INTRODUCTION
The Anomalodesmata Dall, 1889 constitutes one of the most anatomically diverse sub-classes of the Bivalvia (Morton, Reference Morton1981a, Reference Morton, Wilbur, Trueman and Clarke1985a). Of the seven extant superfamilies of the Anomalodesmata (Morton, Reference Morton2012), four stand out as of especial interest, not just anatomically but also because of their lifestyles. The first of these is the Clavagelloidea d'Orbigny, 1844 with two constituent families – the Clavagellidae and the Penicillidae Bruguière, 1879 – representatives of which are all convergently either adventitious crypt or tube inhabitants and constitute some of the rarest bivalve taxa all inhabiting extremely narrow intertidal and shallow subtidal niches (Morton, Reference Morton2007). The other three superfamilies are the deep-water Verticordioidea Stoliczka, 1871, Poromyoidea Dall, 1886 and Cuspidarioidea Dall, 1886 which are sometimes grouped together as the Septibranchia although this term has fallen from wider taxonomic use, since not all taxa of the three superfamilies, for example Lyonsiella (Allogramma) formosa (Jeffreys, 1882) and Bentholyonsia teramachii (Habe, 1952) (Verticordioidea: Lyonsiellidae Dall, 1895), possess a septum but, rather, lamellibranch ctenidia but are nevertheless still predatory (Morton, Reference Morton1984, Reference Morton2003).
The term Septibranchia was first coined by Pelseneer (Reference Pelseneer1888) as an Order (Ordnung) of the Class Bivalvia (Muscheln), and who gave an account of the anatomy of a number of species of Cuspidaria collected by the Challenger Expedition (1872–1876). Subsequently, Pelseneer (Reference Pelseneer1911) described the cuspidariids collected by the Siboga Expedition (1899–1900), although Grobben (Reference Grobben1892) had earlier described in greater detail the anatomy of Cuspidaria cuspidata (Olivi, 1792). Plate (Reference Plate1897) described briefly, without figures, the anatomy of C. obesa (Lovén, 1846) and then Ridewood (Reference Ridewood1903) made a fuller illustrated description of the septum and labial palps of C. glacialis (G.O. Sars, 1878). Research on the septibranchs remained negligible until, 25 years later, the pioneering work of Yonge (Reference Yonge1928) laid the foundations for an interpretation of the functional morphology of C. rostrata (Spengler, 1793) (and with notes on C. cuspidata and C. obesa) and Poromya granulata (Nyst & Westendorp, 1839) (Poromyoidea). Yonge not only established details of anatomy, but also suggested how both taxa fed and on what. And, finally, showed how such food was digested.
There, research on the Septibranchia mainly halted until, from the 1970s onwards, various authors began to re-examine them – commensurate upon greater opportunities to collect such species from deeper, sometimes abyssal, depths. Knudsen (Reference Knudsen1967) examined the deep-sea Bivalvia collected by the John Murray Expedition of 1933–1934 and, subsequently (Knudsen, Reference Knudsen1970), examined the deep-water bivalves collected by the Danish Galathea Expedition plus other material and produced a beautifully illustrated account of the world's abyssal and, now, hadal, taxa including various species of Septibranchia. Allen & Turner (Reference Allen and Turner1974) described the anatomies of a range of verticordiid taxa, including species of Lyonsiella, Verticordia, Policordia, Laevicordia and Halicardia, from the abyssal Atlantic. Bernard (Reference Bernard1974) undertook the same task for a greater variety of taxa, including species of Verticordiidae, but also Poromyidae and Cuspidariidae from the abyssal Eastern Pacific. These descriptions included generalized illustrations of anatomy.
More detailed studies of cuspidariid anatomy include, importantly, interpretations of how prey was captured and their digestion accomplished by Reid & Reid (Reference Reid and Reid1974), Reid (Reference Reid1978) and Reid & Crosby (Reference Reid and Crosby1980). These authors researched Cuspidaria rostrata and C. obesa but also the closely related Cardiomya planetica (Dall, 1908). Such detailed anatomical and physiological (enzymatic) research first identified how gastric protein digestion was achieved and was supplemented by the work of Purchon (Reference Purchon1956, Reference Purchon1958, Reference Purchon1962, Reference Purchon1987) on septibranch stomach morphology. Allen & Morgan (Reference Allen and Morgan1981) undertook a study of the species diversity and anatomy of representatives of the Atlantic deep-water Cuspidariidae and Poromyidae, concluding with an interpretation, following on from the pioneer work of Yonge (Reference Yonge1928), of the evolution of the septibranch condition. Morton (Reference Morton1981b) re-examined Poromya granulata and showed how prey was captured – in a manner quite unlike that seen in cuspidariids. Later, the same author (Morton, Reference Morton1987) compared siphonal prey capture in a variety of septibranchs, showing that the process in each family was unique. Included in this survey was Parilimya fragilis (Grieg, 1920), a species of the newly erected family Parilimyidae Morton, Reference Morton1982 with links to the ancient anomalodesmatan stem family – the Pholadomyidae Gray, 1847 – and thereby representing yet another attempt by this sub-class of bivalves to occupy the deep sea and adopt a carnivorous lifestyle (Morton, Reference Morton1982). Morton (Reference Morton2003) studied the detailed anatomy of Bentholyonsia teramachii, a representative of the Lyonsiellidae, this species, in the possession of reduced ctenidia, non-sorting labial palps and a simplified stomach architecture, offering clues to the origin of bivalve predation in the deep sea.
Ten years later, Tëmkin & Strong (Reference Tëmkin and Strong2013) re-examined stomach structure in the cuspidariid Bathyneaera demistriata (Allen & Morgan, Reference Allen and Morgan1981) (=B. hadalis (Knudsen, Reference Knudsen1970)) and the unrelated but also predatory deep-water scallop Propeamussium jeffreysii (E. A. Smith, 1885), a species previously described anatomically by Morton & Thurston (Reference Morton and Thurston1989). Recently, Oliveira & Sartori (Reference Oliveira and Sartori2014) have described the arenophilic radial glands of species of Cuspidaria and Cardiomya, structures not hitherto identified for the Cuspidariidae. Most recently, Morton et al. (Reference Morton, Machado and Passos2015) have described the anatomy of Grippina coronata Machado and Passos, 2015 and shown it too to be predatory on deep-water epipsammic crustaceans but, also, therefore to constitute a second family, the Spheniopsidae Gardner, 1928, of the Cuspidarioidea.
Throughout the Atlantic Ocean, Allen (Reference Allen2008) recorded 54 species of Cuspidariidae, this family representing the most numerous anomalodesmatan bivalve predators followed by representatives of the Verticordiidae (27) and Poromyidae (6). Hitherto, only two species of the predatory septibranchs, Cardiomya costellata (Deshayes, 1833) and Cuspidaria atlantica Allen & Morgan, Reference Allen and Morgan1981, have been recorded from the waters of the Açorean Archipelago (Martins et al., Reference Martins, Ávila, Borges, Madeira, Morton and Martins2009). Of these two taxa, only the former occurred as living individuals that were, therefore, available for study. In addition, Morton et al. (Reference Morton, Tristão da Cunha and de Frias Martins2013) showed that Açorean bivalves from all depths and including C. costellata were approximately half the shell length of conspecifics from elsewhere in the Atlantic. There is no comprehensive study of the anatomy of any species of Cardiomya, only specific details of individual taxa. Accordingly, a study was undertaken of the Açorean C. costellata individuals, its principal aims being to: (i) provide a comprehensive account of the species’ anatomy; (ii) determine the reproductive strategy; (iii) examine if the species’ small size in these nutrient-poor waters was the result of a lack of food (or not); and, finally (iv) to elucidate if this size reduction could affect the species’ life history trait and, if so, (v) discuss what might be the consequences.
MATERIALS AND METHODS
In 2006, a dredge survey of the benthic fauna of the southern seabed of São Miguel, Açores was conducted for 60 stations at depths ranging from 5–350 m (see Martins et al., Reference Martins, Ávila, Borges, Madeira, Morton and Martins2009, figure 1 for station identifications). All the molluscan taxa obtained from the survey were identified to species level and preserved in 70% ethanol. These have been reported upon by Martins et al. (Reference Martins, Ávila, Borges, Madeira, Morton and Martins2009) and Morton et al. (Reference Morton, Tristão da Cunha and de Frias Martins2013). Subsequently, the entire collection of specimens, including the living individuals and empty shell valves, obtained from the 60 stations encompassing a large area of the seabed, and previously rough-sorted, was examined to obtain measurements of the shell lengths of the commonest predatory bivalve, Cardiomya costellata. The measurements, to the nearest 0.5 mm using vernier calipers, have been used to obtain a picture of population structure.
Finally, 10 individuals of Cardiomya costellata were dissected to examine their morphology and two others were further fixed in 4% formalin and, following routine histological procedures, serially sectioned at 6 µm and the resulting slides stained in Ehrlich's haematoxylin and eosin.
RESULTS
Biology
Tebble (Reference Tebble1966) detailed the distribution of Cardiomya costellata and described how it occurs widely in the North Atlantic from the Norwegian Sea south around the northern coasts of the British Isles to Madeira, the Canary Islands and the Açores (as also recorded herein) and further south to Liberia, Ghana and Gabon. It also occurs throughout the Mediterranean (Poppe & Goto, Reference Poppe and Goto1993). These authors record the species as occurring at depths >18 m and to 200 m, respectively. Allen & Morgan (Reference Allen and Morgan1981) recorded the species from depths of between 5–2000 m. Similarly, Sneli et al. (Reference Sneli, Schiøtte, Jensen, Wikander, Stockland and Sørensen2005) recorded C. costellata from 205–515 m around the Faroe Islands and provided a world bathymetrical range of between 4–1900 m although whether these shells represented living individuals or not was undisclosed.
In the North Atlantic and Mediterranean, Cardiomya costellata is said (Tebble, Reference Tebble1966; Poppe & Goto, Reference Poppe and Goto1993) to grow to a shell length of 9.5 and 12.0 mm, respectively. The population structure of all individuals of C. costellata from living and empty right valves collected during the survey undertaken within Açorean waters is shown in Figure 1. Living individuals ranged in shell length from 4–6 mm and empty valves from 3–6.5 mm, as recorded earlier by Morton et al. (Reference Morton, Tristão da Cunha and de Frias Martins2013). Such a distribution appears uni-modal, possibly suggesting that the species lives for only 1 year although nothing is known of the growth rates of any cuspidarioid.

