INTRODUCTION
Representatives of the Pinnoidea Leach, 1819 (Pterioida Beurlen, 1944) with a single family, the Pinnidae, are variously known colloquially as either pen shells or fan shells and comprise three extant genera: Pinna Linnaeus, 1758, Atrina Gray, 1842 and Streptopinna von Martens, 1880. With planktonic larvae (Allen, Reference Allen2011), representatives of the Pinnidae are distributed widely throughout the warmer oceans of the world but have their greatest diversity in the tropics (Lemer et al., Reference Lemer, Buge, Bemis and Giribet2014, Reference Lemer, Combosch, Sotto, Giribet and Dumale2016). With a fossil history dating back to the Lower Carboniferous (Cox & Hertlein, Reference Cox, Hertlein and Moore1969), such bivalves are characterized by a thin, brittle, equivalve shell, often with radiating riblets and a fluted ornamentation and into which the contained animal can retreat deeply. The shell lacks any form of dentition and the sub-internal ligament extends along almost the entire length of the dorsal shell margin. Each individual is positioned vertically, typically within offshore seagrass-associated sediments, and to the constituents of which it attaches by a large byssus comprising many fine, silken, threads. These were once of significant economic importance (Maeder, Reference Maeder2002).
Internally, representatives of the Pinnidae are characterized by two adductor muscles, the posterior larger than the anterior and associated with which are paired pedal retractor muscles, again the former considerably larger than the latter. Species are also characterized by two ‘glands’: a single pallial gland (but herein re-defined as a buccal gland) anterior to the mouth and an also single pallial organ posterior to the ligament (Stenta, Reference Stenta1906; Grave, Reference Grave1911; Pelseneer, Reference Pelseneer1911; Yonge, Reference Yonge1953; Liang & Morton, Reference Liang and Morton1988).
This is a study of the largest fan shell Pinna nobilis Linnaeus, 1758, which can reach a shell length of >100 cm but is more usually 20–80 cm (Poppe & Goto, Reference Poppe and Goto1993). Pinna nobilis is endemic to the Mediterranean, the Adriatic Sea (Šiletić & Peharda, Reference Šiletić and Peharda2003; Šiletić, Reference Šiletić2006) and the Dardenelles (Palaz & Selcuk, Reference Palaz and Selcuk2005) where it lives offshore at depths from 0.5–60 m and occurs, partially buried vertically, within areas of softer sediment often associated with beds of the seagrass Posidonia oceanica (L.) Delille and mixed meadows of this species and Cymodocea nodosa (Ucria) Ascherson, 1870 (Zavodnik, Reference Zavodnik1967; Zavodnik et al., Reference Zavodnik, Hrs-Brenko, Legac, Boudouresque, Avon and Gravez1991). High density values similar to those recorded by Theodorou et al. (Reference Theodorou, James, Tagalis, Τzοvenis, Hellio and Katselis2017) for P. nobilis from the shallow waters of Maliakos Gulf in Greece (19–50 ind. 100 m−2), have been identified for the Adriatic Sea (Šiletić & Peharda, Reference Šiletić and Peharda2003). Further, Šiletić (Reference Šiletić2006) showed that P. nobilis is distributed widely in the Croatian Adriatic with densities of 2–20 ind. 100 m−2 being recorded from the marine Mljet National Park.
In recent years P. nobilis has become endangered (Katsanevakis, Reference Katsanevakis2006; Katsares et al., Reference Katsares, Tsiora, Galinou-Mitsoudi and Imsiridou2008) due, in part, to fishing, trawling, boat anchoring, over-collecting by scuba divers and the Mediterranean-wide decline in seagrass beds. As a consequence, P. nobilis is listed under the European Council Habitats Directive 92/43/EEC on the Conservation of Natural Habitats and Wild Fauna and Flora. The directive states that P. nobilis is strictly protected under Annex IV of EEC 1992 from all forms of deliberate collection and killing. Accordingly, permission was obtained from the Ministry of Environment and Energy of the Croatian Government, Radnička cesta 80, Zagreb, Croatia (Permission number UP/I-612-o7/17-48/95; 517-07-1-1-17-4), to collect the individuals of P. nobilis herein reported upon from Croatian waters in order to examine the morphology, particularly, of the species’ pallial organ, buccal gland, its stomach and contents. This is because, despite the earlier observations identified above pertaining to the first two structures, there are still questions remaining regarding the functions of these organs that are unique to representatives of the Pinnidae.
Liang & Morton (Reference Liang and Morton1988) investigated the pallial organ of Atrina pectinata (Linnaeus, 1767) but did not examine other details of the species’ anatomy although, as identified above, those of other taxa have been described principally by Grave (Reference Grave1911) and Yonge (Reference Yonge1953). This, therefore, is primarily a study of P. nobilis but in order to complete a generic comparison with A. pectinata in relation to this organ and the buccal gland (if present), two individuals of this species have been obtained from Hainan Island, China, and will also be reported upon herein. Finally, a comparison has been made of the stomach of P. nobilis with those of Atrina vexillum (Born, 1778) and Pinna atropurpurea G. B. Sowerby I, 1825 described and illustrated by Purchon (Reference Purchon1957).
Abbreviations:
AAM, Anterior adductor muscle; AMC, Acidic mucopolysaccharide cell; AN, Anus; APON, Anterior pallial organ nerve; APRM, Anterior pedal retractor muscle; ASC, Acid crystal-secreting cell; ASE, Acid-secreting epithelium; BG, Byssal groove; BP, Basal pouch; BUG, Buccal gland; BUGD, Buccal gland duct; BUGP, Buccal gland pore; BY, Byssus; CC, Ciliated cell; CEO, Cut edge of oesophagus; CH, Central haemocoel; CI, Cilia; CM, Circular muscle; C-P-V-C, Cerebro-pleural – visceral connective; CS, Crystalline style; CSS/MG, Conjoined style sac and mid gut; CTN, Ctenidial nerve; DD, Digestive diverticulae; DDD1–3, Ducts to the digestive diverticulae1–3; DH, Diffuse haemocoel; DOH, Dorsal hood; EC, Epithelial cell; F, Foot; GS, Gastric shield; H, Heart; HG, Hind gut; ID, Inner demibranch; IG, Intestinal groove; ILM, Inner lip of the mouth; ILP, Inner labial palp; INC, Inferior channel; K, Kidney; KN, Kidney nerve; LH, Lateral haemocoel; LP, Left pouch; M, Mouth; MAT, Major typhlosole; MC, Mucous cell; MI, Microvilli; MIT, Minor typhlosole; MM, Mantle margin; N×3, Three nerves of unknown role; ND, Nerve division; NMC, Neutral polysaccharide cell; O, Oesophagus; OD, Outer demibranch; OE, Outer epithelium; OLM, Outer lip of the mouth; OLP, Outer labial palp; OP, Oesophageal pleats; PAM, Posterior adductor muscle; PAM(1), Posterior component of the posterior adductor muscle; PAM(2), Anterior component of the posterior adductor muscle; PAMN, Posterior adductor muscle nerve; PN1,2,3,5, Pallial nerves 1,2,3,5; PO(H), Pallial organ (head); PO(S), Pallial organ (stalk); POG, Proximal oral groove; POGV, Proximal oral groove valve; PPON, Posterior pallial organ nerve; PPRM, Posterior pedal retractor muscle; PT, Primary tubules; R, Rectum; RM, Retractor muscle; SA, Sorting area; SE, Sub-epithelial layer; SLT, Secretions in the lumen of the tubule; ST, Secondary tubules; SUC, Superior channel; SUC(I), Superior channel (Inner flank); SUC(O), Superior channel (Outer flank); V, Vacuole; VA, Valvular separation; VC, Vacuolated cell; VG, Visceral ganglion; VGC, Visceral ganglia connective; VM + MG, Visceral mass and mid gut.
