liljeborg, Reference Liljeborg1852 (Sheader, Reference Sheader1983) and the vent-dwelling Bouvierella curtirama Bellan-Santini & Thurston, Reference Bellan-Santini and Thurston1996 (Sheader et al., Reference Sheader, Van Dover and Thurston2004). In the food-limited abyssal plains resources are more constrained and therefore input into reproductive development is delayed until further growth has been achieved.
Ecological implications
Paralicella caperesca has the characteristics of a semelparous organism capable of one reproductive event in a lifetime and, as demonstrated by its abundance and ubiquity (Thurston, Reference Thurston1979, Reference Thurston1990; Lampitt et al., Reference Lampitt, Merrett and Thurston1983; Barnard & Karaman, Reference Barnard and Karaman1991; Jones et al., Reference Jones, Collins, Bagley, Addison and Priede1998; Duffy et al., Reference Duffy, Horton and Billett2012, Reference Duffy, Horton, Sheader and Thurston2013; Horton et al., Reference Horton, Thurston and Duffy2013), it is very successful in abyssal habitats. In his comparison of two scavenging fish species, Coryphaenoides acrolepis (Bean, 1884) and C. armatus (Hector, 1875), Drazen (Reference Drazen2002) hypothesized that the latter, an abyssal species, is semelparous owing to the nutrient regime of the abyssal zone. While food supplies in the abyss are low, they are also generally stable. This means survivorship of both juveniles and adults is relatively constant, making semelparity a successful and energetically conservative reproductive strategy. The same appears to be true for P. caperesca.
The small, sexually mature, P. caperesca females identified in material reported by Thurston (Reference Thurston1979, Reference Thurston1990) together with variability in sexual development across size-classes reported in this study suggest that maturation, and hence reproduction, of P. caperesca relies on an environmental trigger rather than being purely age or size dependent. There is strong evidence that this trigger is primarily nutrient availability. As an obligate scavenger capable of gorging itself (Shulenberger & Barnard, Reference Shulenberger and Barnard1976; Thurston, Reference Thurston1979), P. caperesca can capitalize on any high nutrient inputs into the abyss, such as large food falls, maturing and reproducing when these events occur. The expected increase in large food falls (Higgs et al., Reference Higgs, Gates and Jones2014) driven by increased sediment input to the submarine canyon system and heightened productivity (Vetter, Reference Vetter1995; Soliman & Rowe, Reference Soliman and Rowe2008; De Leo et al., Reference De Leo, Smith, Rowden, Bowden and Clark2010; van Oevelen et al., Reference van Oevelen, Soetaert, García, de Stigter, Cunha, Pusceddu and Danovaro2011) and the semelparity of P. caperesca allow this species to dominate canyon communities (Duffy et al., Reference Duffy, Horton and Billett2012).
The semelparous lifestyle of P. caperesca provides an interesting contrast to A. abyssorum, another deep-sea scavenging amphipod that has been studied in comparative detail (Duffy et al., Reference Duffy, Horton, Sheader and Thurston2013), which is more often associated with the bathyal zone (Horton et al., Reference Horton, Thurston and Duffy2013). Abyssorchomene abyssorum expresses the characteristics of an iteroparous organism capable of producing multiple broods of similar size to other iteroparous amphipod species (Sainte-Marie, Reference Sainte-Marie1991; Duffy et al., Reference Duffy, Horton, Sheader and Thurston2013). It is a less specialized scavenger than P. caperesca and believed to be a facultative rather than obligate scavenger (Shulenberger & Barnard, Reference Shulenberger and Barnard1976; Thurston, Reference Thurston1979). This may go some way toward explaining the differing reproductive strategies of these two species. Investing in a single, large, reproductive output in response to a food fall allows P. caperesca to maximize its success under the feast-famine regime experienced by obligate scavengers in abyssal environments and capitalize when the only nutrient resource it can consume becomes available. As A. abyssorum cannot gorge to the same extent as a specialist necrophage (Shulenberger & Barnard, Reference Shulenberger and Barnard1976; Dahl, Reference Dahl1979; Thurston, Reference Thurston1979), a more generalist feeding strategy where smaller amounts are consumed at more regular intervals is likely. This strategy lends itself to the multiple smaller reproductive outputs of iteroparity. These differing reproductive and scavenging strategies have allowed these two species to become highly successful and abundant within their respective environments (Duffy et al., Reference Duffy, Horton and Billett2012; Horton et al., Reference Horton, Thurston and Duffy2013).
Abyssorchomene abyssorum and P. caperesca have bathymetric distributions that overlap to a limited degree (Thurston, Reference Thurston1990; Horton et al., Reference Horton, Thurston and Duffy2013). Hydrostatic pressure and water temperature are often identified as factors controlling scavenging amphipod distributions and community composition (Thurston, Reference Thurston1990; Thurston et al., Reference Thurston, Petrillo and Della Croce2002) but trophic conditions have also been implicated as a contributory factor (Premke et al., Reference Premke, Klages and Arntz2006; Horton et al., Reference Horton, Thurston and Duffy2013). Our improved understanding of the contrasting feeding and reproductive strategies of scavenging amphipod species supports this theory. Obligate necrophages, such as P. caperesca, prevail in abyssal habitats, where background nutrients are poor and large inputs are likely to be patchy and often infrequent, through facultative breeding (i.e. based on food availability). If food and sufficient energy are available individuals will gorge, mature rapidly, breed, brood and die. Where episodic nutrient-rich inputs, such as large food falls, occur more frequently, species capable of earlier maturation and a large reproductive output when trophic conditions are favourable are able to dominate communities (Duffy et al., Reference Duffy, Horton and Billett2012). In regions where the trophic conditions are more stable and nutrients are less limited, generalists following an iteroparous lifestyle, with multiple small reproductive events, are at an advantage. Abyssorchomene abyssorum has adopted this lifestyle (Duffy et al., Reference Duffy, Horton, Sheader and Thurston2013) which may facilitate community dominance in shallower deep-sea habitats such as mid-ocean ridges (Horton et al., Reference Horton, Thurston and Duffy2013). Deep-sea scavenging fishes of the genus Coryphaenoides show similar patterns in reproductive strategy differences between abyssal and bathyal habitats (Drazen, Reference Drazen2002).
This study presents strong evidence for semelparity and nutrient-mediated growth, maturation, and reproduction in Paralicella caperesca. Comparing canyon and non-canyon populations of this species highlights how this strategy affects population dynamics, with canyon cohorts having consistently larger body size and expressing sexual characteristics at a smaller size. These findings provide important insights into the ecology of deep-sea scavenging amphipods. Semelparity complements the obligate necrophagy practised by P. caperesca and has been pivotal to the success of this species in both abyssal and deep-sea submarine canyon habitats.
ACKNOWLEDGEMENTS
The authors would like to thank the crews and scientists of all research expeditions that led to the collection of material used in this study. In particular, we are grateful to Ben Boorman for his assistance on multiple expeditions throughout his time at the National Oceanography Centre, Southampton. Thanks also go to Luke Fothergill for the development of HaMS software.
FINANCIAL SUPPORT
The research leading to these results has received funding from the European Community's Seventh Framework Programme (FP7/2007-2013) under the HERMIONE project, grant agreement no. 226354.