Fig. 1. Cardiomya costellata. Population structure of all individuals, living and empty right valves, collected during the survey.
No identifiable prey items have been recovered from either the infra-setal chamber or stomach of preserved individuals of Cardiomya costellata, although, as will be described, the skeletal remains of such prey have been identified in the stomach, hind gut and rectum of the sectioned individuals. Bernard (Reference Bernard1974, pp. 13, 14) provided details of the prey identified from other septibranch bivalves, including three species of Cardiomya. In the case of the latter, material found in the intestine included polychaete setae, ostracods, dinoflagellates, sponge spicules and sand grains. In the Açores, where the current specimens of C. costellata were obtained, benthic crustaceans are smaller than their mainland European conspecifics (Costa, personal communication). The significance of this will be discussed.
Anatomy
THE SHELL
The shell of Cardiomya costellata is illustrated in Figure 2 wherein it is shown from the right (2A), dorsal (2B), ventral (2C), posterior (2D) and anterior (2E) aspects. The fragile, near-translucent white shell reaches a length of 9.5 mm according to Tebble (Reference Tebble1966). The shell is roundly fat, except posteriorly where it is formed into a rostrum that constitutes some one-third of the total shell length. Anteriorly, the antero-dorsal margin of the shell slopes downwards to individually varying degrees so that the anterior margin is pointedly oval. The shell is characterized by some 20 radiating riblets which are most prominent posteriorly, the last demarcating the rostrum. These overlay a background of fine commarginal lines. The most prominent ribs are the two posterior-most ones extending from the umbos to the end of the rostrum. These separate the mostly smooth rostrum from the main body of the shell. The greatest shell width (Figure 2A–C, a---b) is approximately mid-way along the body of the shell so that the narrowly triangular rostrum is a posterior extension of it. The shell is thus distinctively inequilateral with the beaks situated slightly posterior to the dorso-ventral mid-line. The greatest left-right width of the shell (Figure 2D, E, x---y) is situated slightly above its mid point, when seen from the lateral, dorsal and ventral aspects (Figure 2A–C), indicating that this plump predator is shallow burrowing. Although the shell at first appears to be equivalve, it is in fact slightly inequivalve, the right portion of the rostrum dorsally overlapping the left (Figure 2B) whereas ventrally the left, crenulated, valve margin interlocks with and overlaps that of the right (Figure 2C–E). Growth lines are not clearly evident on the shell.