MATERIALS AND METHODS
Permission was given for a total of 15 P. nobilis individuals to be collected, five from each of three size classes classified as ‘small’ with shell lengths in the range of 18.5–26.5 cm, ‘medium’ 34–49 cm and ‘large’ 56–69 cm following an earlier study by Davenport et al. (Reference Davenport, Ezgeta-Balić, Peharda, Skejić, Nincevi, Ninčević-Gladan and Matijević2011). The authorized individuals of P. nobilis were collected from Kaštela Bay, Croatia, during the early mornings of May 2017 using scuba at depths of 2–4 m. Water temperature and salinity were measured with a hand-held held multimeter (Mettler Toledo X-matePro MX300). The average temperature recorded at the surface of the water column on the collection days was 17.5 °C, while the salinity was 32‰.
The 15 individuals of P. nobilis were examined alive and observations made on and photographs taken of the behaviour of their pallial organs. Subsequently, the pH values of the stalk and head of this organ of the 15 P. nobilis were measured using litmus papers and average values for each obtained. The pallial organs were subsequently excised from five of these individuals and given three consecutive baths in 3.2% sodium chloride. The organs were then immersed in 1.0% barium chloride for 10, 20, 30, 40, 50 and 60 min and finally fixed in 10% formaldehyde. Control individuals were not bathed in barium chloride. In the presence of sulphuric acid, as identified earlier using this technique for A. pectinata (Liang & Morton, Reference Liang and Morton1988), barium chloride is converted to barium sulphate, the hyaline crystals of which shine under phase contrast microscopy (Thompson, Reference Thompson1983). Longitudinal sections of these pallial organs were lightly counterstained in eosin and, accordingly, examined under phase contrast microscopy for the presence and distribution of barium sulphate crystals in their tissues and cells.
The food sources and diet of P. nobilis were examined by a microscopic analysis of the stomach contents of 10 of the freshly collected individuals. Their contents were collected by transversely cutting the stomachs of ‘medium’ and ‘large’ individuals (see above) and flushing them out. Stomach contents were preserved in 70% ethanol and these were examined subsequently under a binocular photomicroscope (Olympus BX60) and any discovered species identified to the lowest possible taxonomic category.
Subsequently, the tissues of all 15 individuals were preserved in 4% formalin and later dissected to illustrate aspects of overall morphology but with especial attention being paid to the anatomy of the pallial organ, the buccal (formerly pallial) organ and the stomach but also the visceral ganglia.
Two pallial organs were excised from the 15 formalin-preserved individuals and, following routine histological procedures, were transversely and longitudinally serially sectioned at 6 µm and the resulting slides stained in Ehrlich's haematoxylin and eosin. Similarly, the dissected buccal organ of two ‘medium’ sized (see above) individuals of P. nobilis was also sectioned at 6 µm and alternate resulting slides stained in either Ehrlich's haematoxylin and eosin or Alcian blue/PAS (Periodic Acid Schiff), the latter to determine the presence or not of Alcian Blue-positive (blue sulphated polysaccharides and acid mucopolysaccharides) and PAS-positive (magenta neutral mucopolysaccharides) secretory cells in the various elements of the pallial gland. Accordingly, the slides were stained with Alcian blue for 15 min. After washing in distilled water, the sections were treated with periodic acid for 10 min and then with Schiff's reagent for 10 min.
Finally, the presence of a buccal gland in two individuals of A. pectinata obtained from Hainan Island, China, has been investigated for comparison with that of P. nobilis.
Voucher specimens of the two species herein investigated have been lodged in the collections of the Natural History Museum, London, where they have the registration numbers: Atrina pectinata (Linnaeus, 1767) registration number NHMUK 20170389/1-2; Pinna nobilis Linnaeus, 1758 registration number NHMUK 20170390 (one shell) and NHMUK 20170391/1-3 (tissues).
RESULTS
Anatomy
The organs of the mantle cavity of P. nobilis, as seen from the right side after removal of the left shell valve and mantle are illustrated in Figure 1. In their relaxed condition, the mantle margins (MM) of P. nobilis extend almost to the posterior edge of the shell, as do the long paired ctenidia (ID, OD). The major muscles are the posterior adductor (PAM) and its much smaller anterior equivalent (AAM). Anterior to the former are the large paired posterior pedal retractor muscles (PPRM), again with much reduced anterior equivalents (APRM).
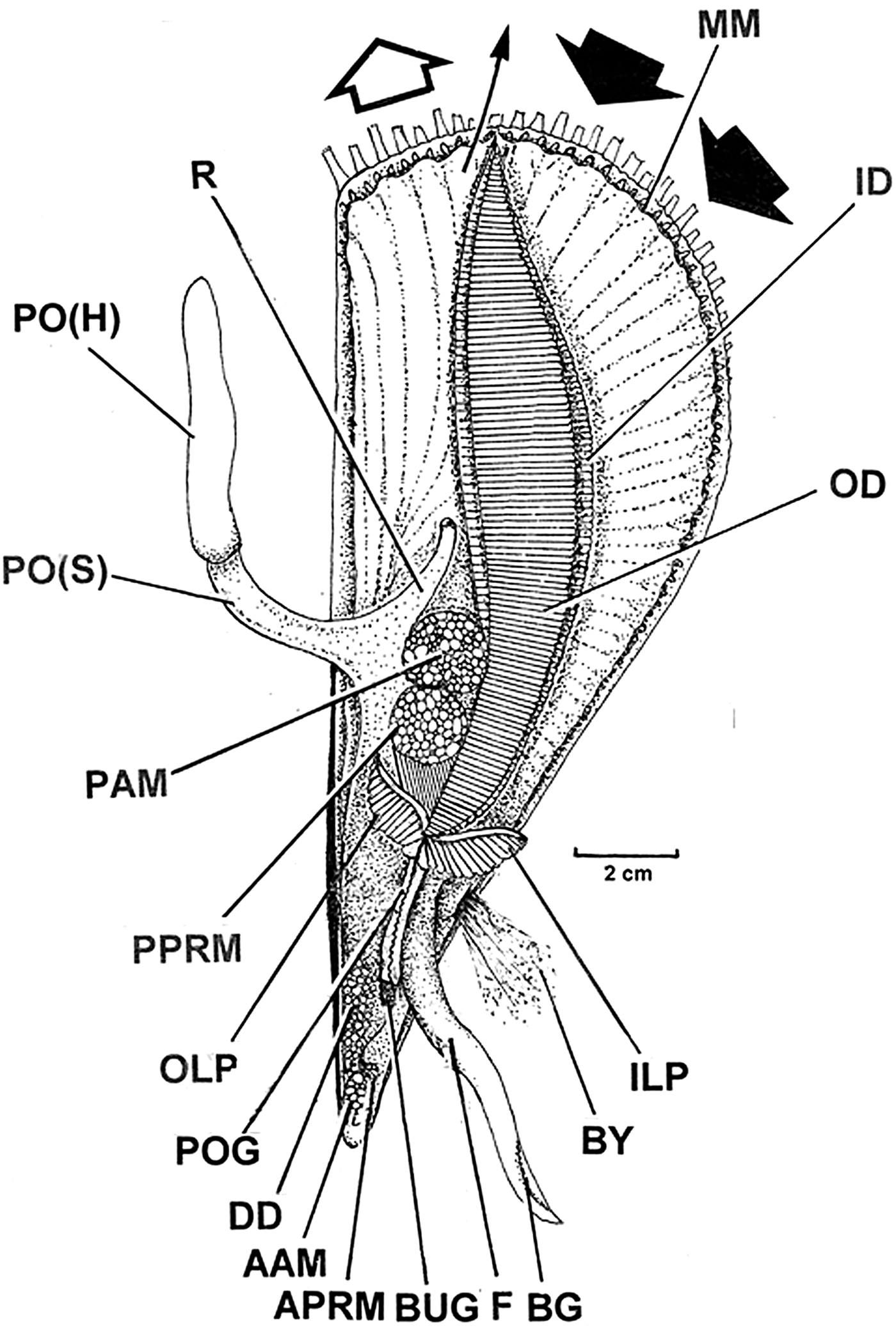
Fig. 1. Pinna nobilis. The organs of the mantle cavity as seen from the right side after removal of the left shell valve and mantle. Large arrows indicate the inhalant (dark) and exhalant (open) currents and the small arrow indicates the expulsion of faeces from the anus. (For abbreviations see Box.)