Fig. 2. Cardiomya costellata. The shell as seen from (A) the right side; (B) dorsally; (C), ventrally; (D) posteriorly and (E) anteriorly. (a---b = the greatest width antero-posteriorly; x---y = the greatest width dorso-ventrally).
Figure 3 is a ventral view of the hinge plate and lithodesma of Cardiomya costellata. There is a single postero-lateral hinge tooth (HT) on the right valve that fits under a small ridge on the left valve. The ligament is sunken, small and located between resilifers (RE) (sometimes referred to as small chondrophores) and barely visible being hidden by its ventral lithodesma (LI), which, when seen from beneath, is laterally oval and slightly enlarged posteriorly. The structure of the ligament of Cuspidaria cuspidata and how it is formed have been described by Yonge & Morton (Reference Yonge and Morton1980, figures 10 & 11) and further illustrated by Allen & Morgan (Reference Allen and Morgan1981, figure 29). The two valves are united dorsally by a pad of ‘fused’ periostracum (FP). ‘Fused’ because although Owen et al. (Reference Owen, Trueman and Yonge1953) coined the term ‘fusion layer’ for the thickened pad covering the primary ligament, Yonge (Reference Yonge1978) clarified its periostracal nature. Clearly, because periostracum must cover the entire shell, there is no fusion, although the term is herein retained simply to emphasize that it is present, as in all other bivalves.
Abbreviations used in the figures: A, A cell epithelium of the crystalline style sac; AAM, Anterior adductor muscle (or scar); AG, Arenophilic gland; AGS, Arenophilic gland sheath; AGSE, Arenophilic gland secretion; AN, Anus; APRM, Anterior pedal retractor muscle (or scar); ASRM, Anterior septal retractor muscle (or scar); AU, Auricle (of the heart); B, B cell epithelium of the crystalline style sac; BG, Byssal groove; BGL, Byssal gland; BU, Buttress; C, C cell epithelium of the crystalline style sac; CC, Ciliated cell; CM, Circular muscle; COS, Collagen sheath; CSS, Crystalline style sac; D, D cell epithelium of the crystalline style sac; DD, Digestive diverticula; ES, Exhalant siphon; F, Foot; FIMMF, Fused inner and inner surfaces of the middle mantle folds; FMF, Fused mantle folds; FP, Fused periostracum; G, Gonad; GE, Germinal epithelium; GS, Gastric shield; GS(NR), Gastric shield (non-removable portion); GS(R), Gastric shield (removable portion); GU, ‘Gutter’ of the crystalline style sac; H, Heart; HA, Haemocoel; HG, Hind gut; HM, Hood of the mouth; HP, Hinge plate; HT, Hinge tooth; IE, Inner epithelium; IMF, Inner mantle fold; IPI, Ingested prey item; IS, Inhalant siphon; ISS, Inter-siphonal septum; JS, Juvenile shell; L, Ligament; LI, Lithodesma; LM, Longitudinal muscle; LSRM, Lateral septal retractor muscle; M, Mouth; MA, Mantle; MG, Mid gut; MM, Mantle margin; MMF, Middle mantle fold; O, Oesophagus; ODD, Openings to the digestive diverticula in the stomach; OE, Outer epithelium; OMF, Outer mantle fold; OO, Oogonium beginning its first division; OSMMF, Outer surface of the middle mantle fold; P, Periostracum; PAM, Posterior adductor muscle (or scar); PC, Pallial crest; PE, Pericardium; PEG, Pericardial gland; PEGA, Pedal ganglia; PGA, Pedal gape; PL, Pallial line; PPRM, Posterior pedal retractor muscle (or scar); PR, Prodissoconch; PRM, Pedal retractor muscle; PRO, Primary oocyte; PRS, Primary spermatocyte; PS, Pallial sinus; PSRM, Posterior septal retractor muscle (or scar); R, Rectum; RE, Resilifer; RO, Rostrum; RT, Rejectory tract; SA, Sorting area in the stomach; SC, Secretory cell; SCD, Secretory cell droplets; SE, Septum; SEP, Septal pore; SG, Siphonal ganglia; SGR, Sand grains; SN, Siphonal nerve; SO, Secondary oocyte; SP, Siphonal papilla; SPC, Siphonal papilla cilia; SPE, Spermatocyte; SPN, Siphonal papilla nerve; SPT, Spermatid; SRM, Siphonal retractor muscle; SRP, Skeletal remains of prey; SS, Siphonal sheath; SSC, Supra-septal chamber; SSP, Siphonal sheath papilla; ST, Stomach; STA, Statocyst; TF, Transverse fibres; TM, Transverse muscles; V, Ventricle (of the heart); VG, Visceral ganglia; VMF, Ventral mantle fusion; VMM, Ventral mantle margin; YR, Yolk reserve.

Fig. 3. Cardiomya costellata. A ventral view of the hinge plate and lithodesma. (For abbreviations see Box.)
After removal of the lithodesma, internal views of the hinge plate (Figure 4) of Cardiomya costellata show the ligament (L) located on left and right resilifers (RE) with a slight swelling – the hinge tooth (HT) – of the posterior region of the right hinge plate (HP) that fits beneath a slight embayment on the opposite valve. There is a small prodissoconch (PR) and a juvenile shell (JS), the nepioconch that is ~250 µm in diameter.

Fig. 4. Cardiomya costellata. Internal views of the hinge plate after removal of the lithodesma. (A) The left and (B) the right valves. (For abbreviations see Box.)
THE MUSCULATURE
Figure 5A is an internal view of the left shell valve of Cardiomya costellata. It identifies the positions of the muscle scars, although these are difficult to discern on this fragile shell. There is a relatively large drop-shaped anterior adductor muscle (AAM) dorsal to which are two minute scars of the anterior septal retractor muscle (ASRM) and the anterior pedal retractor muscle (APRM). The posterior adductor muscle scar (PAM) is smaller than that of the anterior and, though also drop-shaped, is oriented in a postero-ventral direction. Antero-dorsal to this are two tiny scars of the posterior septal retractor muscle (PSRM) and the posterior pedal retractor muscle (PPRM) although the latter is hard to see because it is located beneath the hinge plate (HP). The two adductor muscle scars are linked ventrally by the extremely thin scar of the pallial line (PL) that, posteriorly, is formed into a shallow pallial sinus (PS). The rostrum (RO) is separated from the main body of the shell by an internal thickening that extends in a postero-dorsal-ventral direction. This is illustrated in greater detail in Figure 5B and is termed a buttress (BU), which, as will be discussed, strengthens the shell when the adductor muscles, particularly the posterior, contract. Here again is illustrated the posterior adductor muscle scar and that of the posterior septal retractor muscle. With the valve tilted slightly upwards, the location of the posterior pedal retractor muscle's insertion on the shell is seen to be internal to that of the septal retractor's.

Fig. 5. Cardiomya costellata. (A) An internal view of the left shell valve. (B) A detail of the posterior adductor muscle scar relationship with the shell and its buttress. (For abbreviations see Box.)
THE SIPHONS
Figure 6 shows the shell of Cardiomya costellata from the ventral aspect. It illustrates how the valve margins should interlock except for the fact that posteriorly, at the rostrum (RO), and anteriorly, at the pedal gape (PGA), the shell is slightly emarginate with adductor muscle contractions only resulting in closure at the mid-ventral base where the buttresses and siphonal retractor muscles (SRM) arise from the pallial line. Fusions (VMF) occur along the entire mid-ventral mantle margin except at the pedal gape (PGA). Posteriorly, the mantle is formed into siphons, but only the inhalant (IS) is apparent from this perspective. The siphons arise from a siphonal sheath that is fringed marginally by papillae (SSP). The sheath itself is hidden partially by sand grains (SGR) that are here glued to it. The siphons are also encircled by seven siphonal papillae (SP).

Fig. 6. Cardiomya costellata. The shell as seen from the ventral aspect showing the mantle fusions and pedal gape. (For abbreviations see Box.)
The siphons of Cardiomya costellata (Figure 7A), as seen from the right side, comprise a short exhalant (ES) with a small, un-fringed aperture and a much larger inhalant (IS) that is apically fringed by approximately 12 larger papillae interspersed by 12 smaller ones. Both siphons arise from an apically papilla-fringed (SSP) siphonal sheath (SS) within the shell rostrum (RO) and are surrounded by seven much larger siphonal papillae, or tentacles (SP). Figure 7B is a vertical section through one of the siphonal papillae. The apex of each papilla is cup-shaped and from which arise around 12 long (~40 µm) paddle-tipped cilia (SPC). These ciliated cells at the base of the cup are in connection with fine nerve fibrils (SPN) that extend down into the papilla, which is also characterized by many haemocoelomic spaces (HA). In transverse section (Figure 7C), each siphonal papilla shows the structure of a central nerve bundle (SPN) surrounded by capacious haemocoelomic spaces (HA).

Fig. 7. Cardiomya costellata. (A) The siphons as seen from the right side. (B) A vertical section through one of the siphonal papillae. (C) A transverse section through one of the siphonal papillae. (For abbreviations see Box.)
Figure 8A illustrates a transverse section through the siphonal sheath and both siphons of Cardiomya costellata. The mantle (M) that forms the siphonal sheath is formed dorsally into a pallial crest (PC) and ventrally there is the ventral mantle margin (VMM), which will be described in greater detail later. The siphons, which are formed by fusions of the inner folds and inner surfaces of the middle mantle folds, are thus of type B (Yonge, Reference Yonge1948, Reference Yonge1982). They are surrounded by seven papillae (SP), three dorsal to the exhalant and four lateral and ventral to the inhalant. More detailed sections through the walls of the exhalant and inhalant siphons are illustrated in Figure 8B and C, respectively. The exhalant comprises simple inner (IE) and outer (OE) epithelia. The inhalant comprises similar epithelia, but they enclose a complex musculature of underlying circular muscles (CM) enclosing blocks of longitudinal muscles (LM) that, in turn, enclose a central haemocoelomic (HA) core. Inner and outer epithelia are cross-connected by transverse bundles of muscle fibres (TM).

Fig. 8. Cardiomya costellata. (A) A transverse section through the siphons. (B and C) More detailed sections through the walls of the exhalant and inhalant siphons, respectively. (For abbreviations see Box.)
At its apex, the siphonal sheath of Cardiomya costellata contains distinctive, albeit small, arenophilic glands. These are illustrated in Figure 9 and have also been described for a number of other cuspidarioids by Oliveira & Sartori (Reference Oliveira and Sartori2014). The glands (AG) comprise, in section, conical clusters of secretory cells that occur ensheathed within a capsule (AGS) and are considered to produce a sticky secretion (AGSE) that is released via pores onto the inner epithelium (IE) of the apex of the siphonal sheath. The discharge of the secretions may be aided by the contractions of circular muscles (CM) in relation to the blood-filled haemocoel (HA) of the sheath. The arenophilic glands, which have been described for a number of anomalodesmatan taxa, produce secretions that are believed to be responsible in them all for the sticking of sand grains around the siphons and the posterior extremities of the shell (Oliveira & Sartori, Reference Oliveira and Sartori2014). In the case of C. costellata, sand grains adhere to the rim of the siphonal sheath and to the posterior end of the shell, thereby aiding in the camouflaging of the siphons when they are retracted into the shell, in turn, hidden in the sediment.
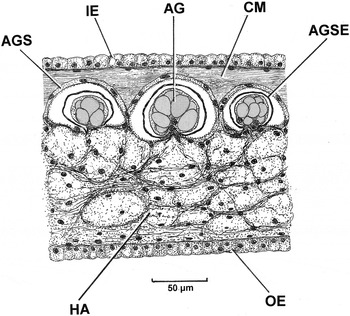
Fig. 9. Cardiomya costellata. A section through the siphonal sheath showing three arenophilic radial mantle gland units. (For abbreviations see Box.)
THE MANTLE MARGIN
The mantle margin of Cardiomya costellata is relatively simple and is illustrated in Figure 10 in transverse section through the region of the pedal gape. It comprises the typical bivalve three folds, although the middle (MMF) and outer (OMF) ones are exceedingly simple and between them give rise to a thin periostracum (P). In contrast, the inner fold (IMF) is capacious and encloses a huge haemocoel (HA). Extending into this fold is a barely discernible pallial retractor muscle (more obvious in the fused mantle margins – Figure 11, PRM) and some basophilic sub-epithelial secretory cells (SC). At the junction of the inner fold with the general mantle is a ciliated rejectory tract (RT).

Fig. 10. Cardiomya costellata. A transverse section through the right mantle margin in the region of the pedal gape. (For abbreviations see Box.)

Fig. 11. Cardiomya costellata. A transverse section through the ventral margin posterior to the pedal gape. (For abbreviations see Box.)
Posterior to the pedal gape, left and right mantle margins of Cardiomya costellata (Figure 11) are fused. Fusion involves the inner folds and the inner surfaces of the middle fold (FIMMF), which is of Type B (Yonge, Reference Yonge1957). The central region of the fused mantle margins are thus characterized by a narrow area of connectivity that in addition to the above also comprises the outer surfaces of the middle folds (OSMMF). The main body of the fused mantle margins comprises a capacious haemocoel (HA) contained within now greatly inflated outer mantle folds (OMF) each with a more obvious pallial retractor muscle (PRM).
THE ORGANS OF THE MANTLE CAVITY
The organs of the mantle cavity of Cardiomya costellata, as seen from the right side, are illustrated in Figure 12. The siphonal structure has already been described. Inside the main, anterior, body of the shell, the visceral mass only occupies the upper part of the mantle cavity and is located between the anterior and posterior adductor muscles (AAM, PAM). Into the visceral mass extend the paired anterior and posterior pedal retractor muscles (APRM, PPRM), which terminate within the foot (F). This structure is characterized by a distinct byssal groove (BG). The ctenidia are formed into a muscular septum, which in this drawing is indicated by dashed lines only. Into it, extend the anterior and posterior septal retractor muscles (ASRM, PSRM) and the fine lateral septal retractor (or elevator) muscles (LSRM). In Figure 12 also, the location of the pedal gape (PGA) is illustrated in relation to the orientation of the foot and, otherwise fused, mantle margin (MM).