The pinnid ctenidia have been described for P. nobilis and four other taxa by Ridewood (Reference Ridewood1903) and in detail for Pinna (=Atrina) fragilis Pennant, 1777 by Atkins (Reference Atkins1938, pp. 419–420, plate 29). The ctenidia terminate in a pair, left and right, of relatively large labial palps (OLP, ILP). Stasek (Reference Stasek1963) did not describe the ctenidial-labial palp junction of any pinnid but it is most like that of other members of the Pterioidea (Category III) as will be described. The labial palps pass their products of food selection into, again left and right, proximal oral grooves that end at the mouth. Anterior to this is a single large buccal gland (BUG). Antero-ventrally there is a large foot (F) arising from the visceral mass that contains the intestine including the anteriormost digestive diverticula (DD) and which, as will be seen, surround the stomach. From the base of the foot arises the stout, finely fibrous, byssus. This is secreted by a byssal gland also in the visceral mass and the secretions from which pass down the foot within the ventral byssal groove (BG) and where, at its tip, they are planted onto elements of the species’ habitat, that is, sand grains, shell debris and small stones.
In Figure 1 too, the large arrows indicate the inhalant (dark) and exhalant (open) currents and the small arrow indicates where the expulsion of faeces takes place from the anus. Here too is illustrated the pallial organ (PO(H) and PO(S)) in a semi-extended state. The pallial organ arises from the dorsal mantle and is united with the rectum (R), which terminates in an anus.
The pallial organ
The pallial organ of P. nobilis is illustrated in Figure 2. It is also illustrated in Figure 3A in its fully extended condition. It comprises two major parts: a proximal stalk (PO(S)) and a distal head (PO(H)). The elongate head is connected by a short neck with the longer stalk and which is united, in turn, with the mantle tissues at its base by a sphincter.

Fig. 2. Pinna nobilis. A photograph of the pallial organ showing the contracted sphincter at its base.
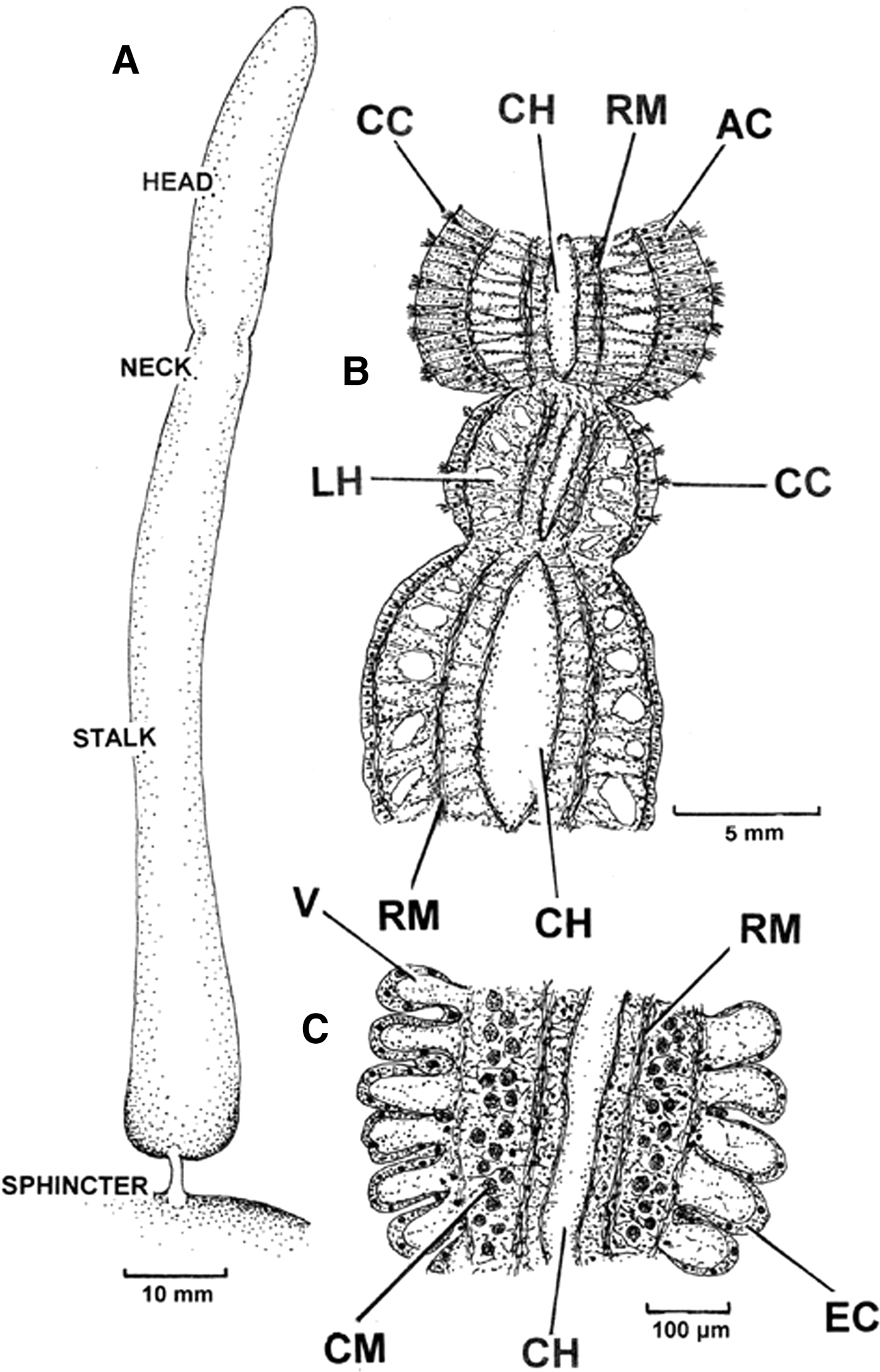
Fig. 3. Pinna nobilis. A, The pallial organ in its fully extended condition. B, A longitudinal section through the stalk and head of the pallial organ showing the connection between the two and C, a longitudinal section through the sphincter at the base of the organ. (For abbreviations see Box.)