Fig. 12. Cardiomya costellata. The organs of the mantle cavity as seen from the right side after removal of the right shell valve. The floor of the septum is indicated by dashed lines only. (For abbreviations see Box.)
Figure 13 is a ventral view of the septum (SE) of Cardiomya costellata. It is held in position by the paired anterior and posterior septal retractor muscles and is perforated by four pairs of septal pores (SEP). Antero-medially, the septum is also perforated by the foot (F), which points towards the mouth (M). The funnel-shaped mouth, also seen in Halicardia nipponensis Okutani, 1957 (Verticordiidae) (Nakazima, Reference Nakazima1967) and Poromya granulata (Poreomyidae) (Morton, Reference Morton1981b) is over-arched anteriorly by an oral hood (HM). There are no labial palps. As first described for Poromya granulata (Poromyidae) by Morton (Reference Morton1981b), the foot is responsible for pushing any captured prey item into the mouth and this function has also been attributed to the foot of Grippina coronata (Spheniopsidae) (Morton et al., Reference Morton, Machado and Passos2015).

Fig. 13. Cardiomya costellata. The septum, foot and oral hood, as seen from the ventral perspective. (For abbreviations see Box.)
In transverse section, the septum of Cardiomya costellata (Figure 14, SE) is thickly muscular being filled with the longitudinal blocks (LM) of the septal retractor muscles and held upwards by the more delicate lateral septal retractor muscles. In this illustration, the septum is divided into left and right elements by the foot and is, thereby, separated from the visceral mass by the supra-septal chamber. The foot is highly glandular, presumably a remnant of the post larval byssal gland (BGL) discharging (at one time) into a byssal groove, and penetrated by the pedal retractor muscles (PRM). Between the connections of the septum, left and right, with the mantle (MA) are ciliated rejectory tracts (RT).

Fig. 14. Cardiomya costellata. A transverse section through the septum and the foot. (For abbreviations see Box.)
THE ORGANS OF THE VISCERAL MASS
The organs of the visceral mass of Cardiomya costellata, as seen from the right side, are illustrated in Figure 15. For the sake of clarity, only the adductor muscles (AAM, PAM) are shown in this drawing. As described, the funnel-shaped mouth (M), situated internal to the anterior adductor muscle, leads into the oesophagus (O). This, in turn, opens into the stomach (ST), which is surrounded by the digestive diverticula (DD). From the postero-vental edge of the stomach arises a short crystalline style sac (CSS). Anterior and separate from this arises the mid gut (MG) that makes a simple loop in the visceral mass to become the hind gut (HG). This passes posteriorly to penetrate the ventricle of the heart (H) as the rectum (R), passes over the posterior adductor muscle (PAM) and terminates in an anus. Surrounding the intestine are the gonads (G).

Fig. 15. Cardiomya costellata. The organs of the visceral mass as seen from the right side. For the sake of clarity only the adductor muscles are shown. (For abbreviations see Box.)
The stomach architectures of the cuspidariids Cuspidaria cuspidata and Barthyneaera demistriata have been described in detail by Purchon (Reference Purchon1956, Reference Purchon1962) and Tëmkin & Strong (Reference Tëmkin and Strong2013), respectively, and that of Cardiomya costellata is little different in that, as will be described, most of its internal surface is covered by a gastric shield, and there is a small sorting area associated with two ducts (SA/ODD) leading to the digestive diverticula. The paired gonads fill the rest of the visceral mass.
THE ALIMENTARY CANAL
Figure 16A is a transverse section through the oesophagus of Cardiomya costellata. In comparison to the funnel-shaped mouth, the oesophagus, situated close to the anterior adductor muscle (AAM) is narrow, almost valve-like, with a convoluted epithelium of ciliated cells (CC). The structure is surrounded by a thick sheath of what appears to be collagen (COS) and cross-connected to the shell on each side by transverse muscle fibres (TF). The stomach (ST) is illustrated in longitudinal section in Figure 16B. It shows the separate crystalline style sac (but with no crystalline style) (CSS) and mid gut (MG). The gastric shield (GS) covers most of the inner surface of the stomach except antero-ventrally where there is a ciliated sorting area (SA). The gastric shield is located internal to a secretory epithelium (SC), which is illustrated in greater detail in Figure 16C. Here, the secretory cells some 60 µm tall, contain numerous dark-staining secretory droplets (SCD) and the gastric shield, up to 50 µm in width, appears to comprise two layers. These are (i) a proximal, ‘non-removable’, layer (GS(NR)) which Halton & Owen (Reference Halton and Owen1968) described for Nucula sulcata Bronn, 1831 as mainly comprising enzymatically active mitochondria and (ii) a thinner distal layer which the same authors described as ‘removable’ (GS(R)). Figure 16D shows a transverse section through the mid gut. It comprises six folded rows of tall ciliated cells (CC) such that the lumen is narrow.

Fig. 16. Cardiomya costellata. Transverse sections through (A) the oesophagus; (B) the stomach at the region of the openings to the crystalline style sac and mid gut; (C) a detail of the gastric shield and (D) the mid gut. (For abbreviations see Box.)
A transverse section through the crystalline style sac of Cardiomya costellata (Figure 17A, CSS) shows that it is separate from the mid gut and that it possesses a distinct ‘gutter’ (GU) as originally described for the style sac of Dreissena polymorpha (Pallas, 1771) by Morton (Reference Morton1969, figure 11). The style sac also comprises a number of epithelial types as also described for D. polymorpha and these are illustrated in Figure 17B (A, B, C and D). Most of the style sac comprises the A type cells with a centrally located nucleus and relatively stiff cilia some 15 µm long. The B type cells are taller and have a more elongate nucleus and longer (>20 µm) cilia. The C type cells abut the B cells, are shorter and make up one side of the gutter with short (10 µm) cilia. Finally, the D cells type line the other side of the ‘gutter’ and thus abut the A cells, and in C. costellata only comprise two cells (in transverse section). In neither of the sectioned individuals was a crystalline style present in the style sac. The significance of this is unknown.