A longitudinal section through the stalk and head of the pallial organ (Figure 3B) shows the connection between the two. Histologically, the stalk of the pallial organ comprises a cuboidal epithelium. There is a capacious central haemocoel (CH) that is enclosed within a lateral haemocoel (LH), which is divided into inner and outer elements by longitudinal retractor muscle fibres (RM). The neck region has a similar structure, except the epithelium also bears ciliated cells (CC). Such cells also occur in the head region of the pallial organ, except here they intersperse between exceptionally tall (>1000 µm) and, as will be seen, acid-secreting cells (AC). Figure 3C is a longitudinal section through the sphincter at the base of the pallial organ. In this region, the enclosing epithelium (EC) comprises cuboidal cells that lie above a highly vacuolated (V) haemocoel. There are here also more well-developed circular muscles (CM) such that when they contract and the longitudinal retractor muscles relax, the sphincter is tightened resulting in a narrowing of the central haemocoel (CH) and the vacuolated areas beneath the epithelium. This is in coordination with blood being forced upwards by hydrostatic forces operating upon haemocoels in the body. Such movements extend the pallial organ to its fullest extent. Only when the sphincter is relaxed does the organ deflate as blood can now flow back from its haemocoels into those of the body.
Transverse sections through the head of the pallial organ of P. nobilis show the entire structure (Figure 4A). As noted earlier, the outer epithelium is ~1000 µm tall and comprises shorter (~80 µm) cilated cells (CC) that are interspersed with and, as will be shown soon, acid-secreting cells. Here, the central haemocoel (CH) is well defined and surrounded by delicate retractor muscles (RM). Around the central haemocoel is a large and diffuse haemocoel (DH) with fine radial muscles interspersed within it. The central region of the pallial organ head is shown in more detail in Figure 4B and illustrates the central haemocoel and ring of retractor muscles. The outer epithelium is illustrated in yet greater detail in Figure 4C and shows the distal triangular ciliated cells approximately interspersing acid crystal-secreting cells (ASC). A few epithelial cells (EC) are possibly newly developing acid crystal-secreting ones.

Fig. 4. Pinna nobilis. Transverse sections through the head of the pallial organ showing A, the entire structure; B, a detail of the central haemocoel and ring of retractor muscles; C, the outer epithelium showing the acid-secreting cells. (See Figure 3 for an indication of where the sections were cut from the pallial organ.) (For abbreviations see Box.)
The pH values of the pallial organ stalk of P. nobilis were consistently pH 7. Those for the head were pH 2 for 11 of the 15 individuals and pH 1 for the other four, giving an overall average value of 1.73. The pallial organ head is thus highly acidic. Lightly eosin-stained sections of the pallial organ head of P. nobilis following soaking in barium chloride for a maximum period of 60 min are illustrated in Figure 5. Sections of control slides (Figure 5A) of a head unbathed in barium chloride did not demonstrate the presence of sulphuric acid. In the experimental heads, however, with time, the rate of barium chloride penetration increased leading to the deposition of successively more and more deeper and larger barium sulphate crystals (Figure 5B–D). With longer barium chloride soak times it is obvious that the apices of the tall epithelial cells and their bases show successively greater incidences of the hyaline crystals of barium sulphate. That is, the epithelial cells secrete sulphuric acid as demonstrated for A. pectinata by Liang & Morton (Reference Liang and Morton1988).

Fig. 5. Pinna nobilis. Lightly eosin-stained sections of the pallial organ head following soaking in barium chloride. A, control; B, after 20 min; C, after 40 min and D, after 60 min, showing a successively greater incidences of barium sulphate hyaline crystals.
The visceral ganglia
The pallial organ of P. nobilis (PO(H), PO(S)) is illustrated in Figure 6 from the right side and shows the posterior musculature, that is, the posterior adductor muscle, which is weakly divided into two elements: fast (PAM(1)) and slow (PAM(2)). Anterior to it are the large posterior pedal retractor muscles, the two structures being separated and partially enclosed ventrally by the paired kidneys (K). Beneath these muscles too are the also paired ctenidia (ID, OD), while arching above them is the rectum (R) ending in an anus (AN). The rectum traverses the ventricle of the heart (H). The anatomical features of the intestine will be described later. Importantly, however, Figure 6 shows the position of the visceral ganglia (circle).

Fig. 6. Pinna nobilis. The posterior musculature and pallial organ, as seen from the right side, showing the position of the visceral ganglia (circle). (For abbreviations see Box.)
The first and only person to investigate the pinnid visceral ganglia was Grave (Reference Grave1911, Figure 11, pp. 433–434) who studied Atrina rigida (Lightfoot, 1876). Figure 7 illustrates the visceral ganglia of P. nobilis and their nerves as seen from the ventral aspect. The visceral ganglia lie on the antero-ventral edge of the posterior adductor muscle (PAM) and posterior to the kidneys (K) and the visceral mass with its enclosed mid gut (VM + VG). The visceral ganglia are connected anteriorly, left and right, to the cerebral ganglia (C–P–V–C) and to each other by the visceral ganglia connective (VGC). Paired nerves arising from the anterior borders of the ganglia include those to the anterior region of the pallial organ (APON) and three, small, others (N×3) of unknown function. Laterally from the ganglia arise large nerves leading to the ctenidia (CTN). Dorsally, nerves (PAMN) lead to the posterior adductor muscle (PAM). Posteriorly, five pairs of nerves arise from the ganglia to the mantle (PN1–5) and of these the second (PN2) is the largest. There are also a pair of nerves (PPON) leading to the posterior region of the pallial organ (PPON).

Fig. 7. Pinna nobilis. The visceral ganglia and their nerves as seen from the ventral aspect. (For abbreviations see Box.)
The buccal gland
Figure 8 is a detailed illustration of the location of the buccal gland in relation to the foot, the labial palps and the proximal oral grooves of P. nobilis. In this figure the large byssus has been cropped so as not to obscure otherwise hidden structures. The paired ctenidia (ID, OD) terminate in a relatively large pair of labial palps – inner (ILP) and outer (OLP). These fulfil the function of suspended potential food sorting prior to ingestion as is typically characteristic of the suspension-feeding Bivalvia. Each pair of labial palps in turn leads anteriorly to a proximal oral groove (POGV), that is swollen proximally. The proximal oral grooves are long and extend over the visceral mass (VM) from which the foot (F) and its byssal groove (BG) extend towards the mouth (M). In addition to them being unusually long, the proximal oral grooves are divided into two channels, one above the other. The basal, or inferior, channel (Figure 8A, INC) has a simple structure and is separated from the upper, superior, channel by slightly flattened ridges. The superior channel is open and comprises inner (SUC(I)) and outer (SUC(O)) flanks. These are delicately folded and ridged and in Figure 8A they have been opened out to expose the inferior channel below them and their ridged form. Figure 8B is a diagrammatic illustration of how, in life, the two components of the proximal oral grooves, superior (SUC) and inferior (INC), are separated by a valve-like ridge (VA). The paired proximal grooves lead to the mouth (M), the lips of which are also complexly folded and pleated. Anterior to the mouth is the buccal gland (BUG) which sits atop the small paired anterior pedal retractor muscles (APRM).

Fig. 8. Pinna nobilis. A, A detailed illustration of the location of the buccal gland in relation to the foot, the labial palps and the proximal oral grooves (the large byssus has been cropped short). B, A digrammatic illustration of how the proximal oral groove is divided into two components, upper and lower. (For abbreviations see Box.)
Stenta (Reference Stenta1906) was the first to describe the pre-oral unpaired buccal gland in P. nobilis but thought it discharged its products into the mantle cavity. He also called it Poli's gland. Pelseneer (Reference Pelseneer1911, pp. 27–28, plate IX, figure 11) described and illustrated the gland for Pinna regia Reeve, 1858 (=Pinna bicolor Gmelin, 1791) and called it ‘la glande prebuccale’. Following Pelseneer, it was termed the pre-oral gland in Pinna attenuata Reeve, 1858 by White (Reference White1942) and the pallial gland by Yonge (Reference Yonge1953) both, presumably, following Stenta's belief that it discharged its products into the mantle cavity, as will be discussed.
Figure 9A is a detailed illustration of the buccal gland and mouth apparatus of Pinna nobilis as seen from the ventral aspect. The outer lip of the mouth is relatively small with the buccal gland partially hidden behind it. In contrast, the inner lip of the mouth is large and delicate. Although here illustrated in a pulled down direction, in life, with P. nobilis oriented vertically in the sediment, it must fall to essentially seal the buccal apparatus, that is, the mouth and the oral terminations of left and right proximal oral grooves. This means that any potential food item, either suspended particles, but now within a mucous-bound strings, plus any small epi- or endo-benthic or mesoplanktonic individuals arriving here are essentially trapped and will be passed into the oesophagus. Figure 9B represents a dissection of the dorsal region of the mouth just inside its outer lip and has revealed the presence of two small ducts (BUGD), <0.5 mm in width, leading from the buccal gland (BUG) and discharging via two minute pores (BUGP) into the dorsal surface of the oesophagus (CEO). The arrow in Figure 9A and B indicates how the ducts from the buccal gland are located behind the outer lip of the mouth. Because of the pleated nature of this area of the mouth and oesophagus (O), these ducts and pores are extremely difficult to detect, accounting for why Stenta (Reference Stenta1906) did not see them, as neither did White (Reference White1942) nor Yonge (Reference Yonge1953).

Fig. 9. Pinna nobilis. A, A detail of the buccal gland and mouth apparatus as seen from the ventral aspect. B, A dissection of the mouth showing how the ducts from the buccal gland enter into the dorsal surface of the oesophagus. (The arrow indicates how the ducts from the buccal gland are located behind the outer lip of the mouth.). C, Atrina pectinata. A detail of the buccal gland and mouth apparatus as seen from the ventral aspect. (For abbreviations see Box.)
Figure 9C is a detailed illustration of the buccal gland and mouth apparatus of A. pectinata as seen from the ventral aspect. The outer lip of the mouth is relatively small, as in P. nobilis but, unlike this species, the buccal gland of A. pectinata is considerably smaller in comparison and appears only as a narrow band just visible behind it. As in P. nobilis, however, the inner lip of the mouth of A. pectinata is relatively large and delicate and, in life, it too must fall close to the buccal apparatus and thereby also entrap any captured food items.
In transverse section the buccal gland of P. nobilis is a complex structure Figure 10A and is enclosed within a cuboidal outer epithelium (OE) beneath which is a sub-epithelial layer of amorphous tissues infiltrated by numerous vacuoles (SE). The greater part of the gland comprises an outer layer of highly convoluted primary tubules (PT). These discharge their secretory products into a ramifying assemblage of secondary tubules (ST) that then collectively open into the two ducts (BUGD). Figure 10B is a more detailed illustration of an outer primary tubule (PT) beneath the outer epidermis (OE) and sub-epithelial layer (SE) showing how the entire structure is glandular and comprises what are believed to be mucous cells (MC) interspersed by vacuolated (presumably discharged mucous) cells. These cells are illustrated in greater detail in Figure 10C. The mucous and vacuolated cells are both up to 10 µm tall and the former have a densely staining apical surface that are possibly microvilli (MI), although this needs confirmation.

Fig. 10. Pinna nobilis. A, A section through the buccal gland with B and C showing the outer epithelium and a single primary tubule, and the cells of the primary tubule, respectively, in greater detail. (For abbreviations see Box.)
Figure 11A is a transverse section though a secondary buccal gland tubule of P. nobilis showing its acidic polysaccharide (AMC) and neutral polysaccharide (NMC) cells. Both cell types are some 50 µm in height and are shown in greater detail in Figure 11B. Figure 11C is a transverse section through one of the buccal gland ducts with a detail of the duct epithelium illustrated in Figure 11D. The paired buccal gland ducts are ~500 µm in width and ~230 µm in depth, that is, they are dorso-ventrally flattened. The duct epithelium comprises tall (up to 60 µm) darkly staining ciliated cells (CC) interspersed by non-ciliated vacuolated cells (VC) of the same height. The cilia are some 20 µm long and the lumen of the duct is partially filled with an amorphous secretion (SLT) almost certainly derived from either the primary or secondary tubules, or both.

Fig. 11. Pinna nobilis. A, A section though a secondary buccal gland tubule with B, acidic polysaccharide and neutral polysaccharide cells shown in greater detail. C, A section through one of the buccal gland ducts with D, a detail of the duct epithelium. (For abbreviations see Box.)
The secretory epithelia of the primary and secondary tubules of P. nobilis are shown in greater detail in Figure 12A and B, respectively. Here, the acidic polysaccharide (AMC) and neutral polysaccharide (NMC) cells are clearly differentiated as a consequence of their respective staining properties, that is, deep blue and pale red, respectively.

Fig. 12. Pinna nobilis. Histological illustrations of A, the primary tubules and B, the secondary tubules showing their secretory characteristics. (For abbreviations see Box.)
The stomach
The stomachs of Atrina vexillum (Born, 1778) and Pinna atropurpurea G.B. Sowerby I, 1825 have been described and illustrated by Purchon (Reference Purchon1957, figures 3 and 4, respectively). The internal architecture of the stomach of P. nobilis is illustrated in Figure 13 as seen from the right side following an incision made along its right lateral axis. As in those taxa described by Purchon, into the stomach of P. nobilis projects a large solid crystalline style (CS), which arises from the crystalline style sac that is conjoined with the mid gut (CSS/MG). Into these apertures pass the major typhlosole (MAT) and the minor typhlosole (MIT). The crystalline style rotates against a relatively small gastric shield (GS) located in the dorsal region of the stomach and which is characterized by an indentation into which the head of the crystalline style fits. This dorsal region of the stomach is also characterized by two openings that Purchon (Reference Purchon1957) termed the dorsal hood (DH) and left pouch (LP).

Fig. 13. Pinna nobilis. A dissection of the stomach, as seen from the right side, showing the principal anatomical features of its interior. (For abbreviations see Box.)
The principal anatomical features of the stomach of P. nobilis described above thus find accord with those taxa described by Purchon but differ in a number of ways, as follows. The stomach of P. nobilis has six openings into the digestive diverticulae (Figure 13, DDD1–3), divided approximately into three groups of two whereas the two species described by Purchon (above) have three and two, respectively. Although each taxon has only one large sorting area associated with the major typhlosole and its intestinal groove (MAT + IG), the sorting area (SA) associated with them is much larger in P. nobilis than those species described by Purchon. There is also a second sorting area (SA) related to the opening of the oesophagus (O) into the stomach. Possibly most importantly, however, is that the stomach of P. nobilis is characterized ventrally by a large, basin-shaped, pouch (BP) that is not typical of the other two species.
The stomach contents
Each stomach of the 10 freshly collected, early morning, individuals of P. nobilis contained whole animals, that is, recognizable species, material and fragments of crushed dead taxa perhaps captured during the previous night's feeding activities. Figure 14A–O are illustrations of the mesozooplanktonic and epi- and endo-benthic species recovered from the stomachs of the 10 individuals of P. nobilis. Collectively, these suggest that P. nobilis can obtain and ingest taxa within the 200–2200 µm size range (Table 1). Fragments of mesophytoplankton were also observed occasionally and in small numbers (Puljas, personal observations).