Fig. 17. Cardiomya costellata. (A) A transverse section through the crystalline style sac and (B) a more detailed section of the sac's ‘gutter’. (For abbreviations see Box.)
Figure 18 illustrates transverse sections through the hind gut (Figure 18A) and rectum (Figure 18B) of Cardiomya costellata. The former has a morphology half way between the mid gut and the latter rectum in that it is mostly composed of tall convoluted ciliated epithelial cells (CC) for two-thirds of its inner circumference but these become more flattened for the remainder. The internal cavity of the hind gut contains some skeletal, chitinous, remains of prey (SRP). The rectum, in contrast, comprises a flattened and ciliated epithelium (CC) and the internal cavity is packed with prey remains (SRP).

Fig. 18. Cardiomya costellata. Transverse sections through (A) the hind gut and (B) the rectum. (For abbreviations see Box.)
THE PERICARDIUM
As illustrated in Figure 15, the pericardium of Cardiomya costellata is situated dorsally between the visceral mass and the posterior adductor muscle. Figure 19 is a transverse section through the posterior region of the body showing the septum (SE) and, above it, the heart. The pericardium (PE), situated between the fused periostracum (FP) mid-dorsally and the supra-septal chamber (SSC) ventrally, contains a central ventricle (V) that is penetrated by the rectum (R). Lateral to the ventricle are the paired auricles (AU), which contain the lightly pink pericardial gland elements within their walls.

Fig. 19. Cardiomya costellata. A transverse section through the posterior region of the body showing the septum and the heart. (For abbreviations see Box.)
THE NERVOUS SYSTEM
Morton (Reference Morton, Wilbur, Trueman and Clarke1985 Reference Mortonb) examined statocyst structure in a wide range of anomalodesmatan bivalves and showed for the Cuspidariidae that Cuspidaria suganumai Nomura, 1940 had statocysts of type C (Figure 3), as did the other examined taxa in this genus. Allen & Morgan (Reference Allen and Morgan1981, figure 8), however, showed that C. obesa had statocysts of Morton's type B1. Figure 20 shows the paired statocysts (STA) of Cardiomya costellata sitting atop the pedal ganglia (PEGA) and that they are thus of type B1. The ganglia themselves are situated between the visceral mass and the foot and held in place by transverse fibres (TF) connecting left and right sides of the foot. The arrows indicate that the statocysts are not situated equilaterally within the visceral mass. The statocysts are exceedingly small (<20 µm in diameter) and no trace of either a statolith or statoconia could be identified inside them.

Fig. 20. Cardiomya costellata. A transverse section through the paired pedal ganglia showing the similarly paired statocysts atop them. The arrows indicate that the statocysts are not situated equilaterally. (For abbreviations see Box.)
The visceral ganglia (VG) and the siphonal ganglia (SG) of Cardiomya costellata, also seen in other cuspidariids and as seen from the right side, are illustrated in Figure 21A. The visceral ganglia, as in the typical bivalve, sit beneath the posterior adductor muscle (PAM). Unlike in Cuspidaria rostrata (Reid & Reid, Reference Reid and Reid1974, figure 3) and Cardiomya planetica (Reid & Crosby, Reference Reid and Crosby1980, figure 4), however, where the ganglia are situated away from the posterior adductor muscle, those of C. costellata abut the muscle posteriorly (Figure 21A, SG and PAM). The paired siphonal ganglia are, moreover, separated by the rectum (R), which ends close to them posteriorly in an anus (AN). This empties into the supra-septal chamber (SSC) leading to the exhalant siphon. Figure 21B represents a transverse section through the siphonal ganglia (SG) showing the above arrangement of structures from another perspective. Here, the ganglia are clearly separated from each other by the rectum (R) and anus (AN), with siphonal nerves (SN) extending outwards and backwards into the siphonal apparatus. Figure 21C shows the visceral ganglia (VG) of C. costellata in transverse section showing its location below the posterior adductor muscle (PAM), with the rectum (R) situated above it.

Fig. 21. Cardiomya costellata. (A) The siphonal ganglia, as seen from the right side; (B) a transverse section through the siphonal ganglia; (C) a transverse section through the visceral ganglia. (For abbreviations see Box.)
SEXUAL EXPRESSION
There has never been a good study of the expression of sexuality in cuspidarioids. Most authors, to be identified in the discussion, consider cuspidarioids to be dioecious, although a recent study by Morton et al. (Reference Morton, Machado and Passos2015) has shown that the spheniopsid Grippina coronata is a self-fertilizing simultaneous hermaphrodite that is also ovoviporous. Figure 22A is a section through part of the gonads of Cardiomya costellata. It shows that the gonad is dominated by mature testicular follicles although there are also developing ovaries adjacent to some of the testicular lobes. This study thus suggests that C. costellata is a protandric consecutive hermaphrodite. Each follicle of the testes is enclosed within a germinal epithelium (GE) that gives rise to primary spermatocytes (PRS). These, in turn, divide again and again to produce primary and secondary spermatids (SPT) and, eventually, spermatozoa (SPE). In the adjacent ovarian follicle, again, an enclosing germinal epithelium (GE) is seen to be budding off oogonia (OO) that, in turn, are dividing to form primary oocytes (PRO) and, eventually, secondary oocytes (SO). Clearly, therefore, the male testes are mature whereas the female ovaries are at an early stage of development.