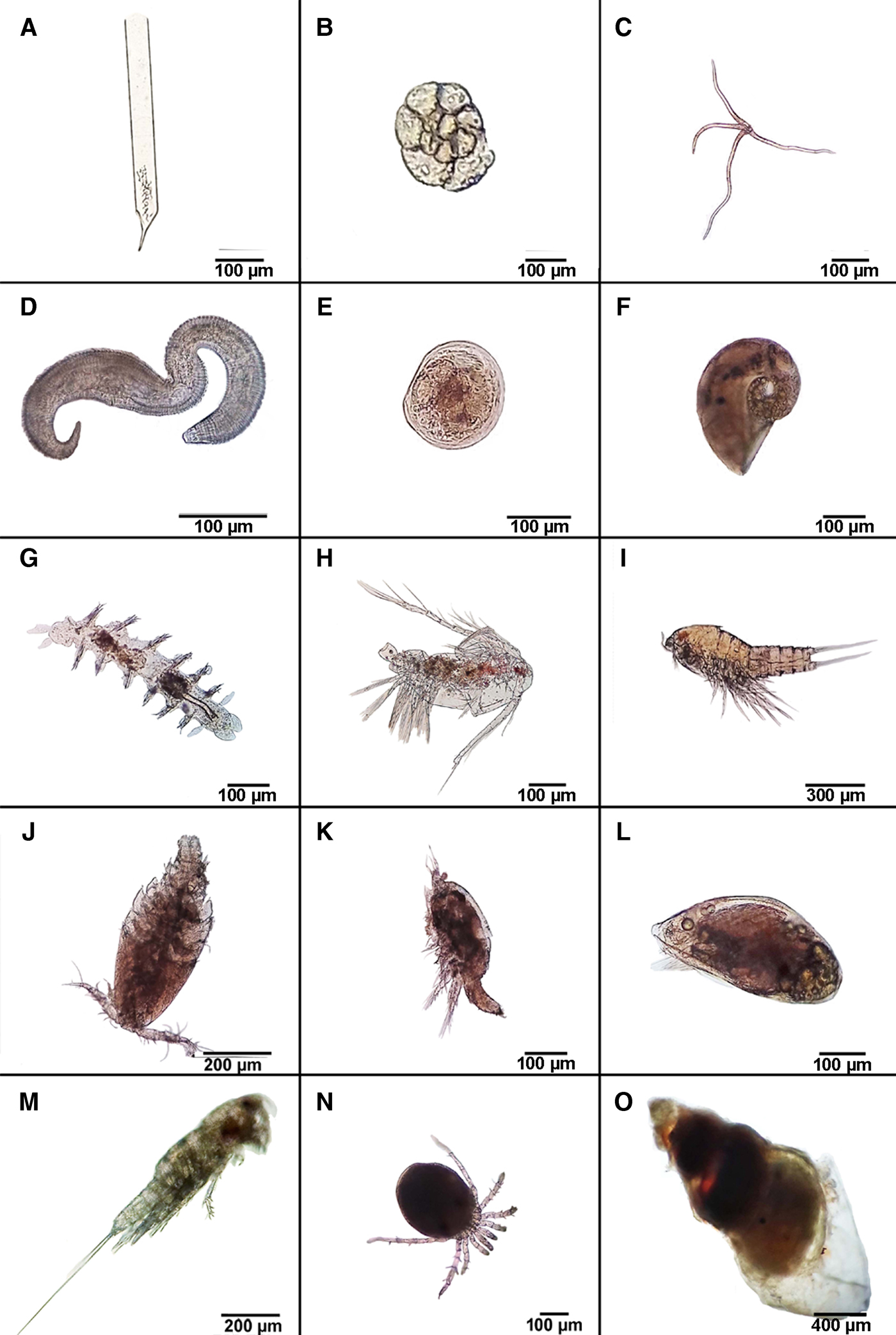
Fig. 14. A–O, Illustrations of the mesoplanktonic and endo- and epi-benthic and planktonic species recorded from the stomachs of 10 freshly collected early-morning individuals of Pinna nobilis from Kaštela Bay, Adriatic Sea, Croatia (see Table 1).
Table 1. A list of mesoplanktonic and epi- and endo-benthic species recorded from the stomachs of 10 freshly collected individuals of Pinna nobilis obtained from Kaštela Bay, Adriatic Sea, Croatia (see Figure 14).

Of the 10 investigated stomachs of P. nobilis, the contained mesozooplanktonic species reflected the estuarine environment of a community typical of the channels in the middle and eastern Adriatic Sea (Vidjak et al., Reference Vidjak, Bojanić, Kušpilić, Marasović, Ninčević Gladan and Brautović2006, Reference Vidjak, Bojanić, Kušpilić, Grbec, Ninčević Gladan and Tičina2007, Reference Vidjak, Bojanić, Kušpilić, Grbec, Ninčević Gladan, Matijević and Brautović2009; Kršinić, Reference Kršinić and Marguš2007; Viličić, Reference Viličić2014). The stomach contents included tinntinids, foraminiferans, cnidarian larvae and bivalve larvae, gastropods, calanoid and harpacticoid copepods, cyprid larvae and unidentifiable eggs. Also identified from the stomachs were epi- and endo-benthic species including cosmopolitan representatives of epsilonematid nematodes (Decraemer et al., Reference Decraemer, Gourbault and Helléouet2001), polychaetes, gastropods (Pyramidellidae, Naticidae) and mites (Acarina) (Pešić et al., Reference Pešić, Sezgin, Karaçuha and Ürkmez2013) (Figure 14, Table 1). The average species size overall was 626 µm. If only planktonic taxa are considered, an average size of 530 µm was obtained whereas for epi- and endo-benthic species, the average size was 820 µm.
DISCUSSION
Save for giant clams (Tridacnidae), P. nobilis is among the largest of marine bivalves and able to reach a maximum shell length of one metre (Poppe & Goto, Reference Poppe and Goto1993). Like all pinnids, P. nobilis is actually a relatively shallow burrower, that is, the pointed anterior end of the shell is held in place in the sediment by an exceedingly large byssus comprising numerous, albeit delicate, threads. These are attached to stones and shell debris by the long foot. Most burrowing bivalves live with their posterior shell margin close to the surface sediment-water interface and are virtually completely enclosed by sand and mud particles and thus largely invisible. In contrast, the posterior region of the shells of most pinnids sit proudly above the sediment-water interface. And this is especially true of P. nobilis. The first question then is: why are representatives of the Pinnidae so different from the typical burrowing bivalves and, in particular, P. nobilis? We will attempt to answer this question after first discussing the results of this research.
Function and behaviour of the pallial organ
We consider that the pallial organ of P. nobilis functions as a disinfecting swab as suggested for A. atropurpurea by Liang & Morton (Reference Liang and Morton1988). When shell cleansing is undertaken, blood is pumped from the main body into the haemocoel of the pallial organ, which then extends. Once extended to its full length, the sphincter at the base closes by contraction of the circular muscles within it, thereby creating a more solid swab at the organ's apex – the head. The swab can then be wiped over the rim of the shell by selective adjustment of the contractile state of the retractor muscles surrounding its central haemocoel. Once cleansing is completed, the sphincter relaxes and blood within the pallial organ flows back into the body's haemocoelic spaces. This is not to say that the pallial head can clean all the shell, in fact, individuals examined in this study were often colonized by small spirorbid and serpulid polychaetes, but always further anterior to the posterior valve margins where presumably the pallial organ cannot reach. Similarly, the organ does not stop the gastrochaenid bivalve Rocellaria dubia (Pennant, 1777) from settling on the shell of P. nobilis and boring into it (Morton et al., Reference Morton, Peharda and Petrić2011).
The discovery of mesoplanktonic and endo- and epi-benthic marine species in the investigated stomachs of P. nobilis may be accounted for, in part at least, by being either stunned or even killed by coming into contact with the extended, sulphuric acid-secreting pallial organ. If so, then such individuals would be taken into the mantle cavity within the strong inhalant stream and join with any suspended material also so collected. Both would thence be transported by the ctenidia towards the labial palps, the proximal oral grooves and eventually the mouth.
Function of the buccal gland
As noted earlier, what is here termed the buccal gland, because of its close association with the mouth and oesophagus of P. nobilis, was originally termed the pallial gland (Yonge, Reference Yonge1953). As this study demonstrates, moreover, the structure is indeed glandular as suggested by previous investigators (Stenta, Reference Stenta1906; Grave, Reference Grave1911; Pelseneer, Reference Pelseneer1911; Yonge, Reference Yonge1953; Liang & Morton, Reference Liang and Morton1988). White (Reference White1942, pp. 53–54, figures 12A–C), in particular, however, followed Stenta (Reference Stenta1906) in suggesting (bottom, p. 53; top, p. 54) that the gland discharged into the infrabranchial chamber of the mantle cavity but then said it opened into the epibranchial chamber (bottom, p. 54). In neither case is this correct, however, and this study has identified a pair of minute ducts leading from the gland and discharging into the oesophagus.
Staining with Alcian blue/PAS has demonstrated secretory activity in the buccal gland for acidic and neutral mucous polysaccharides, which probably produce mucous-rich saliva for the agglutination of food particles. The explanation for the presence of an acidic mucopolysaccharides secretion, that is, glycosaminoglycans from the buccal gland, is that they may also play a role in digestion. Perhaps either lowering the pH of the stomach's secretions and thereby optimizing enzymatic activity therein or joining with sulphuric acid from the pallial organ in dissolving and pre-digesting prey items protected by a cuticle or a calcareous shell (or both).
The stomach
Little attention has been paid to the effect of bivalves in general and P. nobilis in particular, on mesozooplankton populations, other than indirectly through competition for phytoplankton resources (Davenport et al., Reference Davenport, Smith and Packer2000). Zooplanktonic species have, however, been reported from the stomachs of scallops and P. nobilis (Mikulich & Tsikhno-Lukanina, Reference Mikulich and Tsikhno-Lukanina1981; Shumway et al., Reference Shumway, Selvin and Schick1987; Davenport et al., Reference Davenport, Ezgeta-Balić, Peharda, Skejić, Nincevi, Ninčević-Gladan and Matijević2011) with indications that digestion had taken place. According to Davenport et al. (Reference Davenport, Smith and Packer2000), the mussel Mytilus edulis Linnaeus, 1758 can capture and ingest zooplanktonic and benthic animals although earlier researchers predicted that such inhaled species would be expelled in pseudofaeces. Davenport et al. (Reference Davenport, Smith and Packer2000, Reference Davenport, Ezgeta-Balić, Peharda, Skejić, Nincevi, Ninčević-Gladan and Matijević2011) concluded that the benthic/benthonic species identified from the stomachs of M. edulis and P. nobilis were typical associates of shallow subtidal algae and seagrass beds and would thus also occur on the shells of the bivalves they investigated. This study confirms this observation with regard to P. nobilis. That is, in addition to being a ctenidial suspension feeder on seston and phytoplanktonic cells in the water column, as in many bivalves, P. nobilis can also collect mesozooplanktonic taxa and capture the epi- and endo-benthic species that are typical of its habitat.
Interestingly, based on similar stable isotope carbon measurements, Kennedy et al. (Reference Kennedy, Richardson, Duarte and Kennedy2001) concluded that the commensal pinnotherid Pontonia pinnophylax (Otto, 1821) assimilated similar food to its bivalve host P. nobilis. Further, and based on the form of the mandibles in the palaemonoids examined by Ashelby et al. (Reference Ashelby, De Grave and Johnson2015), six types appear to relate to an individual taxon's feeding mode and/or diet. Pontonia pinnophylax has Type A mandibles that are also typical of the largely carnivorous palaemonid Palaemon macrodactylus Rathbun, 1902. Although the exact diet of P. pinnophylax is unknown, its apparently carnivorous mandibular type might be associated with the herein hypothesized mixed diet of P. nobilis and, if so, is supportive of such an hypothesis.
Comparisons with other bivalve predators
The great majority of all bivalves are either suspension or deposit feeders (Morton, Reference Morton and Taylor1995). This is not true however for the deep sea where a diverse assemblage of usually small anomalodesmatan bivalves broadly and colloquially referred to as the Septibranchia are obligate carnivores. In direct contrast to P. nobilis, at up to one metre in shell length, most representatives of the Septibranchia have lengths of only millimetre proportions. For example, the tiny Spheniopsis brasiliensis Machado & Passos, 2015 has a shell length of <2.6 mm (Morton, Reference Morton2016) while the smallest carnivorous bivalve known to date, Grippina coronata Machado & Passos, 2015, has a shell length of <2 mm (Morton et al., Reference Morton, Machado and Passos2015). Both have been shown to prey on epipsammic micro-crustaceans. In addition, although the majority of carnivorous anomalodesmatans have been recorded from the deep sea floor, more recent researches have shown the presence of such predators in shallower waters, such as, for example, G. coronata (21–53 m) and Cardiomya cleryana (d'Orbigny, 1842) (10–20 m) (Morton et al., Reference Morton, Machado and Passos2016; Machado et al., Reference Machado, Morton and Passos2017). Such anomalodesmatans have a wide variety of specialized morphological adaptations that facilitate prey capture, ingestion and digestion and, thus, an exclusively carnivorous diet on the sea floor.
A second example of a deep-sea predatory bivalve is Propeamussium lucidum (Jeffreys in Wyville-Thomson, 1873) (Pectinoidea), and presumably its congenerics, which feeds largely on tiny crustaceans (Morton & Thurston, Reference Morton and Thurston1989). This species has a specialized buccal pouch formed from the reduced labial palps and outer lip of the mouth and the foot is used to push captured prey items into it. The stomach is also specialized morphologically for the digestion of contained prey items, which include, from an analysis of its contents, foraminiferans, various larvae and either whole or the remains of harpacticoid copepods.
In contrast to these, often miniature, obligate deep-sea predatory bivalves, more shallow-water species such as the intertidal suspension-feeding Cerastoderma edule (Linnaeus, 1758) also predates settling conspecific larvae (André et al., Reference André, Jonsson and Lindegarth1993). These authors estimated that mean survival time for competent C. edule larvae drifting over sediment populated by feeding adults (380 ind. m−2) was 64 s and 75% of them were inhaled. Similarly, the pectinid Placopecten magellanicus (Gmelin, 1791) and the mytilid M. edulis have been reported to be at least partially carnivorous (Shumway et al., Reference Shumway, Selvin and Schick1987; Davenport et al., Reference Davenport, Smith and Packer2000). In the case of the former, such an occurrence must be regarded as ‘accidental’ because representatives of the Pectinidae (and Limidae) have been shown by Gilmour (Reference Gilmour1964), in the context of the present research, to possess a complex lip apparatus that would generally prevent the ingestion of such large mesozooplanktonic and epi- and endo-benthic individuals. In the case of the latter, however, Davenport et al. (Reference Davenport, Smith and Packer2000) and Lehane & Davenport (Reference Lehane and Davenport2002) identified molluscan eggs, trochophores and veligers (41%), barnacle zoeae, cyprid larvae, rotifers, cladocerans, calanoid copepods (38%) and polychaete larvae, harpacticoid copepods, fish eggs, protists, mites, bryozoan larvae, the zoeae of Carcinus maenus (Linnaeus, 1758), nematodes, amphipods, a flatworm and an echinoderm larva (21%) in their stomachs. Although such a compendium of species is similar to that recorded herein from the stomachs of P. nobilis, these prey taxa are, however, we believe, and like C. edule described above, also ‘accidental’ captures. This is because the anatomically well known M. edulis (White, Reference White1937), again as with C. edule, has no specialized structural means of processing prey other than the smallest of individuals accompanying inhalations of seawater into the mantle cavity. Further, Defossez & Hawkins (Reference Defossez and Hawkins1997) demonstrated that M. edulis preferentially rejected larger inhaled particles from the mantle cavity as pseudofaeces. We thus preclude the notion that such species are truly carnivorous – only incidentally so.