Fig. 22. Cardiomya costellata. (A) Sections through a lobe of the mature testes and through a single, developing, ovarian follicle. (B) A primary spermatocyte; (C) a secondary spermatocyte; (D) a spermatid; (E) a spermatozoa and (F) an oogonium beginning its division, with a forming first polar body, into a primary oocyte. (For abbreviations see Box.)
Figure 22A, B and C shows a primary spermatocyte (B), a secondary spermatocyte (C), a spermatid (D) and a spermatozoan (E) with an elongate head and a double tail. Figure 22F shows a primary oocyte of C. costellata, which is seen to be budding off from the germinal epithelium and undergoing meosis that will eventually result in secondary oocytes and the first polar body. There is no evidence for parental larval brooding by either C. costellata or any other cuspidariid, unlike in the spheniopsid Grippina coronata (Morton et al., Reference Morton, Machado and Passos2015).
DISCUSSION
The pronounced ribbing of the Cardiomya costellata shell undoubtedly confers strength to an otherwise brittle and fragile structure and is particularly important in relation to shell adduction. This is because the valve margins do not ventrally meet everywhere, meaning that strong adduction pressures could crack the shell along its left and right lines of greatest weakness. This is particularly true postero ventrally and, here, an internal buttress in each valve that extends from mid-dorsally to the postero-ventral margins of each valve additionally strengthens the shell. Such structures have been reported upon earlier for a number of other bivalves including all species of the anomalodesmatan genus Laternula, for example Laternula truncata (Lamarck, 1818) (Morton, Reference Morton1973) and the burrowing arcoid Cucullaea concamerata G.B. Sowerby II, 1869 (=C. petita Iredale, 1939) (Morton, Reference Morton1981c). In each case, the posterior adductor muscle is situated internal to the buttress such that when it contracts the pressure lines on the shell change from a mid-dorso-ventral axis to a mid dorsal postero-ventral one with the maximum stresses on the shell valves matching the lines taken by the buttresses, as described in detail for L. truncata (Morton, Reference Morton1973).
The ligament of Cardiomya costellata is typical of other cuspidariids in that it is internal and characterized by a lithodesma, which, effectively, restricts the extent of valve gape when the adductor muscles relax. Typically, cuspidariids are considered to lack hinge teeth, as do most anomalodesmatans (Morton, Reference Morton1981a, Reference Morton, Wilbur, Trueman and Clarke1985a) but Tebble (Reference Tebble1966) records, as does this study, a small, ineffective posterior lateral hinge tooth on the right valve. Ineffective because there is no definable socket for it to interlock with. Bernard (Reference Bernard1974, figure 4) illustrated the same hinge plate structures for a variety of cuspidariids.
As in other cuspidariids, as so clearly illustrated by Reid & Reid (Reference Reid and Reid1974) and Reid & Crosby (Reference Reid and Crosby1980) for Cardiomya planetica, the siphonal apparatus of Cardiomya costellata is adapted for the capture of small swimming epipsamic, probably crustacean, prey. Like other cuspidariids too, C. costellata has the same array of seven siphonal papillae that in the possession of clusters of apical cilia are clearly sensory mechanoreceptors, which detect possible prey. As also described by Reid & Reid (Reference Reid and Reid1974), the mantle and siphons of C. costellata all possess extensive haemocoels, which through their compression upon adduction are responsible for the rapid eversion of the inhalant siphon to capture prey. Finally, the siphonal sheath of C. costellata, as in some other cuspidariids (Oliveira & Sartori, Reference Oliveira and Sartori2014), possesses a distal array of arenophilic radial mantle glands that produce secretions, which allow the adhesion of sand grains to this structure thereby assisting in the camouflaging of the siphons, when retracted. Camouflage possibly from predators, but also so that the siphonal apertures are indistinguishable from the surrounding sediments by any passing potential prey.
The organs of the mantle cavity of Cardiomya costellata are described in detail and, as in many other cuspidariids (Allen & Morgan, Reference Allen and Morgan1981), the septum comprises four pairs of septal pores and a central anterior opening for the foot to be extended into the infra-septal chamber to: (i) effect burrowing and (ii) as proposed by Morton (Reference Morton1981b) for Poromya granulata, push captured prey into the funnel-shaped mouth. There are no labial palps, although other cuspidariids do possess, albeit extremely reduced, such structures (Bernard, Reference Bernard1974; Allen & Morgan, Reference Allen and Morgan1981). The numbers of septal pores in the different families of the Cuspidarioidea are different, sometimes between species of the same genus as described and illustrated for Octoporia taxa (Halonymphidae) by Krylova (Reference Krylova1994, figure 3). Species of Bathyneaera, like those of Cuspidaria (Pelseneer, Reference Pelseneer1888, figures 1–4) and Cardiomya (Allen & Morgan, Reference Allen and Morgan1981, this study), seem to consistently possess four pairs of septal pores (Krylova, Reference Krylova1993, figure 2D). Fascinatingly, species of the Protocuspidariidae Scarlato and Starobogatov, 1983 (Allen & Morgan, Reference Allen and Morgan1981; Krylova, Reference Krylova1995) have a short rostrum, the typical cuspidariid array of seven siphonal papillae, but possess a unique septum that is partly filamentous and partly septal and, thus, intermediate between the typical verticordiid ctenidia and cuspidariid septum.
The stomach of Cardiomya costellata conforms to the structure seen in other cuspidariids (Purchon, Reference Purchon1956; Tëmkin & Strong, Reference Tëmkin and Strong2013). There is an extensively cuticularized area, the gastric shield, with ventral openings to: (i) the crystalline style sac; (ii) the mid gut; and (iii) two openings left and right to the digestive diverticula associated with a central sorting area comprising a folded ciliated epithelium. The gastric shield of Nucula sulcata was shown by Halton & Owen (Reference Halton and Owen1968, plate 8) to comprise two layers: firstly, an internal ‘non removable’ layer mainly composed of microvilli arising from the secretory cells underlying it and a matrix of non-cellular material which fills the spaces between the microvilli, and, secondly, an external ‘removable’ layer, composed exclusively of microvilli and chitin. McQuiston (Reference McQuiston1970) showed that the fine structure of the gastric shield of Lasaea rubra (Montagu, 1803) (=L. adansoni [Gmelin, 1791]) was essentially the same as that of N. sulcata, except that no outer ‘removable’ layer was identified. It seems possible that such a layer might be formed and lost with the periodic dissolution and re-formation of the crystalline style revolving against it, but this is unknown. It is, moreover, the case that a crystalline style has rarely been recorded from representatives of the Septibranchia (Morton, Reference Morton2003, Table 1), although this structure was present in specimens of Grippina coronata investigated by Morton et al. (Reference Morton, Machado and Passos2015, figure 16).
Table 1. An anatomical comparison between Grippina coronata (Spheniopsidae) and Cardiomya costellata (Cuspidariidae). After Morton et al. (Reference Morton, Machado and Passos2015) and this study, respectively.