With regard to P. nobilis, however, in addition to the small mesoplanktonic zooplankters and epi- and endo-benthic individuals identified herein from the stomachs of this fan shell, Davenport et al. (Reference Davenport, Ezgeta-Balić, Peharda, Skejić, Nincevi, Ninčević-Gladan and Matijević2011, p. 252) also concluded for this species that ‘It is possible that some benthonic harpacticoids were intercepted when travelling between benthic plants, but given the numbers (and the quantities of detritus ingested) it is far more likely that they were incidentally ingested with inhaled detritus.’ The present authors do not concur with the use of ‘incidental’ to describe this particular predator–prey relationship, since our findings indicate that the digestion of such animals takes place and is initiated by secretions emanating from the buccal gland. Further, this study has identified features of the stomach such as the capacious basal pouch that may accommodate relatively large prey items.
Before defining the predator–prey relationship identified herein for P. nobilis, another such relationship identified for the wood-dwelling Idas argenteus Jeffreys, 1876 (Ockelmann & Dinesen, Reference Ockelmann and Dinesen2011) has to addressed. These authors demonstrated, despite being unable to determine the structure of the intestine due to their study material's long-term preservation, that I. argenteus had large numbers of the early spat of predominantly Xyloredo ingolfia Turner, 1972 in its stomach and hind gut. This species shares the same pieces of wood as I. argenteus on the deep sea floor and, thus, such an occurrence could also be construed as simply ‘accidental’. Moreover, Thubaut et al. (Reference Thubaut, Corbari, Gros, Duperron, Couloux and Samadi2013) showed that Idas iwaotakii (Habe, 1958) possesses extracellular symbiotic bacteria in its ctenidia and only identified one foraminiferan test from within one individual's stomach thereby suggesting that such captured ‘prey’ are not significant elements of its normal diet.
With regard to the predatory activities of P. nobilis, however, we prefer the term ‘opportunistic’ as this species has in the structure and function of the buccal gland and the stomach the potential means to initiate and complete, respectively, the digestion of such captured prey items. Pinna nobilis is thus not simply an ‘accidental’ or ‘incidental’ predator of mesozooplanktonic and epi- and endo-benthic species but is, on the basis of evidence presented in this paper, an ‘opportunistic’ predator. It sits vertically with the posterior shell margin well clear of the sediment-water interface and clearly captures epi- and endo-benthic taxa crawling up its shell and which, possibly, face the prospect of being stunned (at least) by the sulphuric acid-producing pallial organ. Conversely, mesozooplanktonic individuals and pelagic larvae may be captured by the strong inhalant stream of such a large bivalve.
Both types of potential prey are transported towards the mouth by the ctenidial food grooves and within the proximal oral grooves. In this context it is perhaps significant that these grooves in P. nobilis are double channelled such that with only the pleated upper channel open, finer particles are transported within it to the mouth. For the transport of larger captured epi- and endo-benthic and mesoplanktonic taxa, however, both channels are presumably opened. At the mouth, such prey items are ingested and subjected initially to secretions produced by the uniquely pinnid buccal gland and are then transported to the stomach for further digestion.
But why is the buccal gland of Pinna nobilis so large in comparison with the other pinnids described by previous authors and in the case of A. pectinata described herein? The waters P. nobilis inhabits are geochemically impoverished with relatively low nutrient concentrations and are characterized by a general west-to-east gradient of increasing oligotrophy (Durrieu de Madron et al., Reference Durrieu de Madron, Guieu, Sempéré, Conan, Cossa, D'Ortenzio, Estournel, Gazeau, Rabouille, Stemmann, Bonnet, Diaz, Koubbi, Radakovitch, Babin, Baklouti, Bancon-Montigny, Belviso, Bensoussan, Bonsang, Bouloubassi, Brunet, Cadiou, Carlotti, Chami, Charmasson, Charrière, Dachs, Doxaran, Dutay, Elbaz-Poulichet, Eléaume, Eyrolles, Fernandez, Fowler, Francour, Gaertner, Galzin, Gasparini, Ghiglione, Gonzalez, Goyet, Guidi, Guizien, Heimbürger, Jacquet, Jeffrey, Joux, Le Hir, Leblanc, Lefèvre, Lejeusne, Lemé, Loÿe-Pilot, Mallet, Méjanelle, Mélin, Mellon, Mérigot, Merle, Migon, Miller, Mortier, Mostajir, Mousseau, Moutin, Para, Pérez, Petrenko, Poggiale, Prieur, Pujo-Pay, Pulido-Villena, Raimbault, Rees, Ridame, Rontani, Ruiz Pino, Sicre, Taillandier, Tamburini, Tanaka, Taupier-Letage, Tedetti, Testor, Thébault, Thouvenin, Touratier, Tronczynski, Ulses, Van Wambeke, Vantrepotte and Vaz2011). We therefore hypothesize that in the particular environment of the Mediterranean and Adriatic Seas, the locally endemic P. nobilis, in addition to being a typical ctenidial suspension feeder, has become, albeit improbably, an opportunistic predator.
SUMMARY
In support of the argument for Pinna nobilis being an opportunistic predator of mesoplanktonic and small epi- and endo-benthic taxa, what follows is a summary of the anatomical features that may facilitate such carnivory.
1. As well as functioning as a cleaning swab, the uniquely pinnid pallial organ may, using its sulphuric acid secretions, immobilize, if touched, any invertebrates approaching the exposed shell margin either via the strong inhalant stream or by crawling up the shell.
2. The proximal oral grooves are large and uniquely dorso-ventrally doubled so that the upper pleated channel is used to transport inhaled and pro-selected suspended seston to the mouth. When larger, mucous-bound, prey items are captured, however, both channels can be opened to accommodate them.
3. Glycosaminoglycans produced from the uniquely pinnid buccal gland may also play a role in digestion, perhaps either lowering the pH of the stomach's secretions and/or pre-digesting prey items.
4. The stomach is unusually capacious to hold such captured prey.
5. Thirteen species of mesoplankters and small epibenthic taxa plus the larvae of bivalves and barnacles have been identified from the stomachs examined.
ACKNOWLEDGEMENTS
The authors are grateful to the Ministry of Environment and Energy of the Croatian Government, Radňicka cesta 80, Zagreb, Croatia, for permission to collect the individuals of Pinna nobilis for the purposes of this study and reported upon herein. We are also grateful to Dr K.F. Leung, Environmental Protection Department of the Hong Kong SAR Government for providing specimens of Atrina pectinata for comparison with Pinna nobilis.