As in other cuspidariids, for example, Bathyneaera demistriata Tëmkin & Strong (Reference Tëmkin and Strong2013), the style sac of Cardiomya costellata is separate from the mid gut and has the complex structure first identified for Dreissena polymorpha by Morton (Reference Morton1969). In neither sectioned individual examined herein was a crystalline style present. Morton et al. (Reference Morton, Machado and Passos2015) showed how the stomach of the spheniopsid Grippina coronata possessed a waste storage pouch wherein the skeletal remains of an individual's prey were stored until death. This was considered analogous, possibly homologous, with the left pouch characteristic of many heterodont bivalves (Purchon, Reference Purchon1958, Reference Purchon1987). The thin intestine did end in an anus in this species, but this was considered to only function in the passage and release of digested waste. Unlike G. coronata, the cuspidariid Cardiomya costellata does not have a waste storage pouch (this study). Instead, the exoskeletal remains of its prey are passed in the normal bivalve manner towards the anus. In the rectum, as illustrated in Figure 18B, large numbers of exoskeletal remains are present and it seems possible that they may remain here until death – as with G. coronata. This is because prey capture must be sporadic and, therefore, undigested remains should logically be evacuated from the anus one by one. The fact that the skeletal remains of many prey items are packed together in the rectum suggests that this is not the case.
This possibility arises, particularly in relation to the Açores, because here Cardiomya costellata reaches a shell length of only <6.5 mm but, as Figure 1 illustrates, the population structure appears unimodal, That is, this species, at least in this location, may be so short-lived, that the regular anal discharge of prey remains is unnecessary. There is another aspect to this argument. That is, although C. costellata only attains a small shell length in the Açores that is half that of mainland conspecifics (as do virtually all bivalve taxa here; Morton et al., Reference Morton, Tristão da Cunha and de Frias Martins2013), so do its potential prey, such as epipsammic amphipods, which are also far smaller than their mainland European conspecifics (A.C. Costa, personal communication). That is, life in the nutrient-poor waters of this remote archipelago has become miniaturized, so that although this cuspidariid predator is small so is its prey. As a consequence, and because there is no evidence from this or any other cuspidariid with regard to the timing of the onset of reproduction, all we can speculate is that here in the Açores, either growth is reduced or longevity is compressed – or perhaps both. Regrettably, there is no information either on the population structure, growth or longevity of any other cuspidariid from elsewhere.
Cuspidariids are generally considered to be dioecious (Bernard, Reference Bernard1974) (Table 1). This is unusual for the Anomalodesmata where all other taxa from every studied family are considered to exhibit some expression of hermaphroditism, although the details of this varies between superfamilies (Morton, Reference Morton1981a, Reference Morton, Wilbur, Trueman and Clarke1985a). Morton et al. (Reference Morton, Machado and Passos2015) showed that the spheniopsid Grippina coronata was a simultaneous hermaphrodite conforming, therefore, to the generalized picture of anomalodesmatan sexual expression. Grippina coronata was, however, shown to be unusual in that the testes and ovaries develop simultaneously in the same follicles and that embryos are not released until death of the parent. Grippina coronata was again shown to be unusual in that it attains only a maximum shell length of <~3 mm and, thus that post mortem not only are the exoskeletal remains of the prey released but so are embryos.
These observations therefore raise new implications with regard to the significance of size in relation to sexual expression. For example, this study suggests, in contrast to other studies of the Cuspidariidae, that Cardiomya costellata is a protandrous consecutive hermaphrodite. But, it also shows that in the Açores it only attains a shell length of half that of mainland European, even Mediterranean (Poppe & Goto, Reference Poppe and Goto1993), conspecifics. This raises the interesting possibility that here on these remote islands, the female phase is rarely attained. Similarly, with smaller prey, that is equivalent for them of an energetically less valuable consumption per unit of prey capture, raises the possibility that individuals of C. costellata rarely obtain a size when they would become a mature female. Morton et al. (Reference Morton, Tristão da Cunha and de Frias Martins2013) suggested that, in the Açores, not only was bivalve size and diversity low, but so were individual numbers for all species. No comparative data on this aspect of these island's biology was obtained. It is nevertheless true that if such, at least deeper water, bivalves are less common than on mainland continental shelves then so might be their food resources. In the case of C. costellata, epipsammic micro-crustacean prey. If so, such reduced productivity would explain why here not only are all benthic bivalves small but also uncommon. Again in the particular case of C. costellata, this would help explain why this protandric simultaneous hermaphrodite was so small but also so rare – individuals are simply less likely to mature into females. Hypothetically, if the species was a protogynous consecutive hermaphrodite, the situation would be different, that is, eggs would be more likely to be fertilized. Finally, unlike G. coronata, C. costellata does not brood fertilized eggs. So, again unlike G. coronata, C. costellata does not possess this compensatory mechanism for enhancing reproductive success; instead it is here, in the remote Açores, literally surviving at the edge.
This more comprehensive study of a deep-water cuspidariid has allowed a more detailed comparison of the Cuspidariidae with its sister family the Spheniopsidae whose features have been elaborated upon by Morton et al. (Reference Morton, Machado and Passos2015) (Table 1). From this table, it can be seen that the two sister families share many features – not the least of which is that they are both predatory carnivores. They differ, firstly, in a number of respects: the shell of Grippina coronata is not rostrate which reflects the fact that it feeds on prey taxa living closer to the sea floor, unlike cuspidariids capturing swimming prey. It also has a different ligament structure – although like cuspidariids it possesses a lithodesma. Both possess a byssal groove and gland in the foot, but no threads are present in adults. This suggests that a byssus – probably only one or two threads – is a post-larval feature of them both. Siphonal and sensory papilla structure are similar except that G. coronata does not have a long protrusible inhalant siphon to catch its prey – again reflecting the differences in prey characteristics. Associated with this, G. coronata does not possess a secondary pair of siphonal ganglia – unlike in all hitherto studied cuspidariids. Arenophilic glands are present in C. costellata but not G. coronata. The septa of both taxa are near identical with four pairs of septal pores and there are no functional labial palps. Stomach structure in both taxa is similar except G. coronata has a short conjoined style sac and mid gut whereas in C. costellata the crystalline style sac is long and separate from the mid gut. The most significant difference between the two taxa, however, is that G. coronata possesses a waste storage pouch for the retention of the exoskeletal remains of prey whereas in C. costellata such remains are possibly stored in the rectum. In both, the intestine is short and complete, that is, both have an open anus.
The most significant differences between the two taxa relate to sexual expression and embryo brooding. Grippina coronata is a simultaneous hermaphrodite, gonads developing in the same follicles and with self-fertilized embryos brooded therein also. Mature oocytes are telolecithal and have a complex structure similar in detail to that described for Entodesma cuneata (Gray, 1828) (Lyonsiidae (Fischer, 1887)) (Campos & Ramorino, Reference Campos and Ramorino1981). Conversely, Cardiomya costellata is a protandric, non-brooding, consecutive hermaphrodite. Nothing is known of the early life history of any cuspidariid. The life history features of G. coronata, however, equip it for a short, carnivorous existence in the deep sea with the exoskeletal remains of prey and brooded embryos being released post mortem. It thus exhibits post-mortem semelparity (Morton et al., Reference Morton, Machado and Passos2015). The reproductive strategy of C. costellata in the Açores is complicated in this special location because here it may only rarely, because of its protandric expression of hermaphroditic sexuality, achieve reproductive success. What is needed now is a study of this species in the less productivity depauperate components of its range and other studies of cuspidariid reproduction.
ACKNOWLEDGEMENTS
I thank Dr Sanja Puljas, University of Split, Croatia, for assistance with the sectioning of two of the specimens herein reported upon.
FINANCIAL SUPPORT
I am grateful to Professor A.M de Frias Martins and Dr Joana Xavier for funding the research visit to the Açores in July 2011 during which the fieldwork for this research was undertaken and for much practical help and warm hospitality during my stay on São Miguel.